- Department of Biochemistry, Microbiology and Biotechnology, Kenyatta University, Nairobi, Kenya
Cowpea [Vigna unguiculata (L.) Walp] is an important crop for smallholder farmers in the marginal areas of sub-Saharan Africa. However, the crop growth and production are affected by low soil fertility due to poor soil management practices. Here, we assessed the effect of native and commercially available rhizobia inoculants on cowpea nodulation, growth, and yields on three local cowpea genotypes (K-80, M-66, and KVU 27-1) in the semiarid areas of Kenya. Field experiments were set in smallholder farms during the 2019 and 2020 cropping seasons. Native rhizobia were isolated from root nodules of cowpea plants used as trap cultures. The isolates were further assessed for symbiotic efficiency (SE) in the greenhouse and field experiments carried out during the short and long rain seasons. Field experiments were laid out in a randomized complete block design with three replications. The treatments consisted of the commercial inoculant (Biofix), native isolates, native + Biofix (consortium), and an uninoculated control. In the greenhouse, the native isolates significantly increased nodule number and dry weight (DW), shoot DW, and root DW when compared to the uninoculated control. Additionally, 50% of the isolates recorded SE of >80%, while 35.7 and 14.3% of the isolates had SE of 51–80 and <50%, respectively. In the field, rhizobia inoculation significantly (P < 0.05) increased nodulation and shoot DW compared to the uninoculated controls. Remarkably, rhizobia inoculation significantly increased yields where inoculation with native isolates recorded 22.7% increase in yield when compared to uninoculated control in the first season and 28.6% increase in yield in the second season. However, the rhizobia inoculants did not show a preference for any of the cowpea genotypes, and their performance was influenced by season and the study location. Our results demonstrate the existence of superior native isolates with potential to be developed to low-cost biofertilizer for sustainable cowpea production.
Introduction
Cowpea [Vigna unguiculata (L.) Walp] is an indigenous leafy vegetable and a grain legume widely grown in the semiarid areas of sub-Saharan Africa (Da Silva et al., 2018). The crop is vital in these areas due to its drought tolerance and ability to grow under water stress conditions, which are frequently experienced due to the global climate change (Agbicodo et al., 2009; Carvalho et al., 2017). Cowpea production in Africa accounts for over 95% of the global production, thus serving as a major source of food to millions of people globally (Samireddypalle et al., 2017). The crop provides both nutritional and nutraceutical benefits, including quality and cheap dietary proteins when consumed both as a vegetable and grain legume (Baptista et al., 2017; Da Silva et al., 2018). Considering its nutritional benefits and resilience under changing climate, cowpea has been recommended as one of the underutilized crops with the potential to alleviate food insecurity, malnutrition, and poverty in sub-Saharan Africa (Owade et al., 2020). In West Africa, the dual-purpose varieties also serve as a source of fodder for the livestock, making cowpea production an attractive venture to the farmers (Samireddypalle et al., 2017). Besides, when integrated into crop rotation systems, cowpea promotes buildup of soil organic matter and carbon and nitrogen fixation. This, in turn, promotes soil fertility and improve the soil physical characteristics such as the water infiltration and retention capacity (Sánchez-Navarro et al., 2019a,b).
The growth and production of cowpea are significantly limited by extreme weather conditions such as drought and heat stress caused by the changing climate (Farooq et al., 2017). Coupled with low soil fertility, this has continuously limited cowpea production primarily due to the high cost associated with inorganic fertilizers (Oruru et al., 2018). Unlike drought, soil fertility in the semiarid areas can be improved to enhance the cowpea production. Nitrogen (N) is one of the limiting nutrients in the semiarid regions, which adversely affect plant growth (Kwena et al., 2019). The ability of cowpea to fix nitrogen through biological nitrogen fixation is a cheap and sustainable alternative to inorganic fertilizers. This is because the performance of cowpea depends on the rhizospheric characteristics; hence, it is able to form a beneficial association with microorganisms present in the rhizosphere (Abdel-Fattah et al., 2016). Rhizobia, a Gram-negative soil rhizobacteria is among the microorganisms present in the cowpea rhizosphere, which establishes a symbiotic association with cowpea (Hamza and Alebejo, 2017). The association between cowpea and rhizobia results in the biological nitrogen fixation (BNF), which benefits the plant by supplying N. Nevertheless, in many smallholder agroecosystems, the indigenous rhizobia isolates are low in population or are not efficient in N fixation (Ngakou et al., 2007), necessitating inoculation with commercial isolates.
Rhizobia inoculation has been regarded as a sustainable and cost-effective technology to augment the plants' N needs (Ondieki et al., 2017). Several studies have reported that inoculation with rhizobia improves legume growth, nutrition, and production (Takács et al., 2018; Aserse et al., 2020). However, inoculation with proven rhizobia inoculants as well as some commercial inoculants has at times failed to yield positive results when inoculation is done in regions with different agroenvironmental conditions to the original habitat. In cowpea, failed response to commercial inoculants has mainly been associated with the availability of more effective native rhizobia (Mathu et al., 2012). The ability of the native rhizobia strains to establish positive interactions with other soil microorganisms and their adaptability to the soil and environmental conditions often explicates their superior characteristics over the introduced strains (Meghvansi et al., 2010).
Native rhizobia inoculated on different legumes including cowpea, common bean, green grams, soybean, and lentils have enhanced legume productivity (Mathu et al., 2012; Ouma et al., 2016; Tena et al., 2016; Koskey et al., 2017). This has been linked to the native rhizobia growth-enhancing traits as well as being well-adapted to the soil and environmental stresses, hence forming effective interactions with the host crops (Mwangi et al., 2011). The effectiveness of rhizobia in fixing N in the soil is also influenced by the cowpea genotype (Leite et al., 2009). The compatibility between rhizobia and the host crop is critical to avoid neutral or negative plant–microbe interactions that do not support crop growth (Xavier and Germida, 2003; Wang et al., 2011). Accordingly, most legume genotypes are non-promiscuous and nodulate with specific rhizobial strains in the rhizosphere (Fauvart and Michiels, 2008). Therefore, the use of a compatible host–rhizobia association may lead to better optimization of the host nutrition, leading to superior plant growth and yield. This necessitates identification of rhizobia isolates and cowpeas genotypes that best responds to this association in terms of growth and production, especially in adverse environmental conditions such as semiarid areas.
The arid and semiarid areas cover about 35% of the global landmass and support over 20% of the global population (Tchakerian, 2015). Much of these areas are highly degraded and eroded, hence underutilized owing to the low soil fertility, including N deficiency and drought experienced in these areas. Cowpea, being a drought-tolerant crop, is suited for the semiarid regions. Enhancement of soil fertility is vital to improve its production to meet the increasing food demands of the growing global population. With cowpea relying on BNF for its nutrition (Pule-Meulenberg et al., 2010), use of rhizobia forming effective symbiotic interaction is a potential sustainable technique to enhance its production in the semiarid areas. We hypothesized that native rhizobia inhabiting the smallholder farms in the semiarid regions of Kenya have different N fixing abilities, and inoculation of rhizobia with cowpea would improve cowpea growth and production. Therefore, the main objective of this work was to identify effective native rhizobia isolates and determine their effect on the nodulation, growth, and yield of different cowpeas genotypes in semiarid areas of Kenya where cowpea is mainly grown.
Materials and Methods
Experimental Sites and Soil Characteristics
The study was carried out in the semiarid areas of Eastern Kenya, which is part of sub-Saharan Africa. Field experiments were set up in 15 selected smallholder farms situated in the semiarid zones of three counties, namely, Tharaka Nithi, Kitui, and Embu. The experiments were conducted during the short and long rain seasons running from October 2019 to February 2020 and March 2020 to July 2020, respectively. The five farms in Tharaka Nithi were situated in the Tunyai area (0°10′33″ S, 37°50′12″ E), which lies at an elevation of 600–1,500 m above sea level (asl). The area is characterized by shallow, stony, and low fertile soil, which requires frequent replenishment of nutrients through the application of organic or inorganic fertilizer (Smucker, 2002; Jaetzold et al., 2006). The soils are sandy loam with physicochemical characteristics ranging from clay, 12–18%; sand, 44–76%; silt, 8–12%; pH 5.7–7.1; total organic carbon (TOC), 1–2.07%; total N, 0.12–0.18%; and P, 15–23 mg kg−1. In Kitui, the five farms were situated at Matinyani (1°18′30.6″ S 37°59′30.2″ E). Matinyani is 400–1,800 m asl with soils characterized by a loose structure and good water infiltration. The soils have sandy loam to sandy clay loam characteristics with physicochemical characteristics ranging from clay, 16–34%; silt, 2–6%; sand, 62–82%; pH 5.74–6.7; TOC, 0.67–1.04%; total N, 0.05–0.11%; and P, 20–100 mg kg−1. In Embu County, the farms were located in Karurumo area (0°29′12″ S, 37°41′50″ E), which lies at an elevation of 1,174 m asl. The soils are sandy clay with physicochemical characteristics ranging from sand, 46–50%; clay, 42–46%; silt, 6–14%; TOC, 1.33–1.77%; pH 5.4–6.1; total N, 0.14–0.16%; and P, 25–50 mg kg−1. The three sites are in the lower midland agroecological zone, receive bimodal rainfall, and are relatively hot and dry. The mean monthly rainfall and the maximum and minimum temperature during the experimental period are given in Figure 1 has been provided in the article. The major crops cultivated in the three areas include maize, sorghum, millet, common beans, green grams, cowpea, and mangos. However, cowpea is the most preferred legume due to its ability to grow in relatively low soil moisture and its drought tolerance.
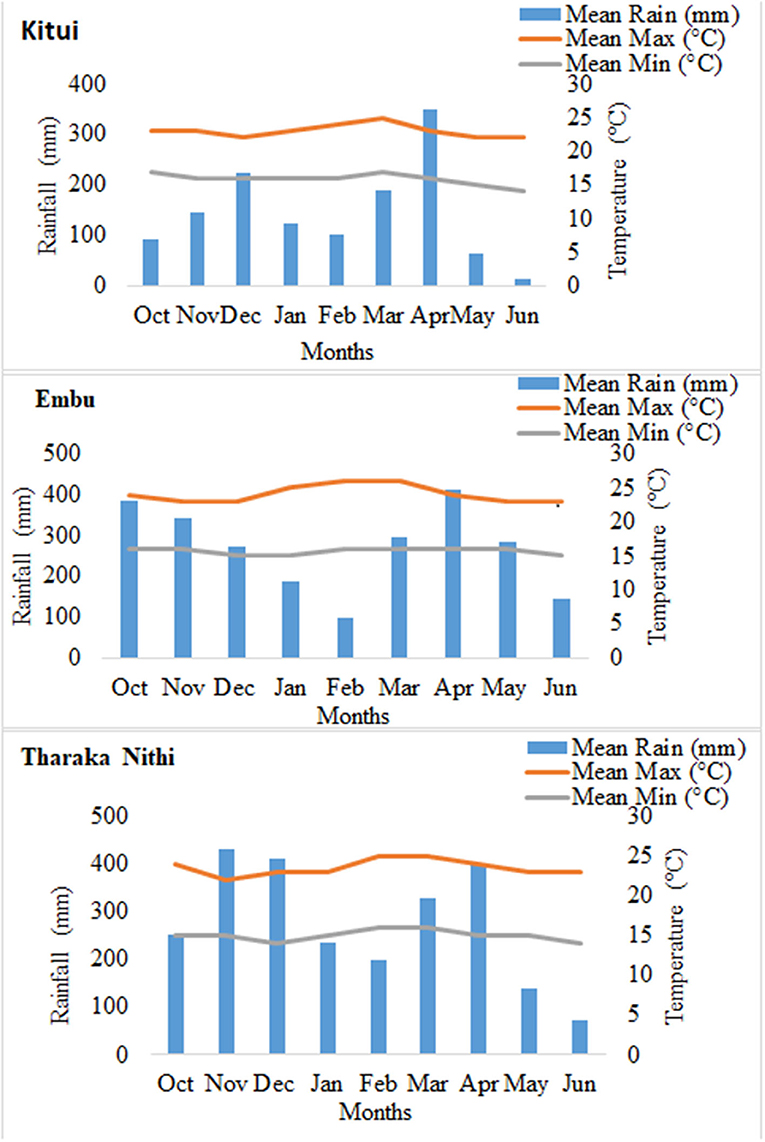
Figure 1. Rainfall and temperature in Kitui, Embu, and Tharaka Nithi study sites from October 2019 to June 2020.
Native Rhizobia Trapping
Field trap cultures were set in selected smallholder farms in the three counties using three cowpea genotypes: Katumani 80 (K80), Machakos 66 (M66), and KVU 27-1. These genotypes are locally grown by smallholder farmers in the study areas and also recommended by the Kenya Agricultural and Livestock Research Organization (KALRO) for the regions. The trapping experiment was done between June and July 2019. The farms were plowed, followed by demarcation into planting plots measuring 2 × 2 m. Cowpea seeds were planted at a spacing of 40 × 15 cm, with each genotype being replicated three times in each farm. Six weeks after planting, three cowpea plants per plot were randomly uprooted, and the root nodules were detached and wrapped with an absorbent paper towel. The nodules were then placed in khaki bags, labeled, and transported to the Kenyatta University, Microbiology Laboratory for rhizobia isolation.
Isolation of Rhizobia
The detached nodules were washed with sterile distilled water to remove adhering soil particles and soaked for 2 h to imbibe and soften for eased crushing. The softened nodules were surface sterilized using 70% ethanol, rinsed with sterile distilled water, and placed in 3% sodium hypochlorite for 2 min. The sterilized nodules were then serially rinsed in six changes of sterile distilled water. After sterilization, the nodules were crushed and plated on the yeast extract mannitol agar (YEMA) containing Congo red dye (0.025 g in 1 L). The Petri plates were incubated at 28 ± 1°C in the dark for 48 h. Single colonies identified on the plates were restreaked three to four times on plates containing freshly prepared YEMA until pure isolate cultures were obtained.
Morphological and Biochemical Characterization
The pure isolates were grouped according to their morphological and biochemical features as described by Ondieki et al. (2017). Individual colonies incubated for 48 h were characterized based on their size, shape, color, elevation, transparency, and margin. The isolates were also classified as fast or slow growing by plating them in YEMA containing bromothymol blue (BTB) (0.025 g/L) followed by a 3-day incubation at 28°C in the dark. Gram staining of the isolates was carried out following the procedure described by Beck et al. (1993). Isolates with similar morphological and biochemical characteristics were grouped together.
Authentication and Symbiotic Efficiency of the Isolates
Authentication and symbiotic efficiency assessment were done in an even span greenhouse with roll-up sides having natural lighting of 12 h. The temperature and relative humidity of the greenhouse ranged from 22 to 28° and 61 to 80%, respectively. A representative isolate in each group was selected for authentication as nodule-forming isolate. Axenic broth cultures of the isolates were inoculated on 7-day-old cowpea seedling grown in sterile vermiculite and supplemented with N-free nutrient solution and maintained for 45 days. The plants were then uprooted and scored for nodulation. Isolates that initiated nodule formation were selected to assess their symbiotic efficiency.
Cowpea seeds of high quality were sterilized using 70% ethanol followed by 3% sodium hypochlorite and finally rinsed in six changes of sterile distilled water. Two seeds were planted in sterile N-free vermiculite in each Leonard jar assembly and supplied with sterile N-free nutrient solution (Broughton and Dilworth, 1971). The assemblies were then put in khaki bags for insulation. Seven days after planting, the seedlings were inoculated with 1 ml [109 colony forming units (CFU)/ml] of broth culture per seedling of the authenticated isolates. Uninoculated plants supplemented with potassium nitrate (KNO3) (1 g/L) as well as those inoculated with a commercial strain (USDA 3456 from MEA Limited Kenya, which is marketed as Biofix) served as positive controls. Uninoculated plants with the N-free nutrient solution served as the negative control. Each treatment had four replicates laid out in a completely randomized design (CRD). Forty-five days after inoculation, the plants were carefully uprooted and the roots washed under running water. The nodules were then detached from the roots and counted. The obtained nodules, roots, and shoots were air dried and their weights determined. The symbiotic efficiency (SE) of the rhizobia isolates was determined following the procedure described by Karaca and Uyanöz (2012). The total dry weight of inoculated plants/total dry weights of N-supplied plants ×100. Isolates that recorded SE higher than 80% were rated as very effective, those with 51–80% as effective, 35–50% as lowly effective, and those with < 35% as ineffective (Koskey et al., 2017).
Land Preparation in the Field
The study sites were prepared before the onset of the rains in September 2019 and February 2020 during the first and second season, respectively, where all the farms were cleared of existing vegetation, followed by plowing and leveling. Cowpea crop was grown in the same farms in both seasons with the change in the specific location on each of the farms in the second season. Plots measuring 2 × 2 m were demarcated with a 1-m space between the plots. Planting holes were prepared at a spacing of 40 × 15 cm in each plot. This is because the soil in the study sites is easily eroded by wind and water; hence, planting holes are necessary to prevent loss of seeds through erosion.
Experimental Design and Treatments
The study comprised three cowpea genotypes, Katumani 80 (K80), Machakos 66 (M66), and KVU 27-1, and four rhizobia treatments arranged in a randomized complete block design (RCBD) with three replications. The rhizobia treatments included Native isolates, Biofix, a mixture of the Biofix and Native (Consortium), and no inoculation (control). The native isolates consisted of a consortium of three highly effective isolates identified from the greenhouse experiment, which also associated with all the three cowpea genotypes in all the three study sites, which were Is2, Is16, and Is19 (Native). Based on 16S ribosomal RNA (rRNA) gene sequencing, these isolates were identified as Rhizobium tropici clone H53, Mesorhizobium sp. WSM3874, and Rhizobium pusense strain Nak353, respectively.
Inoculum Application and Planting
Application of the inoculum on the cowpea seeds was done in the field under a shade before planting. Native isolates were inoculated on the seeds as broth using sugar as the sticker material for the rhizobia inoculum. Biofix was applied to the seeds following the manufacturers' guidelines (100 g of inoculum per 15 kg of seeds). The control plots with no rhizobia treatment were planted first to avoid cross-contamination, followed by other treatments. Two cowpea seeds were planted per planting hole.
Cowpea Crop Management
Gapping was done immediately after germination in both seasons. The plots were kept free of weeds by occasionally weeding as needed. At the onset of flowering, the plants were sprayed with Aceprid 20 WSP (Acetamiprid 200 g/kg) to prevent attack by thrips that infest the cowpea flowers, causing flower abortion and subsequently leading to declined yields. A second spray with Evisect (Thiocyclam hydrogen oxalate) was done to control the whiteflies that had infested the cowpea plants. The experiment was rain fed with the rainfall and temperature averages during the growing seasons indicated in Figure 1 has been provided in the article.
Field Sampling
Six weeks after germination, during the flowering stage, the cowpea plants were sampled to collect data on their growth and nodulation. Three plants per plot were randomly selected and carefully uprooted. All the nodules were detached from the roots, counted, wrapped in an absorbent paper towel, and placed in labeled khaki bags. The height and the number of leaves per plant were also determined. The samples were transported to Kenyatta University Microbiology Laboratory, where the shoots and nodules dry weights were determined.
Harvesting
Cowpea was harvested in February 2020 and July 2020 in the first and second season, respectively, when the plants had reached physiological maturity. Three cowpea plants per plot were randomly selected and harvested. For each plant, the seeds were separated from the pods. The seeds and the stover were then transported to the laboratory where they were dried and weighed. In addition, the 100 seed weight per plot was determined.
Statistical Analysis
The data on nodulation, shoot dry weight, yield, and stover weight were analyzed using analysis of variance (ANOVA) in statistical analysis software (SAS) version 9.1. Before data analysis, data were tested for homogeneity of variance using the Bartlett test. To fulfill assumptions of analysis of variance, log transformation was done where necessary and later back-transformed for presentation in tables. The means were separated at a 5% level of significance by the Tukey's honest significance difference (HSD).
Results
Rhizobia Isolation and Morphological Grouping
One hundred and three isolates were obtained from the root nodules of the three cowpea genotypes (K80, M66, and KVU 27-1) in the three study regions. Out of these, 38 isolates were from Tharaka Nithi County, 36 from Embu County, and 29 from Kitui County. The isolates had colony and growth characteristics of rhizobia as described by Somasegaran and Hoben (1994). Based on these characteristics, the isolates were grouped into 20 distinct groups. Group 19 had the highest number of isolates (16.5%), while groups 12, 13, and 15 had the least with (0.9%) (Table 1). All the isolates were Gram-negative and did not absorb Congo red dye when cultured in YEMA containing the dye and turned YEMA-BTB media from green to yellow. Sixteen isolates changed YEMA-BTB from green to yellow within 3 days, while the other four had the media change between 7 and 10 days. The isolates exhibited diverse characteristics, as indicated in Table 1.
Authentication and Symbiotic Efficiency of the Native Isolates
Authentication was based on the presence of at least one nodule in the roots of the cowpea plants. Out of the 20 representative group isolates, nodulation was positive in 14 groups with no presence of nodules observed in groups 4, 5, 8, 9, 11, and 18. Further, the symbiotic efficiency of the isolates that induced nodulation was determined together with their effect on cowpea nodulation and shoot and root dry weights upon inoculation. There were significant differences (P < 0.05) in nodule number (Nod No), dry nodule weight (Nod DW), shoot dry weight (DW), root DW, and symbiotic efficiency (SE) (Table 2). Is13 recorded the highest Nod No (50.00 ± 8.15), which was not statistically different from the rest of the isolates except for Is15, which had the least number of nodules (0.75 ± 0.48). Biofix recorded the highest Nod DW, which was similar to all the tested isolates except Is6, Is15, and Is16. As expected, uninoculated control and KNO3-supplemented plants did not form any nodules. Inoculation with the different native rhizobia isolates increased the shoot DW. Is1, Is2, Is3, Is14, Is16, and Is19 recorded shoot DW statistically similar to that of Biofix and KNO3-supplemented plants. The rest of the isolates recorded shoot DW significantly lower than that of Biofix and KNO3-supplemented plants with the uninoculated control having the least shoot DW (0.20 ± 0.03 g plant−1) (Table 2). Is13 recorded the highest root DW, which was statistically similar to Is1, Is2, Is7, Is14, Is16, Is19, and Is20, while the uninoculated control recorded the least root DW. Out of the 14 isolates tested, 7 had SE higher than 80%, hence classified as very effective (Table 2). This represented 50% of the isolates. Five other isolates with SE ranging between 51 and 80% were rated as effective. Only two isolates had SE below 50% (Is6 and Is12) and were both rated as lowly effective in fixing N.
Cowpea Nodulation and Growth
The Nod No and Nod DW were significantly increased by rhizobia inoculation (P < 0.0001) during season 1 when compared to the control (Table 3). However, in season 2, rhizobia inoculation recorded no significant effect on the Nod No. Nevertheless, native and consortium recorded the highest Nod DW, while the control had the least Nod DW in season 2. Overall, better nodulation was observed in season 1 compared to season 2. For example, the consortium that had the highest number of nodules in both seasons recorded 41.58 ± 3.60 and 14.02 ± 0.82 nodules plant−1 in seasons 1 and 2, respectively. Of the three genotypes, K-80 and KVU 27-1 registered statistically high Nod No when compared to M-66 in both seasons (Table 3). While no significant difference in Nod DW among the three genotypes was recorded in season 1, in season 2, KVU 27-1 showed the highest Nod DW (0.05 ± 0.01 g plant−1), while M-66 had the least (0.036 ± 0.00 g plant−1). The Nod DW in K-80 was not significantly different from the other two genotypes. The nodule number and dry weight also differed significantly among the three sites in the two seasons. In season 1, Tharaka Nithi recorded the highest Nod No and Nod DW, while in season 2 Embu recorded the highest Nod No and Nod DW (Table 3). Kitui County recorded the least Nod No in both seasons. Additionally, the site × rhizobia inoculant interaction significantly (P < 0.05) affected Nod No in season 1 (Table 3). During this season, plants inoculated with consortium recorded a higher nodule number in all the sites than when inoculated with native and Biofix. Moreover, the site × inoculant interaction was also significant for Nod DW in both seasons, whereby plants inoculated with native showed increased Nod DW in all the three study locations.
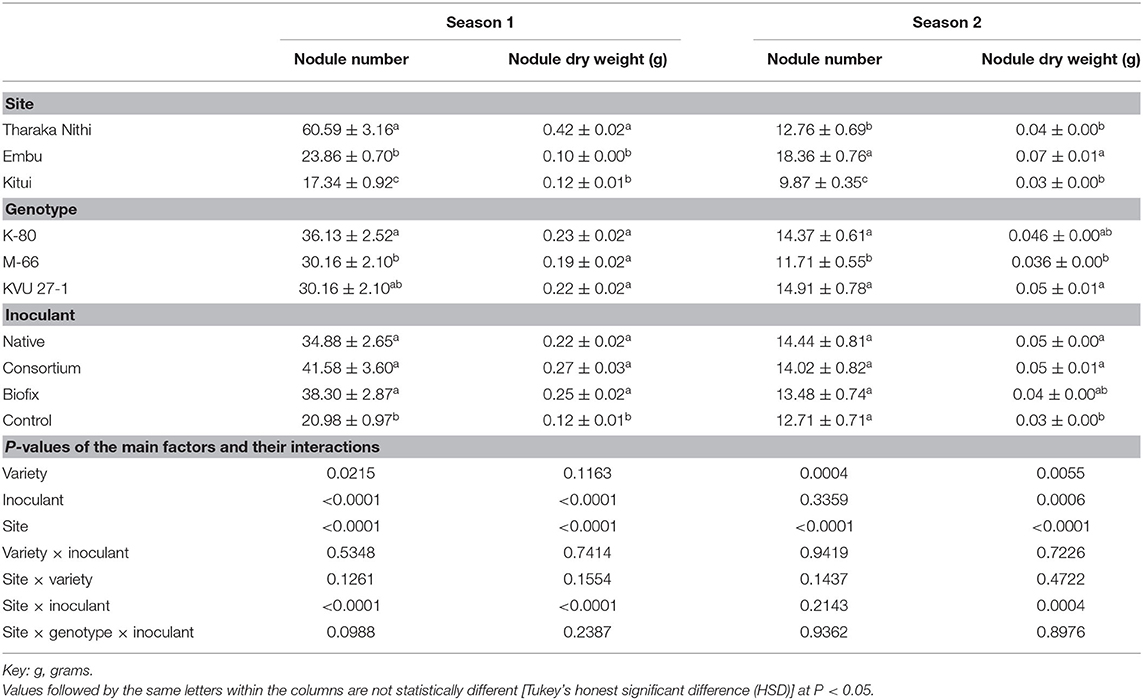
Table 3. Effect of rhizobia inoculation on nodule number and nodule dry weight at flowering stage on three cowpea genotypes in three field sites.
Rhizobia inoculation led to increased shoot DW at flowering when compared to the uninoculated controls. However, no significant effect was recorded on the number of leaves per plant and the height of plants in both seasons (Table 4). Additionally, the number of leaves, plant height, and shoot DW were statistically similar among the three cowpea genotypes in season 1. Among the study locations, cowpea inoculated with rhizobia in Tharaka Nithi County recorded the highest (32.51 ± 1.40 g plant−1) shoot DW, while those in Kitui had the least (8.18 ± 0.29 g plant−1). Embu County recorded the highest number of leaves and plants heights, followed by Tharaka Nithi and Kitui, respectively (Table 4). In season 2, there was no significant difference in the shoot DW and plant height among the three genotypes. However, K-80 and KVU 27-1 were more productive in terms of the number of leaves, while M-66 had the least number of leaves per plants. Among the study locations, the number of leaves and shoot DW recorded in Embu and Tharaka Nithi were not statistically different. Cowpea plants in Kitui County recorded the least number of leaves, plants height, and shoot DW (Table 4).
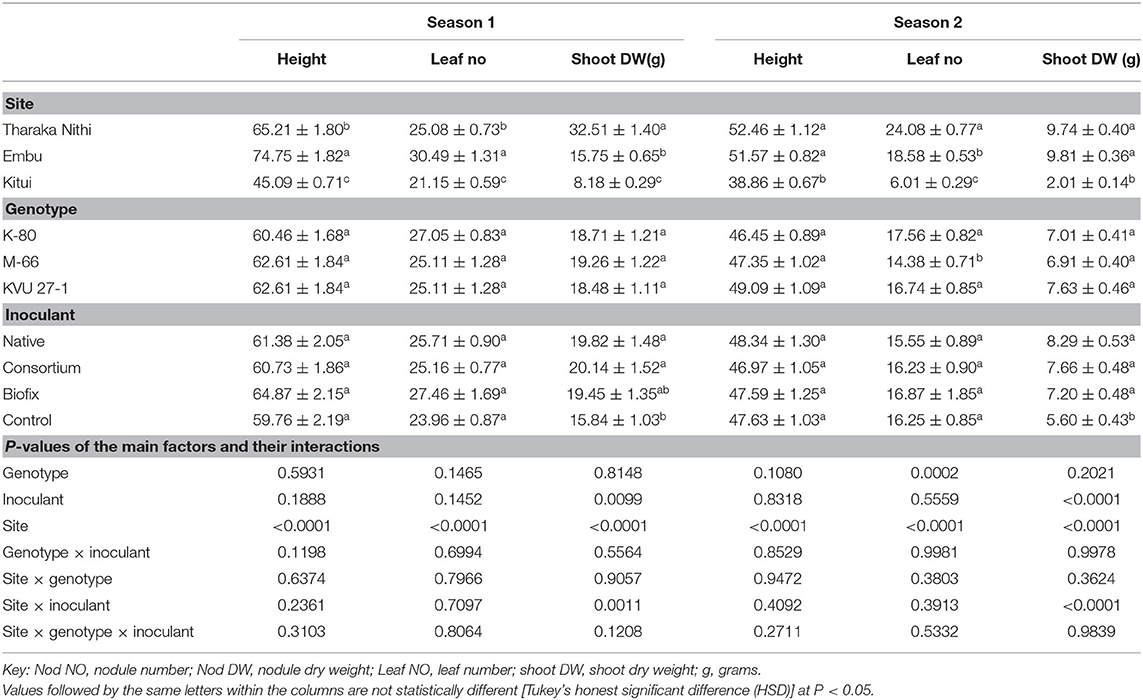
Table 4. Effect of rhizobia inoculation on cowpea plants height, leaf number and shoot dry weight at flowering on three cowpea genotypes in three field sites.
Moreover, the site × inoculant interaction on the shoot DW was significant at P < 0.05 in both seasons (Table 4). In season 1, plants inoculated with the consortium and native isolates in all the three sites recorded significantly high shoot DW. In comparison, cowpea plants inoculated with native isolates recorded the highest shoot DW in all the three locations in season 2.
Effect of Rhizobia Inoculation on Cowpea Yield
Rhizobia inoculation significantly (P < 0.05) increased cowpea yields in both seasons. In season 1, cowpea inoculated with the native isolates recorded the highest yields (940.90 ± 71.88 kg ha−1), while the control had the least (766.60 ± 61.86 ha−1), which is a 22.7% increase when compared to the uninoculated control. Consortium and Biofix recorded an 18 and 7.8% increase, although this did not differ significantly from the uninoculated control (Table 5). Similarly, cowpea inoculated with native isolates recorded the highest yields in season 2 (800 ± 79.75 kg ha−1), which was a 28.6% increase over the uninoculated control. This was not significantly different from yields of plants inoculated with the consortium (716.83 ± 68.77 kg ha−1). However, this differed significantly from those inoculated with Biofix and the uninoculated control (Table 5). Additionally, cowpea plants inoculated with Biofix produced seeds with the highest 100 seed weight in season 1. However, their weights did not differ significantly with those inoculated with native and consortium at P < 0.05. Uninoculated plants recorded the least 100 seed weight in season 1. In contrast, all treatments recorded similar 100 seed weight in season 2 (Table 5). Similarly, the influence on rhizobia inoculation on the stover weight was only recorded in season two (Table 5).
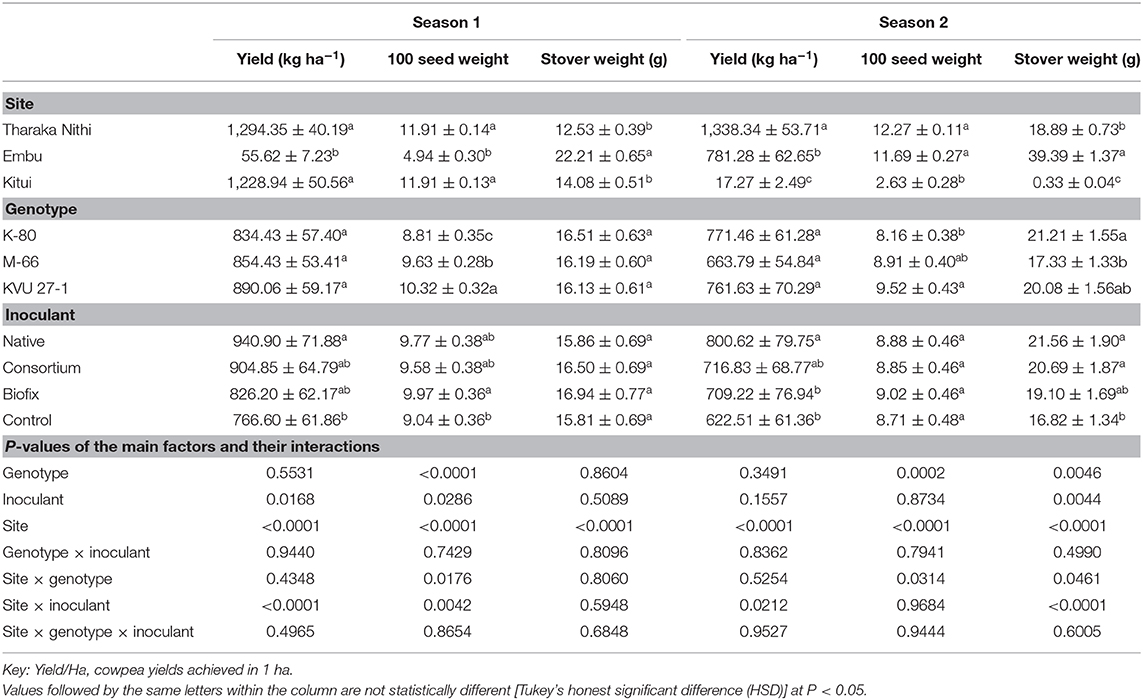
Table 5. Effect of rhizobia inoculation on yield, 100 seed weight, and dry stover weight over two growing seasons on three cowpea genotypes in three field sites.
The seeds' quality in terms of the 100 seed weight depended on the cowpea genotype (Table 5). KVU 27-1 and K-80 recorded the highest and lowest 100 seed weight across the two seasons. A significant genotype × inoculant interaction was recorded on the 100 seed weight in both seasons. In this case, plants inoculated with native and Biofix yielded the highest 100 seed weight in seasons 1 and 2, respectively. While no significant difference in stover weight was observed in season 1 among the three genotypes, K-80 recorded the highest stover weight at harvest in the second season.
Additionally, cowpea yield, 100 seed weight, and the stover weight were significantly (P <0.0001) influenced by the study site in both seasons. In the first season, Tharaka Nithi and Kitui regions recorded the highest yields, while Embu recorded the least yields (55.62 ± 7.23 kg ha−1). Tharaka Nithi recorded the highest yields during the second season, which was significantly higher than that of Embu, while Kitui recorded the lowest yields (Table 5). Additionally, there was a significant interaction between site and inoculant in both seasons (Table 5). In both cases, inoculation with native isolates led to better yields when compared to the other inoculants. Correspondingly, Tharaka Nithi and Kitui regions had the highest 100 seed weight, while Embu had the least during the first season. In the second season, Kitui recorded a significantly low 100 seed weight compared to Tharaka Nithi and Embu. The highest stover weight was recorded in the Embu region during the two seasons. It was significantly higher than in the other two areas. However, no significant difference in stover weight between Kitui and Tharaka Nithi was observed in season 1, although they differed significantly in season 2. A significant site × genotype interaction on stover weight was recorded in the second season. In this case, K-80 plants recorded the highest stover weight in Embu and Tharaka Nithi, while M-66 plants recorded the highest stover weight in Kitui.
Discussion
Morphological and Biochemical Classification
In this study, we sought to identify effective native rhizobia isolates and determine their potential to promote nodulation, growth, and yield of different cowpeas genotypes, which are grown in semiarid areas of Kenya. The results of the field trapping experiment identified morphological and biochemical characteristics of native rhizobia associating with the cowpea genotypes on YEMA media (Somasegaran and Hoben, 1985). All the isolates were Gram-negative and did not absorb Congo red dye when incubated in the dark. The isolates were white, cream white, or milky white, which is an indication that they did not absorb Congo red. According to Somasegaran and Hoben (1985), one of the distinctive characteristics of rhizobia is their inability to absorb Congo red dye. Further, the isolates turned YEMA-BTB media from green to yellow. This indicated that all the isolates were fast growing and were producing acid while growing. Fast-growing rhizobia acidify YEMA-BTB, turning it from green to yellow, while the slow growing alkalize the YEMA-BTB from green to blue (Boakye et al., 2016). The fast growth characteristic of rhizobia has been found as an adaptive characteristic of rhizobia growing in arid and semiarid areas as a survival strategy that enables them to multiply rapidly over a short duration of time (Borges et al., 2010). The significant difference in the isolates' morphological characteristics could be an indicator of the existence of diverse native rhizobia-nodulating cowpea in the study locations. Isolates well adapted to the local environmental and climatic conditions with a high BNF could be considered for the development of commercial rhizobia inocula (Berrada et al., 2012).
Authentication and Symbiotic Efficiency of the Isolates
Results of the greenhouse experiment showed that inoculation with the native isolates significantly increased nodulation and shoot and root DW when compared to the uninoculated control. The potential of the symbiotic interaction in benefiting the legume crop is dependent on the effectiveness of the rhizobia to fix N into ammonia. The most effective rhizobia strains on the legumes have been utilized through the application as biofertilizer, which is a sustainable technique for promoting legume production (Stajkovic et al., 2011). Most of the native isolates exhibited superior performance in nodulation, shoot DW, root DW, and SE. This reflects the existence of superior rhizobia strains in the family farming systems, which have the potential for exploitation as low-cost inoculants to improve cowpea production. The isolates that yielded the highest nodule numbers also translated to a high nodule dry weight. This mirrors effective symbiosis between the host and rhizobia especially in cases where it translates to higher biomass production (Gyogluu et al., 2018). However, for isolate Is13, the high nodule number did not translate to a high shoot DW. This indicates that the nodule number alone may not reflect the effectiveness of an isolate in fixing N. Some rhizobia strains can form a large number of nodules but have little or no N fixing abilities (Abd El-Maksoud and Keyser, 2010). These strains may have parasitic behaviors; hence, they nodulate with their host but are not efficient in N fixation (Denison and Kiers, 2004). This is especially because legumes cannot consistently discriminate against strains with low N fixation abilities.
Compared to uninoculated control, inoculation of cowpea with native rhizobia increased the shoot DW and root DW. The increased shoot DW on legumes following inoculation with native rhizobia could be attributed to the fact that rhizobia enhanced plant growth leading to increased biomass production (Kawaka et al., 2014; Jalloh et al., 2020; Matse et al., 2020). The high root DW and high shoot DW of native treated plants could be due to the production of plant-growth-promoting hormones such as 3-indoleacetic acid (IAA) by the native isolates (Mabrouk et al., 2018). In KNO3-treated plants and the negative controls, no nodules were formed, which confirmed the absence of any contamination. The use of sterile vermiculite ensured the absence of any rhizobia contaminants in the controls, hence the lack of N fixation attributing to the reduced biomass formation and, subsequently, the low shoot DW in the negative control (Muleta et al., 2017). The better growth of cowpea recorded in native isolates compared to the Biofix and the uninoculated controls indicate that the native isolates are superior, hence have the potential for exploitation as rhizobia inoculants to enhance cowpea production.
Effectiveness of Rhizobia in Field Conditions
Rhizobia inoculation enhanced cowpea nodulation and increased shoot dry weight at flowering when compared to the control plants. The consortium and native isolates showed superior performance in all the tested parameters at the flowering stage. While their performance did not differ significantly from the performance of Biofix, the Nod DW and shoot DW in seasons 2 and 1, respectively, following Biofix inoculation was not significantly different from the uninoculated controls. Consortium's enhanced performance could be associated with the native and commercial rhizobia strains working synergistically to infect and induce nodule formation on cowpea. Due to this positive synergistic interaction, the Biofix was able to form a positive interaction in a new environment with native rhizobia in the soil, hence forming an association with cowpea, which led to increased nodulation and nodule dry weights although not as effective as the native isolates. Commercial rhizobia strain effectively nodulate and fix N with other different legume crops (Ulzen et al., 2016; Kyei-Boahen et al., 2017). However, in the presence of more superior native rhizobia than the introduced strains, inoculation causes no significant effect on nodulation when compared to the uninoculated controls (Mathu et al., 2012). The superiority of the native isolates could be linked with good adaptability to the local agroecological environment. Therefore, their reintroduction increases their numbers in the fields, leading to increased N fixation. Reintroduction of well-adapted rhizobia strains on legumes from which they were isolated has been reported to result in the formation of effective symbiotic associations leading to increased nodule formation and nodule dry weight (Matse et al., 2020).
Nodule formation on the roots of control plants could be associated with the free-living rhizobia present in the soil before planting. Therefore, the increased nodule formation following inoculation could result from increased rhizobia occupancy in the cowpea root nodules due to increased rhizobia population in the soil. The increased nodule occupancy leads to better N fixation, which is necessary for shoot development in plants (Abou-Shanab et al. (2019). Among the three cowpea genotypes, K-80 and M-66 formed the highest and lowest nodule number and nodule dry weight, respectively. The differences in legume varietal response to inoculation in terms of nodulation has been reported as an important trait in legume crop that can be exploited by plant breeders to have crops with a high N fixation ability (Hossain et al., 2016). This is especially in cowpea, where nodulation has been reported to be dependent on the cowpea genotype (Njeru et al., 2020). Although M-66 genotype had the least number and dry weight of nodules, the dry shoot matter did not differ significantly with the other two genotypes. This could be because all the three genotypes are well-adapted to both the study regions' environmental and ecological conditions (Recha et al., 2013). In addition, it seems all the three genotypes did not discriminate against the inoculants; thus, no interaction of inoculant by genotype was present, which suggests that the cowpea genotypes' response to inoculation was the same across the three study sites. However, in the study locations, significant differences were present in the nodulation and shoot dry weight. This could be associated with the varying soil physicochemical characteristics in the study sites. The performance of individual genotype did not differ across the location. However, a better response to inoculation was present in Tharaka Nithi when compared to the other two regions.
Similarly, the season of planting greatly influenced nodulation and the shoot dry matter. Tharaka Nithi and Kitui regions recorded a significantly low nodule number and dry weight in the second season. This could be associated with the unexpected low rainfall received in these region during the second season. Moisture stress and drought has been reported to limit nodule formation, subsequently limiting N fixation (Sindhu et al., 2020). This has been linked to increased acid phosphatases and antioxidant activity in the root nodules during drought conditions (Mouradi et al., 2018).
Cowpea yield in the field increased with inoculation. Remarkably, native isolates R. tropici clone H53, Mesorhizobium sp. WSM3874, and R. pusense strain Nak353 recorded the highest yield per hectare. At the same time, the performance of consortium and Biofix did not differ significantly from the control plants. This superiority of the native rhizobia isolates indicates that there exist effective rhizobia in these regions with the potential to enhance cowpea production. This could be associated with the better adaptability of native rhizobia to the ecological surrounding, hence their ability to infect and form a positive association with cowpea under the prevailing conditions (Koskey et al., 2017; Matse et al., 2020). Besides, the native rhizobia can form positive interactions with the naturally occurring soil microbiota, which enhances the host crop nutrition and health. This includes interactions with plant-growth-promoting bacteria and bioenhancers such as arbuscular mycorrhizal fungi, which increase the supply and access to other nutrients (Yadav and Verma, 2014; Karthikeyan and Arunprasad, 2019). According to Koskey et al. (2017), inoculation of common bean with native rhizobia outperformed other inoculants in respect to yield, which is in agreement with the findings of our study. The average performance of Biofix is linked to moderate adaptability and unfavorable agroecological setting in the study area, which negatively impacted the Biofix–cowpea association (Koskey et al., 2017). This is also seen in the cowpea response to inoculation with the consortium. This is mainly because the effectiveness of rhizobia inoculants on the improvement of yields is not dependent on the diverse rhizobia population of the inoculant. Inoculation with a diverse rhizobia population increases chances of incompatibility and negative interactions, which occurs at the expense of N fixation (Martinez-Romero, 2003). Additionally, cowpea is a promiscuous legume that enables it to nodulate with many rhizobia strains, including the ineffective strains that lead to poor responses to rhizobia inoculation (Kanonge-Mafaune et al., 2018). Therefore, it is necessary to use effective rhizobia adapted to the agroecological condition for maximum cowpea production. Besides the influence on yield, all the three inoculants significantly increased the stover weight at harvest during the second season when compared to the control. The stover is important, as it supplements the soil with nutrients and organic matter upon decomposition needed for the subsequent crop and soil structure improvement.
The rhizobia inoculants did not show any preference for any cowpea genotype in yields, as no significant interaction of rhizobia inoculant and cowpea genotype was observed. This could be attributed to the fact that all three genotypes have been bred to suit the environmental and climatic conditions of our study sites. There is a likelihood that these genotypes have been empirically selected to form efficient interaction with the indigenous rhizobia (De Freitas et al., 2012). This opposes the finding by Karasu et al. (2011), who reported a significant interaction between genotype and rhizobia inoculant. Further differences in the 100 seed weight could be attributed to the varying sizes of the seeds in each of the cultivars.
Additionally, the associated difference in stover weight among the genotypes could be linked to the different growth forms of these genotypes in the field. Similarly, significant differences were recorded in all the parameters across the three study sites between the two seasons. These differences can be attributed to the varying climatic conditions in the three locations in the two growing seasons. The significant differences in yields between seasons could be attributed to the extreme rainfall during the flowering and podding stage during the first season. The associated rainfall impact on the flowers and small pods causes flower and pod abortion, reducing the number of pods that reach maturity. This consequently affects the yields achieved per plant and, subsequently, the yield achieved per hectare.
Conclusion
Our study has demonstrated the existence of effective native rhizobia isolates in the smallholder farms with great potential to improve cowpea growth and yield under a changing climate. Interestingly, while all the inoculants enhanced nodulation, shoot DW, and yields, native isolates R. tropici clone H53, Mesorhizobium sp. WSM3874, and R. pusense strain Nak353 showed more superiority in all the tested parameters. Although the cowpea genotypes did not show any preference for any of the inoculants, the increased nodulation and yields following inoculation show that all genotypes performed similarly, and inoculation enhances their growth and yields. Further studies should focus on extensive field testing of the native isolates to identify the “best bet” isolates for the development of cheap and effective biofertilizer that will augment sustainable cowpea production in smallholder agroecosystems.
Data Availability Statement
The original contributions presented in the study are included in the article/Supplementary Materials, further inquiries can be directed to the corresponding author/s.
Author Contributions
EN conceived and designed the experiments. JN and EN participated in setting up the experiments, data collection, analysis, developed the manuscript, reviewed, and approved the final manuscript. All authors contributed to the article and approved the submitted version.
Funding
This work was supported by The Future Leaders—African Independent Researchers (FLAIR) Fellowship Programme, which is a partnership between the African Academy of Sciences and the Royal Society funded by the UK Government's Global Challenges Research Fund (Grant number FLR/R1/190944).
Conflict of Interest
The authors declare that the research was conducted in the absence of any commercial or financial relationships that could be construed as a potential conflict of interest.
Acknowledgments
The authors appreciate Abdul A. Jalloh, Priscilla Wakarera, Kibet Charles Kirui, Kipkorir Koech, Richard Awino, Morris Muthini, Reverend Anthony Njeru Jesse, and Mercy Karimi, for their precious help in conducting the field experiments. Moreover, we acknowledge the smallholder farmers from Tharaka Nithi, Embu, and Kitui counties of Kenya for their participation and cooperation in this study.
Supplementary Material
The Supplementary Material for this article can be found online at: https://www.frontiersin.org/articles/10.3389/fagro.2020.606293/full#supplementary-material
References
Abd El-Maksoud, H. K., and Keyser, H. H. (2010). Restriction specificity of some soybean genotypes to Bradyrhizobium japonicum serogrous. Int. J. Agric. Biol. Eng. 4, 824–827. doi: 10.5281/zenodo.1062044
Abdel-Fattah, G. M., Rabie, G. H., Lamis, D. S., and Rabab, A. M. (2016). The impact of the arbuscular mycorrhizal fungi on growth and physiological parameters of cowpea plants grown under salt stress conditions. IJASBT 4, 372–379. doi: 10.3126/ijasbt.v4i3.15775
Abou-Shanab, R. A. I., Wongphatcharachai, M., Sheaffer, C. C., and Sadowsky, M. J. (2019). Response of dry bean (Phaseolus vulgaris L.) to inoculation with indigenous and commercial Rhizobium strains under organic farming systems in Minnesota. Symbiosis 78, 125–134. doi: 10.1007/s13199-019-00609-3
Agbicodo, E. M., Fatokun, C. A., Muranaka, S., Visser, R. G. F., and Linden van der, C. G. (2009). Breeding drought tolerant cowpea: constraints, accomplishments, and future prospects. Euphytica 167, 353–370. doi: 10.1007/s10681-009-9893-8
Aserse, A. A., Markos, D., Getachew, G., Yli-Halla, M., and Lindström, K. (2020). Rhizobial inoculation improves drought tolerance, biomass and grain yields of common bean (Phaseolus vulgaris L.) and soybean (Glycine max L.) at Halaba and Boricha in Southern Ethiopia. Arch. Agron. Soil Sci. 66, 488–501. doi: 10.1080/03650340.2019.1624724
Baptista, A., Pinho, O., Pinto, E., Casal, S., Mota, C., and Ferreira, I. M. (2017). Characterization of protein and fat composition of seeds from common beans (Phaseolus vulgaris L.), cowpea (Vigna unguiculata L. Walp) and bambara groundnuts (Vigna subterranea L. Verdc) from Mozambique. J. Food Meas. Charact. 11, 442–450. doi: 10.1007/s11694-016-9412-2
Beck, D. P., Materon, L. A., and Afandi, F. (1993). Practical Rhizobium-legume technology manual. Pract. Rhizobium-Legume Technol. Manual. 9:389.
Berrada, H., Nouioui, I., Houssaini, M. I., Gtari, M., and Benbrahim, K. F. (2012). Phenotypic and genotypic characterizations of rhizobia isolated from root nodules of multiple legume species native of Fez, Morocco. Afr. J. Microbiol. Res. 6, 5314–5324. doi: 10.5897/AJMR11.1505
Boakye, E. Y., Lawson, I. Y. D., and Danso, S. K. A. (2016). Characterization and diversity of rhizobia nodulating selected tree legumes in Ghana. Symbiosis 69, 89–99. doi: 10.1007/s13199-016-0383-1
Borges, W. L., Xavier, G. R., and Rumjanek, N. G. (2010). Characterization of indigenous rhizobia from Caatinga. Braz. J. Microbiol. 41, 201–208. doi: 10.1590/S1517-83822010000100029
Broughton, W. J., and Dilworth, M. J. (1971). Control of leghaemoglobin synthesis in snake beans. Biochem. J. 125, 1075–1080. doi: 10.1042/bj1251075
Carvalho, M., Lino-Neto, T., Rosa, E., and Carnide, V. (2017). Cowpea: a legume crop for a challenging environment. J. Sci. Food Agri., 97, 4273–4284. doi: 10.1002/jsfa.8250
Da Silva, A. C., da Costa Santos, D., Junior, D. L. T., da Silva, P. B., dos Santos, R. C., and Siviero, A. (2018). “Cowpea: a strategic legume species for food security and health,” in Legume Seed Nutraceutical Research, eds. J. C. Jimenez-Lopez and A. Clemente (London: IntechOpen). doi: 10.5772/intechopen.79006
De Freitas, A. D. S., Silva, A. F., and Sampaio, E. V. D. S. B. (2012). Yield and biological nitrogen fixation of cowpea varieties in the semi-arid region of Brazil. Biomass Bioenergy 45, 109–114. doi: 10.1016/j.biombioe.2012.05.017
Denison, R. F., and Kiers, E. T. (2004). Lifestyle alternatives for rhizobia: mutualism, parasitism, and forgoing symbiosis. FEMS Microbiol. Lett. 237, 187–193. doi: 10.1111/j.1574-6968.2004.tb09695.x
Farooq, M., Gogoi, N., Barthakur, S., Baroowa, B., Bharadwaj, N., Alghamdi, S. S., et al. (2017). Drought stress in grain legumes during reproduction and grain filling. J. Agron. Crop. Sci. 203, 81–102. doi: 10.1111/jac.12169
Fauvart, M., and Michiels, J. (2008). Rhizobial secreted proteins as determinants of host specificity in the rhizobium–legume symbiosis. FEMS Microbiol. Lett. 285, 1–9. doi: 10.1111/j.1574-6968.2008.01254.x
Gyogluu, C., Mohammed, M., Jaiswal, S. K., Kyei-Boahen, S., and Dakora, F. D. (2018). Assessing host range, symbiotic effectiveness, and photosynthetic rates induced by native soybean rhizobia isolated from Mozambican and South African soils. Symbiosis 75, 257–266. doi: 10.1007/s13199-017-0520-5
Hamza, T. A., and Alebejo, A. L. (2017). Isolation and characterization of rhizobia from rhizosphere and root nodule of Cowpea, elephant and lab plants. IJNRIS 4, 1–7.
Hossain, Z., Wang, X., Hamel, C., Knight, J. D., Morrison, M. J., and Gan, Y. (2016). Biological nitrogen fixation by pulse crops on semi-arid Canadian prairies. Can. J. Plant Sci. 97, 119–131. doi: 10.1139/CJPS-2016-0185
Jaetzold, R., Schmidt, H., Hornetz, B., and Shisanya, C. (2006). “Farm management handbook of Kenya Vol. II–natural conditions and farm management information−2nd Edition part c; East Kenya Subpart C1 Eastern province,” in Ministry of Agriculture (Nairobi: Germany Agency for Technical Cooperation (GTZ)).
Jalloh, A. A., Njeru, E. M., and Maingi, J. M. (2020). Potential of native rhizobia isolates to improve production of legume crops in small holder farms. Biosci. Res. 17, 1498–1510. Available online at: https://ssrn.com/abstract=3653170
Kanonge-Mafaune, G., Chiduwa, M. S., Chikwari, E., and Pisa, C. (2018). Evaluating the effect of increased rates of rhizobial inoculation on grain legume productivity. Symbiosis 75, 217–227. doi: 10.1007/s13199-018-0550-7
Karaca, Ü., and Uyanöz, R. (2012). Effectiveness of native Rhizobium on nodulation and growth properties of dry bean (Phaseolus vulgaris L.). Afr. J. Biotechnol. 11, 8986–8991. doi: 10.5897/AJB11.4087
Karasu, A., Oz, M., and Dogan, R. (2011). The effect of bacterial inoculation and different nitrogen doses on yield and yield components of some dwarf dry bean cultivars (Phaseolus vulgaris L.). Bulg. J. Agric. Sci. 17, 296–305. Available online at: http://www.agrojournal.org/17/03-04-11.pdf
Karthikeyan, A., and Arunprasad, T. (2019). Growth response of Pterocarpus santalinus seedlings to native microbial symbionts (arbuscular mycorrhizal fungi and Rhizobium aegyptiacum) under nursery conditions. J. For. Res. 30, 1–7. doi: 10.1007/s11676-019-01072-y
Kawaka, F., Dida, M. M., Opala, P. A., Ombori, O., Maingi, J., Osoro, N., et al. (2014). Symbiotic efficiency of native rhizobia nodulating common bean (Phaseolus vulgaris L.) in soils of Western Kenya. Int. Sch. Res. Notices 2014:258497. doi: 10.1155/2014/258497
Koskey, G., Mburu, S. W., Njeru, E. M., Kimiti, J. M., Ombori, O., and Maingi, J. M. (2017). Potential of native rhizobia in enhancing nitrogen fixation and yields of climbing beans (Phaseolus vulgaris L.) in contrasting environments of Eastern Kenya. Front. Plant Sci. 8:443. doi: 10.3389/fpls.2017.00443
Kwena, K., Karuku, G. N., Ayuke, F. O., and Esilaba, A. O. (2019). Nitrogen deficiency in semi-Arid Kenya: can pigeon pea fix it? East Afr. Agric. Forestry J. 83, 322–340. doi: 10.1080/00128325.2019.1658696
Kyei-Boahen, S., Savala, C. E., Chikoye, D., and Abaidoo, R. (2017). Growth and yield responses of cowpea to inoculation and phosphorus fertilization in different environments. Front. Plant Sci. 8:646. doi: 10.3389/fpls.2017.00646
Leite, J., Seido, S. L., Passos, S. R., Xavier, G. R., Rumjanek, N. G., and Martins, L. M. V. (2009). Biodiversity of rhizobia associated with cowpea cultivars in soils of the lower half of the São Francisco River Valley. Rev. Bras. Ciênc. Solo 33, 1215–1226. doi: 10.1590/S0100-06832009000500015
Mabrouk, Y., Hemissi, I., Salem, I. B., Mejri, S., Saidi, M., and Belhadj, O. (2018). Potential of rhizobia in improving nitrogen fixation and yields of legumes. Symbiosis 107:73495. doi: 10.5772/intechopen.73495
Martinez-Romero, E. (2003). Diversity of Rhizobium-Phaseolus vulgaris symbiosis: overview and perspectives. Plant Soil 252, 11–23. doi: 10.1023/A:1024199013926
Mathu, S., Herrmann, L., Pypers, P., Matiru, V., Mwirichia, R., and Lesueur, D. (2012). Potential of indigenous bradyrhizobia versus commercial inoculants to improve cowpea (Vigna unguiculata L. walp.) and green gram (Vigna radiata L. wilczek.) yields in Kenya. J. Soil Sci. Plant Nutr. 58, 750–763. doi: 10.1080/00380768.2012.741041
Matse, D. T., Huang, C. H., Huang, Y. M., and Yen, M. Y. (2020). Nitrogen uptake and growth of white clover inoculated with indigenous and exotic Rhizobium strains. J. Plant Nutr. 43, 2013–2027. doi: 10.1080/01904167.2020.1758134
Meghvansi, M. K., Prasad, K., and Mahna, S. K. (2010). Symbiotic potential, competitiveness and compatibility of indigenous Bradyrhizobium japonicum isolates to three soybean genotypes of two distinct agro-climatic regions of Rajasthan, India. India. Saudi J. Biol. Sci. 17, 303–310. doi: 10.1016/j.sjbs.2010.06.002
Mouradi, M., Farissi, M., Bouizgaren, A., Qaddoury, A., and Ghoulam, C. (2018). Medicago sativa-rhizobia symbiosis under water deficit: physiological, antioxidant and nutritional responses in nodules and leaves. J. Plant Nutr. 41, 384–395. doi: 10.1080/01904167.2017.1385805
Muleta, D., Ryder, M. H., and Denton, M. D. (2017). The potential for rhizobial inoculation to increase soybean grain yields on acid soils in Ethiopia. Soil Sci. Plant Nutr. 63, 441–451. doi: 10.1080/00380768.2017.1370961
Mwangi, S. N., Karanja, N. K., Boga, H., Kahindi, J. H. P., Muigai, A., Odee, D., et al. (2011). Genetic diversity and symbiotic efficiency of legume nodulating bacteria from different land use systems in Taita Taveta, Kenya. Trop. Subtrop. Agroecosyst. 13, 109–118. Available online at: http://scielo.unam.mx/pdf/tsa/v13n1/v13n1a16.pdf
Ngakou, A., Nwaga, D., Ntonifor, N. N., Tamo, M., Nebane, C. L. N., and Parh, I. A. (2007). Contribution of arbuscular mycorrhizal fungi (AMF), rhizobia and Metarhizium anisopliae to cowpea production in Cameroon. Int. J. Agric. 2, 754–764. doi: 10.3923/ijar.2007.754.764
Njeru, E. M., Muthini, M., Muindi, M. M., Ombori, O., Nchore, S. B., Runo, S., et al. (2020). “Exploiting arbuscular mycorrhizal Fungi-Rhizobia-legume symbiosis to increase smallholder farmers' crop production and resilience under a changing climate,” in Climate Impacts on Agricultural and Natural Resource Sustainability in Africa, eds. B. Singh, A. Safalaoh, N. Amuri, L. Eik, B. Sitaula, and R. Lal (Cham: Springer), 471–488. doi: 10.1007/978-3-030-37537-9_27
Ondieki, D. K., Nyaboga, E. N., Wagacha, J. M., and Mwaura, F. B. (2017). Morphological and genetic diversity of Rhizobia nodulating cowpea (Vigna unguiculata L.) from agricultural soils of lower eastern Kenya. Int. J. Microbiol. 2017:8684921. doi: 10.1155/2017/8684921
Oruru, M. B., Njeru, E. M., Pasquet, R., and Runo, S. (2018). Response of a wild-type and modern cowpea cultivars to arbuscular mycorrhizal inoculation in sterilized and non-sterilized soil. J. Plant Nutr. 41, 90–101. doi: 10.1080/01904167.2017.1381728
Ouma, E. W., Asango, A. M., Maingi, J., and Njeru, E. M. (2016). Elucidating the potential of native rhizobial isolates to improve biological nitrogen fixation and growth of common bean and soybean in smallholder farming systems of Kenya. Int. J. Agron. 2016:4569241. doi: 10.1155/2016/4569241
Owade, J. O., Abong,', G., Okoth, M., and Mwang'ombe, A. W. (2020). A review of the contribution of cowpea leaves to food and nutrition security in East Africa. Food Sci. Nutr. 8, 36–47. doi: 10.1002/fsn3.1337
Pule-Meulenberg, F., Belane, A. K., Krasova-Wade, T., and Dakora, F. D. (2010). Symbiotic functioning and bradyrhizobial biodiversity of cowpea (Vigna unguiculata L. Walp.) in Africa. BMC Microbiol. 10:89. doi: 10.1186/1471-2180-10-89
Recha, J., Kinyangi, J., and Omondi, H. (2013). “Climate related risk and oppportunities for agricultural adaption and mitigation in semi-arid Eastern Kenya,” in CCAFS East Africa Program Project Report. (Copenhagen: CGIAR Research Program on Climate Change, Agriculture and Food Security).
Samireddypalle, A., Boukar, O., Grings, E., Fatokun, C. A., Kodukula, P., Devulapalli, R., et al. (2017). Cowpea and groundnut haulms fodder trading and its lessons for multidimensional cowpea improvement for mixed crop livestock systems in West Africa. Front. Plant Sci. 8:30. doi: 10.3389/fpls.2017.00030
Sánchez-Navarro, V., Zornoza, R., Faz, Á., and Fernández, J. A. (2019a). Comparing legumes for use in multiple cropping to enhance soil organic carbon, soil fertility, aggregates stability and vegetables yields under semi-arid conditions. Sci. Hortic. 246, 835–841. doi: 10.1016/j.scienta.2018.11.065
Sánchez-Navarro, V., Zornoza, R., Faz, Á., and Fernández, J. A. (2019b). Does the use of cowpea in rotation with a vegetable crop improve soil quality and crop yield and quality? A field study in SE Spain. Eur. J. Agron. 107, 10–17. doi: 10.1016/j.eja.2019.03.007
Sindhu, S., Dahiya, A., Gera, R., and Sindhu, S. S. (2020). Mitigation of abiotic stress in legume-nodulating Rhizobia for sustainable crop production. Agric. Res. 9, 444–459. doi: 10.1007/s40003-020-00474-3
Smucker, T. A. (2002). Land Tenure Reform and Changes in Land use and Land Management in Semi-Arid Tharaka, Kenya. Available online at: https://hdl.handle.net/10568/1889 (accessed August 14, 2020).
Somasegaran, P., and Hoben, H. J. (1985). Methods in Legume-Rhizobium Technology; NIFTAL, MIRCEN. Maui: University of Hawaii Press.
Somasegaran, P., and Hoben, H. J. (1994). “Collecting nodules and isolating Rhizobia,” in Handbook for Rhizobia, (New York, USA: Springer). doi: 10.1007/978-1-4613-8375-8_1
Stajkovic, O., Delic, D., Josic, D., Kuzmanovic, D., Rasulic, N., and Knezevic-Vukcevic, J. (2011). Improvement of common bean growth by co-inoculation with Rhizobium and plant growth-promoting bacteria. Rom. Biotechnol. Lett. 16, 5919–5926.
Takács, T., Cseresnyés, I., Kovács, R., Parádi, I., Kelemen, B., Szili-Kovács, T., et al. (2018). Symbiotic effectivity of dual and tripartite associations on soybean (Glycine max L. Merr.) cultivars inoculated with Bradyrhizobium japonicum and AM Fungi. Front. Plant Sci. 9:1631. doi: 10.3389/fpls.2018.01631
Tchakerian, V. P. (2015). “Hydrology, floods and droughts: deserts and desertification,” in Encyclopedia of Atmospheric Sciences, 2nd Edn, eds. J. Pyle and F. Zhang (Texas: Elsevier Inc.), 185–192. doi: 10.1016/B978-0-12-382225-3.00035-9
Tena, W., Wolde-Meskel, E., and Walley, F. (2016). Symbiotic efficiency of native and exotic Rhizobium strains nodulating lentil (Lens culinaris Medik.) in soils of Southern Ethiopia. Agronomy 6, 1–11. doi: 10.3390/agronomy6010011
Ulzen, J., Abaidoo, R. C., Mensah, N. E., Masso, C., and AbdelGadir, A. H. (2016). Bradyrhizobium inoculants enhance grain yields of soybean and cowpea in Northern Ghana. Front. Plant Sci. 7:1770. doi: 10.3389/fpls.2016.01770
Wang, X., Pan, Q., Chen, F., Yan, X., and Liao, H. (2011). Effects of co-inoculation with arbuscular mycorrhizal fungi and rhizobia on soybean growth as related to root architecture and availability of N and P. Mycorrhiza 21, 173–181. doi: 10.1007/s00572-010-0319-1
Xavier, L. J., and Germida, J. J. (2003). Selective interactions between arbuscular mycorrhizal fungi and Rhizobium leguminosarum bv. viceae enhance pea yield and nutrition. Biol. Fertil. Soils. 37, 261–267. doi: 10.1007/s00374-003-0605-6
Keywords: cowpea, native rhizobia, smallholder, sustainability, nitrogen fixation
Citation: Nyaga JW and Njeru EM (2020) Potential of Native Rhizobia to Improve Cowpea Growth and Production in Semiarid Regions of Kenya. Front. Agron. 2:606293. doi: 10.3389/fagro.2020.606293
Received: 14 September 2020; Accepted: 18 November 2020;
Published: 14 December 2020.
Edited by:
Rahul Mahadev Shelake, Gyeongsang National University, South KoreaReviewed by:
Anket Sharma, Zhejiang Agriculture and Forestry University, ChinaDinesh Panday, The University of Tennessee, Knoxville, United States
Copyright © 2020 Nyaga and Njeru. This is an open-access article distributed under the terms of the Creative Commons Attribution License (CC BY). The use, distribution or reproduction in other forums is permitted, provided the original author(s) and the copyright owner(s) are credited and that the original publication in this journal is cited, in accordance with accepted academic practice. No use, distribution or reproduction is permitted which does not comply with these terms.
*Correspondence: Ezekiel Mugendi Njeru, njeruezek@gmail.com