- 1Department of Entomology, Federal University of Lavras (UFLA), Lavras, Brazil
- 2Applied Chemical Ecology Technology, Cornell AgriTech, Department of Entomology, Cornell University, New York, NY, United States
- 3Plant Biostimulants Lab, Agrarian Sciences College of Lavras (ESAL), Department of Agriculture, Federal University of Lavras (UFLA), Lavras, Brazil
- 4Laboratory of Chemical Ecology of Insect-Plant Interactions (LEQIIP), Department of Entomology, Federal University of Lavras (UFLA), Lavras, Brazil
The search for innovative and alternative methods for chemical control to manage pests is an increasingly growing reality. The use of biostimulants such as plant growth promoting rhizobacteria (PGPR) and humic acids (HA) has been shown to improve many agronomic characteristics of plants while increasing yield. These biostimulants also alter the production of secondary metabolites with consequences for insect herbivores. Here we review the role of biostimulants such as PGPR and HA in promoting and eliciting plant defenses. The cascading effects of using these biostimulants on insect herbivores and their natural enemies are discussed in this context. Synergism between biostimulants are also discussed. The potential role of these products in augmenting agricultural productivity is highlighted as is further need for additional research. This review highlights the potential of this tool to enhance integrated pest management in agricultural production systems, reduce the use of pesticides, and increase the efficiency of fertilization while supporting healthier more pest-resistant plants.
1. Introduction
Plants are constantly under threat in the field, either by abiotic stresses or by the attack of diseases and pests, which can directly and often negatively influence their productivity (Al-Juthery et al., 2020; Vasseur-Coronado et al., 2021). Infestation by pests can result in damage ranging from leaf/root area reduction of the shoot/root by herbivory to the spread of pathogens, causing irreversible damage to the crop. However, in order to deal with herbivory and minimize the subsequent impacts caused by pests, plants have developed several adaptations that include constitutive and induced defense mechanisms of a physical and/or chemical nature (Dicke and van Loon, 2000; Moreira et al., 2014, 2018).
Constitutive chemical defenses comprise metabolites that are present in plant tissues without the need for the action of an herbivore for its expression, while induced defenses are activated following herbivory (Arimura et al., 2005). Chemical defenses can affect herbivores directly, through toxic or repellent secondary metabolites or anti-nutritional proteins (e.g., protease inhibitors, lectins) and indirectly by the release of volatile organic compounds (VOCs) attractive or repellant to herbivores and/or attractive to natural enemies (Dicke and van Loon, 2000; Halitschke et al., 2001; Chen, 2008; Rasmann et al., 2017; Aljbory and Chen, 2018). Synthesis of induced chemical defenses is triggered by elicitors derived from an herbivore's oral secretions and damage-associated molecular patterns (DAMPs), and is modulated by changes in levels of the phytohormones salicylic acid (SA), jasmonic acid (JA), and ethylene (ET) (Hatcher et al., 2004; Kaplan et al., 2008; Schmelz et al., 2009; Erb et al., 2012; Lazebnik et al., 2014).
The protection conferred by these induced defenses can be exploited in pest management through application of substances that induce or strengthen plant defenses prior to herbivory. Induction through exogenous application can be accomplished by a range of products ranging from synthetic plant hormone mimics to soil amendments and microbes. Many of these lead to an increase in the resistance of plants to insects through induction of pre-existing mechanisms latent in the plant or defense priming (Stout et al., 2002; Walters and Heil, 2007; Bektas and Eulgem, 2015). Substances that act as priming agents promote defense activation more quickly after an attack which can be advantageous both to plants in terms of a lower energy cost approach to defense and to agricultural managers (Pieterse et al., 2001; Balmer et al., 2015; Conrath et al., 2015; Martinez-Medina et al., 2016). Generalizing response from application of substances designed to stimulate plant defense pathways is difficult, however. Each substance and compound is unique and can not only produce different and specific biochemical properties in the plant, but also result in different behavioral responses on the part of herbivores and natural enemies (Sobhy et al., 2012; Peñaflor and Bento, 2013; Filgueiras et al., 2019).
While some of these effects are relatively well-characterized, especially in terms of elicitors such as synthetic plant hormones that stimulate specific plant defense pathways (e.g., methyl salicylate), the effects of many biostimulants on induced plant defenses are only beginning to be quantified. This is in part due to the vast number of possible products encompassed in the definition of biostimulants (Brown and Saa, 2015; Du Jardin, 2015). Essentially any substance applied to plants with the express interest of enhancing positive effects on growth and productivity could be considered a biostimulant (Du Jardin, 2015; Al-Juthery et al., 2020). These products are obtained naturally from various economically and environmentally viable sources. Currently these products include extracts of seaweed, humic substances (humic acids and fulvic acids), chitin and chitosan derivatives, amino acids, protein hydrolysates, and microbes (Al-Juthery et al., 2020). Some have been showing promise for commercial agriculture, not only for enhancing plant growth above- and below-ground and/or crop productivity as it has been reported currently (Grover et al., 2020; Jiménez-Arias et al., 2021; Sakr et al., 2021), but also more specifically in enhancing plant defense against pests and pathogens (Brown and Saa, 2015).
Two of the more promising biostimulants used in this regard are microbial inoculants in the form of Plant Growth Promoting Rhizobacteria (PGPR) and the organic fertilizer Humic Acid (HA) (Figure 1). Each has an established history of efficacy from the standpoint of enhancing plant growth and each has produced mounting evidence in recent years that point to roles in stimulating plant defenses. While this is an emerging area and research is in its infancy, there is evidence of synergism between these biostimulants and clear paths forward in terms of expanding commercial use with benefits for large scale agriculture. Here we review the role and point out the potential of PGPR and HA in increasing plant resistance against insect herbivores.
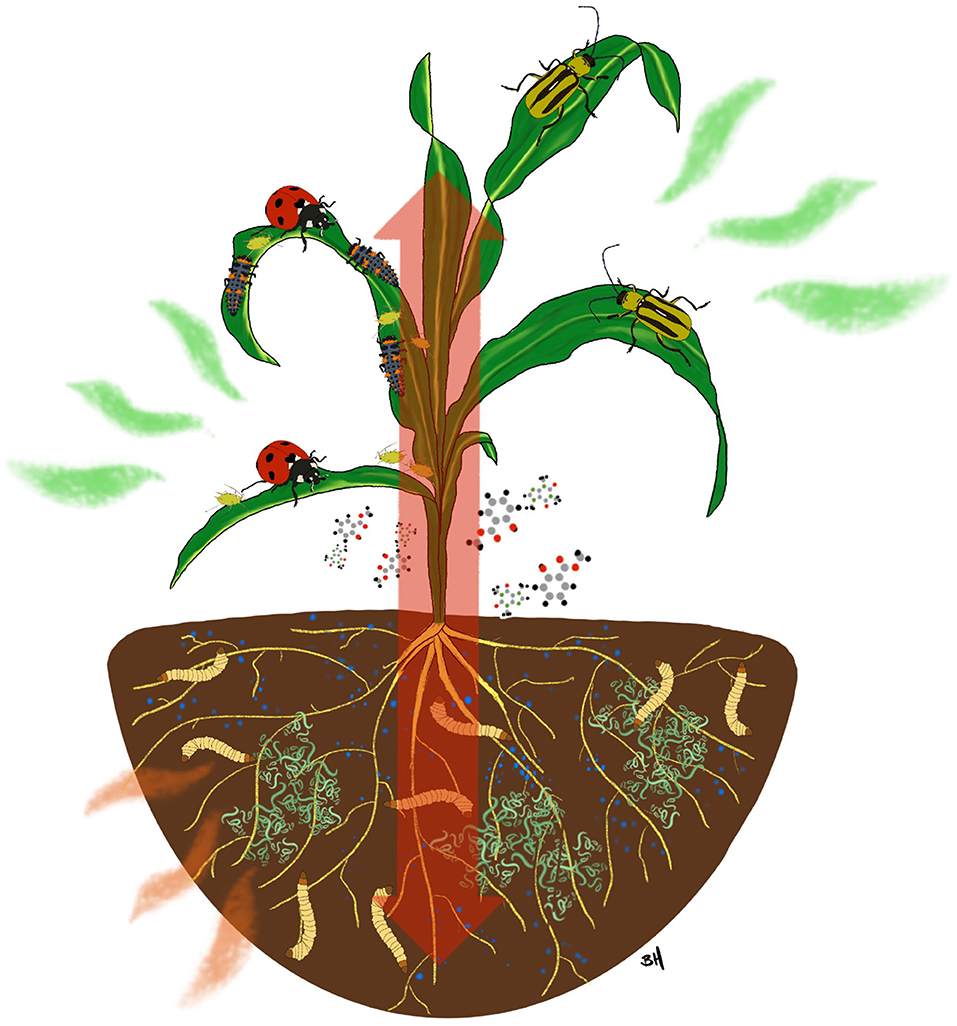
Figure 1. Additions of biostimulants in the form of Plant Growth Promoting Bacteria and Humic Acids can have consequences for a range of other organisms including insect herbivores and insect natural enemies. Red arrow is meant to symbolize the systemic nature of many intra-plant communication systems that connect aboveground and belowground inputs.
2. Plant Growth Promoting Rhizobacteria (PGPR)
Plant growth promoting rhizobacteria (PGPR) are a common form of microbial inoculant already used as biofertilizers in agriculture for facilitating and improving nutrient absorption by plants while influencing plant morphogenesis and development (Bhattacharyya and Jha, 2012). PGPR has a long and storied history with the use of legumes to increase fertility documented before the common era (Bhattacharyya and Jha, 2012). These rhizobacteria are mostly gram-negative bacteria that can form associations with plant roots and increase plant growth through nitrogen fixation, phosphate solubilization, production of phytosiderophores, and modulation of phytohormones (Adesemoye et al., 2009). These changes in phytohormones influence plant responses to biotic and abiotic stress because they modulate defense signaling pathways that culminate in the production of secondary metabolites as well as activate defense priming (Pieterse et al., 2001, 2014; Bhattacharyya and Jha, 2012; Bulgarelli et al., 2013; Backer et al., 2018). The enhanced ability to defend itself in PGPR-colonized plants is often given by the activation of induced systemic resistance (ISR), but in some cases also by systemic acquired resistance (SAR) (Bhattacharyya and Jha, 2012; Annapurna et al., 2013; Pieterse et al., 2014; Conrath et al., 2015; Mhatre et al., 2019). Initially, both defense-priming processes were thought to act against only pathogens, however, they also play a role in enhancing plant defensive response against insect herbivores (Zamioudis and Pieterse, 2012; Conrath et al., 2015).
It has been well-documented that colonization by PGPRs can confer resistance to insect pests that feed on shoots or roots (Rasmann et al., 2017). These effects range from repellence and weight reduction effects on larvae (Disi et al., 2019), to an increase in nymph mortality (Dorta et al., 2020). Colonization by PGPRs can also reduce the feeding period and reproductive rate of some insects (Rashid et al., 2017). Other studies to a lesser extent have also documented that, depending on the system, PGPR colonization has either a neutral (Boutard-Hunt et al., 2009; Brock et al., 2018) or a positive effect on the insect herbivore population (Kempel et al., 2009; Table 1A). In addition, the effects of PGPR on plant-herbivore interactions can vary according to the level of specialization as well as the feeding habit of the herbivore (Van Oosten et al., 2008; Pineda et al., 2010). Generalist herbivores tend to suffer a greater impact than specialists. Experiments, with Arabidopsis thaliana plants inoculated with Pseudomonas fluorescens negatively affected the behavior of the generalist herbivore (Spodoptera exigua), but did not affect the specialist (Pieris rapae) (Van Oosten et al., 2008).
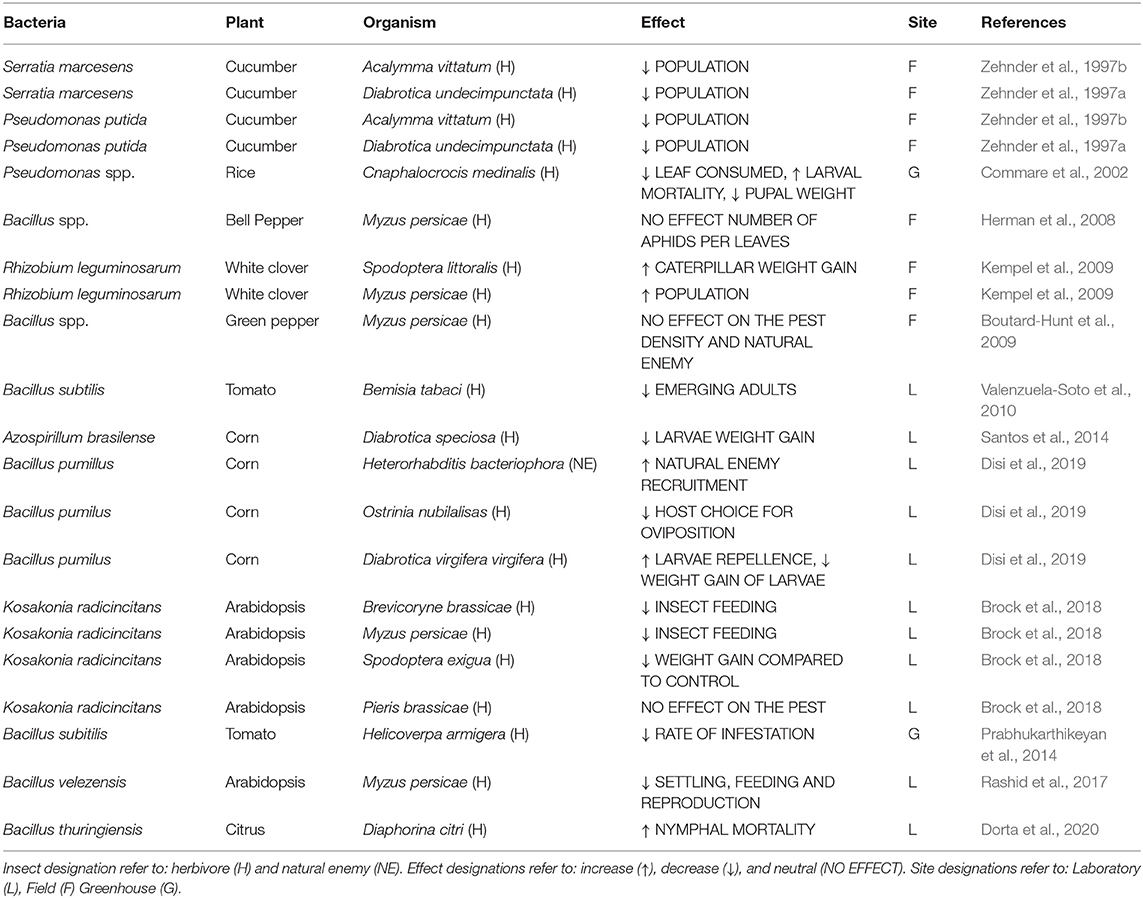
Table 1A. Examples of wide use of beneficial bacteria in different cultures affecting the behavioral response of insects and other natural enemies of different orders.
Other PGPR biostimulants have been well-studied in plants and have been shown to be responsible for modifying plant defenses and the profile of plant secondary metabolites increasing levels of hydroxycinnamates, benzoates, flavonoids, glycoalkaloids, phenols, stilbenoids, coumarins, lignins, monoterpenes, and sesquiterpenes (Tomczyk, 2002; Salomon et al., 2014; Mhlongo et al., 2020; Jeon et al., 2021). Applications of PGPR can also induce systemic resistance in Arabidopsis plants by increasing the levels of glucosinolates and camalexin with negative impacts on herbivore performance (Pangesti et al., 2015). This phenomenon is not limited to Arabidopsis, however. PGPR can induce systemic resistance across plant families; in cotton (Gossypium hirsutum), for example, induced plants show higher levels of defense compounds such as gossypol that contribute to an increase in the plant's resistance to pest attack (Zebelo et al., 2016).
PGPR can also increase plant resistance via recruitment of natural enemies in both above- and belowground environments. At the belowground level, for example, association with PGPR can change the emission of root volatiles in ways that increase the recruitment of entomopathogenic nematodes, which are important biological control agents of soil pests (Santos et al., 2014). At the aboveground level, PGPR can increase the diversity and abundance of predators and optimize host searching by parasitoids as host-infested plants emit a more attractive blend of herbivore-induced plant volatiles (HIPVs) (Pangesti et al., 2015).
3. Humic Substances and Humic Acids
Humic acids (HAs) are a subset of a broader category of humic substances (HSs) and comprise a biologically active fraction characterized by being soluble in alkaline media and insoluble in acidic media (Garćıa et al., 2016). In addition to being able to modify the physical, chemical and biological properties of the soil, these compounds can alter plant hormonal metabolism resulting in the acceleration of plant growth and development, root elongation, increase in germination rates, mitigation of osmotic and heavy metal stress, and increase in biomass (Trevisan et al., 2011; Baldotto and Baldotto, 2014; Canellas et al., 2015; Nardi et al., 2017; Shah et al., 2018; Wong et al., 2020). Furthermore, they can also modify the metabolic profile of plant defenses, which promotes greater resistance against insects (Schiavon et al., 2010; Razmjou et al., 2011).
Similar to PGPRs, HAs have a storied history of use by humans. Vermicompost containing humic acids have been used extensively in small-scale agriculture. Soil amendment with vermicompost makes plants not only more vigorous, but also resistant to insect pests (Cardoza, 2011; Cardoza and Buhler, 2012; Sedaghatbaf et al., 2018). Despite the early evidence of vermicompost in enhancing plant resistance, just recently the role of HA has been investigated. The few studies available concern only aboveground insect pests and show that amendment with HA impacts development, survival, and fecundity of lepidopteran and aphid pests, resulting in reduced population growth (Mohamadi et al., 2017a,b; Nasab et al., 2019; Jafary-Jahed et al., 2020; Table 1B).
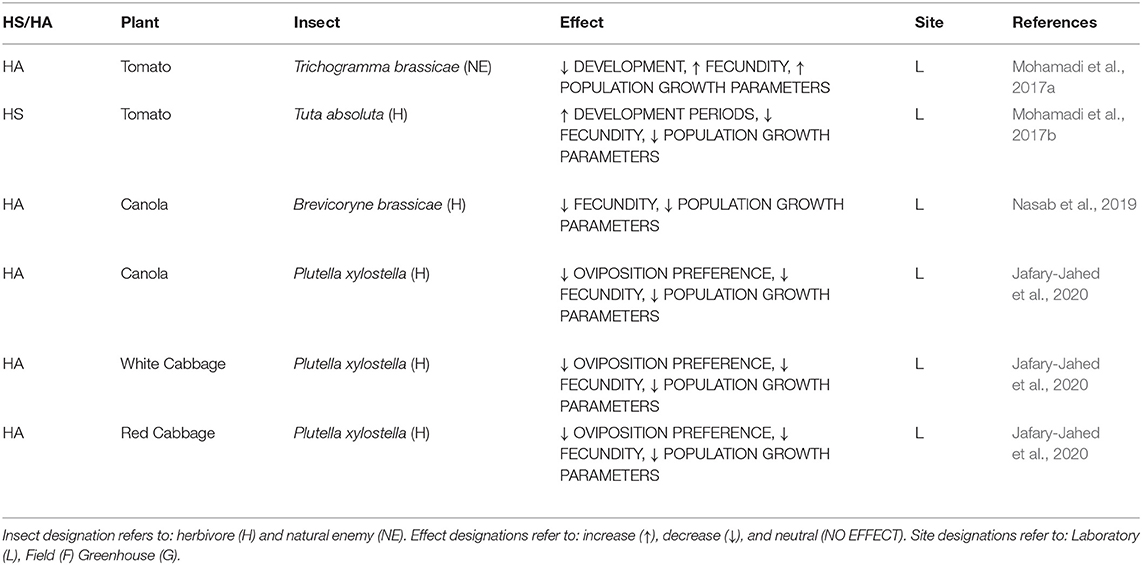
Table 1B. Examples of wide use of humic substances (HS) and humic acid (HA) affecting the behavioral response of insects and other natural enemies of different orders.
Little is known about the HA-induced changes in plant phenotype that affect insect herbivore performance nor the underlying mechanisms responsible for the increased resistance to insect pests. For example, the lower performance of the aphid Brevicoryne brassicae in HA-treated canola was not associated with the level of glucosinolates, phenols or flavonoids, secondary plant metabolites with putative defensive functions against insects (Nasab et al., 2019). Although not in the context of insect-plant interactions, it is well documented that HA or HS amendment increases the level of metabolites linked to the shikimic acid pathway, such as flavonoids and benzoic acids, because of the greater activity of the phenylalanine amnonia lyase and induction of the primary metabolism (Schiavon et al., 2010; Olivares et al., 2017; Aguiar et al., 2018). Despite the relevant role of phenolics in plant resistance against insect pests, the few suggestions in the literature to the protective function of HA against biotic stress only discuss phytopathogens (Aguiar et al., 2018; Jindo et al., 2020), while the potential to increase crop resistance to pests has been overlooked.
Recent evidence that HA (or HS in general in some cases) influences phytohormone signaling networks, transcription level of phytohormone-sensitive genes and transcription factors indicate their potential as a priming agent of anti-herbivore defenses. The role of HA as a priming agent has been highlighted as alleviating abiotic stress through regulation of several genes sensitive to jasmonates (jasmonic acid: JA), ethylene (ET) and salicylic acid (SA), which are important phytohormones in modulating herbivore-induced plant defenses (Canellas et al., 2020). More recent promising results have shown that either foliar or root HS treatment induces augmented levels of JA and JA-Isoleucine (the active form) in roots after 72 h (De Hita et al., 2020). Interestingly, their results coincide with the temporal dynamic of early alterations in abscisic acid (ABA) and indole-3-acetic acid (IAA) followed by JA, in similar fashion to the modulation of herbivore-induced plant defenses (Erb et al., 2012; Vos et al., 2013; Machado et al., 2016).
Another important aspect of signaling and modulating plant defense response to herbivores is the balance of oxidative metabolism (Erb and Reymond, 2019). Herbivory elicits the production of reactive oxygen species (ROS), which despite being important early signals for activating plant defenses, cause oxidative injury in cells and can interact antagonistically with the production of JA in ways that affect negatively plant resistance to some insects (Miller et al., 2009; Block et al., 2018). Treatment with HA often elevates the activity of antioxidative enzymes (e.g., superoxide dismutase, catalase, and peroxidase), which are important in ROS scavenging under stress conditions (Pizzeghello et al., 2001). The enhanced capacity in preventing oxidative injury in HA-treated plants might also alleviate the potential negative effects on herbivore-induced plant defenses (Aguiar et al., 2016; Hatami et al., 2018). However, further investigation is necessary to determine whether the greater antioxidative enzyme activity observed in HA-treated plants affects the modulation of induced plant defense against herbivores.
Information on the effect of HA or HS amendment on the third trophic level is scarce. Mohamadi et al. (2017a) found that, even though the herbivore performance is poorer in HA-treated plants, they are better hosts to egg parasitoids relative to those fed on untreated plants. The egg parasitoids develop faster and have higher fecundity when multiplied in hosts fed on HA-treated plants. This finding is in line with the positive effects on the biology of predators fed on prey reared in vermicompost-treated plants (Mottaghinia et al., 2016; Alizamani et al., 2017). Nevertheless, the mechanism by which HA or vermicompost amendment influences the biology of the third trophic level via host/prey remains unknown. Moreover, future research is needed to address whether HA amendment changes the emission of constitutive and herbivore-induced plant volatiles with consequences for the recruitment of the third trophic level. Given the established influence of HA on the shikimic acid pathway, as mentioned before, and the ecological role of shikimic-acid derived volatiles to natural enemies (D'Alessandro et al., 2006; Ye et al., 2018), this question deserves attention as HA amendment likely interferes in the attraction of biological control agents. For example, if HA amendment elicits in intact plants the emission of attractive volatiles to natural enemies, it might have unintended consequences to pest biological control because of the enhanced attractiveness of plants with no host/prey (reward).
4. Interactions
Interference in pest biological control is a concern especially when multiple biostimulants are used in conjunction with each other. Plant defense pathways are known to exhibit cross-talk where activation of one pathway can have antagonistic effects on other pathways (Checker et al., 2018). Similar questions are eminently applicable to the combined use of different forms of biostimulants both for enhancing plant growth and for augmenting pest control: Can biostimulants be synergistic?
The short answer is yes. While studies are still somewhat scarce and synergistic effects are more often reported in terms of plant growth, some work has shown that the combined use of two biostimulants can have a synergistic effect on plants, making them more resistant than when treated with only a single biostimulant (Aguiar et al., 2018). Humic acid application can stimulate the production of root hair in the root system as well as the formation of adventitious roots (Olivares et al., 2017). This effect provides an increase of microorganisms adherence to these structures, making the inoculation potential for rhizobacteria greater and more efficient, since it is in this region that bacteria colonize and associate with plants (Olivares et al., 2017). Interestingly, synergism between biostimulants does not necessarily imply positive independent effects. In tomatoes and spinach, treatments of PGPR alone had no effect, while treatments of PGPR in conjunction with vermicompost containing humic substances had positive effects on yield, plant nutritional quality, and markers of soil quality (Song et al., 2015). Synergistic effects of combined biostimulant application are beginning to be validated across multiple systems and research groups have been able to reproduce results in the same crop types (Song et al., 2015; Rouphael and Colla, 2018).
Antagonist effects of combined biostimulant application are not unreported anecdotally and should be considered in terms of effects on a plant's ability to resist insect pests. While many of the synergistic effects described above may be excellent for plant growth, more nutritive tissue could also be highly attractive to pests, especially if such tissue is not defended. Studies on the effects of combined application of biostimulants on herbivores are limited and deserve further attention as efforts are made to implement use of these substances at larger agricultural scales.
5. Conclusions
Despite the nascency of understanding mechanistically the effects of biostimulant application in isolation and in combination, biostimulants continue to be used in agriculture. The means by which use of these substances can be optimized for enhanced agricultural productivity is of primary importance for continued development of their potential. With a multitude of effects—many that could potentially be synergistic—the use of PGPRs and HAs (or HSs) holds the promise to not only increase production of nutritive plants but also do so in such a manner that the plants themselves are well defended and require less use of pesticides. In implementing such techniques and fostering further research, holistic approaches to cascading effects of application resulting from induced plant defenses will go a long way.
Author Contributions
RP, CF, JD, MP, and DW each contributed to the research and writing of this manuscript. All authors contributed to the article and approved the submitted version.
Funding
CAPES (Coordenação de Aperfeiçoamento de Pessoal de Nível Superior) and CNPq (438949/2018-8) provided support for work on this project.
Conflict of Interest
The authors declare that the research was conducted in the absence of any commercial or financial relationships that could be construed as a potential conflict of interest.
References
Adesemoye, A., Torbert, H., and Kloepper, J. (2009). Plant growth-promoting rhizobacteria allow reduced application rates of chemical fertilizers. Microb. Ecol. 58, 921–929. doi: 10.1007/s00248-009-9531-y
Aguiar, N., Medici, L., Olivares, F., Dobbss, L., Torres-Netto, A., Silva, S., et al. (2016). Metabolic profile and antioxidant responses during drought stress recovery in sugarcane treated with humic acids and endophytic diazotrophic bacteria. Ann. Appl. Biol. 168, 203–213. doi: 10.1111/aab.12256
Aguiar, N. O., Olivares, F. L., Novotny, E. H., and Canellas, L. P. (2018). Changes in metabolic profiling of sugarcane leaves induced by endophytic diazotrophic bacteria and humic acids. PeerJ 6:e5445. doi: 10.7717/peerj.5445
Alizamani, T., Razmjou, J., Naseri, B., Hassanpour, M., Asadi, A., and Kerr, C. (2017). Effect of vermicompost on life history of hippodamia variegata preying on aphis gossypii glover. J. Entomol. Res. Soc. 19, 51–60.
Aljbory, Z., and Chen, M.-S. (2018). Indirect plant defense against insect herbivores: a review. Insect Sci. 25, 2–23. doi: 10.1111/1744-7917.12436
Al-Juthery, H. W., Drebee, H. A., Al-Khafaji, B. M., and Hadi, R. F. (2020). “Plant biostimulants, seaweeds extract as a model (article review),” in IOP Conference Series: Earth and Environmental Science, Vol. 553 (Al-Qadisiyah: IOP Publishing), 012015. doi: 10.1088/1755-1315/553/1/012015
Annapurna, K., Amod Kumar, L., Vithal Kumar, V., Govindasamy, P. B., and Ramadoss, D. (2013). “PGPR-induced systemic resistance (ISR) in plant disease management,” in Bacteria in Agrobiology: Disease Management (Berlin; Heidelberg: Springer), 405–425.
Arimura, G.-i., Kost, C., and Boland, W. (2005). Herbivore-induced, indirect plant defences. Biochim. Biophys. Acta 1734, 91–111. doi: 10.1016/j.bbalip.2005.03.001
Backer, R., Rokem, J. S., Ilangumaran, G., Lamont, J., Praslickova, D., Ricci, E., et al. (2018). Plant growth-promoting rhizobacteria: context, mechanisms of action, and roadmap to commercialization of biostimulants for sustainable agriculture. Front. Plant Sci. 9:1473. doi: 10.3389/fpls.2018.01473
Baldotto, M. A., and Baldotto, L. E. B. (2014). Ácidos húmicos. Rev. Ceres 61, 856–881. doi: 10.1590/0034-737x201461000011
Balmer, A., Pastor, V., Gamir, J., Flors, V., and Mauch-Mani, B. (2015). The “prime-ome:” towards a holistic approach to priming. Trends Plant Sci. 20, 443–452. doi: 10.1016/j.tplants.2015.04.002
Bektas, Y., and Eulgem, T. (2015). Synthetic plant defense elicitors. Front. Plant Sci. 5:804. doi: 10.3389/fpls.2014.00804
Bhattacharyya, P. N., and Jha, D. K. (2012). Plant growth-promoting rhizobacteria (PGPR): emergence in agriculture. World J. Microbiol. Biotechnol. 28, 1327–1350. doi: 10.1007/s11274-011-0979-9
Block, A., Christensen, S. A., Hunter, C. T., and Alborn, H. T. (2018). Herbivore-derived fatty-acid amides elicit reactive oxygen species burst in plants. J. Exp. Bot. 69, 1235–1245. doi: 10.1093/jxb/erx449
Boutard-Hunt, C., Smart, C. D., Thaler, J., and Nault, B. A. (2009). Impact of plant growth-promoting rhizobacteria and natural enemies on Myzus persicae (hemiptera: Aphididae) infestations in pepper. J. Econ. Entomol. 102, 2183–2191. doi: 10.1603/029.102.0622
Brock, A. K., Berger, B., Schreiner, M., Ruppel, S., and Mewis, I. (2018). Plant growth-promoting bacteria kosakonia radicincitans mediate anti-herbivore defense in Arabidopsis thaliana. Planta 248, 1383–1392. doi: 10.1007/s00425-018-2964-0
Brown, P., and Saa, S. (2015). Biostimulants in agriculture. Front. Plant Sci. 6:671. doi: 10.3389/fpls.2015.00671
Bulgarelli, D., Schlaeppi, K., Spaepen, S., Van Themaat, E. V. L., and Schulze-Lefert, P. (2013). Structure and functions of the bacterial microbiota of plants. Annu. Rev. Plant Biol. 64, 807–838. doi: 10.1146/annurev-arplant-050312-120106
Canellas, L. P., Canellas, N. O., Irineu, L. E. S. d. S., Olivares, F. L., and Piccolo, A. (2020). Plant chemical priming by humic acids. Chem. Biol. Technol. Agric. 7, 1–17. doi: 10.1186/s40538-020-00178-4
Canellas, L. P., Olivares, F. L., Aguiar, N. O., Jones, D. L., Nebbioso, A., Mazzei, P., and Piccolo, A. (2015). Humic and fulvic acids as biostimulants in horticulture. Sci. Hortic. 196, 15–27. doi: 10.1016/j.scienta.2015.09.013
Cardoza, Y. J. (2011). Arabidopsis thaliana resistance to insects, mediated by an earthworm-produced organic soil amendment. Pest Manage. Sci. 67, 233–238. doi: 10.1002/ps.2059
Cardoza, Y. J., and Buhler, W. G. (2012). Soil organic amendment impacts on corn resistance to helicoverpa zea: constitutive or induced? Pedobiologia 55, 343–347. doi: 10.1016/j.pedobi.2012.08.002
Checker, V. G., Kushwaha, H. R., Kumari, P., and Yadav, S. (2018). “Role of phytohormones in plant defense: signaling and cross talk,” in Molecular Aspects of Plant-Pathogen Interaction (Singapore: Springer), 159–184.
Chen, M.-S. (2008). Inducible direct plant defense against insect herbivores: a review. Insect Sci. 15, 101–114. doi: 10.1111/j.1744-7917.2008.00190.x
Commare, R. R., Nandakumar, R., Kandan, A., Suresh, S., Bharathi, M., Raguchander, T., et al. (2002). Pseudomonas fluorescens based bio-formulation for the management of sheath blight disease and leaffolder insect in rice. Crop Protect. 21, 671–677. doi: 10.1016/S0261-2194(02)00020-0
Conrath, U., Beckers, G. J., Langenbach, C. J., and Jaskiewicz, M. R. (2015). Priming for enhanced defense. Annu. Rev. Phytopathol. 53, 97–119. doi: 10.1146/annurev-phyto-080614-120132
D'Alessandro, M., Held, M., Triponez, Y., and Turlings, T. C. (2006). The role of indole and other shikimic acid derived maize volatiles in the attraction of two parasitic wasps. J. Chem. Ecol. 32, 2733–2748. doi: 10.1007/s10886-006-9196-7
De Hita, D., Fuentes, M., Fernández, V., Zamarre no, A. M., Olaetxea, M., and García-Mina, J. M. (2020). Discriminating the short-term action of root and foliar application of humic acids on plant growth: emerging role of jasmonic acid. Front. Plant Sci. 11:493. doi: 10.3389/fpls.2020.00493
Dicke, M., and van Loon, J. J. (2000). Multitrophic effects of herbivore-induced plant volatiles in an evolutionary context. Entomol. Exp. Appl. 97, 237–249. doi: 10.1046/j.1570-7458.2000.00736.x
Disi, J. O., Mohammad, H. K., Lawrence, K., Kloepper, J., and Fadamiro, H. (2019). A soil bacterium can shape belowground interactions between maize, herbivores and entomopathogenic nematodes. Plant Soil 437, 83–92. doi: 10.1007/s11104-019-03957-7
Dorta, S. d. O., Balbinotte, J., Monnerat, R., Lopes, J. R. S., da Cunha, T., Zanardi, O. Z., et al. (2020). Selection of Bacillus thuringiensis strains in citrus and their pathogenicity to Diaphorina citri (hemiptera: Liviidae) nymphs. Insect Sci. 27, 519–530. doi: 10.1111/1744-7917.12654
Du Jardin, P. (2015). Plant biostimulants: definition, concept, main categories and regulation. Sci. Hortic. 196, 3–14. doi: 10.1016/j.scienta.2015.09.021
Erb, M., Meldau, S., and Howe, G. A. (2012). Role of phytohormones in insect-specific plant reactions. Trends Plant Sci. 17, 250–259. doi: 10.1016/j.tplants.2012.01.003
Erb, M., and Reymond, P. (2019). Molecular interactions between plants and insect herbivores. Annu. Rev. Plant Biol. 70, 527–557. doi: 10.1146/annurev-arplant-050718-095910
Filgueiras, C. C., Martins, A. D., Pereira, R. V., and Willett, D. S. (2019). The ecology of salicylic acid signaling: primary, secondary and tertiary effects with applications in agriculture. Int. J. Mol. Sci. 20:5851. doi: 10.3390/ijms20235851
Garía, A. C., De Souza, L. G. A., Pereira, M. G., Castro, R. N., García-Mina, J. M., Zonta, E., et al. (2016). Structure-property-function relationship in humic substances to explain the biological activity in plants. Sci. Rep. 6, 1–10. doi: 10.1038/srep20798
Grover, M., Bodhankar, S., Sharma, A., Sharma, P., Singh, J., and Nain, L. (2020). PGPR mediated alterations in root traits: way towards sustainable crop production. Front. Sustain. Food Syst. 4:287. doi: 10.3389/fsufs.2020.618230
Halitschke, R., Schittko, U., Pohnert, G., Boland, W., and Baldwin, I. T. (2001). Molecular interactions between the specialist herbivoremanduca sexta (lepidoptera, sphingidae) and its natural host nicotiana attenuata. III. Fatty acid-amino acid conjugates in herbivore oral secretions are necessary and sufficient for herbivore-specific plant responses. Plant Physiol. 125, 711–717. doi: 10.1104/pp.125.2.711
Hatami, E., Shokouhian, A. A., Ghanbari, A. R., and Naseri, L. A. (2018). Alleviating salt stress in almond rootstocks using of humic acid. Sci. Hortic. 237, 296–302. doi: 10.1016/j.scienta.2018.03.034
Hatcher, P. E., Moore, J., Taylor, J. E., Tinney, G. W., and Paul, N. D. (2004). Phytohormones and plant-herbivore-pathogen interactions: integrating the molecular with the ecological. Ecology 85, 59–69. doi: 10.1890/02-0724
Herman, M., Nault, B., and Smart, C. (2008). Effects of plant growth-promoting rhizobacteria on bell pepper production and green peach aphid infestations in New York. Crop Protect. 27, 996–1002. doi: 10.1016/j.cropro.2007.12.004
Jafary-Jahed, M., Razmjou, J., Nouri-Ganbalani, G., Naseri, B., and Hassanpour, M. (2020). Bottom-up effects of organic fertilizers on Plutella xylostella (l) with selected cruciferous crop plants. J. Lepidopterists' Soc. 74, 7–17. doi: 10.18473/lepi.74i1.a2
Jeon, J.-S., Carreno-Quintero, N., van Eekelen, H. D., De Vos, R. C., Raaijmakers, J. M., and Etalo, D. W. (2021). Impact of root-associated strains of three paraburkholderia species on primary and secondary metabolism of Brassica oleracea. Sci. Rep. 11, 1–14. doi: 10.1038/s41598-021-82238-9
Jiménez-Arias, D., García-Machado, F. J., Morales-Sierra, S., García-García, A. L., Herrera, A. J., Valdés, F., et al. (2021). A beginner's guide to osmoprotection by biostimulants. Plants 10:363. doi: 10.3390/plants10020363
Jindo, K., Olivares, F. L., da Paix ao Malcher, D. J., Sánchez-Monedero, M. A., Kempenaar, C., and Canellas, L. P. (2020). From lab to field: role of humic substances under open-field and greenhouse conditions as biostimulant and biocontrol agent. Front. Plant Sci. 11:426. doi: 10.3389/fpls.2020.00426
Kaplan, I., Halitschke, R., Kessler, A., Sardanelli, S., and Denno, R. F. (2008). Constitutive and induced defenses to herbivory in above-and belowground plant tissues. Ecology 89, 392–406. doi: 10.1890/07-0471.1
Kempel, A., Brandl, R., and Schädler, M. (2009). Symbiotic soil microorganisms as players in aboveground plant-herbivore interactions-the role of rhizobia. Oikos 118, 634–640. doi: 10.1111/j.1600-0706.2009.17418.x
Lazebnik, J., Frago, E., Dicke, M., and van Loon, J. J. (2014). Phytohormone mediation of interactions between herbivores and plant pathogens. J. Chem. Ecol. 40, 730–741. doi: 10.1007/s10886-014-0480-7
Machado, R. A., Robert, C. A., Arce, C. C., Ferrieri, A. P., Xu, S., Jimenez-Aleman, G. H., et al. (2016). Auxin is rapidly induced by herbivore attack and regulates a subset of systemic, jasmonate-dependent defenses. Plant Physiol. 172, 521–532. doi: 10.1104/pp.16.00940
Martinez-Medina, A., Flors, V., Heil, M., Mauch-Mani, B., Pieterse, C. M., Pozo, M. J., et al. (2016). Recognizing plant defense priming. Trends Plant Sci. 21, 818–822. doi: 10.1016/j.tplants.2016.07.009
Mhatre, P. H., Karthik, C., Kadirvelu, K., Divya, K., Venkatasalam, E., Srinivasan, S., et al. (2019). Plant growth promoting rhizobacteria (PGPR): a potential alternative tool for nematodes bio-control. Biocatal. Agric. Biotechnol. 17, 119–128. doi: 10.1016/j.bcab.2018.11.009
Mhlongo, M. I., Piater, L. A., Steenkamp, P. A., Labuschagne, N., and Dubery, I. A. (2020). Metabolic profiling of PGPR-treated tomato plants reveal priming-related adaptations of secondary metabolites and aromatic amino acids. Metabolites 10:210. doi: 10.3390/metabo10050210
Miller, G., Schlauch, K., Tam, R., Cortes, D., Torres, M. A., Shulaev, V., et al. (2009). The plant NADPH oxidase RBOHD mediates rapid systemic signaling in response to diverse stimuli. Sci. Signal. 2:ra45. doi: 10.1126/scisignal.2000448
Mohamadi, P., Razmjou, J., Naseri, B., and Hassanpour, M. (2017a). Humic fertilizer and vermicompost applied to the soil can positively affect population growth parameters of Trichogramma brassicae (hymenoptera: Trichogrammatidae) on eggs of Tuta absoluta (lepidoptera: Gelechiidae). Neotrop. Entomol. 46, 678–684. doi: 10.1007/s13744-017-0536-9
Mohamadi, P., Razmjou, J., Naseri, B., and Hassanpour, M. (2017b). Population growth parameters of Tuta absoluta (lepidoptera: Gelechiidae) on tomato plant using organic substrate and biofertilizers. J. Insect Sci. 17:36. doi: 10.1093/jisesa/iex011
Moreira, X., Abdala-Roberts, L., Gols, R., and Francisco, M. (2018). Plant domestication decreases both constitutive and induced chemical defences by direct selection against defensive traits. Sci. Rep. 8, 1–11. doi: 10.1038/s41598-018-31041-0
Moreira, X., Mooney, K. A., Rasmann, S., Petry, W. K., Carrillo-Gavilán, A., Zas, R., et al. (2014). Trade-offs between constitutive and induced defences drive geographical and climatic clines in pine chemical defences. Ecol. Lett. 17, 537–546. doi: 10.1111/ele.12253
Mottaghinia, L., Hassanpour, M., Razmjou, J., Hosseini, M., and Chamani, E. (2016). Functional response of aphidoletes aphidimyza rondani (diptera: Cecidomyiidae) to aphis Gossypii glover (hemiptera: Aphididae): effects of vermicompost and host plant cultivar. Neotrop. Entomol. 45, 88–95. doi: 10.1007/s13744-015-0343-0
Nardi, S., Ertani, A., and Francioso, O. (2017). Soil-root cross-talking: the role of humic substances. J. Plant Nutr. Soil Sci. 180, 5–13. doi: 10.1002/jpln.201600348
Nasab, R. S., Yali, M. P., and Bozorg-Amirkalaee, M. (2019). Effects of humic acid and plant growth-promoting rhizobacteria (PGPR) on induced resistance of canola to Brevicoryne brassicae l. Bull. Entomol. Res. 109, 479–489. doi: 10.1017/S0007485318000779
Olivares, F. L., Busato, J. G., de Paula, A. M., da Silva Lima, L., Aguiar, N. O., and Canellas, L. P. (2017). Plant growth promoting bacteria and humic substances: crop promotion and mechanisms of action. Chem. Biol. Technol. Agric. 4, 1–13. doi: 10.1186/s40538-017-0112-x
Pangesti, N., Weldegergis, B. T., Langendorf, B., van Loon, J. J., Dicke, M., and Pineda, A. (2015). Rhizobacterial colonization of roots modulates plant volatile emission and enhances the attraction of a parasitoid wasp to host-infested plants. Oecologia 178, 1169–1180. doi: 10.1007/s00442-015-3277-7
Pe naflor, M., and Bento, J. (2013). Herbivore-induced plant volatiles to enhance biological control in agriculture. Neotrop. Entomol. 42, 331–343. doi: 10.1007/s13744-013-0147-z
Pieterse, C. M., Van Pelt, J. A., Van Wees, S. C., Ton, J., Léon-Kloosterziel, K. M., Keurentjes, J. J., et al. (2001). Rhizobacteria-mediated induced systemic resistance: triggering, signalling and expression. Eur. J. Plant Pathol. 107, 51–61. doi: 10.1023/A:1008747926678
Pieterse, C. M., Zamioudis, C., Berendsen, R. L., Weller, D. M., Van Wees, S. C., and Bakker, P. A. (2014). Induced systemic resistance by beneficial microbes. Annu. Rev. phytopathol. 52, 347–375. doi: 10.1146/annurev-phyto-082712-102340
Pineda, A., Zheng, S.-J., van Loon, J. J., Pieterse, C. M., and Dicke, M. (2010). Helping plants to deal with insects: the role of beneficial soil-borne microbes. Trends Plant Sci. 15, 507–514. doi: 10.1016/j.tplants.2010.05.007
Pizzeghello, D., Nicolini, G., and Nardi, S. (2001). Hormone-like activity of humic substances in fagus sylvaticae forests. New Phytol. 151, 647–657. doi: 10.1046/j.0028-646x.2001.00223.x
Prabhukarthikeyan, R., Saravanakumar, D., and Raguchander, T. (2014). Combination of endophytic bacillus and beauveria for the management of fusarium wilt and fruit borer in tomato. Pest Manage. Sci. 70, 1742–1750. doi: 10.1002/ps.3719
Rashid, M., Khan, A., Hossain, M. T., and Chung, Y. R. (2017). Induction of systemic resistance against aphids by endophytic Bacillus velezensis YC7010 via expressing phytoalexin deficient4 in arabidopsis. Front. Plant Sci. 8:211. doi: 10.3389/fpls.2017.00211
Rasmann, S., Bennett, A., Biere, A., Karley, A., and Guerrieri, E. (2017). Root symbionts: powerful drivers of plant above-and belowground indirect defenses. Insect Sci. 24, 947–960. doi: 10.1111/1744-7917.12464
Razmjou, J., Mohammadi, M., and Hassanpour, M. (2011). Effect of vermicompost and cucumber cultivar on population growth attributes of the melon aphid (hemiptera: Aphididae). J. Econ. Entomol. 104, 1379–1383. doi: 10.1603/EC10120
Rouphael, Y., and Colla, G. (2018). Synergistic biostimulatory action: designing the next generation of plant biostimulants for sustainable agriculture. Front. Plant Sci. 9:1655. doi: 10.3389/fpls.2018.01655
Sakr, M. T., Ibrahim, H. M., ElAwady, A. E., and Abo El Makarm, A. A. (2021). Growth, yield and biochemical constituents as well as post-harvest quality of water-stressed broccoli (Brassica oleraceae l. var. italica) as affected by certain biomodulators. Sci. Hortic. 275:109605. doi: 10.1016/j.scienta.2020.109605
Salomon, M. V., Bottini, R., de Souza Filho, G. A., Cohen, A. C., Moreno, D., Gil, M., and Piccoli, P. (2014). Bacteria isolated from roots and rhizosphere of Vitis vinifera retard water losses, induce abscisic acid accumulation and synthesis of defense-related terpenes in in vitro cultured grapevine. Physiol. Plant. 151, 359–374. doi: 10.1111/ppl.12117
Santos, F., Pe naflor, M. F. G., Paré, P. W., Sanches, P. A., Kamiya, A. C., Tonelli, M., et al. (2014). A novel interaction between plant-beneficial rhizobacteria and roots: colonization induces corn resistance against the root herbivore diabrotica speciosa. PLoS ONE 9:e113280. doi: 10.1371/journal.pone.0113280
Schiavon, M., Pizzeghello, D., Muscolo, A., Vaccaro, S., Francioso, O., and Nardi, S. (2010). High molecular size humic substances enhance phenylpropanoid metabolism in maize (Zea mays l.). J. Chem. Ecol. 36, 662–669. doi: 10.1007/s10886-010-9790-6
Schmelz, E. A., Engelberth, J., Alborn, H. T., Tumlinson, J. H., and Teal, P. E. (2009). Phytohormone-based activity mapping of insect herbivore-produced elicitors. Proc. Natl. Acad. Sci. U.S.A. 106, 653–657. doi: 10.1073/pnas.0811861106
Sedaghatbaf, R., Samih, M. A., Zohdi, H., and Zarabi, M. (2018). Vermicomposts of different origins protect tomato plants against the sweetpotato whitefly. J. Econ. Entomol. 111, 146–153. doi: 10.1093/jee/tox238
Shah, Z. H., Rehman, H. M., Akhtar, T., Alsamadany, H., Hamooh, B. T., Mujtaba, T., et al. (2018). Humic substances: determining potential molecular regulatory processes in plants. Front. Plant Sci. 9:263. doi: 10.3389/fpls.2018.00263
Sobhy, I. S., Erb, M., Sarhan, A. A., El-Husseini, M. M., Mandour, N. S., and Turlings, T. C. (2012). Less is more: treatment with BTH and laminarin reduces herbivore-induced volatile emissions in maize but increases parasitoid attraction. J. Chem. Ecol. 38, 348–360. doi: 10.1007/s10886-012-0098-6
Song, X., Liu, M., Wu, D., Griffiths, B. S., Jiao, J., Li, H., and Hu, F. (2015). Interaction matters: synergy between vermicompost and PGPR agents improves soil quality, crop quality and crop yield in the field. Appl. Soil Ecol. 89, 25–34. doi: 10.1016/j.apsoil.2015.01.005
Stout, M. J., Zehnder, G. W., and Baur, M. E. (2002). Potential for the use of elicitors of plant resistance in arthropod management programs. Arch. Insect Biochem. Physiol. 51, 222–235. doi: 10.1002/arch.10066
Tomczyk, A. (2002). Changes in secondary plant metabolites in cucumber leaves induced by spider mites and plant growth promoting rhizobacteria (PGPR). IOBC WPRS Bull. 25, 67–70.
Trevisan, S., Botton, A., Vaccaro, S., Vezzaro, A., Quaggiotti, S., and Nardi, S. (2011). Humic substances affect arabidopsis physiology by altering the expression of genes involved in primary metabolism, growth and development. Environ. Exp. Bot. 74, 45–55. doi: 10.1016/j.envexpbot.2011.04.017
Valenzuela-Soto, J. H., Estrada-Hernández, M. G., Ibarra-Laclette, E., and Délano-Frier, J. P. (2010). Inoculation of tomato plants (solanum lycopersicum) with growth-promoting bacillus subtilis retards whitefly bemisia tabaci development. Planta 231, 397–410. doi: 10.1007/s00425-009-1061-9
Van Oosten, V. R., Bodenhausen, N., Reymond, P., Van Pelt, J. A., Van Loon, L., Dicke, M., et al. (2008). Differential effectiveness of microbially induced resistance against herbivorous insects in arabidopsis. Mol. Plant Microbe Interact. 21, 919–930. doi: 10.1094/MPMI-21-7-0919
Vasseur-Coronado, M., du Boulois, H. D., Pertot, I., and Puopolo, G. (2021). Selection of plant growth promoting rhizobacteria sharing suitable features to be commercially developed as biostimulant products. Microbiol. Res. 245:126672. doi: 10.1016/j.micres.2020.126672
Vos, I. A., Verhage, A., Schuurink, R. C., Watt, L. G., Pieterse, C. M., and Van Wees, S. (2013). Onset of herbivore-induced resistance in systemic tissue primed for jasmonate-dependent defenses is activated by abscisic acid. Front. Plant Sci. 4:539. doi: 10.3389/fpls.2013.00539
Walters, D., and Heil, M. (2007). Costs and trade-offs associated with induced resistance. Physiol. Mol. Plant Pathol. 71, 3–17. doi: 10.1016/j.pmpp.2007.09.008
Wong, W. S., Zhong, H. T., Cross, A. T., and Yong, J. W. (2020). “Plant biostimulants in vermicomposts: characteristics and plausible mechanisms,” in The Chemical Biology of Plant Biostimulants, eds D. Geelen and L. Xu (Wiley), 155–180. doi: 10.1002/9781119357254.ch6
Ye, M., Veyrat, N., Xu, H., Hu, L., Turlings, T. C., and Erb, M. (2018). An herbivore-induced plant volatile reduces parasitoid attraction by changing the smell of caterpillars. Sci. Adv. 4:eaar4767. doi: 10.1126/sciadv.aar4767
Zamioudis, C., and Pieterse, C. M. (2012). Modulation of host immunity by beneficial microbes. Mol. Plant-Microbe Interact. 25, 139–150. doi: 10.1094/MPMI-06-11-0179
Zebelo, S., Song, Y., Kloepper, J. W., and Fadamiro, H. (2016). Rhizobacteria activates (+)-δ-cadinene synthase genes and induces systemic resistance in cotton against beet armyworm (Spodoptera exigua). Plant Cell Environ. 39, 935–943. doi: 10.1111/pce.12704
Zehnder, G., Kloepper, J., Tuzun, S., Yao, C., Wei, G., Chambliss, O., et al. (1997a). Insect feeding on cucumber mediated by rhizobacteria-induced plant resistance. Entomol. Exp. Appl. 83, 81–85.
Keywords: induced plant defense, plant stimulants, integrated pest management, plant growth promoting rhizobacteria, PGPR, humic acid, HA, natural enemies
Citation: Pereira RV, Filgueiras CC, Dória J, Peñaflor MFGV and Willett DS (2021) The Effects of Biostimulants on Induced Plant Defense. Front. Agron. 3:630596. doi: 10.3389/fagro.2021.630596
Received: 17 November 2020; Accepted: 04 June 2021;
Published: 22 July 2021.
Edited by:
Elvira S. De Lange, University of California, Davis, United StatesReviewed by:
Morgane Ourry, INRA Centre Bretagne-Normandie, FranceSilvia Proietti, University of Tuscia, Italy
Copyright © 2021 Pereira, Filgueiras, Dória, Peñaflor and Willett. This is an open-access article distributed under the terms of the Creative Commons Attribution License (CC BY). The use, distribution or reproduction in other forums is permitted, provided the original author(s) and the copyright owner(s) are credited and that the original publication in this journal is cited, in accordance with accepted academic practice. No use, distribution or reproduction is permitted which does not comply with these terms.
*Correspondence: Denis S. Willett, deniswillett@cornell.edu