- 1Central Agricultural University, Imphal, India
- 2Division of Agronomy, Indian Council of Agricultural Research-Indian Agricultural Research Institute, New Delhi, India
Soil is an important natural resource providing water, nutrient, and mechanical support for plant growth. In agroecosystem, continuous manipulation of soil is going on due to addition of input, removal of nutrients, changing water balance, and microbial life. These processes affect soil properties (physical, chemical, and biological), and the deviation of these properties from the normal status is controlled by soil buffering capacity and soil resilience. If these changes are beyond the reach of soil resilience, then soil loses its original state, leading to soil degradation. At present, the extent of the degraded area in the world is 1,036 to 1,470 million ha. This urges the need for maintaining soil health rather than the mere addition of input for crop production. Soil health is an integrative property that reflects the capacity of soil to respond to agricultural intervention, so that it continues to support both agricultural production and the provision of other ecosystem services. Maintaining the physical, chemical, and biological properties of soil is needed to keep it healthy, and this is possible through the adoption of different agronomic approaches. The diversification of nutrient sources with emphasis on organic sources, adoption of principles of conservation agriculture, enhancement of soil microbial diversity, efficient resource recycling through the integrated farming system, and amendment addition for correcting soil reactions are potential options for improving soil health, and are discussed in this review. This article reviewed the concept of soil health and its development, issues related to soil health, and indicators of healthy soil. At the same time, the impact of the ill health of the soil on crop productivity and resource use efficiency reported in different parts of the world in recent years are also reviewed. The agro-techniques such as green and brown manuring in arable land and agroforestry on degraded and marginal land were followed on piece meal basis and for economic gain. The potential of these and several other options for maintaining soil need to be recognized, evaluated, and quantified for their wider application on the front of soil health management avenues. The use of crop residue, agro-industrial waste, and untreated mineral or industrial waste (basic slag, phosphogypsum, etc.) as soil amendments has a huge potential in maintaining healthy soil along with serving as sources of crop nutrition. The review emphasizes the evaluation and quantification of present-day followed agro-techniques for their contribution to soil health improvement across agro-climatic regions and for wider implications. Furthermore, emphasis is given to innovative approaches for soil health management rather than mere application of manures and fertilizers for crop nutrition.
Introduction to Soil Health
The soil, supplier of water, nutrient, and mechanical support to crop plants, is explained as four-dimensional, unconsolidated, and dynamic in nature (Lal, 2016). The major components of the soil system consist of mineral matter, which acts as an inherent source of 14 essential mineral plant nutrients and organic matter, which acts as a storehouse (Elixir). Soil also supplies essential mineral plant nutrients along with carbon and pore space occupied by water and air supplying three basic non-mineral plant nutrients viz., carbon (C), oxygen (O), and hydrogen (H). In the ideal state, the proportions of these factors are 45% mineral matter and 5% organic matter; while the remaining 50% is occupied by pore space. This four-dimensional nature and distinct proportions of solid and pore space give soil distinct physical, chemical, and biological properties that change over time dimensions. Any significant variation in these factors beyond the range of crop tolerance limits makes soil unfit for crop cultivation and will be the most important reason for soil illness. The tolerance limit for plant growth is expressed as the different parameters that express the physical, chemical, and biological properties of the soils; while the soil with all properties in the acceptable range is considered healthy.
Soil health is defined by various authors in different ways because of the involvement of a large number of soil health indicators (Van Bruggen and Semenov, 2000; Nielsen and Winding, 2002; Brevik, 2009; Katyal et al., 2016; Haney et al., 2018; Wander et al., 2019) and their suitable combination for different land use systems. Definitions given by different authors and organization are shown in Table 1. The concept of soil health started with the use of the term “soil health” by Wallace (1910) in regard to the capacity of humus to provide a solution to almost all soil-related problems and the major historical development of the concept of soil health (Brivik, 2018) is shown in Table 2. As different soil properties are considered in explaining the concept of soil health, and act as indicators of soil health, it can also be defined in terms of soil properties viz. soil physical health, soil chemical health, and soil biological health. The soil with the ability to meet plant and ecosystem requirements for water, aeration, and strength over time, and to resist and recover from processes that might diminish this ability is considered as physically healthy (McKenzie et al., 2011; Are, 2019). Soil biological health is the ability of soil to support large and diverse microbial communities, suppress pathogens, and support healthy crop development (Brackin et al., 2017); while chemically healthy soil has plant nutrients in optimum quantity, available form, and balanced proportions, and which are available to plants without the hindrance of other chemical compound and properties. Soil chemical health also considers the presence or absence of harmful soil agrochemicals and pollutants.
Considering the variety of chemical, physical, and biological properties of soils, there were attempts to categorize some soil properties as indicators of soil health (Magdoff, 2001; Brevik, 2009; Cardoso et al., 2013; Haney et al., 2018; Pawlas et al., 2019), which are mentioned in Table 3. Along with soil health indicators, Magdoff (2001) listed the characteristics of healthy soil (Table 4). The importance of soil health in sustaining the agricultural ecosystem is well-recognized (Wienhold et al., 2008; Jat et al., 2015; NAAS, 2018; Jian et al., 2020; Tahat et al., 2020), and considering the varied levels of sensitivity of soil health indicators (Table 3), it is imperative to discuss the different issues and concerns of soil health.
Issues Related With Soil Health
Factors that cause deviation of healthy soil are issues related with soil health, and the level of impact of these factors on soil health decides their order of significance and make them a concern. Studies on these issues are important because of the following reasons:
• The health of soil has a direct influence on the sustainability of agro-ecosystems, as soil is a feeding substratum for all types of vegetation.
• Healthy soil will be more resilient to extreme weather phenomenon (drought, flood, etc.) and frequency of these phenomenons is expected to increase on the front of climate change (Mirzabaev et al., 2019; Olsson et al., 2019).
• A healthy soil should provide more ecosystem services such as biogeochemical cycling of nutrients, enhanced microbial population, and diversity (Costanza et al., 1997; Baveye et al., 2016).
• Maintaining soil health contributes to the sustainable development goals of the United Nations, such as alleviating poverty, reducing hunger, improving health, and promoting economic development (Lal, 2016).
• Maintaining soil health is now almost important for enhancing crop productivity because of the occurrence of multi-nutritional deficiency in soil (Rattan et al., 2009), increased soil degradation (Bhattacharyya et al., 2015), and accumulation of harmful pesticide residues in soil that adversely affect soil microorganisms (Meena et al., 2020).
• Maintaining soil health also contributes to carbon sequestration, as soil organic carbon is one of the most important criteria for soil health evaluation (Lal, 2016).
• Intensification of agriculture with imbalance in the use of artificial resources and less attention on the potential of natural resources adversely affects soil health.
Stakeholders, researchers, and policy planners have shown an increased attention for soil health management as proved by an increased rate of the adoption of conservation agriculture (Kassam et al., 2019), emphasis on organic farming, promotion of diversification in agriculture, development and adoption of land use classification (USDA, 1961; Grose, 1999), and adoption of farming system-based approach rather than using cropping system alone. Policies/schemes such as soil health card schemes also address one or more soil health-related issues (Wienhold et al., 2008; Anonymous, 2011; Islam et al., 2017; Reddy, 2017). Terms mainly used to describe degraded soil health are land degradation, soil degradation, soil desertification, and soil pollution. Land degradation is the loss of actual or potential productivity or utility as a result of natural or anthropogenic factors. It is a decline in land quality or a reduction in land productivity (Eswaran et al., 2001); while IPCC (Olsson et al., 2019) define land degradation as a native trend in land condition, caused by direct or indirect human-induced processes such as anthropogenic climate change, expressed as long-term reduction or loss in at least one of the following: biological productivity, ecological integrity, or value to humans. Soil degradation is considered as a subset of land degradation (Olsson et al., 2019), which directly affects soil and is defined as a decline in the productivity of soil through adverse changes in nutrient status, soil organic matter, structural attributes, and concentrations of electrolytes and toxic chemicals (Aulakh and Sidhu, 2015). The other term, soil desertification, is mainly related to the physical degradation of soil and is defined as land degradation in arid, semi-arid, and dry sub-humid areas, collectively known as drylands, resulting from many factors, such as human activities and climatic variations (Mirzabaev et al., 2019). The term soil pollution was defined as the build-up of persistence toxic compounds, chemicals, salts, radioactive materials, or disease-causing agents in soils, which have an adverse effect on plant growth and animal health (Okrent, 1999). In this section, issues of soil degradation are discussed separately in three headings viz. physical, chemical, and biological degradation of soil. This will help in addressing the wide variation in factors that needs to be taken into consideration while discussing the issues of soil degradation.
Soil Physical Degradation
Major processes that cause physical degradation in soil include water erosion, wind erosion, wave erosion, coastal erosion, soil crusting, compaction, and hardening (Saha, 2003; Karlen and Rice, 2015). At the same time, agricultural practices that cause soil physical degradation include increased tillage intensity, inappropriate timing of tillage, aerobic-anaerobic cycles of soil moisture status in intensive cereal-based cropping systems (rice-wheat cropping system; Chauhan et al., 2012), lower addition of bulky organic manures, and removal of all dry matter produced, making soil devoid of vegetation. Soil physical degradation is mainly caused by either loss of soil from the area or modification of soil physical properties without any accountable loss in soil from the area.
Loss of Soil From the Area
Among the above-mentioned processes, soil erosion is the most prominent cause of soil physical degradation. At a global level, the estimated area affected by land degradation is 19.65 million km2 (Obalum et al., 2017); while in India, the estimated area affected by soil erosion is 31.5 to 166.1 million ha (m ha) (Bhattacharyya et al., 2015) with total soil losses of 5,334 million tons year−1 (16.35 t ha−1year−1) (Dhruvanarayana and Babu, 1983; Aulakh and Sidhu, 2015). Bhattacharyya et al. (2015) reported that a 94-mha area is affected by water erosion and 9 mha by wind erosion; while Lal (2001) reported that the area affected by water erosion and wind erosion was 32.8 and 10.8 m ha, respectively. In the process of soil erosion, detachment and transportation of soil particles happen from one place to another. Dhruvanarayana and Babu (1983) reported that 29% of the total displaced soil is lost permanently to the sea. Agents causing soil erosion are water and wind; the erosion caused by the combined action of water and wind that prominently occurs along canals and river banks is called wave erosion. Factors that decide the rate of water erosion are rainfall characteristics (intensity, distribution, and frequency), soil erodibility, steepness and length of the slope, crop cultivation practices, special practices for erosion control, and the erosivity of an agent (water) that causes erosion. The relative significance of these factors are varied over time, and space dimension and soil erosion were calculated from these factors using the Universal Soil Loss Equation (USLE) given by Wishmeier and Smith (1960); Wischmeier and Smith (1978). Factors affecting wind erosion are soil cloddiness, surface cover, surface roughness, soil textural class, local wind factor, wind width factor, wind direction, and wind barrier, while the functional relationship of these factors and the calculation of soil losses by wind erosion were given by Woodruff and Siddoway (1965). Singh et al. (1992) made an attempt to locate e iso-erosion lines on the map of India and quantify the rate of soil erosion in different areas (Table 5). The loss of soil due to erosion, according to them, ranges from 5 to 80 Mg ha−1 year−1.
Modification of Soil Physical Properties
Crusting and compaction: Soil crusting is a surface phenomenon in which a hard thin layer of soil is formed on the surface of the soil. Valentin and Brasson (1997) defined soil crusting as the forming processes and the consequences of a thin layer at the soil surface with reduced porosity and high penetration resistance. In the formation of a soil crust, soil aggregates get broken down and the soil becomes more compact with less porosity (Manyevere et al., 2015). The properties of the soil are modified due to crust formation leading to (a) initiation or increase rate of erosion; (b) adverse effect on plant germination and crop growth and; (c) modification of water entry and movement. Another term used to describe soil crusting is surface sealing, and Morin (1993) defined surface sealing as the orientation and packing of dispersed soil particles that are disintegrated from soil aggregates because of the impact of rain drops. The types of crusts are structural, depositional, erosion, chemical, and biological (Valentin and Brasson, 1992; Morin, 1993; Pagliai and Stoops, 2010; Williams et al., 2018), and are defined as follows (Valentin and Brasson, 1992):
• Structural crusts: These are crusts that formed because of the in-situ arrangement of soil particles/aggregates without any lateral movement, and based on their morphology and formation process they are named as slaking crust, infiltration crust, coalescing crust, and sieving crust. The USDA natural resource conservation service defined soil structural crusts as relatively thin, dense, somewhat continuous layers of non-aggregated soil particles on the surface of tilled and exposed soils.
• Depositional crusts: In this type of crust, an external material is involved, and they are formed when the external material is carried by the flow of water settled after infiltration and evaporation of water.
• Erosion crusts: Erosion crusts consist of only a rigid, thin, and smooth surface layer enriched in fine particles (Valentin and Brasson, 1992).
• Chemical crusts: These are a type of crust formed because of the precipitation of chemicals or salts with surface sealing/hardening properties.
• Biological crusts: Formed because of colonization of different microorganisms forming community all around soil particles/aggregates. The distinctive characteristic of this type of crust is that it protects soil from erosion, and it contributes to soil organic carbon and nutrient accumulation (Belnap, 2005).
Mechanisms of crust formation
• Mechanical destruction of soil surface aggregates by raindrop impact (Le Bissonnais, 1996).
• Leaching of fine particles and their subsequent deposition in underlying pores.
• Compaction of soil surface to form a thin film that restricts both further entry of water and movements of fine particles in soil pores.
• Chemical dispersion of clay particles.
• Soil degradation due to intensive land use.
The formation of soil crust contributes to soil erosion and, ultimately, to soil degradation in one of the following ways:
• decreased hydraulic conductivity and infiltration rate (Nciizah and Wakindiki, 2015);
• loosening of soil aggregates, decrease in aggregate stability and, ultimately, disturbance in soil structure;
• increases the rate of runoff;
• and the deposition of eroded materials, which causes surface sealing.
Major soil and climatic conditions that promote soil crust formation:
• medium-textured soil;
• predominance of smectite, illite, and micaceous minerals;
• high exchangeable sodium percentage and low organic matter;
• in arid and semi-arid regions but also commonly occur in cultivated soils in other climates (Williams et al., 2018);
• and water content during a rainfall event.
Soil compaction: It is the state of land in which soil porosity decrease is accompanied by an increase in bulk density. Major reasons for soil compaction include: continuous tillage at same soil depths, higher traffic, continuous use of heavy machinery, tillage practices at improper moisture in the soil, and decreased addition of organic amendments. Soil compaction affects soil health similarly as that of soil crust in surface layer; while below soil surface layers, decreased porosity, increased bulk density, reduction in downward and lateral movements of water are the other important effects of soil compaction that negatively affect soil health parameters.
Soil desertification: Soil desertification is another type of land degradation whose impact not only limits soil health assessment but is also important from the point of view of climate change, food security, and economics (Anonymous, 2018b; Mirzabaev et al., 2019; Wijitkosum, 2020). The organization of a conference on desertification by the United Nations in 1977, the constitution of the United Nations Convention to Combat Desertification (UNCCD) in 1994, and soil desertification place in sustainable development goal number 15 also highlight the severity of the problem. The term desertification was used for the first time in a broader sense by Aubreville in 1949 (after Luvauden in 1927). It is defined as the type of land degradation in arid, semi-arid, or dry sub-humid areas caused by human activities and climatic variation; while Sterk and Stoorvogel (2020) considered it as land degradation in dry land areas. The conference on desertification by the United Nations described the phenomenon of desertification as “the diminution or destruction of the biological potential of the land, which can lead ultimately to desert-like conditions. It is an aspect of the widespread deterioration of ecosystems and has diminished or destroyed the biological potential (plant and animal production), for multiple use purposes at a time when increased productivity is needed to support growing populations in quest of development.” The most recent estimate (Le et al., 2014) cited in Sterk and Stoorvogel (2020) indicated that, a 1,470-million-ha area, which is 29% of the total dry land, is affected by one or the other types of desertification; while Sterk and Stoorvogel (2020) had an opinion that a 1,036-million-ha area, which is 20.5% of total dry land, is affected by some form of soil degradation. In India, an 82.34-million-ha area (Anonymous, 2018b) is affected by desertification and includes all areas affected by one or the other types of land degradation. The extent of desertification is mainly judged based on the world map of the status of human-induced soil degradation, which was developed by the Global Assessment of Soil Degradation project (GLASOD) and based on expert knowledge of soil degradation processes and their spread in a large number of countries. According to a United Nations environmental program (Middleton and Thomas, 1997), desertification is the outcome of the following activities:
• climatic factors (temperature, rainfall, etc.),
• overgrazing,
• deforestation,
• agricultural activities,
• overexploitation of vegetation for domestic use,
• and bio-industrial activities.
Desertification contributes to the degradation of soil health through the following:
• rapid loss of vegetative cover on the soil surface and decrease in soil organic carbon;
• facilitation of the movement of soil/sand from one place to another, leading to expansion of desert;
• increased susceptibility of soil to wind and water erosion;
• adverse effect on the microbial population and diversity in the soil;
• and variation in soil surface relief and topography due to physical movement of soil.
Waterlogging: It is the state of soil moisture at which soil is saturated with water (all soil pores filled with water) and also used to indicate raising groundwater to the surface level (Awad and El Fakharany, 2020). In India, waterlogging is one of the important reasons for soil degradation, and the area affected by water logging is 11.6 million ha (Roy Chowdhury et al., 2018). The type may be surface waterlogging in which excess water is seen above the soil surface, or a subsurface type in which excess water remains below the soil surface. Soil characteristics, climate (rainfall), and plant cover have a profound influence on waterlogging. The areas and conditions in which waterlogging occurs are listed as follows:
• areas with heavy rainfall (the intensity of rainfall plays a major role);
• over irrigation mainly found in canal command areas in India;
• areas along river banks because of expansion of agricultural land up to riverbanks (mainly during flood situation);
• low elevated land where the collection of water causes waterlogging;
• areas around water reservoirs because of seepage of water;
• low infiltration rate and hardpan formation, and the presence of chemical salts, such as sodium and its compound aggravate the problem of waterlogging.
Water logging affects soil health adversely in one of the following ways:
• disturbing soil physical health through reduced aeration, structural stability, and lowering down of soil temperature;
• reducing soil oxygen level, anaerobically decomposing soil organic matter, and accumulating toxic gases and other products of decomposition;
• Change in soil reaction along with losses in soil nutrients through leaching and overland flow.
• changing soil microbial population from aerobic to anaerobic or facultative aerobic, which leads to adverse effects on several microbial processes and biogeochemical cycling of nutrients;
• and unfavorably affecting soil tillage properties and making soil unsuitable for cultivation of most crops.
Soil Chemical Degradation
Soil chemical health gets more attention from both researchers and stakeholders because of its most direct and significant influence on agricultural productivity, growing need for external addition of amendments and nutrient sources, and the profound influence of soil chemical properties on modification of soil biological and physical health. The chemical degradation of soil is discussed under the following subsections:
• Reduction in soil carbon;
• Changes in soil reaction (acidification and sodification);
• Modification of soil mineral nutrient status (nutrient imbalance, multi-nutrient deficiency);
• Accumulation of toxic compound (agrochemicals);
• and Soil pollution.
Reduction in Soil Organic Matter
Soil is an important carbon pool at the global level, with 1,895–2,530 Pg carbon, which is two times as that of carbon present in the atmosphere and three times as that of biotic carbon pool. Out of total carbon in soil, 695–930 Pg is inorganic and 1,200–1,600 Pg is organic in nature (Sahoo et al., 2019). Among these two fractions, organic carbon is more important from a soil health point of view, and studies on factors that have a significant impact on soil organic carbon are also important considering the significant decrease in soil organic carbon in Indian soil (Reddy, 2017) and in world agricultural production systems (Song et al., 2005; Grace et al., 2006; Gardi et al., 2016; Wiesmeier et al., 2016; Blecourt et al., 2019). At the same time, as soil organic carbon serves as a source and storehouse of plant nutrients, it has a great role in crop production improvement along with its significance in soil health. The functions of soil organic carbon in soil health, crop productivity, and ecosystem services are given in Table 6.
Factors affecting soil organic carbon status: Wide variations in land use changes and crop husbandry across agricultural production systems, and sensitivity of soil organic carbon to these changes, are responsible for significant variations in the organic carbon content of soil. The soil organic carbon content was affected in three different ways viz., decrease in soil organic carbon, improvement due to fertility addition, and changes due to crop cultivation. The decrease in soil organic carbon is mainly due to land use changes caused by tillage in arable crops and types of crops grown if considered at the agro-ecosystem level. The impact of tillage on soil organic carbon can be seen by comparing the plow-based conventional tillage system, which is widely followed all over the world, with conservation tillage, which is currently getting momentum because of its several positive impacts on soil, plant, and water (Table 7). In fact, the adverse effect of the conventional plow-based tillage system on soil health was one of the reasons for the origin of conservation tillage. The breaking of soil aggregates, exposure of soil organic carbon to different types of degradation and decomposition, complete removal of dry matter produced by crops, burning of crop residue, dependence on inorganic fertilizers, mono-cropping of few crops, and less addition of organic nutrient sources are factors that intensify the decrease in soil organic carbon; while three principles of conservation agriculture (Kassam et al., 2019) counteract these adverse effects of conventional tillage. At the same time, the availability of a large array of selective herbicides, availability of machinery for sowing and subsoil placement of fertilizer, and increased interest at research and development front in the modification of nutrient release patterns from crop residues through different ways (Singh et al., 2009b; Swarnalakshmi et al., 2013; Choudhary et al., 2016; Gangaiah and Prasad Babu, 2016) also promote conservation tillage-based agriculture.
In regard to the effect of fertility addition and crop cultivation on soil organic carbon, the results from several long-term experiments will be the proof for the same (Reddy et al., 2017). Improvement in soil organic carbon due to the addition of an optimum dose of chemical fertilizers and the combination of chemical fertilizers with organic sources, and a decrease in soil organic carbon over the years due to cultivation of crops (Mandal et al., 2007), are the major findings of long-term experiments in India. The variation in soil organic carbon in permanent agriculture has a different pattern compared with the growing of arable crops (Bernardi et al., 2007; Ganeshamurthy et al., 2020) because of variation in the frequency of land disturbances. Along with this, the variation in carbon sequestration potential of crops (Ghosh et al., 2006; Brar et al., 2015) is another important factor affecting soil organic carbon in cultivated areas.
In land use change, bringing the marginal land under cultivation has an adverse effect on soil organic carbon; while the utilization of degraded land for agro-forestry or energy plantation will successfully maintain or enhance soil organic carbon. Another land use change that significantly affects soil organic carbon content and most prominent innorth east India is shifting cultivation (Bhuyan, 2019). The clearing of natural vegetation and bringing the land under cultivation reduce soil organic carbon (Sharma et al., 2019).
Change in Soil Reactions (Acidification and Sodification)
The study on soil reactions for their effect on soil chemical health is also important because of the following reasons:
• Mineral nutrient availability is affected by soil reactions.
• Soil properties such as cation exchange capacity, base saturation, chelation of micronutrients, and anion exchange capacity, are responsible for the retention and movement of nutrients in the soil. These properties change with a change in soil reaction.
• Soil physical properties such as aggregation and erodibility are also affected by a change in soil reactions. Mineral elements such as sodium have a significant impact on soil aggregation and their presence in soil is controlled, to a large extent, by soil reactions.
• The relative proportion of different forms of mineral nutrients present in soil and inter-convergence is affected by soil reactions.
• The biogeochemical cycling of nutrients and the role of microorganisms in it are also modified with changes in soil reactions.
A soil reaction near neutral pH is mostly suitable for the cultivation of crops and different properties of soil; while an abnormal change in soil reactions affects soil chemical health. Changes in soil reactions due to human-induced changes in soil, water, and plant are observed at a very slow rate because of buffering capacity of soil and predominance of soil mineral matters (occupying 45% of the total soil volume) in deciding soil reactions. Environmental factors that cause changes in soil reactions include:
• weather factors, mainly rainfall patterns and temperature (causes leaching and erosion of soil mineral and organic matter);
• climatic factors intensify weathering which creates changes in soil parent materials;
• and topographical factors, topography of surface, and presence or absence of vegetation on the soil surface.
Human-induced changes in soil pH are mainly caused by the application of amendments for improving soil properties (liming or gypsum application), fertility addition through organic and inorganic sources of nutrients, and changes in land use (Mishra et al., 2006b). The effect of both the natural- and human-induced factors on the pH of the soil is conditioned by time. Major reactions that make soil chemically unfit for agriculture include the following:
• Acidification: It is the process of decreasing soil pH to such an extent that the soil becomes unfit for cultivation, and it is caused by both natural- and human-induced processes. Major natural processes causing acidification include acid rain, application of acid-forming fertilizers, mineralization of organic matter, nutrient uptake by roots, root exudates, and nitrogen fixation by legumes (Goulding, 2016). Soil acidification adversely affects soil health by changing the modification of nutrient availability, soil microbial population, and toxicity to the roots of plants due to increased levels of one or more mineral element concentrations. The area under acidic soil conditions in India is 17.9 million ha (Anonymous, 2016); while in the world 3,950 mha of arable land is affected by soil acidity (Bian et al., 2013), indicating the severity of the problem.
• Sodification: This phenomenon is the opposite of soil acidification, because soil pH is increased by the predominance of carbonates and bicarbonates of sodium. The presence of sodium in soil significantly modifies the soil properties, thereby affecting soil health and productive potential. The major changes in soil due to sodification include dispersion of soil aggregates leading to poor soil physical condition, reduced hydraulic conductivity and infiltration rate, changes in nutrient availability, and toxicity of higher concentration of sodium to plant roots.
Modification of Soil Mineral Nutrient Status (Nutrient Imbalance, Multi-Nutrient Deficiency, and Nutrient Mining)
Among the major input additions in present-day agriculture, nutrient application plays an important role and is mainly due to the increased response of crops to nutrient application, crop and/or cropping system intensification in special and temporal dimensions to feed burgeoning population, and decrease in the level of soil nutrient status. The modification of soil nutritional status is mainly expressed as nutrient imbalance, multi-nutritional deficiency, and nutrient mining. The imbalance arises because of differential nutrient uptake and fertility addition, which does not match the plant uptake; while the present status of multi-nutritional deficiency was increased because of the addition of only primary nutrients (especially N and P) with complete dependence on soil nutrient reserves for other nutrients. Nutrient mining is another term used to indicate the negative balance between nutrient addition and nutrient removed by crops. At present, Indian soils are at negative balance of 8 to 10 million tons per year (NAAS, 2018); while Jones et al. (2013) and Henao and Baanante (2006) reported nutrient mining practices at the global level. The significance of soil mineral nutrient status with respect to soil health and overall agricultural productivity can be explained using the following points:
• increase in the number of nutrients showing deficiency in cultivated soil;
• extent of negative balance of nutrients in the soil;
• responsiveness of crops to the application of nutrients;
• possibility of reducing nutrient mining by utilizing crop by-products as a nutrient source and avoiding their ineffective use such as in-situ burning;
• share and role of organic material addition in meeting the nutrient need of agriculture;
• role of microbes in enhancing the nutritional status of soil;
• long-term effect of application of recommended rate of nutrients on soil nutrient status;
• short and long-term impact of nutrient mining on crop productivity and economics;
• effect of changing soil nutrient supplying capacity due to change in soil organic carbon in arable soils;
• effect of imbalance in the use of chemical fertilizers on soil nutritional status;
• lack of attention for soil and water conservation practices leading to loss of fertile top soil layer rich in plant nutrients;
• and soil fertility changes due to cultivation of crops on marginal and degraded land as well as intensive cereal-based crops/cropping systems with replacement of fertility restorer crops.
Accumulation of Toxic Compound (Agrochemicals)
This is the major source of toxic compound which get accumulated in soil thereby affect the soil health. The use of agrochemicals for plant protection and weed management leads to considerable increase in accumulation of toxic compound in soil. This can be seen from an increase in the use of agro-chemicals from 39,773 to 52,980 metric tons of technical grade material (Bhardwaj and Sharma, 2013; Indira Devi et al., 2017). Even with this significant increase in agrochemical consumption, per hectare consumption in India is 291 g ha−1, which is far lesser than the consumption in developed countries (Indira Devi et al., 2017). The use of pesticides in Japan, China, and Mexico is 18.94, 10.45, and 7.87 kg ha−1, respectively (Zhang, 2018). Along with that, excessive use of chemical salts to provide nutrition is the other source of toxic compounds. Some organic sources of crop nutrition, such as sewage and sludge and night soil, are also reported to contain a high amount of heavy metals (Walia and Goyal, 2010; Saha et al., 2018), causing adverse effects on soil health. The reason for the increasing contribution of agrochemicals to soil chemical degradation is their unregulated and uncontrolled use (Bhardwaj and Sharma, 2013) and lack of proper knowledge and awareness on the use of agrochemicals. The major adverse effects of using agrochemicals on soil health include: (i) adverse effect on the population dynamics of soil microflora and microfauna, (ii) affecting the rate of biogeochemical cycling of nutrients, and (iii) adverse effect on the growth of plants along with bioaccumulation of agrochemicals in plant and animals. Considering the role of agrochemicals in crop production and, overall, in agriculture, their complete elimination is difficult, but following regulations and recommendations in their use can be helpful in minimizing their build-up to an extent that they are causing adverse effects on soil health.
Soil Pollution
Soil pollution in cultivated fields is another emerging problem that is considered as a major outcome of modern agrochemical-based agriculture and lack of accounting of footprint of agricultural activities. Soil pollution is defined as a physical, chemical, biological, or radiological modification of the surface layer of the crust of the earth by the accumulation of a large quantity of natural materials or occurrence of new synthetic materials that disturb the composition of the soil, influence the natural balance of the ecological system, and disable the purification process (self-cleaning) of the soil (Backovic, 2008; Ashraf et al., 2014). The causes of soil pollution in agricultural land are:
• inappropriate use of chemical fertilizers especially phosphatic fertilizers, herbicides, and use of agrochemicals for insect-pest and diseases management;
• application of materials rich in pollutants and use of industrial waste;
• use of inferior plastic films;
• use of polluted water for irrigation;
• use of polluted area for agriculture or growing of crops along a city landfill;
• improper disposal of industrial wastes;
• seepage from landfills and percolation of pollutants along with infiltrating water;
• longer persistence of biochemical compounds in wastes and lack of soil flora and fauna for decomposition of agrochemicals;
• and neglecting the significance of soil pollution remediation measures.
These sources have varied effects on soil health and ultimately on agricultural productivity. The effects of soil pollutions are as follows:
• Soil properties such as porosity, base saturation, soil reaction, soil salinity, and nutrient toxicity are affected because of soil pollution (Backovic, 2008).
• Soil pollution caused by industrial waste or sewage-sludge may lead to the accumulation of heavy metals that may enter in the food web, leading to bioaccumulation of these heavy metals in animals or human beings, leading to several health hazards (Khan et al., 2015). The identification of adverse effects of such pollutants on human health sometimes becomes difficult, as they are seen after long exposure and continue across generation.
• The pollutants present in soil may escape and add to groundwater because of leaching or enter into above-ground water reservoirs, thereby causing pollution in these water bodies. This makes the water unsafe for use and also harms aquatic life (Khanna and Gupta, 2018).
• Pollutants that accumulate in soil up to the toxic level may affect the germination and growth of the next crop in succession.
• Soil pollution may adversely affect the population dynamics of soil microorganisms and thereby nutrient cycling.
• In extreme cases, they make soil unfit for normal crop cultivation.
• Pollutants such as heavy metals are non-degradable by any biological or physical means and therefore remain in soil over longer duration (Selvi et al., 2019).
• Heavy metal pollution is one of the hurdles of direct use of nutrient-containing minerals in agriculture and more especially in organic farming (Mortvedt, 1995).
Soil Biological Degradation
The biological properties of soil are the last to get attention. However, they started getting attention when their normal activities and functioning became affected significantly by modern agricultural practices. Soil biological degradation is defined as the impairment or elimination of one or more significant populations of microorganisms in the soil, often with resulting changes in biochemical processing within the associated ecosystem (Sims, 1990). At present, considering their significant role in different soil processes and functional activities, soil microbial properties are studied as rhizosphere dynamics (Kumar et al., 2013) and soil genomics (Singh et al., 2009a) level. Soil biological properties that can be used to judge the biologically degraded soil (Bedano et al., 2011; Lehman et al., 2015), as given by Mishra and Dhar (2004), are listed below:
• abnormality in microbial community diversity indicated by viable count (colony forming unit);
• reduction in either species richness or evenness of allocation of individuals among various species or both the above-mentioned characteristics;
• adversely affected major soil processes such as soil respiration, different enzyme activities, nutrient cycling, and degradation of organic compounds;
• symptoms of accumulation of toxic compounds in the soil due to their reduced rate of decomposition;
• and an increase in the population of undesirable microorganisms/pathogens causing diseases or serve as a vector for the transfer of different diseases.
The major difficulties in determining soil biological health and evaluating the indicators of soil biological health mentioned by Brackin et al. (2017) are as follows:
• complex relationships of soil microbial life with soil properties and crop plants;
• highly dynamic and sensitive to changes in soil management (such as tillage and amendment addition, etc.);
• difficulty in the identification and quantification of exact soil microbial life affecting soil health because of their very large diversity (Nielsen et al., 2016);
• and use in short-term evaluation because of their higher sensitivity to changes in soil conditions (Obalum et al., 2017).
Soil Ecosystem Services
There are several types of degradation processes acting side by side as discussed in previous sections (Soil Physical Degradation to Soil Ecosystem Services) due to continuous human interferences. All these processes lead to drastic changes in ecosystem services provided by the soil, as listed below:
• reduction in nutrient-supplying capacity of soil with a net negative nutrient balance;
• reduction in the rate of decomposition of soil pollutants due to biological degradation of soil;
• reduction in capacity to act as net carbon sinks because of continuous reduction in soil organic carbon content in most of the agricultural land;
• increasing and decreasing the population diversity of undesirable microbes (pathogen) and useful microbes in the soil;
• increase in areas under salt-affected soil conditions, thereby reducing their productivity potential;
• and reduction in productive potential and future carrying capacity of soil due to the above-mentioned five points.
At the same time, studies on soil ecosystem services are important because of the following points:
• increased level of the human footprint on natural resources;
• faster rate of degradation of natural resources;
• increasing concerns of climate change and its effect on soil ecosystem services;
• increase in the human and animal population, which increases the burden on limited natural resource;
• and economic and global model of development adopted by the world, with less consideration to ecological aspects.
Effect of Soil Degradation on Plant Growth
Considering the level of degradation of soil as discussed in previous sections, the effect of land degradation on soil productivity needs to be quantified. In this section, attempts were made to review the effect of land degradation on plant growth using the study conducted by different researchers from different parts of the world.
Productivity and Profitability
The effect of several land degradation problems on crop productivity can be studied either by accounting for the losses in natural resources due to different processes at the global level, or from the reduced productive potential of degraded soil. In the European Union, Panagos et al. (2018) used microeconomics models and reported that 12 m ha of agricultural areas in the European Union have degraded soil. This led to economic losses in the agricultural sector to be close to €300 million and loss in GDP to be about €155 million. In Senegal, Sonneveld et al. (2016) reported that severe types of land degradation were associated with a decline in crop productivity. Pimentel and Burgess (2013) also reported a significant impact of soil erosion on food production. In the Canadian prairies, Cann et al. (1992) showed a compilation of the significant impacts of soil degradation on different crop yields. In India, Bhattacharyya et al. (2015) reported that the total cost of land degradation varies from US$1,037.94 to 6,191.81 million [1 US dollar ($) = 72.45 Indian rupee (₹)] per annum with the highest cost of land degradation due to soil erosion. This leads to a loss in crop production, which varies between US$93,305 and 4,982.71 million per annum. Zingore et al. (2015) reported different soil quality constraints for crop production in sub-Saharan Africa, and these problems, according to their significance in terms of area affected, are aluminum toxicity > low cation exchange capacity> soil erosion > high phosphorus fixation > vertic properties > salinity > sodicity. They reported that in sub-Saharan Africa the total crop production area affected by these soil constraints was 23 billion ha. These constraints are an indication of degraded soil, and significantly reduce the productivity of the soil. In Australia, Koch et al. (2015) reported the significance of soil security in achieving food security and provision of ecosystem services. Mythili and Goedecke (2016) used a total economic value approach for the calculation of the cost of land degradation and reported that the annual cost of land degradation in India in 2009 was US $5,152.46 million. This indicates that land degradation puts a significant economic footprint along with a footprint on natural resources.
Along with these impacts of soil degradation at a large landscape, the effect of different soil ill health on crop productivity and economics, as well as the response of crop grown in such soil to various amendments reported by different authors are summarized in Table 8. The significant contribution of soil degradation to the reduction in crop productivity can be judged from the accumulation of a large number of such studies (Frye et al., 1982; Lal and Moldenhauer, 1987; Pierce and Lal, 1994; Mantel and Van Engelen, 1997; Wiebe, 2003; Rickson et al., 2015). These different studies showed that soil physical and chemical degradation had a significant and negative impact on soil health. Along with it, the adverse effect of soil biological degradation was also reported (Song et al., 2017) showing a reduction in the germination of different grasses due to the formation of cyanobacteria-dominated crust.
Resource Use Efficiency
Soil salinity is one of the most important problems affecting soil health in irrigation command areas. In saline soil, the amount of water required is higher than the water required for raising crops on normal soil in order to maintain salt balance in root zone depth, and because of that, water productivity is lower in saline soil. In Iran, various options for improvement in water productivity under saline soil conditions were reported by Heydari (2019). He showed that optimum border irrigation and basin irrigation had higher water productivity (1.36 and 1.04 kgm−3) over the traditionally followed basin irrigation method. The salinity of irrigation water is also an important problem that leads to the build-up of soil salinity. Pressurized irrigation systems such as drip irrigation are reported to be most effective in improving water use efficiency and productivity; while the use of saline water for irrigation drip systems is a debatable issue due to root zone accumulation of salt and functioning of drip systems (clogging). Tingwu et al. (2003) showed that, use of saline water through drip irrigation on soil with ≤ 75% silt once every 2 days, at 60% of the Chinese pan evaporation had significantly higher yield and quality of watermelon over control even though water use efficiency in control (39.2 kg m−3) was significantly higher than treatment with 60% of the Chinese pan evaporation (21.45 kg m−3). They also reported that an increase in soil salinity build up averaged over soil profile in irrigation at 60% of the Chinese pan evaporation was very small over original soil salinity. Singh et al. (2018) reported that the application of irrigation water through a sprinkler or low energy water application each at 2 days interval with 4 cm depth at each irrigation significantly improved the water productivity and energy productivity over a surface method of irrigation with a similar level of rice grain yield in all irrigation systems. This finding again reports the successful use of a micro-irrigation system in problematic soil.
Nutrient use efficiency is another major challenge on the front of low nutrient use efficiency of major nutrients and reduction in the partial factor productivity of major nutrients due to multi-nutritional deficiency. Degradation of soil is one of the important reasons for the reduction in nutrient use efficiency and the need of higher fertilization. This can be clear from increasing the number of nutrients showing response to application (Rattan et al., 2009), the status of soil degradation (Bhattacharyya et al., 2015), and increase in the area showing the deficiency of secondary nutrients such as sulfur and micronutrients viz. Zn and Fe (Tandon, 2013). Therefore, the application of amendments for soil improvement may contribute to nutrient use efficiency. Murtaza et al. (2017) reported a significant variation in nitrogen use efficiency in saline-sodic soil in a rice-wheat cropping system. They found that the application of 100 kg N ha−1 with 50% soil gypsum requirement recorded the highest partial factor productivity; and that the application of 130 kg N ha−1 and 100% soil gypsum requirement had the highest agronomic use efficiency of nitrogen in both rice and wheat. Yaduvanshi (2003) reported the positive effect of green manure application and farm yard manure on nitrogen and phosphorus recovery in reclaimed saline sodic soil in a rice-wheat cropping system. They reported that the addition of green manure of Sesbania @ 4.2 t ha−1 with 60 kg N, 13 kg P, and 21 kg K ha−1 had significantly improved N recovery; and that application of Sesbania @ 4.2 t ha−1 with 120 kg N, 26 kg P, and 42 kg K ha−1 had recovery efficiency of 52.8% in wheat. In another study, Barbieri et al. (2006) reported that out of the total nitrogen applied in tall wheatgrass (Elytrigia elongate), recovery efficiency was 23–41% in the 1st year and 67–69% in the second year in sodic soil. They suggested the split application of nitrogen and the use of nitrogen sources other than urea as a strategy to reduce losses. At the same time, the response of different treatments for the correction of soil degradation problems in terms of improving nutrient use efficiency and water use efficiency is mentioned in Table 9. The significance of soil biological degradation in terms of increasing the population of disease-causing pathogens is significantly reducing the efficiency of different resources through their influence on crop growth and yield. Oerke (2006) reported that in the world, crop yield loss due to all major pest and diseases, such as weeds, for wheat, rice, maize, potato, soybean, and cotton was 28.2, 37.4, 31.2, 40.3, 26.3, and 28.8%, respectively, from 2001 to 2003. The loss due to insect–pest in India for cotton, rice, oilseed, pulses, groundnut, and wheat were 30, 25, 20, 15, 15, and 5%, respectively, out of their total production (Dhaliwal et al., 2010). The loss in yield ultimately remains as the natural and artificial resources applied unutilized, which may be lost by one or other pathways thereby reducing their efficiency as well as may cause pollution or degradation of soil and other natural resources.
Novel Agronomic and Innovative Soil Management Approaches for Improving Soil Health
Diversification of Nutrient Sources
The nutrient need of plant is catered by soil inherent supply or externally applied plant nutrients through organic sources, inorganic sources, and microbial inoculants. Along with the supply of nutrients, these externally applied sources of plant nutrition had varied impacts on soil properties, and may be positive or negative. The monotonous use of any one source (especially chemical fertilizers) over a long duration may change soil properties to an extent that leads to making soil ill. The use of chemical fertilizers is getting movement because of quick response, easy availability on subsidized rate, and a significant increase in crop yield, leading to higher economic gain in early year of availability; while during the latter part, the inability of other sources to cater to the need of plant nutrition, intensification of cropping systems to cater to the need of growing human and cattle population and decreasing availability, along with the increased cost of other sources of plant nutrition such as animal waste, are major reasons for the monopoly of chemical fertilizers. These nutrients supplied through chemical fertilizers remain available for a short period of time because of their property of changing chemical nature, and may get lost from the scene along with moving water. The imbalance in the use of these fertilizers and lack of attention for fertilization of secondary nutrients, such as sulfur, and micronutrients, viz. Fe and Zn, lead to their widespread deficiency (Tandon, 2013). This all leads to multi-nutritional deficiency and varied levels of soil degradation (Section Issues Related With Soil Health).
At present, the selection of nutrient source should be such that it provides multiple nutrients for higher yield, has considerable residual effects, and positive influence on soil properties, thereby on soil health and less on environmental footprints. This all will be difficult to achieve through a single source of nutrition. At the same time, the economy of crop nutrition may be improved through partly replacement of chemical fertilizers with other on-farm sources or cost-effective off-farm resources. The sources of crop nutrition, which helps in maintaining or improving soil health along with providing nutrition, are agro-industrial wastes, minerals without processing, green and brown manures, weed manures, and bio-fertilizers. The diversification of nutrient sources toward more responsiveness to soil health is constrained by the availability of highly subsidized chemical fertilizers and their quick, significant, and positive impact on crop production, lack of sufficient organic sources of nutrition, as well as their logistic and on-point availability, less contribution of other sources (microbial inoculation and mineral wastes) to crop nutrition. These sources along with their impact on soil health are discussed below.
Green and Brown Manures
The growth of leguminous plants and their in-situ trampling at the flowering stage by tillage (plowing) or incorporation of leaves and young twigs of plants collected from another area is called green manuring. The significance of using of green manuring crops has been recognized long ago (Pieters, 1927) for its capacity to provide nitrogen (Yang et al., 2018) and enhance soil organic carbon (Ramesh and Chandrasekaran, 2004); while its multifarious effects on crop production (Fageria, 2007; Valadares et al., 2016) and their quantification in various crops and locations are getting movement afterward. The use of green manuring is more common in rice-based cropping systems and, again, in lowland or irrigated rice ecosystems (Pooniya et al., 2012). Brown manuring is a co-culture of Sesbania and rice, in which after 40–50 days of sowing, Sesbania is knocked down by a spray of herbicide (2,4-D). It is more common in upland rice and reported for its potential for controlling weeds in direct seeded rice (Gangaiah and Prasad Babu, 2016; Maitra and Zaman, 2017). The significance of green and brown manuring in soil health improvement reported by different authors is summarized in Table 10. All these reports indicate that both green and brown manuring have a significant and positive effect on soil health along with their contribution to yield improvement and saving on the application of chemical fertilizers. At the same time, green manure has an immense potential to be an important source of crop nutrition in organic farming, which is getting momentum in India. Green manure crops occupy the land for 40 to 55 days during which one productive crop can be raised. At the same time, sufficient water in the soil is needed for proper decomposition and release of nutrients for present season crops, which is a major constraint in rainfed agriculture. Additional cost is incurred in the purchase of seeds and the application of nutrients to green manure crops (phosphorus application) and knockdown of brown manure crops. These are the weak points that make green or brown manuring difficult to be adopted by farmers on a large scale.
Use of Crop Residue and Agro-Industrial Waste
Arable crop production occupies the largest area out of the gross cropped area under cultivation as compared with other crops, such as horticultural crops. Considering the harvest index of arable crops and the nutrient composition of these residues (Sadh et al., 2018), these may be the potential options for the diversification of nutrient sources in agriculture. At the same time, most of the wastes generated from agriculture are voluminous and will add a large amount of organic carbon in soil, which is a backbone of different processes. Another important fact about crop residue is that a large part remains unutilized in cereal-based cropping systems in irrigated areas; while in rainfed farming, due to a large number of competitive uses, it is not available as a nutrient source. The logistic and policy initiative for residue utilization as a source of crop nutrition, blending of different crop residues to enhance nutrient content and faster release of nutrients and location-specific identification, and promotion of cost-effective processes for converting crop residues into suitable forms to be used as a source of nutrition are the thrust area for promoting the use of crop residues as a source of nutrition. The amount of crop residues generated in India from major crops is given in Figure 1. At the global level, residues produced from six major crops (rice, wheat, barley, sugarcane, maize, and soybean) is 3.7 Pg (billion tons) dry matter year−1 (Bentsen et al., 2014); while Lal (2005) reported 3.8 Pg year−1 residue production. The use of crop residues as a source of crop nutrition will be a win-win situation, as it helps to reduce the unutilized waste for agriculture, and their contribution to pollution and footprint, and success in diversifying chemical fertilizer-dominated nutrient management strategies. The co-culture of legumes in cereal-dominated cropping systems, changing nutrient management strategies by accounting the nitrogen need for in-situ decomposition of high C:N ratio crop residue, increased the availability of seeding machines in residue retention and adapting harvesting techniques that maintain a sufficient amount of residues in the soil at marginal farms need to be considered as options to attract stakeholders toward utilization of crop residues as a potential option for crop nutrition. In the case of organic farming, there is a need for such options as the use of in-situ organic sources of nutrition will be more cost-effective than purchased organic sources of nutrition considering increasing prices of off-farm organic sources of nutrition. Out of the total crop residue generated, the share of cereal crops is highest, which have a higher C:N ratio and takes longer time for decomposition and causes immobilization of soil nitrogen; while other crop residues have competitive uses. Crop residues infected with pests and diseases may increase the inoculums for the infection of the crop in the succeeding growing season.
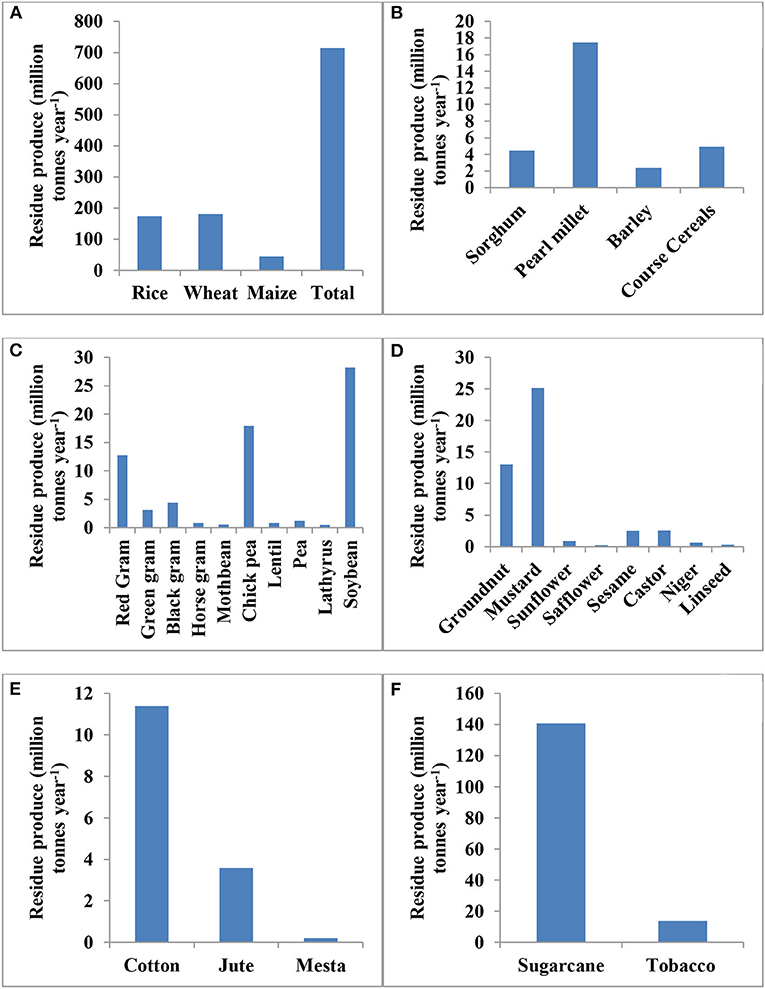
Figure 1. Residue production from different crops in India (A–F) (Anonymous, 2019).
In addition to the above-discussed unprocessed crop residues, residues are also generated while making crop produce suitable for consumption like processing and value addition of crop produce. Major residues from the processing industry include sugarcane factory waste (bagasse, pressmud, and molasses), waste from rice and wheat milling industry, waste from the fruit and vegetable processing industry, waste from the edible and non-edible oil extraction industry, waste generated during the marketing of perishable commodities, and food wastage. The use of agro industrial waste is constrained by the fact that part residues generated from these agro-industries have more economical competitive uses and therefore remain unavailable to be used as a source of nutrient; while part of unutilized residues needs some treatment before being utilized as a crop nutrient source. Information of such pre-treatments and facilities at the community or individual farmer level will be helpful for enhancing their utilization. Another difficulty is associated with the logistics of such agro-industrial waste on account of their large volume.
The significance of using crop residues and agro-industrial wastes in soil health is listed as follows:
• improvement in soil organic carbon content;
• serves as the food and fuel for microbial diversity, and also help in enriching the population diversity of desirable microbes in soil;
• help in reducing the impact of soil physical degradation processes because of positive impact on soil physical properties such as soil aggregation and infiltration rate;
• soil organic carbon enhances the cation exchange capacity, base saturation, and chelation of micronutrients, buffering pH, thereby enhancing soil chemical health;
• and improves soil chemical health through the process of decomposing soil pollutants, which is also fastening by increasing soil organic carbon.
Use of Minerals and Mineral Waste
The restricted supply of micronutrients is a common constraint for plant growth worldwide, especially in organic farming systems where nutrient supply to crops mostly depends on the mineralization of native soil organic matter, decomposition of applied manures, and crop residues. Based on a laboratory incubation study conducted for 140 days to investigate the potential release of copper (Cu), manganese (Mn), and zinc (Zn) from the rock mineral flour (RMF), the results showed that about 4.6% of Cu added as RMF was released irrespective of the quantity of the RMF applied. Zn release from RMF increased from 5.8 to 15.5%, with an increase in the amount of RMF applied (Shivay et al., 2010). These results showed that RMF could be used to meet Cu, Mn, and Zn requirements of organically grown cereals. The use of minerals as a source of crop nutrition without any chemical processing (Kulasekaran et al., 2015) is getting highlighted, and their significance can be explained as follows:
• inability of organic resource to fulfill the need for crop nutrition at present production requirement;
• identification of microbial processes and availability of microbial cultures for enhancing the nutrient availability from minerals making the mineral matter available form;
• availability of mineral waste generated by different processing industries and problem of their disposal;
• increased cost of processing of minerals to make chemical fertilizers and dependence on import of raw material for preparation of chemical fertilizers;
• availability of large amount of mineral, which is unsuitable to be used as raw material for preparation of chemical fertilizers (Kumari and Phogat, 2008);
• suitability of minerals in raw form in specific situation such as suitability of rock phosphate in acidic soil (Sharma and Prasad, 2003);
• and despite the above-mentioned positive impacts on soil health, there are several constraints that make the use of mineral waste difficult. The amount of heavy metals present in minerals and their availability to crop, logistics of voluminous raw minerals, and awareness of the processes and conditions making minerals a suitable source of crop nutrition are the primary hurdles that need to be addressed in making use of minerals in agriculture a suitable option for diversification of nutrient sources.
The low nutrient content, slow release of minerals due to longer time required for disintegration, less change of acting as a non-point source of pollution, lower cost of by-product of processing industry such as basic slage and phosphogypsum., and capacity to work as complimentary and supplementary sources of crop nutrition in organic farming are the other points that need to be considered in making mineral and mineral waste a possible alternative for diversification of nutrient sources.
The positive effects of these on soil health include:
• enhance the soil mineral composition from a crop production point of view thereby increasing the soil inherent nutrient supplying capacity;
• enhance the population and diversity of desirable microbes that are needed for biogeochemical cycling of nutrients in soil;
• and reduce the adverse effect of chemical fertilizer-generated abnormalities on soil properties.
Use of Weeds as Manures
The weed being unwanted plants and categorized as biotic stress can be harvested and used as manure. The weed plants as a composite group generally had a higher concentration of nutrients as compared with crop plants. The important points to be considered while using weeds as manure in crop cultivation are as follows:
• Weeding is generally done during early growth, leading to low dry matter accumulation and thereby lower nutrient accumulation in them.
• Not a viable option of nutrient diversification after seed formation, as it again creates a problem in the next year.
• If precautions are taken, such as using pre-emergence herbicides and following proper cultural measures, then the population and dry matter generated would be very minimal.
• The weeds are composite flora and because of the diversity of species with respect to time and space dimension, it becomes difficult to quantify the expected amount of the nutrients added by weeds.
• At the same time, weeds compete with crop plants and absorb nutrients supplied for crop plants, thereby affecting their growth.
• Some species of weeds have an allelopathic effect on crop plants while decomposing their residue. This may affect the growth of crop plants.
• Weeds grown during the fallow period help in conserving soil moisture and also reduce losses in the fertile top soil layer. This will help in maintaining soil fertility.
• Other positive effects of weeds manures on soil health are the same as those of the addition of crop residues through organic matters.
Adaptation of Modern Tillage System (Minimum/ Zero Tillage, Stubble Mulch Tillage)
The conventional plow tillage involves physical manipulation of soil; therefore, it has several implications on soil health that can be seen primarily on soil physical health, soil biological health, and lastly on soil chemical health. The major objective of conventional plow-based tillage is managing weeds along with preparing of seedbeds with required soil physical properties. Due to the availability of an alternative strategy for weed management (herbicides) and maintaining soil physical condition suitable for sowing of crops without tillage, the present plow-based tillage system is molding to a new form, which is collectively called conservation tillage. The other reasons responsible for the emergence and adoption of the conservation tillage system include the adverse effect of plow-based tillage on soil degradation through erosion and fading organic carbon, increasing prices of energy (petroleum) required for tillage operation, government policy orientation in developed countries during early days, problem of disposal of crop residue in intensive cereal-based cropping systems, short time availability for field preparation in intensive cropping systems, availability of tillage equipment for seeding with least disturbance to soil and in layer of crop residue, and positive effect of conservation tillage in various combinations of resource conservation technology.
The conservation tillage system is based on three major principles, viz. continuous or minimal mechanical soil disturbance, maintenance of a permanent biomass soil mulch cover on the ground surface, and diversification of crop species (Kassam et al., 2019). It consists of different forms such as zero tillage, minimum tillage, and stubble mulch tillage. The positive effect of this tillage system on soil health is indicated by the three above-mentioned principles of conservation agriculture, and increasing area under conservation tillage indicates economic gain either in tangible or non-tangible forms by stakeholders. The health improvements achieved by following the conservation tillage system are listed below:
• Reduction in the rate of soil erosion through wind and water action, which can be achieved because of a reduction in erodibility.
• Increase in soil organic carbon as a minimum 30% of surface covered with crop residue is the principle of the conservation tillage system.
• Enhance the microbial population and diversity, soil microbial biomass carbon and nitrogen, and soil microbial enzymatic activities of microorganisms because of the availability of organic matters as their food.
• Improvement in major soil physical parameters such as water holding capacity, soil aggregation, infiltration rate, porosity, bulk density, and soil strength, thereby making soil physically healthy.
• Added crop residues are a source of multiple plant nutrients and therefore enhance the chemical health of soil.
• Soil chemical properties such as temperature moderation, buffering soil pH, nutrient holding capacity, and ion exchange capacity are positively affected by conservation tillage.
• Some hurdles in the adoption of the conservation tillage-based system include competitive uses of crop residues, immobilization of nitrogen during residue decomposition, acting of crop residues as a hibernating material for crop pests and diseases causing pathogen, build-up of termite population, reduced crop germination, and difficulty in manure and fertilizer application.
• The third principle of CA (crop species diversification) can reduce the extraction of nutrients from the same soil layer, and if fertility restorer crops (such as legumes and grasses) are included in the cropping system, then it will have positive and beneficial effects on soil health.
• Maintaining crop residues also helps in correcting soil root zone salinity because of reduced evaporation losses.
Enhancing Soil Microbial Diversity (Use of PGPR and Microbial Consortia, Use of Biocontrol Agents)
There are two possible ways to enhance soil microbial diversity. The first one is the direct addition of microbial culture and the other is the enhancement of the inherent soil microbial population by providing a suitable environment for microbial growth. The direct improvement of soil microbial diversity was started with the use of biofertilizers (microbial inoculation having the capacity of nutrient acquisition/ fixation) for nitrogen fixation. The possible options for enhancing microbial diversity are as follows:
• Use of microbial cultures that have a capacity for nutrient acquisition and fixation.
• Use of microbial cultures that have an antagonistic interaction with disease-causing microorganisms and deleterious rhizobacteria.
• Use of microbial cultures that fasten the rate of organic matter turnover (Choudhary et al., 2016).
• Use of microorganisms that secrete growth-promoting hormones such as auxin (Zahir et al., 2004).
• Use of microbes that have a capacity to fasten the decomposition residue of agrochemicals or soil pollutants (soil security; Nayak et al., 2018).
The indirect ways for enhancing microbial population diversity include:
• Using organic sources of crop nutrition as per availability and economic consideration in varied combinations of chemical fertilizers.
• Changing tillage system from conventional plow-based to conservation tillage.
• Use of soil amendments for correcting soil reactions as a pH range near neutral is suitable for different types of microbial growth and processes.
• Crop diversification with place for legumes and forage crops. Legume crops secrete a large amount of carbon material through their roots, and their rhizosphere is rich in microbial diversity (Kumar et al., 2018). Forage crops, such as Napier grass, produce a large amount of root biomass; while growing of berseem was reported to have a positive effect on soil physical and chemical properties.
• Following harvesting methods that maintain at least part of above-ground plants on the soil surface.
• Irrigation management practices for modification of soil microclimates suitable for microbial growth that include drainage of excess water, creation and utilization of irrigation facility from rain water or water from above ground or below ground reservoirs, and irrigation for reducing soil salinity.
• Increasing the use of resource conservation technologies such as green and brown manuring, use of organic mulches and different land configurations such as permanent beds.
There are certain lacunae that make it difficult to adopt the direct methods of enhancing soil microbial population, and include low economic gain and less visibility of crop growth and yield improvement due to their uses, the growth and population build-up is affected by soil environment and weather condition, higher sensitivity to agrochemicals, and their availability in pure form without admixture of any other material. The indirect options of enhancing soil microbial diversity have economic bias; hence, their uniform and wider implications in the favor of enhancing the soil health remain frozen.
Positive Effects of Microbial Enhancement on Soil Health
The impact of improvement in microbial diversity on soil health is overlapped by the impact of diversification of nutrient sources, as both are interdependent on their capacity to improve soil health. The crop residue serves as a raw material for microbial activities; while microbes are important agents for decomposition or turnover of diversified nutrient sources. Some of the additional positive effects of enhancing soil microbial diversity are as follows:
• Enhancing soil microbial diversity fastens the decomposition of agrochemicals and other harmful plant secretions, thereby making soil pollution free.
• Short term storage of plant nutrition through the process of immobilization, thereby reducing losses in plant nutrients.
• Helps in reducing the population of soil-borne disease-causing microorganisms because of antagonistic interaction and competition for same natural resources.
• Improves soil chemical health by increasing the share of fixed forms of nutrients in crop nutrition. This is most prominently seen in the case of phosphorus, as the use efficiency of phosphorus applied through soluble chemical fertilizers hardly exceeds 15–20% (Roberts and Johnston, 2015; Prasad et al., 2018); while most of the phosphorus remains in the soil in a fixed form.
Efficient Resource Cycling Through Integrated Farming System
In the urge of an ambitious project of doubling the income of farmers in India, several agricultural interventions have to play an integrated role (Anonymous, 2018a). One such option suggested to achieve this target is curtailing the cost of purchased resources through the generation and use of on-farm resources and their recycling or multiple uses in production systems. This is possible through the integrated farming system (IFS) approach involving the integration of more than one enterprise complementing the main enterprise (which is most of the time a cropping system). As this resource cycling through IFS is linked with economic gain, it can be smoothly adopted by farmers, and soil health improvement through this option is complimentary with the involvement of very less monetary inputs.
The possible options for soil health improvement through resource recycling in IFS are:
• Incorporation of small animals and birds (poultry) with higher liquidity of capital (as the investment on feed and space is less and for short time). These animals can be reared on on-farm inputs, and their excreta are a boon to soil health improvement.
• Installation of crop by-product enrichment plants such as vermi-composting unit and composting unit.
• Installation of a biogas unit and use of slurry as manure (it reduces methane emission from direct application of biomass).
• Planting of leguminous plants such as Leucana leucocephala, Gliricidia, which can serve as green manuring crops.
The integrated farming system has a positive effect on soil due to the followings reasons:
• The efficient cycling of by-products reduces wastage and enhances the biogeochemical cycling of plant nutrition, which is the basis of soil chemical health.
• The final by-products after multiple uses (such as use of crop residue for cattle feed or for mushroom production or for vermi-composting) of the resources have a retained and sometimes even enhanced nutritional value, which can be a valuable soil amendment.
• The complementary interaction between natural resources and different enterprises helps in making a closed system of nutrient cycling. This ultimately helps in enhancing the sustainability of the system.
Marginal farm area, difficulty in marking of small produce, complex interactions among enterprises, difficulty at farmer level to have expertise in all enterprises, lack of awareness on the positive interactions among enterprises, low risk-bearing ability, and capital investment are the major bottlenecks of implementing IFS-based systems.
Soil Health Improvement in Problem Soil (Through Use of Soil Amendments, and by Crop Cultivation Practices and Phytoremediation)
Problematic soils in India mainly consist of salt-affected soil and acidic soil with an area extension of 6.73 million ha (Sharma et al., 2016) and 15.93 million ha, respectively. However, at the global level, 0.34 billion and 0.56 billion ha of the area have saline and sodic soil, respectively (Shahid et al., 2018). Along with this, there are soils that are getting polluted because of untreated industrial effluents, sewage water and waste from landfill areas, and seepage of industrial pollutants. These soils have several problems and need special management practices and input addition along with normal management practices for successful crop production. These practices are broadly divided as follows.
Use of Soil Amendments and Its Effect on Soil Health
Soil amendments are mainly added to bring the soil reaction to the desirable range, thereby improving soil health. Considering soil reactions, exchangeable sodium percentage and electrical conductivity of the soil are broadly classified as saline, sodic (alkali), and saline-sodic (alkali) soil. Saline soil is dominated by soluble salts such as sulfate and sodium chloride; while the dominant salt in sodic soil is sodium carbonate. In the case of saline soil, the leaching of soluble salts below the root zone with plenty of fresh water is followed. Along with that, limestone and iron pyrite are chemical soil amendments that can be added. In the case of sodic soil, gypsum, sulfur, iron sulfate, and iron pyrite may be added to improve the soil condition. The improvement for acidic soil is done by liming with calcium oxide, calcium hydrate, dolomite, calcite, or basic slag.
The application of soil amendments for the correction of sodic soil has a significant and positive effect on soil health through improvement in soil properties such as aggregation, porosity, and infiltration rate, replacing exchangeable sodium concentration from exchange complexes and bringing the pH in the neutral range. In acidic soil, the application of liming materials leads to a reduction in the toxic concentration of metal elements such as Fe, Mn, and Al, enhancement of the availability of phosphorus, calcium, magnesium, and potassium, and enhancement of the activity and diversity of microbes in the soil. These improvements in soil health make the soil fit for crop cultivation.
Cultivation Practices
Along with the addition of soil amendments, cultivation practices are also reported to be beneficial for the management of problematic soil. These are as follows:
Soil Tillage
Deep plowing in order to increase infiltration of rainfall moisture to a considerable depth, compartmental bunding, which increases the opportunity time for infiltration of rainwater and opening of a dead furrow, which acts as a drainage channel during an event of heavy rainfall and stores moisture, are suggested modifications.
Land Configuration
Land leveling, which reduces depression spots where water gets collected and there may be an accumulation of salts and different land configurations such as ridges and furrows, and sowing of crop ¾ height of ridges are also suggested for efficient crop cultivation in problematic soils.
Selection of Crops, Mulching, and Irrigation
Crops tolerant of saline soil such as mustard, barley, cotton, and sugar beet (Jehangir et al., 2013) are suggested; while for sodic/alkali soil, Karnal grass, para grass, rhodes grass, rice, sugar beet, and green manure crops such as dhaincha (Sesbania aculeata) are suggested (Chhabra, 1996). Other suggested measures are the application of excessive water during pre-sowing irrigation for leaching of salts, frequent and shallow irrigation, use of fresh quality irrigation water, and use of organic mulches to reduce evaporation losses, which will reduce the upward movement of salts.
All these cultivation practices improve soil physical properties and promote soil microbial population and diversity, which ultimately contribute to soil health improvement. The addition of organic matters due to the growing of crops, application of mulches, and suitable microclimate provided by irrigation help in increasing microbial population, thereby improving soil biological health.
Phytoremediation
It is defined as the use of higher plants for the cost effective, environmental-friendly rehabilitation of soil and groundwater contaminated by toxic metals and organic compounds (Aken, 2011). Phytoremediation plays a role in soil health improvement through its capacity to combat soil pollution. It is achieved by phytoextraction (phytoaccumulation), phytovolatilization, phytostabilization, or phytodegradation (Yan et al., 2020). This strategy is important for heavy metal pollutants, organic pollutants, industrial effluents, sewage water, waste for landfills used as manure, etc. Nowadays, phytoremediation is essential as town compost and waste water from cities is increasingly used in agriculture in peri-urban areas mainly for the growing of vegetables and flowers. Therefore, these areas have polluted soil that needs to be reclaimed in a cost-effective way. At the same time, the use of agrochemicals is now a regular practice and is increasing day by day because of changes in the level of biotic stresses and the need to produce more from limited resources. Therefore, soil pollution is going to be an important reason for soil degradation in times to come. Some of such situations are observed in parts of India where soil ground water is becoming polluted because of the excessive use of agrochemicals (Kaur and Kaur, 2019). Considering this, it has become essential to incorporate the phytoremediation strategy in agricultural production systems. Besides pollution in agricultural land, areas for dumping of waste are increasing at an alarming rate (Kumar et al., 2017; Kiran et al., 2020), and they will act as a source of contaminants for agriculturally useful land in the future, and these are areas within the scope of phytoremediation. Another important consideration for the phytoremediation technique is that it does not show any significant effect on crop growth and development in the short term, but it helps in improving soil health by reducing the adverse effect of pollutants on human and animal health.
Mimicry of Natural Ecosystem in Agro-Ecosystem for Soil Health Improvement
An agroecosystem is a natural ecosystem modified for the production of different provisional services (Hodgson, 2012), and it is characterized by both planned and unplanned diversities (Power, 2013). It differs from a natural ecosystem in terms of low species and genetic diversity, open system of nutrient cycling, simple and linear tropical interaction, and, most importantly, it heavily depends on human interference for its different functions (Odum, 1969). All of these make an agroecosystem fragile, leading to concern about its sustainability. Along with it, several types of human-induced land degradation (Sections Soil Physical Degradation to Soil Ecosystem Services) add to the instability of agroecosystems. On the other hand, natural ecosystems have several types of self-regulating and self-sustaining functions having the potential to be used in agroecosystems. Studying such functions and identifying the optimum niche of agroecosystems for their successful incorporation in agroecosystems is called mimicking the natural ecosystem. According to Dore et al. (2011), the incorporation of certain characteristics of natural ecosystems into agroecosystems would improve some properties of agroecosystems, such as productivity, stability, and resilience, and that could be considered as mimicry of agroecosystems. This mimicry of natural ecosystems needs to have an economic bias along with improving long-term sustainability for higher adoption at the used end. For the successful implementation of mimicry of natural ecosystems in agroecosystems, Dore et al. (2011) mentioned certain steps, which are listed below:
• Selection of functions that agronomists wish to improve.
• Identification, in natural ecosystems, of characteristics modifying these functions (diversity, microclimate, soil microbes interaction).
• Definition of qualitative and quantitative relationships linking properties and functions (slash and burn cultivation).
• Transposition of these functions to agricultural conditions.
• Use of these functions for the design of agroecosystems with specified aims.
• Checking that the new agroecosystems express the targeted functions and have no undesirable properties.
Along with this, the concept of ecological intensification (Tottonell, 2014) of agriculture also found a sustainable strategy and had a positive impact on soil health. The options for mimicking natural ecosystems with economic consideration include diversification of cropping systems, crop intensification in space and time dimensions (mixed or inter cropping and crop rotation), residue incorporation, less disturbance to soil (changing tillage system to zero or minimum tillage), multi-storied cropping, and many more. The concept of conservation agriculture, organic farming, integrated farming systems, and groups of resource conservation technologies are parallel with the concept of mimicry of natural ecosystems. Therefore, the positive effect of mimicry of natural ecosystems on soil health will be the same as that of the effect of the above-mentioned technology.
Alternative Agriculture (Agroforestry) for Soil Health Management of Marginal Land
Along with the strategy for reducing the degradation of agricultural land, a suitable strategy for the management of already severely degraded land or marginal land unsuitable for regular cultivation is the need of hours. At the global level, the extent of degraded land has been reported from <1 billion to as high as 6 billion ha (Gibbs and Salmon, 2015). Further, degraded land can be realized by seeing the land use pattern of India, which shows that 17.47 million ha of the area have barren and un-culturable lands and 13.24 million ha of the area have culturable waste lands (Anonymous, 2019). The areas are hardly suitable for regular cultivation of arable crops and if desired, then additional management practices are required, which may not be economical. A suitable economical alternative for restoration of such areas is possible through alternative agriculture such as agroforestry (Anonymous, 2018b). The food and agricultural organization define agroforestry as a collective name of land use systems and technologies where woody perennials are deliberately used on the same land management units as agricultural crops and/or animals, in some form of special arrangement or temporal sequence. The system is self-sustaining because of the involvement of diversified components such as arable crops, forage species, tree components, and domestic animals, with three basic systems, viz. agrisilviculture, silvopastoral, and agrisilvopastoral. The positive effects of agroforestry on soil health are as follows:
• The tree component of agroforestry protects soil from erosion through an extensive root network and large canopy. It is also helpful in stabilizing gullies and preventing their spread. At the same time, it produces a large amount of woody matter if retained over a longer duration and can be claimed as carbon credit.
• The grass component involved in agroforestry helps conservation of soil against erosion due to thick cover on ground and also enhances soil organic carbon. This leads to reduction in land degradation.
• Leguminous tree and shrubs species such as Acacia Senegal (L) Willd., Cajanuscajan L., Gliricidia sepium, Sesbania sesban, and Tephrosia spp., enrich the soil through biologically fixed nitrogen along with the addition of organic matter through leaf fall (Ribeiro-Barros et al., 2018). This will help enhance soil biological health.
• As a self-sustaining system, agroforestry is a cost-effective option for the management of soil health on degraded and waste land, with additional income through wood and fodder produced.
• The areas along field boundaries, farm roads, or canals that remain barren and severely affected by one or other types of land degradation will also be suitable for one or other components of agroforestry. This leads to enhanced biodiversity of cultivated farms, thereby enhancing the soil health of farms as a whole.
• The agroforestry system, as a whole, generates several functions that will help in biogeochemical nutrient cycling with the active involvement of biosphere components such as plants and microorganisms.
Conclusions
In the present day, soil no more remains a medium for plant growth but it turns into a valuable resource for mankind to meet its requirement of provisional services from plants and animals receding in agroecosystems. Considering the present level of land degradation, there is a need to develop and implement novel approaches to maintain soil health with a similar or even higher level of production from agroecosystems. Concepts such as diversification of nutrient sources with emphasis on the use of organic manures and other alternatives to compliment and supplement the chemical fertilizer-based approach will have the potential to contribute significantly to the improvement of soil health. The diversification of production systems through the adoption of conservation agriculture and organic farming is worth considering their role in soil health improvement. The closed system of nutrient cycling achieved through an integrated farming system, will be the self-sustained option of soil health management, along with improvement in resource use efficiency. There is a need to give attention to soil biological health, with the involvement of attempts to enhance soil microbial diversity and curtailment of soil pollution caused by the extensive use of agrochemicals (such as chemical fertilizers).
Author Contributions
AS has prepared the first draft of the manuscript. YS have conceptualized and edited the manuscript. Both authors contributed to the article and approved the submitted version.
Conflict of Interest
The authors declare that the research was conducted in the absence of any commercial or financial relationships that could be construed as a potential conflict of interest.
Publisher's Note
All claims expressed in this article are solely those of the authors and do not necessarily represent those of their affiliated organizations, or those of the publisher, the editors and the reviewers. Any product that may be evaluated in this article, or claim that may be made by its manufacturer, is not guaranteed or endorsed by the publisher.
References
Aken, B. V. (2011). “Transgenic plants and associated bacteria for phyto-remediation of organic pollutants,” in Comprehensive Biotechnology, 2nd Edn, eds M. Moo-Young (Cambridge, MA: Academic press), 223–237. doi: 10.1016/B978-0-08-088504-9.00330-5
Al-shammary, A. A. G., Kouzani, A. Z., Kaynak, A., Khoo, S. Y., Norton, M., and Gates, W. (2018). Soil bulk density estimation methods: a review. Pedosphere 28, 581–596. doi: 10.1016/S1002-0160(18)60034-7
Anonymous (2011). Methods Manual on Soil Testing in India. Department of Agriculture & Cooperation Ministry of Agriculture Government of India, 207. Available online at: http://agriculture.uk.gov.in (accessed May 23, 2021).
Anonymous (2016). Guidelines for Reclamation of Problem Soils (RPS)- A Sub Scheme of RKVY, Natural Resource Management Division, Ministry of Agriculture and Farmer Welfare, Government of India, New Delhi, 1–12. Available online at: agricoop.nic.in (accessed February 7, 2021).
Anonymous (2018a). Report of the Committee on Doubling Farmers' Income, Volume IX, Farmer Linked Activities and Secondary Agriculture, Department of Agriculture, Cooperation and Farmers' Welfare, Ministry of Agriculture and Farmers' Welfare, 154+v. Available online at: http://agricoop.gov.in/sites/default/files/DFI%20Volume%209.pdf (accessed February 7, 2021).
Anonymous (2018b). Economics of Desertification, Land Degradation and Drought in India, Volume I: Microeconomic Assessment of the Cost of Land Degradation in India. New Delhi: The Energy and Resource Institute, 149+xviii.
Anonymous (2019). Agricultural Statistics at a Glance 2019, Directorate of Economics and Statistics, Department of Agriculture Cooperation and Farmers Welfare, Ministry of Agriculture and Farmers Welfare, Government of India, xviii+315. Available online at: agricoop.nic.in; http://eands.dacnet.nic.in (accessed December 30, 2020).
Are, K. S. (2019). “Bioachar and soil physical health,” in Bioachar-An Imperative Amendment for Soil and Environment, eds V. Abrol and P. Sharma [ebook (pdf)]. ISBN, 978–1-83881-989-7.
Arshad, M. A., and Gill, K. S. (1996). Field pea response to liming of an acid soil under two tillage systems. Can. J. Soil Sci. 68, 549–555. doi: 10.4141/cjss96-068
Ashraf, M. A., Maah, M. J., and Yusoff, I. (2014). “Soil contamination, risk assessment and remediation,” in Environmental Risk Assessment of Soil Contamination, ed M. C. Hernandez-Soriano (IntechOpen publication). Available online at: https://www.intechopen.com/books/environmental-risk-assessment-of-soil-contamination/soil-contamination-risk-assessment-and-remediation (accessed January 4, 2021).
Aulakh, M. S., and Sidhu, G. S. (2015). “Soil degradation in India: causes, major threats, and management options,” in MARCO Symposium 2015 – Next Challenges of Agro-Environmental research in Monsoon Asia. Tsukuba: National Institute for Agro-Environmental Sciences (NIAES), 151–156.
Awad, S. R., and El Fakharany, Z. M. (2020). Mitigation of waterlogging problem in El-Salhiya area, Egypt. Water Sci. 34, 1–12. doi: 10.1080/11104929.2019.1709298
Backovic, D. (2008). “Soil pollution,” in Encyclopaedia of Public Health, ed W. Kirch (Dordrecht: Springer). doi: 10.1007/978-1-4020-5614-7_3280
Balfour, E. B. (1943). The Living Soil (1st Edn.). Faber and Faber publication. Available online at: https://www.pinterest.com/pin/522206519262354047/
Barbieri, P. A., Echeverria, H. E., Rozas, H. R. S., and Picone, L. I. (2006). Nitrogen use efficiency from urea applied to a wheatgrass (Elytrigia elongate) prairie is a sodic soil. Austr. J. Exp. Agri. 46, 535–543. doi: 10.1071/EA04180
Batey, T. (2009). Soil compaction and soil management- a review. Soil Use Manag. 25, 335–345. doi: 10.1111/j.1475-2743.2009.00236.x
Baveye, P. C., Baveye, J., and GOwdy, J. (2016). Soil ecosystem services and natural capital: critical appraisal of research on uncertain ground. Front. Environ. Sci. 4:41. doi: 10.3389/fenvs.2016.00041
Bedano, J. C., Dominguez, A., and Arolfo, R. (2011). Assessment of soil biological degradation using mesofauna. Soil Tillage Res. 117, 55–60. doi: 10.1016/j.still.2011.08.007
Belnap, J. (2005). “Crust- biological,” in Encyclopedia of Soils in the Environment, ed D. Hillel (London: Elsevier publication), 339–334. doi: 10.1016/B0-12-348530-4/00131-4
Bennett, H. H. (1943). Adjustment of agriculture to its environment. Ann. Assoc. Am. Geogr. 33, 163–198. doi: 10.1080/00045604309357249
Bentsen, N. S., Felby, C., and Thorsen, B. J. (2014). Agricultural residue production and potential for energy and materials services. Progr. Energy Combust. Sci. 40, 59–73 doi: 10.1016/j.pecs.2013.09.003
Berihun, T., Tolosa, S., Tadele, M., and Kebede, F. (2017). Effect of biochar application on growth of garden pea (Pisum sativum L.) in acidic soils of Bule Woreda Gedeo Zone Southern Ethiopia. Int. J. Agron. 2017:6827323. doi: 10.1155/2017/6827323
Bernardi, A. C. C., Almeida Machado, P. L. O., Madari, B. E., Tavares, S. R. L., Campos, D. V. B., and Crisostomo, L. A. (2007). Carbon and nitrogen stocks of an arenosol under irrigated fruit orchards in semiarid Brazil. Sci. Agricola 64, 169–175 doi: 10.1590/S0103-90162007000200010
Bhardwaj, T., and Sharma, J. P. (2013). Impact of pesticides application in agricultural industry: an Indian Scenario. Int. J. Agri. Food Sci. Technol. 4, 817–822. Available online at: http://www.ripublication.com/ijafst.htm
Bhattacharyya, R., Ghosh, B. N., Mishra, P. K., Mandal, B., Rao, C. S., Sarkar, D., et al. (2015). Soil degradation in India: challenges and potential solutions. Sustainability 7, 3528–3570. doi: 10.3390/su7043528
Bhuyan, R. (2019). A review note on shifting cultivation in Northeast India amidst changing perceptions. Dhaulagiri J. Sociol. Anthropol. 13, 90–95. doi: 10.3126/dsaj.v13i0.24252
Bian, M., Zhou, M., Sun, D., and Li, C. (2013). Molecular approaches unravel the mechanism of acid soil tolerance in plants. Crop J. 1, 91–104. doi: 10.1016/j.cj.2013.08.002
Blecourt, M. D., Grongroft, A., Baumann, S., and Eschenbach, A. (2019). Losses in soil organic carbon stocks and soil fertility due to deforestation for low-input agriculture in semi-arid southern Africa. J. Arid Environ. 165, 88–96. doi: 10.1016/j.jaridenv.2019.02.006
Bouma, J., Dekker, L. W., and Haans, J. C. F. M. (1980). Measurement of depth of water table in heavy clay soil. Soil Sci. 130, 264–270 doi: 10.1097/00010694-198011000-00006
Bouyoucos, G. (1962). Hydrometer method for making particle size analysis of soils. J. Agron. 54, 464–465. doi: 10.2134/agronj1962.00021962005400050028x
Brackin, R., Schmidt, S., Walter, D., Bhuiyan, S., Buckley, S., and Anderson, J. (2017). Soil biological health—what is it and how can we improve it? Proc. Austr. Soc. Sugarcane Technol. 39, 141–154. Available online at: https://www.wettropicsplan.org.au/var/nrm/storage/original/application/9db142b1bdc67da1900dd29e0df13ecb.pdf
Brar, B. S., Dheri, G. S., Lal, R., Singh, K., and Walia, S. S. (2015). Cropping system impacts on carbon fractions and accretion in typic Ustochrept soil of Punjab. J. Crop Improv. 29, 291–300. doi: 10.1080/15427528.2015.1016251
Bremner, J. M. (1960). Determination of nitrogen in soil by Kjeldahl method. J. Agri. Sci. 55, 11–33. doi: 10.1017/S0021859600021572
Brevik, E. C. (2009). “Soil health and productivity,” in Soils, Plant Growth and Crop Production. Encyclopedia of Life Support Systems (EOLSS), Developed under the Auspices of the UNESCO, ed W. Verheye (Oxford: EOLSS Publishers). Available online at: http://www.eolss.net (accessed January 4, 2021).
Brevik, E. C., and Sauer, T. J. (2015). The past, present, and future of soils and human health studies. SOIL 1, 35–46. doi: 10.5194/soil-1-35-2015
Brevik, E. C., Steffan, J. J., Burgess, L. C., and Cerdà, A. (2017). “Links between soil security and the influence of soil on human health,” in Global Soil Security. Progress in Soil Science Series, eds D. Field, C. Morgan, and A. McBratney (Rotterdam: Springer), 261–274. doi: 10.1007/978-3-319-43394-3_24
Brivik, E. C. (2018). A Brief History of Soil Health Concept. Available online at: http://profile.soil.org/posts/field-and-hostorical-notes/a-brife-historyof-soil-health-concept (accessed November 30, 2020).
Cann, M., Dumanski, J., and Brklacich, M. (1992). The Impacts of Soil Degradation on Crop Yields in the Canadian Prairies: An Annotated Bibliography. Available online at: https://www1.agric.gov.ab.ca/$department/deptdocs.nsf/all/sag11262/$file/Impact_of_Soil_Degradation_on_Crop_Yields.pdf? (accessed January 30, 2021).
Cardoso, E. J. B. N., Vasconcellos, R. L. F., Bini, D., Miyauchi, M. Y. H., Santos, C. A., Alves, P. R. L., et al. (2013). Soil health: looking for suitable indicators. What should be considered to assess the effects of use and management on soil health? Sci. Agricola 70, 274–289. doi: 10.1590/S0103-90162013000400009
Casanova, M., Tapia, E., Seguel, O., and Salazar, O. (2016). Direct measurement and prediction of bulk density on alluvial soils of central Chile. Chilean J. Agric. Res. 76:105–113 doi: 10.4067/S0718-58392016000100015
Casida, L. E. J., Klein, D. A., and Santaro, T. (1964). Soil dehydrogenase activity. Soil Sci. 98, 371–376. doi: 10.1097/00010694-196412000-00004
Chapman, H. D. (1965). Cation-exchange capacity. In: C.A. Black (ed.). Methods of soil analysis - Chemical and microbiological properties. Agronomy 9, 891–901. doi: 10.2134/agronmonogr9.2.c6
Chaudhary, T. N., and Kar, S. (1998). “Aggregate and clod size distribution,” in Theory and Practices in Agrophysics Measurements, eds R. P. Gupta and B. P. Ghildyal (Mumbai: Allied publishers Ltd.), 61–71.
Chauhan, B. S., Mahajan, G., Sardana, V., Timsina, J., and Jat, M. L. (2012). Productivity and sustainability of the rice-wheat cropping system in indo-Gangetic plains of the Indian subcontinent: problems, opportunities' and strategies. Adv. Agron. 117, 315–369. doi: 10.1016/B978-0-12-394278-4.00006-4
Chhabra, R. (1996). Soil Salinity and Water Quality. Brookfield, TV: CRC Press, A. A. Balkema publishers), xv+282.
Choudhary, K., Mohanty, M., Sinha, N. K., Rawat, A., Hati, K. M., Saha, R., et al. (2015). Rooting behaviour of chickpea (Cicer arietinum) as affected by soil compaction levels in Vertisol of central India. Ind. J. Agri. Sci. 85, 1085–1091.
Choudhary, M., Sharma, P. C., Jat, H. S., Nehra, V., McDonald, A. J., and Garg, N. (2016). Crop residue degradation by fungi isolated from conservation agriculture fields under rice-wheat system of North-west India. Int. J. Recycl. Org. Waste Agri. 5, 349–360. doi: 10.1007/s40093-016-0145-3
Costanza, R., D'Arge, R., de Groot, R., Farberk, S., Grasso, M., Hannon, B., et al. (1997). The value of world's natural ecosystem services and natural capital. Nature 387, 253–260. doi: 10.1038/387253a0
Das, D. K., and Chong, W. N. (1998). “Particle size distribution analysis of soil and textural classification,” in Theory and Practices in Agrophysics Measurements, eds R. P. Gupta, and B. P. (Mumbai: Ghildyal Allied publishers Ltd.), 31–44.
Das, S., Bhattacharyya, R., Das, T. K., Sharma, A. R., Dwivedi, B. S., Meena, M. C., et al. (2021). Soil quality indices in a conservation agriculture based rice-mustard cropping system in North-western Indo-Gangetic plains. Soil Tillage Res. 208:104914. doi: 10.1016/j.still.2020.104914
Davidson, E. A., Savage, K., Verchot, L. V., and Navarro, R. (2002). Minimizing artifacts and biases in chamber-based measurements of soil respiration. Agric. For. Meteorol. 113, 21–37 doi: 10.1016/S0168-1923(02)00100-4
Dhaliwal, G. S., Jindal, V., and Dhawan, A. K. (2010). Insect-pest problems and crop losses: changing trends. Ind. J. Ecol. 74, 1–7.
Dhruvanarayana, V. V., and Babu, R. (1983). Estimation of soil erosion in India. J. Irrigation Drain. Engg. ASCE 109, 419–434. doi: 10.1061/(ASCE)0733-9437(1983)109:4(419)
Doran, J. W., and Zeiss, M. R. (2000). Soil health and sustainability: managing the biotic component of soil quality. Appl. Soil Ecol. 15, 3–11 doi: 10.1016/S0929-1393(00)00067-6
Dore, T., Makowaski, D., Malezieux, E., Jolain, N. M., Tchamitchian, M., and Tittonell, P. (2011). Facing up paradigm of ecological intensification in agronomy: revisiting methods, concepts and knowledge. Eur. J. Agron. 34, 197–210. doi: 10.1016/j.eja.2011.02.006
Eswaran, H., Lal, R., and Reich, P. F. (2001). “Land degradation: an overview,” in Responses to Land Degradation. Proc. 2nd. International Conference on Land Degradation and Desertification, KhonKaen, Thailand. eds E. M. Bridges, I. D. Hannam, L. R. Oldeman, F. W. T. Pening de Vries, S. J. Scherr, and S. Sompatpanit (New Delhi: Oxford Press). Available online at: https://www.nrcs.usda.gov/wps/portal/nrcs/detail/soils/use/?cid=nrcs142p2_054028 (accessed December 24, 2020).
Fageria, N. K. (2007). Green manuring in crop production. J. Plant Nutr. 30, 691–719. doi: 10.1080/01904160701289529
FAO (2008). An International Technical Workshop. Investing in Sustainable Crop Intensification. The Case for Improving Soil Health, Vol. 6. Rome: Integrated Crop Management, FAO.
Field, D. J. (2017). “Soil security: dimensions,” in Global Soil Security. Progress in Soil Science, eds D. J. Field, C. L. S. Morgan, and A. B. McBratney (Cham: Springer). doi: 10.1007/978-3-319-43394-3
Frye, W. W., Ebelhar, S. A., Murdock, L. W., and Blevins. (1982). Soil erosion effects on properties and productivity of two Kentucky soils. Soil Sci. Soc. America J. 46, 1051–1055. doi: 10.2136/sssaj1982.03615995004600050033x
Ganeshamurthy, A. N., Kalaivanan, D., and Rajendiran, S. (2020). “Carbon sequestration potential of perennial horticultural crops in India tropics,” in Carbon Management in Tropical and Subtropical Terrestrial Systems, eds P. K. Ghosh, S. K. Mahanta, D. Mandal, B. Mandal, and S. Ramakrishnan (Singapore: Springer), 20. doi: 10.1007/978-981-13-9628-1_20
Gangaiah, B., and Prasad Babu, M. B. B. (2016). Brown manuring as a tool of weed management and contributor to nitrogen nutrition of direct wet seeded rice. Oryza 53, 415–421.
Gardi, C., Visioli, G., Conti, F. D., Scotti, M., Menta, C., and Bodini, A. (2016). High natural value farmland: assessment of soil organic carbon in Europe. In: optimizing the delivery of multiple ecosystem goods and services in agricultural systems. Front. Environ. Sci. 2016:47. doi: 10.3389/fenvs.2016.00047
Gerard, C. J., Burleson, C. A., Cowley, W. R., Bloodworth, M. E., and Kunza, G. W. (1964). Hardpan Formation in Course and Medium Textured Soils in the Lower Rio Grande Valley of Texas. Texas A and M University, Texas agricultural experiment station, R. E. Patterson, Director, College station, Texas, 1–12. Available online at: http://oakstrust.library.tamu.edu/bitstream/handle/1969.1/90489/bull1007.pdf?sequence=13 (assessed December 12, 2020).
Ghosh, M., and Devi, A. (2019). Assessment of crop growth, soil properties and crop yield in an upland acidic soil with inorganic fertilizer blended with organic amendments in summer rice cropping seasons. Int. J. Recycl. Org. Waste Agri. 8(Suppl.1), S1–S9. doi: 10.1007/s40093-019-0252-z
Ghosh, P. K., Manna, M. C., Dayal, D., and Wanjari, R. H. (2006). Carbon sequestration potential and sustainable yield index for groundnut and fallow-based cropping system. J. Agri. Sci. 144, 249–259. doi: 10.1017/S0021859606006046
Gibbs, H. K., and Salmon, J. M. (2015). Mapping of world degraded lands. Appl. Geogr. 57, 12–21. doi: 10.1016/j.apgeog.2014.11.024
Gillman, G. P., and Sumpter, E. A. (1986). Modification to the compulsive exchange method for measuring exchange characteristics of soils. Austr. J. Soil Res. 24, 61–66. doi: 10.1071/SR9860061
Goulding, K. W. T. (2016). Soil acidification and their importance of liming agricultural soils with particular reference to the United Kingdom. Soil Use Manag. 32, 390–399. doi: 10.1111/sum.12270
Grace, P. R., Post, W. M., and Hennessy, K. (2006). The potential impact of climate change on Australia's soil organic carbon resources. Carbon Balance Manag. 1:14. doi: 10.1186/1750-0680-1-14
Grose, C. J. (1999). Land Capability Handbook, - Guidelines for Classification of AGRICULTURAL LAND in Tesmania, Second Edition, Department of Primary Industrial, Water and Environment, printed by: Foot and Playsted, Launceston, Tasmansia, Australia, 70 + iv.
Gupta, R. P. (1998a). “Macro pore space analysis,” in Theory and Practices in Agrophysics Measurements, eds R. P. Gupta and B. P. Ghildyal (Mumbai: Allied Publishers Ltd.), 140–146.
Gupta, R. P. (1998b). “Particle size analysis by pipette method,” in Theory and Practices in Agrophysics Measurements, eds R. P. Gupta and B. P. Ghildyal (Mumbai: Allied Publishers Ltd.), 45–49.
Gupta, R. P. (1998c). “Penetration resistance and cone index measurements,” in Theory and Practices in Agrophysics Measurements, eds R. P. Gupta and B. P. Ghildyal (Mumbai: Allied Publishers Ltd.), 110–116.
Gupta, V. K. (2013). “Soil analysis for available micronutrients,” in Methods of Analysis of Soils, Plants, Waters Fertilizers and Organic Manures, ed H. L. S. Tandon (New Delhi: Fertilizer Development and Consultation Organization), 204 + xii.
Hafez, E. M., El Hassan, W. H. A., Gaafar, I. A., and Seleiman, M. F. (2015). Effect of gypsum application and irrigation intervals on clay saline-sodic soil characterization, rice water use efficiency, growth and yield. J. Agri. Sci. 208–219. doi: 10.5539/jas.v7n12p208
Halim, N. S. A., Abdullah, R., Karsani, S. A., Osman, N., Panhwar, Q. A., and Ishak, C. F. (2018). Influence of soil amendments on the growth and yield of rice in acidic soil. Agronomy 8:165. doi: 10.3390/agronomy8090165
Halvorson, J. J., Smith, J. L., and Papendick, R. I. (1996). Integration of multiple soil parameters to evaluate soil quality: a field example. Biol. Fertil. Soils 21, 207–214. doi: 10.1007/BF00335937
Haney, R. L., Haney, E. B., Smith, D. R., Harmel, D, and White, M. J. (2018). The Soil Health Toll- a theory and initial broad scale application. Appl. Soil Ecol. 125, 162–168. doi: 10.1016/j.apsoil.2017.07.035
Henao, J., and Baanante, C. (2006). Agricultural Production and Soil Nutrient Mining in Africa, Implications for Resource Conservation and Policy Development, Technical Bulletin. Muscle Shoals, AB: An International Center for Soil Fertility and Agricultural Development.
Herrick, J. E., and Jones, T. L. (2002). A dynamic cone penetrometer for measuring soil penetration resistance. Soil Sci. Soc. Am. J. 66, 1320–1324. doi: 10.2136/sssaj2002.1320
Heydari, N. (2019). “Water productivity improvement under saline condition: case study of the saline areas of lower Kharkheh river basin, Iran,” in Multifunctionality and Impact of Organic and Conventional Agriculture, eds J. Moudry, J. Bernas and K. F. Mendes (IntechOpen Publication), 1–20. doi: 10.5772/intechopen.86891
Hodgson, E. (2012). “Human environment: definition, scope and role of toxicology,” in Progress in Molecular Biology and Translational Science, ed E. Hodgson (Cambridge, MA: Academic Press), 1–10.
Idowu, J., Ghimire, R., Flynn, R., and Ganguli, A. (2019). Soil Health: Importance, Assessment and Management. Cooperative Extension Service, College of Agriculture, Consumer and Environmental Sciences, Circular 694B aces.nmsu.edu/pubs. Available online at: http://aces.nmsu.edu/pubs/_circulars/CR694B/welcome/html (accessed December 9, 2020).
Indira Devi, P., Thomas, J., and Raju, R. K. (2017). Pesticide consumption in India: a spatiotemporal analysis. Agri. Econ. Res. Rev. 30, 163–172. doi: 10.5958/0974-0279.2017.00015.5
Islam S.M. D. U. Bhuiyan M. A. H. Mohinuzzaman M. Ali Md,. H And Moon S. R. (2017). A soil health card (SHC) for soil quality monitoring of agricultural lands in south-eastern costal region of Bangladesh. Environ. Syst. Res. 6:15. doi: 10.1186/s40068-017-0092-7
Jat, H. S., Datta, A., Sharma, P. C., Kumar, V., Yadav, A. K., Choudhary, M., et al. (2018). Assessing soil properties and nutrient availability under conservation agriculture practices in a reclaimed sodic soil in cereal-based systems of North-West India. Archiv. Agron. Soil Sci. 64, 531–545. doi: 10.1080/03650340.2017.1359415
Jat, M. L., Majumdar, K., McDonald, A., Sikka, A. K., and Porada, R. S. (2015). Book of Extended Summaries. National Dialogue on Efficient Nutrient Management for Improving Soil Health, September 28-29, 2018. New Delhi: TAAS, ICAR, CIMMYT, IPNI, CSISA, FAI, 56.
Jehangir, I. A., Khan, M. H., and Bhat, Z. A. (2013). Strategies for increasing crop production and productivity in problem soils. Int. J. Forestry Soil Environ. 3, 73–78. Available online at: https://www.ijfse.com/uploadedfiles/IJFSEArchive/IJFSE2013/3(2)/05.pdf
Jian, J., Du, X., and Stewart, R. D. (2020). A database for global soil health assessment. Sci. Data 7:16. doi: 10.1038/s41597-020-0356-3
Johnson, A. I. (1963). A Field Method for Measurement of Infiltration. Geological survey water supply paper 1544-F. Washington, DC: United State Government Printing Office, F-26.
Jones, D. L., Cross, P., Withers, P. J. A., DeLuca, T. H., Robinson, D. A., Quillium, R. S., et al. (2013). Nutrient stripping: the global disparity between food security and soil nutrient stocks. J. Appl. Ecol. 50, 851–862. doi: 10.1111/1365-2664.12089
Karlen, D. L., and Rice, C. W. (2015). Soil degradation: will humankind ever learn? Sustainability 7, 12490–12501. doi: 10.3390/su70912490
Kassam, A., Friedrich, T., and Derpsch, R. (2019). Global spread of conservation agriculture. Int. J. Environ. Stud. 76, 29–51. doi: 10.1080/00207233.2018.1494927
Katyal, J. C., Datta, S. P., and Golui, D. (2016). Global review on state of soil health. In: Katyal, JC, Chaudhari, SK, Dwivedi, BS, Biswas, DR, Rattan, RK and Majumdar, K (Editors) (2016) Soil Health: Concept, Status and Monitoring. Bullet. Ind. Soc. Soil Sci. 30, 1–13.
Kaur, P., and Kaur, L. (2019). Effects of dangerous chemicals present in the environment on the health of rural peoples in the south western region of Punjab. J. Pharmacogn. Phytochemistr. SP4, 159–162. Available online at: https://www.phytojournal.com/archives/2019/vol8issue4S/PartE/SP-8-4-43-808.pdf
Kemper, W. D. (1965). Method of soil analysis: part 1 physical and mineral properties, including statistics of measurement and sampling 9, 511–519. doi: 10.2134/agronmonogr9.1
Khan, A., Khan, S., Khan, M. A., Qamar, Z., and Waqas, M. (2015). The uptake and bioaccumulation of heavy metals by food plants, their effects on plants nutrients and associated health risk: a review. Environ. Sci. Pollut. Res. 22, 13772–13799. doi: 10.1007/s11356-015-4881-0
Khanna, R., and Gupta, S. (2018). Agrochemicals as a potential cause of groundwater pollution: a review. Int. J. Chem. Stud. 6, 985–990. Available online at: https://www.chemijournal.com/archives/2018/vol6issue3/PartO/6-3-164-332.pdf
Kibblewhite, M. G., Ritz, K., and Swift, M. J. (2008). Soil health in agricultural system. Philos. Trans. Royal Soc. B 363, 685–701. doi: 10.1098/rstb.2007.2178
Kiran, S. C., Nagarajaiah, C., Murthy, M. M., and Ranjith, P. C. (2020). Effect of municipal solid waste open dumping on soil, water, crop, human health and its prospective. Int. J. Environ. Climate Change 10, 36–45. doi: 10.9734/ijecc/2020/v10i830216
Koch K. Chappell A. Eyres M. And Scott E. (2015). Monitor soil degradation or triage of soil security: an Australian Challenge. Sustainability 7, 4870–4892. doi: 10.3390/su7054870
Kulasekaran, R., Biswas, A. K., and Patra, A. K. (2015). Zeolitic farming. Ind. J. Agron. 60, 185–191. doi: 10.4103/0019-5154.152525
Kumar, D., Shivay, Y. S., Shiva, D., Kumar, C., and Prasad, R. (2013). Phizospheric flora and influence of agronomic practices on them: a review. Proc. Natl. Acad. Sci. India Sec. B Biol. Sci. 83, 1–14. doi: 10.1007/s40011-012-0059-4
Kumar, M., Jha, A. K., Hazarika, S., Verma, B. C., Choudhury, B. U., Ramesh, T., et al. (2016). Micronutrients (B, Zn, Mo) for improving crop production on acidic soils of northeast India. Natl. Acad. Sci. Lett. 39, 85–89. doi: 10.1007/s40009-015-0409-x
Kumar, R., Chatterjeea, D., Kumawat, N., Pandey, A., Roy, A., and Kumar, M. (2014). Productivity, quality and soil health as influenced by lime in rice bean cultivars in foothills of north-eastern India. Crop J. 2, 338–344. doi: 10.1016/j.cj.2014.06.001
Kumar, S., Meena, R. S., Lal, R., Yadav, G. S., Mitran, T., Meena, B. L., et al. (2018). “Role of legumes in soil carbon sequestration,” in Legumes for Soil Health and Sustainable Management, eds R. Meena, A. Das, G. Yadav, R. Lal (Singapore: Springer), 109–138. doi: 10.1007/978-981-13-0253-4_4
Kumar, S., Smith, S. R., Fowler, G., Velis, C., Kumar, S. J., Arya, S., Rena, K.umar R., and Cheeseman, C. (2017). Challenges and opportunities associated with waste management in India. Royal Soc. Open Sci. 4:160764. doi: 10.1098/rsos.160764
Kumari, K., and Phogat, V. K. (2008). Rock phosphate: its availability and solubility and solubilization in the soil. Agri. Rev. 29, 108–116. Available online at: https://arccjournals.com/journal/agricultural-reviews/ARCC2494
Lal, R. (2005). World Crop residue production and implications of its use as a biofuel. Environ. Int. 31, 575–584. doi: 10.1016/j.envint.2004.09.005
Lal, R. (2016). Soil health and carbon management. Food Energy Security 5, 212–222. doi: 10.1002/fes3.96
Lal, R., and Moldenhauer, W. C. (1987). Effects of soil erosion on crop productivity. Crit. Rev. Plant Sci. 5, 303–367. doi: 10.1080/07352688709382244
Le Bissonnais, Y. (1996). Aggregate stability and measurement of soil crustability and erodibility: I. Theory and methodology. Eur. J. Soil Sci. 47, 425–437. doi: 10.1111/j.1365-2389.1996.tb01843.x
Le, Q. B., Nkonya, E., and Mirzabaev, A. (2014). Biomass Productivity-based mapping of global land degradation hotspots; ZEF-Discussion Papers on Development Policy No. 193. Bonn: University of Bonn.
Lehman, R. M., Cambardella, C. A., Stott, D. E., Martinez, V. A., Manter, D. K., Buyer, J. S., et al. (2015). Understanding and enhancing soil biological health: the solution for reversing soil degradation. Sustainability 7, 988–1027. doi: 10.3390/su7010988
Lindsay, W. L., and Norvell, W. A. (1978). Development of DTPA soil test for zinc, iron, manganese and copper. Soil Sci. Soc. America J. 42, 421–428. doi: 10.2136/sssaj1978.03615995004200030009x
Magdoff, F. (2001). Concept, components and strategies of soil health in agroecosystems. J. Nematol. 33, 169–172.
Maitra, S., and Zaman, A. (2017). Brown manuring, an effective technique for yield sustainability and weed management of cereal crops: a review. Int. J. Bioresour. Sci. 4, 1–5. doi: 10.5958/2454-9541.2017.00001.9
Maity, S. K., and Mukherjee, P. K. (2009). Effect of brown manuring on grain yield and partial factor productivity of nutrients in dry direct seeded summer rice (Oryza sativa L.) under Terai agro-ecological region of West Bengal. J. Crop Weed 5, 31–35. Available online at: https://www.cropandweed.com/archives/2009/vol5issue2/7.pdf
Mandal, B., Majumdar, B., Bandyopadhyay, P. K., Hazra, G. C., Gangopadhyoy, A., Samantaray, R. N., et al. (2007). The potential of cropping systems and soil amendments for carbon sequestration in soils under long-term experiments in subtropical India. Glob. Change Biol. 13, 357–368. doi: 10.1111/j.1365-2486.2006.01309.x
Mandal, U. K., Singh, G., Victor, U. S., and Sharma, K. L. (2003). Green manuring: its effects on soil properties and crop growth under rice-wheat cropping system. Eur. J. Agron. 19, 225–237. doi: 10.1016/S1161-0301(02)00037-0
Mantel, S., and Van Engelen, V. W. P. (1997). The impact of land degradation on food productivity- Case studies of Uruguvay, Argentina and Kenya. Volume 1: Main report. Report 97/01. Wagenningen, DC: International Soil Reference and Information Centre, 44+iv.
Manyevere, A., Bangira, C., Gotosa, J., Munjonji, L., and Chikwari, E. (2015). Characteristics and management options of crusting soils in a smallholder farming area of the Zambezi metamorphic belt in northern Zimbabwe. South African J. Plant Soil 32, 157–164. doi: 10.1080/02571862.2015.1018355
McGonigle, T. P., Millers, S. M. H., Evans, D. G., Fairchild, G. L., and Swan, J. A. (1990). A new method which gives an objective measure of colonization of roots by vesicular-arbuscular mycorrhizal fungi. New Phytol. 115, 495–501. doi: 10.1111/j.1469-8137.1990.tb00476.x
McKenzie, B. M., Tisdall, J. M., and Vance, W. H. (2011). “Soil physical quality,” in Encyclopedia of Agrophysics, Encyclopedia of Earth Sciences Series, eds J. Glinski, J. Horabik, and J. Lipiec (Dordrecht: Springer), 770–777.
Meena, R. S., Kumar, S., Datta, R., Lal, R., Vijaykumar, V., Betnicky, M., et al. (2020). Impacts of agrochemicals on soil microbiota and management: a review. Land 9:34. doi: 10.3390/land9020034
Middleton, N., and Thomas, D. (1997). World Atlas of Desertification. 2nd Edn. United Nation Environment Programme. London: Arnold publisher, 182+vii. Available online at: http://hdl.handle.net/2050011822/30300 (assessed December 27, 2020).
Mirzabaev, A., Wu, J., Evans, J., García-Oliva, F., Hussein, I. A. G., Iqbal, M. H., et al. (2019). “Desertification,” in Climate Change and Land: An IPCC Special Report on Climate Change, Desertification, Land Degradation, Sustainable Land Management, Food Security, and Greenhouse Gas Fluxes in Terrestrial Ecosystems, eds P. R. Shukla, J. Skea, E. Calvo Buendia, V. Masson-Delmotte, H.-O. Pörtner, D. C. Roberts, et al. (IPCC).
Mishra, B. N., Prasad, R., Gangaiah, B., and Shivakumar, B. G. (2006a). Organic manure for increased productivity and sustained supply of micronutrients zinc and copper in rice-wheat cropping system. J. Sustain. Agri. 28, 55–66. doi: 10.1300/J064v28n01_06
Mishra, U., and Dhar, D. W. (2004). Biodiversity and biological degradation of soil. Resonance 9, 26–33. doi: 10.1007/BF02902526
Mishra, V. K., Sara, R., and Singh, R. K. (2006b). Physical characterization of soils under different land use systems using non-limiting water range as a soil physical index. J. Ind. Soc. Soil Sci. 54, 146–150.
Mishra, V. K., Srivastava, S., Bhardwaj, A. K., Sharma, D. K., Singh, Y. P., and Nayak, A. K. (2015). Resource conservation strategies for rice-wheat cropping systems on partially reclaimed sodic soils of the indo-Gangetic region and their effects on soil carbon. Nat. Resour. For. 39, 110–122. doi: 10.1111/1477-8947.12071
Morin, J. (1993). “Soil crusting and sealing,” in Soil and Tillage in Africa: Need and Challenges, FAO Soil Bulletin 69, Editorial Group. Rome: FAO information Division. Available online at: http://www.fao.org/3/T1696E/t1696e06.htm (accessed December 3, 2020).
Mortvedt, J. J. (1995). Heavy metal contaminants in inorganic and organic fertilizers. Fertil. Res. 43, 55–61. doi: 10.1007/BF00747683
Murtaza, B., Murtaza, G., Imran, M., Amjad, M., Naeem, A., Shah, G. M., et al. (2017). Yield and nitrogen use efficiency in rice-wheat cropping system in gypsum amended saline-sodic soil. J. Soil Sci. Plant Nutr. 17, 686–701. doi: 10.4067/S0718-95162017000300011
Mythili, G., and Goedecke, J. (2016). “Economics of land degradation in India,” in Economics of Land Degradation and Improvement – A Global Assessment for Sustainable Development, eds E. Nkonya, A. Mirzabaev, and J. Von Braun (Washington, DC: Springer Open), 431–469. doi: 10.1007/978-3-319-19168-3_15
NAAS (2018). Soil Health: New Policy Initiatives for Farmers Welfare. Policy Brief No. 3. New Delhi: National Academy of Agricultural Sciences, 19.
Nawaz, A., Farooq, M., Lal, R., Rehman, A., Hussain, T., and Nadeem, A. (2016). Influence of Sesbania brown manuring and rice residue mulch on soil health, weeds and system productivity of conservation rice-wheat systems. Land Degrad. Dev. 28, 1078–1090. doi: 10.1002/ldr.2578
Nayak, S., Prasanna, R., Pabby, A., Dominic, T. K., and Singh, P. K. (2004). Effect of urea and BGA-Azolla bio-fertilizers on nitrogen fixation and chlorophyll accumulation in soil cores from rice fields. Biol. Fertil. Soils 40, 67–72. doi: 10.1007/s00374-004-0738-2
Nayak, S. K., Dash, B., and Baliyarsingh, B. (2018). “Microbial remediation of persistent agro-chemicals by soil bacteria: an overview,” in Microbial Biotechnology, eds J. Patra, G. Das, H. S. Shin (Singapore: Springer), 13. doi: 10.1007/978-981-10-7140-9_13
Nciizah, A. D., and Wakindiki, I. I. C. (2015). Soil sealing and crusting effects on infiltration rate: a critical review of shortfalls in prediction models and solutions. Archiv. Agron. Soil Sci. 61, 1211–1230. doi: 10.1080/03650340.2014.998203
Nelson, D. W., and Sommers, L. E. (1980). Total nitrogen analysis of soil and plant tissues. J. Assoc. Off. Analyt. Chemists 63, 770–778. doi: 10.1093/jaoac/63.4.770
Nia, S. H., Zarea, M. J., Rejali, F., and Verma, A. (2012). Yield and yield components of wheat as affected by salinity and inoculation with Azospirillum strains. J. Saudi Soc. Agri. Sci. 11, 113–121. doi: 10.1016/j.jssas.2012.02.001
Nielsen, K. B., Kjoller, R., Bruun, H. H., Schnoor, T. K., and Rosendahl, S. (2016). Colonization of new land by arbuscular mycorrhizal fungi. Fungal Ecol. 20, 22–29. doi: 10.1016/j.funeco.2015.10.004
Nielsen, M. N., and Winding, A. (2002). Microorganisms as Indicator of Soil Health. Denmark: National Environmental Research Institute. Technical Report No. 388.
Norment, C. J. (1987). A comparison of three methods for measuring arthropod abundance in Tundra habitats and its implications in avian ecology. Northwest Sci. 61, 191–198.
Nunan, N., Morgan, M. A., and Herlihy, M. (1998). Ultraviolet absorbance (280 nm) of compounds released from soil during chloroform fumigation as an estimate of the microbial biomass. Soil Biol. Biochem. 30, 1599–1603. doi: 10.1016/S0038-0717(97)00226-5
Obalum, S. E., Chibuike, G. U., Peth, S., and Ouyang, Y. (2017). Soil organic matter as sole indicator of soil degradation. Environ. Monitor. Syst. 189:176. doi: 10.1007/s10661-017-5881-y
Odum, E. P. (1969). The strategy of ecosystem development. Science 164, 262–270. doi: 10.1126/science.164.3877.262
Oerke, E. C. (2006). Centenary review- crop losses to pests. J. Agri. Sci. 144, 31–43. doi: 10.1017/S0021859605005708
Okrent, D. (1999). On intergenerational equity and its clash with intragenerational equity and on the need for policies to guide the regulation of disposal of wastes and other activities posing very long time risks. Risk Anal. 19, 877–901. doi: 10.1111/j.1539-6924.1999.tb00449.x
Olsen, S. R., Cole, C. V., Watanabe, F. S., and Dean, L. (1954). “Estimation of available phosphorus in soil by extraction with sodium carbonate,” in USDA Conc (Washington, DC), 933.
Olsen, S. R., and Sommers, L. E. (1982). “Phosphorus,” in Methods of Soil Analysis, Part 2: Chemical and Microbiological Properties, 2nd Edn, ed A. L. Page (Madison, WI: American Society of Agronomy), 406–407.
Olsson, L., Barbosa, H., Bhadwal, S., Cowie, A., Delusca, K., Flores-Renteria, D., et al. (2019). “Land degradation,” in Climate Change and Land: An IPCC Special Report on Climate Change, Desertification, Land Degradation, Sustainable Land Management, Food Security, and Greenhouse Gas Fluxes in Terrestrial Ecosystems, eds P. R. Shukla, J. Skea, E. Calvo Buendia, V. Masson-Delmotte, H.-O. Pörtner, D. C. Roberts, et al. (IPCC).
Pagliai, M., and Painuli, D. K. (1998). “Soil porosity and structure quantification by image analysis and soil micromorphology,” in Theory and Practices in Agrophysics Measurements, eds R. P. Gupta and B. P. Ghildyal (Mumbai: Allied Publishers Ltd), 128–139.
Pagliai, M., and Stoops, G. (2010). “Physical and biological crusts and seals,” in Interpretation of Micromorphological Features of Soils and Regoliths, eds G. Stoops, V. Maecelino and F. Mees (Amsterdam: Elsevier), 419–440. doi: 10.1016/B978-0-444-53156-8.00019-2
Panagos, P., Standardi, G., Borrelli, P., Lugato, E., Montanarella, L., and Bosello, F. (2018). Cost of agricultural productivity loss due to soil erosion in the European Union: from direct cost evaluation approaches to the use of macroeconomic models. Land Degrad. Dev. 29, 471–484. doi: 10.1002/ldr.2879
Patel, T. R., and McFadden, B. A. (1976). A simple spectrophotometric method for measurement of nematode populations. Analyt. Biochem. 70, 447–453. doi: 10.1016/0003-2697(76)90469-3
Pattanayak, S. K., Mishra, U. K., Sarkar, A. K., and Majumdar, K. (2011). Integrated nutrient management for groundnut and redgram on acid soils in Odisha. Better Crops South Asia 2011, 8–10. Available online at: http://www.ipni.net/publication/bca.nsf/0/E74D54E10E51925A852579A4007A2931/$FILE/Page%208%20BCSA%202011.pdf
Pawlas, A. K., Jaruga, A. U., and Smreczak, B. (2019). Soil quality index for agricultural areas under different levels of anthropopressure. Int. Agrophys. 33, 455–462. doi: 10.31545/intagr/113349
Pierce, F. J., and Lal, R. (1994). “Monitoring the impact of soil erosion on crop productivity,” in Soil Erosion: Research Methods, 2nd Edn, ed R. Lal (Ankeny, IA: Soil and Water Conservation Society), 235–263. doi: 10.1201/9780203739358-10
Pieters, A. J. (1927). Green Manuring: Principles and Practices. New York, NY: John Wiley and Sons, Inc.
Pimentel, D., and Burgess, M. (2013). Soil erosion threatens food production. Agriculture 3, 443–463. doi: 10.3390/agriculture3030443
Pooniya, V., Shivay, Y. S., Rana, A., Nain, L., and Prasanna, R. (2012). Enhancing soil nutrient dynamics and productivity of Basmati rice through residue incorporation and zinc fertilization. Eur. J. Agron. 41, 28–37. doi: 10.1016/j.eja.2012.03.004
Power, A. G. (2013). “Ecology of agriculture,” in Encyclopaedia of Biodiversity, 2nd Edn, ed S. A. Levin (Cambridge, CA: Academic Press), 9–15. doi: 10.1016/B978-0-12-384719-5.00006-X
Prasad, R., Hobbs, P., and Shivay, Y. S. (2018). New frontiers in phosphate fertilizers. Ind. J. Fertilisers 14, 20–26.
Prasad, R., Shivay, Y. S., Kumar, D., and Sharma, S. N. (2006). Learning by Doing Exercises in Soil Fertility (A Practical Manual for Soil Fertility). New Delhi: Division of Agronomy, Indian Agricultural Research Institute, 68.
Qaswar, M., Huang, J., Ahmed, W., Li, D., Liu, S., Ali, S., et al. (2019). Long term green manure rotations improve soil biochemical properties, yield sustainability and nutrient balances in acidic paddy soil under rice-based cropping system. Agronomy 9:780. doi: 10.3390/agronomy9120780
Qureshi, J. N., and Njihia, C. M. (1984). “Soil related constraints to crop production in East Africa,” in Proceedings of the 6th Annual General Meeting of the Soil Science Society of East Africa (Nairobi: Kenya Soil Survey).
Ramesh, K., and Chandrasekaran, B. (2004). Soil organic carbon build up and dynamics in rice-rice cropping system. J. Agron. Crop Sci. 190, 21–27. doi: 10.1046/j.0931-2250.2003.00069.x
Rao, A. S., and Reddy, K. S. (2013). “Analysis of soils for pH, EC and available major nutrients,” in Methods of Analysis of Soils, Plants, Waters Fertilizers and Organic Manures, ed H. L. S. Tandon (New Delhi: Fertilizer Development and Consultation Organization), 204 + xii.
Rao, Y. N., and Jo, I. S. (1998). “Pore space analysis by using mercury intrusion porosimetry,” in Theory and Practices in Agrophysics Measurements, eds R. P. Gupta and B. P. Ghildyal (Mumbai: Allied Publishers Ltd.), 117–126.
Rattan, R. K., Kumar, M., Narwal, R. P., and Singh, A. P. (2009). “Soil health and nutritional Security-Micronutrients,” in Proceedings of Platinum Jubilee Symposium of Indian Society of Soil Science, 249–265.
Ray, S. S., and Gupta, R. P. (2001). Effect of green manuring and tillage practices on physical properties of puddled loam soil under transplanted rice-wheat cropping system. J. Ind. Soc. Soil Sci. 49, 670–678. Available online at: https://www.indianjournals.com/ijor.aspx?target=ijor:jisss&volume=49&issue=4&article=012
Reddy, A. A. (2017). Impact Study of Soil Health Card Scheme. Hyderabad: National institute of Agricultural Extension management (MANAGE), 210+ xxiii.
Reddy, C. V., Tiwari, A., Tedia, K., Verma, A., and Saxena, R. P. (2017). Long term fertilizer experiment on soil properties and yield of rice on chromustert. Int. J. Farm Sci. 7, 87–91. doi: 10.20546/ijcmas.2017.611.012
Ribeiro-Barros, A. I., Silva, M. J., Moura, I., Ramalho, J. C., Maguas-Hanson, C., and Ribeiro, N. S. (2018). “The potential of tree and shrub legumes in agroforestry systems,” in Nitrogen in Agriculture Updates, ed Amanullah (IntechOpen), 223–240. doi: 10.5772/intechopen.69995
Richards, L. A., and Weaver, L. R. (1943). Pressure-plate apparatus for measuring moisture sorption and transmission by soils. Soil Sci. 56, 395–404. doi: 10.1097/00010694-194312000-00001
Rickson, R. J., Deeks, L. K., Graves, A., Harris, J. A. H., Kibblewhite, M. G., and Sakrabani, R. (2015). Input constraints to food production: The impact of soil degradation. Food Security 7, 351–367. doi: 10.1007/s12571-015-0437-x
Roberts, T. L., and Johnston, A. E. (2015). Phosphorus use efficiency and management in agriculture. Resour. Conserv. Recycl. 105, 275–281. doi: 10.1016/j.resconrec.2015.09.013
Rodale, J. I. (1945). Pay Dirt: Farming and Gardening with Composts. New York, NY: Devin Adair Company.
Romig, D. E., Garlynd, M. J., Harris, R. F., and McSweeney, K. (1995). How farmers assess soil health and quality. J. Soil Water Cons. 50, 229–236.
Roy Chowdhury, S., Nayak, A. K., Brahmanand, P. S., Mohanty, R. K., Chakraborty, S., Kumar, A., and Ambast, S. K. (2018). Delineation of Waterlogged Areas using Spatial Techniques for Suitable Crop Management in Eastern India. Bulletin No.79. Bhubaneswar: ICAR Indian Institute of Water Management, 44.
Sadh, P. K., Duhan, S., and Duhan, J. S. (2018). Agro-industrial waste and their utilization using solid state fermentation: a review. Bioresour. Bioproces. 5:1. doi: 10.1186/s40643-017-0187-z
Saha, S. K. (2003). “Water and wind induced soil erosion assessment and monitoring using remote sensing and GIS,” in Satellite Remote Sensing and GIS Applications in Agricultural Meteorology, Proceeding of Workshop, eds M. V. K. Sivakumar, P. S. Roy, K. Harmsen and S. K. Saha, 315–330.
Saha, S. S., Saha, B. N., Hazra, G. C., Pati, S., Pal, B., Kundu, D., et al. (2018). Assessing the suitability of sewage-sludge produced in Kolkata, India for their agricultural use. Proc. Ind. Natl. Sci. Acad. 84, 781–792. doi: 10.16943/ptinsa/2018/49410
Sahoo, U. K., Singh, S. L., Gogoi, A., Kenye, A., and Sahoo, S. S. (2019). Active and passive soil organic carbon pools as affected by different land use types in Mizoram, Northeast India. PLoS ONE 14:e0219969. doi: 10.1371/journal.pone.0219969
Samineni, S., Siddique, K. H. M., Gaur, P. M., and Colmer, T. D. (2011). Salt sensitivity of the vegetative and reproductive stages in chickpea (Cicer arietinum L.): podding is a particularly sensitive stage. Environ. Exp. Bot. 71, 260–268. doi: 10.1016/j.envexpbot.2010.12.014
Scofield, A. M. (1986). Organic farming – the origin of the name. Biol. Agri. Horticult. 4, 1–5. doi: 10.1080/01448765.1986.9754481
Sekhar, M., Tomer, S. K., Thiyaku, S., Giriraj, P., Murthy, S., and Mehta, V. K. (2018). Groundwater level dynamics in Bengaluru city, India. Sustainability 10:26. doi: 10.3390/su10010026
Selvi, A., Rajasekar, A., Theerthagiri, J., Ananthaselvam, A., Sathishkumar, K., Madhavan, J., et al. (2019). Integrated remediation processes toward heavy metal removal/recovery from various environments-a review. Front. Environ. Sci. 7:66. doi: 10.3389/fenvs.2019.00066
Shaheenuzzamn, M.d. (2014). Screening of chickpea genotypes against salinity stress. Bangladesh J. Agri. Res. 39, 605–619. doi: 10.3329/bjar.v39i4.22539
Shahid, S. A., Zaman, M., and Heng, L. (2018). “Soil salinity: historical perspectives and a world overview of the problem,” in Guidelines for Salinity Assessment, Mitigation and Adaptation Using Nuclear and Related Techniques (Chem: Springer), 43–53. doi: 10.1007/978-3-319-96190-3_2
Shamshuddin, J., Panhwar, Q. A., Shazana, M. A. R. S., Elisa, A. A., Fauziah, C. I., and Naher, U. A. (2016). Improving the productivity of acid sulfate soils for rice cultivation using limestone, basalt, organic fertilizer and/or their combinations. Sains Malaysiana 45, 383–392. Available online at: http://www.ukm.my/jsm/pdf_files/SM-PDF-45-3-2016/08%20J.%20Shamshudin.pdf
Sharma, D. K., Singh, A., Sharma, P. C., Dagar, J. C., and Chaudhari, S. K. (2016). Sustainable management of sodic soils for crop production: opportunities and challenges. J. Soil Salinity Water Qual. 8, 109–130.
Sharma, G., Sharma, L. K., and Sharma, K. C. (2019). Assessment of land use change and its effect on soil carbon stock using multitemporal satellite data in semiarid region of Rajasthan, India. Ecol. Proces. 8:42. doi: 10.1186/s13717-019-0193-5
Sharma, S. N., and Prasad, R. (2003). Yield and P uptake by rice and wheat grown in a sequence as influenced by phosphate fertilization with diammonium phosphate and Mussoorie rock phosphate with or without crop residues and phosphate solubilizing bacteria. J. Agri. Sci. 141, 359–369. doi: 10.1017/S0021859603003678
Shetty, R., and Prakesh, N. B. (2020). Effect of different biochars on acid soil and growth parameters of rice plants under aluminium toxicity. Sci. Rep. 10:11249. doi: 10.1038/s41598-020-69262-x
Shivay, Y. S., Krogstad, T., and Singh, B. R. (2010). Mineralization of copper, manganese and zinc from rock mineral flour and city waste compost for efficient use in organic farming. Plant Soil 326, 425–435. doi: 10.1007/s11104-009-0023-0
Sims, G. K. (1990). “Biological degradation of soil,” in Advances in Soil Science, eds R. Lal, and B. A. Stewart (New York, NY: Springer), 289–330. doi: 10.1007/978-1-4612-3322-0_8
Singh, A. K., Arora, S., Singh, Y. P., Verma, C. L., Bhardwaj, A. K., and Sharma, N. (2018). Water use in rice crop through different methods of irrigation in a sodic soil. Paddy Water Environ. 16, 587–593. doi: 10.1007/s10333-018-0650-2
Singh, B. K., Campbell, C. D., Sorenson, S. J., and Zhou, J. (2009a). Soil genomics. Nat. Rev. Microbiol. 7:756. doi: 10.1038/nrmicro2119-c1
Singh, D., Chhonkar, P. K., and Pandey, R. N. (1999). Soil, Plant, Water Analysis – A Methods Manual. New Delhi: Division of Soil Science and Agricultural Chemistry, IARI.
Singh, G., Babu, R., Narain, P., Bhushan, L. S., and Abrol, I. P. (1992). Soil erosion rates in India. J. Soil Water Conserv. 47, 97–99.
Singh, R. P., Singh, P. K., and Singh, A. K. (2009b). Effect of green manuring on physic-chemical properties of soil and productivity of rice. Oryza 46, 120–123.
Singh, V. K., Dwivedi, B. S., Tiwari, K. N., Majumdar, K., Rani, M., Singh, S. K., et al. (2014). Optimizing nutrient management strategies for rice–wheat system in the Indo-Gangetic Plains of India and adjacent region for higher productivity, nutrient use efficiency and profits. Field Crop Res. 164, 30–44. doi: 10.1016/j.fcr.2014.05.007
Singh, Y. P., Singh, R., Sharma, D. K., Mishra, V. K., and Arora, S. (2016). Optimizing gypsum levels for amelioration of sodic soils to enhance grain yield and quality of rice (Oryza sativa L.). J. Ind. Soc. Soil Sci. 64, 33–40. doi: 10.5958/0974-0228.2016.00005.0
Song, G., Li, L., Pan, G., and Zhang, Q. (2005). Topsoil organic carbon storage of china and its loss by cultivation. Biogeochemistry 74, 47–65. doi: 10.1007/s10533-004-2222-3
Song, G., Li, S., and Hui, R. (2017). Effect of biological soil crusts on seed germination and growth of an exotic and two native plant species in an arid ecosystem. PLoS ONE 12:e0185839. doi: 10.1371/journal.pone.0185839
Sonneveld, B. G. J. S., Keyzer, M. A., and Ndiaye, D. (2016). Quantifying the impact of land degradation on crop production: the case of Senegal. Solid Earth 7, 93–103. doi: 10.5194/se-7-93-2016
Sterk, G., and Stoorvogel, J. J. (2020). Desertification: scientific versus political realities. Land 9:156. doi: 10.3390/land9050156
Stewart, W. D. P., Fitzgerald, G. P., and Burris, R. H. (1967). In-situ studies on N2 fixation using the acetylene reduction technique. Proc. Natl. Acad. Sci. U. S. A. 58, 2071–2078. doi: 10.1073/pnas.58.5.2071
Subbiah, B. V., and Asija, G. L. (1956). A rapid procedure for assessment of available nitrogen in rice soils. Curr. Sci. 25, 259–260.
Sur, H. S., and Gupta, R. P. (1998). “Infiltration rate measurements,” in Theory and Practices in Agrophysics Measurements, eds R. P. Gupta and B. P. Ghildyal (Mumbai: Allied Publishers Ltd.), 191–194.
Swaminathan, M. S. (1987). “The promise of agroforestry for ecological and nutritional security,” in Agroforestry: A Decade of Development, eds H. A. Steppler and P. K. R. Nair (Nairobi: International Council for Research in Agroforestry), 25–41.
Swarnalakshmi, K., Prasanna, R., Kumar, A., Pattnaik, S., Chakravarty, K., Shivay, Y. S., et al. (2013). Evaluating the influence of novel cyanobactrial biofilmedbiofertilizers on soil fertility and plant nutrition of wheat. Eur. J. Soil Biol. 55, 107–116. doi: 10.1016/j.ejsobi.2012.12.008
Tabatabai, M. A., and Bremner, J. M. (1969). Use of p-Nitrophenyl phosphate for assay of soil phosphatase activity. Soil Biol. Biochem. 1, 301–307. doi: 10.1016/0038-0717(69)90012-1
Tabatabai, M. A., and Bremner, J. M. (1972). Assay of urease activity in soils. Soil Biol. Biochem. 4, 479–487. doi: 10.1016/0038-0717(72)90064-8
Tahat, M. M., Alananbeh, K. M., Othman, Y. A., and Lescovar, D. I. (2020). Soil health and sustainable agriculture. Sustainability 12:4859. doi: 10.3390/su12124859
Tandon, H. L. S. (2013). Methods of Analysis of Soils, Plants, Waters, Fertilizers and Organic Manures. New Delhi: Fertilizer Development and Consultation Organization, 204 + xii.
Timsina, J., Singh, V. K., and Majumdar, K. (2013). Potassium management in rice-maize systems in south Asia. J. Plant Nutr. Soil Sci. 176, 317–330. doi: 10.1002/jpln.201200253
Tingwu, L., Juan, X., Guangyong, L., Jianhua, M., Jianping, W., Zhizhong, L., et al. (2003). Effect of drip irrigation with saline water on water use efficiency and quality of watermelons. Water Resour. Manag. 17, 395–408. doi: 10.1023/B:WARM.0000004917.16604.2c
Tottonell, P. (2014). Ecological intensification of agriculture- sustainable by nature. Curr. Opin. Environ. Sustainabil. 8, 53–61. doi: 10.1016/j.cosust.2014.08.006
USDA (1936). Agricultural Adjustment Administration, Soil Health and National Wealth. Washington, DC: USDA.
USDA (1961). Land capability classification, Agriculture Handbook No. 210.Soil conservation service. Washington, DC: U.S. Department of Agriculture, U.S. Government Printing Office, 21 + iii.
Valadares, R. V., Avila-Silva, L. D., Teixeira, R. D. S., De Sousa, R. N., and Vergutz, L. (2016). “Green manures and crop residues as source of nutrients in tropical environment,” in Organic Fertilizers – From Basic Concepts to Applied Outcomes, eds M. L. Larramendy and S. Soloneski (IntechOpen publication). Available online at: https://www.intechopen.com/books/organic-fertilizers-from-basicconcepts-to-applied-outcomes/green-manures-and-crop-residues-assource-of-nutrients-in-tropical-environment (accessed November 20, 2020).
Valentin, C., and Brasson, L. M. (1992). Morphology, genesis and classification of surface crusts in loamy and sandy loam soil. Geoderma 55, 225–245. doi: 10.1016/0016-7061(92)90085-L
Valentin, C., and Brasson, L. M. (1997). “Soil crusting,” in Methods for Assessment of Soil Degradation, Advances in Soil Science, eds R. Lal, W. H. Blum, C. Valentine and B. A. Stewart (Boca Raton, FL: CRC Press), 89–107.
Van Bruggen, A. H. C., and Semenov, A. M. (2000). In search of biological indicators for soil health and disease suppression. Appl. Soil Ecol. 15, 13–24. doi: 10.1016/S0929-1393(00)00068-8
Walia, M., and Goyal, S. (2010). Effect of heavy metal contaminated sewage sludge on soil microbiological properties and growth of India mustard. Archiev. Agron. Soil Sci. 56, 563–574. doi: 10.1080/03650340903165923
Walkley, A. J., and Black, I. A. (1934). An examination of the Degtjareff method for determination of soil organic matter and a proposed modification of the chromic acid titration method. Soil Sci. 37, 29–38. doi: 10.1097/00010694-193401000-00003
Wallace, H. A. (1910). Relation Between Livestock Farming and the Fertility of the Land. Retrospective Theses and Dissertations, 98. Available online at: http://lib.dr.iastate.edu/rtd/98 (accessed November 20, 2020).
Wander, M. M., Cihacek, L. J., Coyne, M., Drijber, R. A., Grossman, J. M., Gutknecht, J. L. M., et al. (2019). Developments in agricultural soil quality and health: reflections by the research committee on soil organic matter management. Front. Environ. Sci. 7:109. doi: 10.3389/fenvs.2019.00109
Weyers, S. L., Schomberg, H. H., Hendrix, P. F., Spokas, K. A., and Endale, D. M. (2008). Construction of an electrical device for sampling earthworm populations in the field. Appl. Eng. Agri. 24, 391–397. doi: 10.13031/2013.24492
Wiebe, K. (2003). Linking land quality, agricultural productivity and food security. Resource economics division, Economic Research Service, Agricultural Economic Report No. 823. Washington, DC: U.S. Department of Agriculture, 60+iii. Available online at: http://www.ers.usda.gov (accessed January 30, 2021).
Wienhold, B. J., Andrews, S. S., Kuykendall, H., and Karlen, D. L. (2008). Recent advances in soil quality assessment in the United States. J. Ind. Soc. Soil Sci. 56, 1–10. Available online at: https://digitalcommons.unl.edu/usdaarsfacpub/1204
Wiesmeier, M., Poeplau, C., Sierra, C. A., Maier, H., Fruhauf, C., Hubner, R., et al. (2016). Projected loss of soil organic carbon in temperate agricultural soils in the 21st century: effects of climate change and carbon input trends. Sci. Rep. 6:32525. doi: 10.1038/srep32525
Wijitkosum, S. (2020). Reducing vulnerability to desertification by using the spatial measures in a degraded area in Thailand. Land 9:49. doi: 10.3390/land9020049
Williams, A. J., Pagliai, M., and Stoops, G. (2018). “Physical and biological surface crusts and seals,” in Interpretation of Micro-Morphological Features of Soil and Regoliths, 2nd Edn, eds G. Stoops, V. Macrcelino and F. Mees (Amsterdam: Elsevier Publication), 539–574. doi: 10.1016/B978-0-444-63522-8.00019-X
Williams, C. H., and Steinberg, A. (1959). Soil sulphur fractions as chemical indices of available sulphur in some Australian soils. Austr. J. Agri. Res. 10, 340–352. doi: 10.1071/AR9590340
Wischmeier, W. H., and Smith, D. D. (1978). Predicting Rainfall Erosion Losses: Guide to Conservation Planning. USDA, Agriculture Handbook 537. Washington, DC: U.S. Government Printing Office.
Wishmeier, W. H., and Smith, D. D. (1960). “A universal soil-loss equation to guide conservation farm planning,” in Transactions of Seventh International Congress on Soil Science, Vol. 1 (Madison, WI), 418–425.
Woodruff, N. P., and Siddoway, F. H. (1965). A wind erosion equation, soil science society of America Proceedings 29, 602–608. doi: 10.2136/sssaj1965.03615995002900050035x
Yadav, G. S., Das, A., Lal, R., Babu, S., Datta, M., Meena, R. S., et al. (2019). Impact of no tillage and mulching on soil carbon sequestration under rice (Oryza sativa L.) rapeseed (Brassica campestris, L. var. rapeseed) cropping system in hilly agro-ecosystem of the Eastern Himalayas, India. Agri. Ecosyst. Environ. 275, 81–92. doi: 10.1016/j.agee.2019.02.001
Yaduvanshi, N. P. S. (2003). Substitution of inorganic fertilizers by organic manures and the effect on soil fertility in a rice-wheat rotation on reclaimed sodic soil in India. J. Agri. Sci. 140, 161–168. doi: 10.1017/S0021859603002934
Yaduvanshi, N. P. S., Setter, T. L., Sharma, S. K., Singh, N. K., and Kulshreshtha, N. (2010). “Waterlogging effect on wheat yield, redox potential, manganese and iron in different soils of India,” in Soil Solutions for a Changing World, 19th World Congress on Soil Science, 1-6 August, 2010 (Brisbane, QLD), 45–48.
Yan, A., Wang, Y., Tan, S. N., Mohd Yusof, M. L., Ghosh, F., and Chen, Z. (2020). Phytoremediation: a promising approach for revegetation of heavy metal-polluted land. Front. Plant Sci. 11:359. doi: 10.3389/fpls.2020.00359
Yang, L., Bai, J., Liu, J., Zeng, N., and Cao, W. (2018). Green manuring effect on changes of soil nitrogen fractions, maize growth, and nutrient uptake. Agronomy 8:261. doi: 10.3390/agronomy8110261
Yoder, R. E. (1936). A direct method of aggregate analysis of soils and a study of the physical nature of erosion losses. Agron. J. 28, 337–351. doi: 10.2134/agronj1936.00021962002800050001x
Zahir, Z. A., Arshad, M., and Frankenberger, W. T. (2004). Plant growth promoting rhizobactaria: application and perspectives in agriculture. Adv. Agron. 81, 97–167. doi: 10.1016/S0065-2113(03)81003-9
Zhang, W. (2018). Global pesticide use: profile, trend, cost/benefit and more. Proc. Acad. Ecol. Environ. Sci. 8, 1–27.
Zingore, S., Mutegi, J., Agesa, B., Tamene, L., and Kihara, J. (2015). Soil degradation in sub-Saharan Africa and crop production options for rehabilitation. Better Crops 99, 24–26. Available online at: https://hdl.handle.net/10568/68702
Keywords: integrated farming system, novel agronomic approaches, soil degradation, soil health, conservation tillage, soil microbial diversity
Citation: Shahane AA and Shivay YS (2021) Soil Health and Its Improvement Through Novel Agronomic and Innovative Approaches. Front. Agron. 3:680456. doi: 10.3389/fagro.2021.680456
Received: 14 March 2021; Accepted: 27 July 2021;
Published: 06 September 2021.
Edited by:
Rajni Singh, Amity University, IndiaReviewed by:
Naleeni Ramawat, Amity Institute of Organic Agriculture (AIOA), IndiaMahendra Vikram Singh Rajawat, National Bureau of Agriculturally Important Microorganisms (ICAR), India
Copyright © 2021 Shahane and Shivay. This is an open-access article distributed under the terms of the Creative Commons Attribution License (CC BY). The use, distribution or reproduction in other forums is permitted, provided the original author(s) and the copyright owner(s) are credited and that the original publication in this journal is cited, in accordance with accepted academic practice. No use, distribution or reproduction is permitted which does not comply with these terms.
*Correspondence: Yashbir Singh Shivay, ysshivay@hotmail.com