- 1RH Smith Institute of Plant Sciences and Genetics in Agriculture, Faculty of Agriculture, Food and Environment, The Hebrew University of Jerusalem, Rehovot, Israel
- 2Department of Plant Pathology and Weed Research, Newe Ya'ar Research Center, Agricultural Research Organization, Ramat Yishay, Israel
Three main Ambrosia species (Ragweed) grow in Israel; the most abundant invasive Ambrosia confertiflora DC, whereas A. artemisiifolia L. and A. tenuifolia Spreng., are of restricted distribution. The present research was aimed to study the effect of temperatures regimes on the development and growth of these Ambrosia species, to elucidate the environmental conditions and plant traits that affect their growth and infestation patterns. All three Ambrosia species germinate best in light from the soil surface with no prerequisite of a stratification period. A. confertiflora seed emergence is inhibited at high temperature regimes (28/34°C). A. artemisiifolia at low temperature regimes (10/16°C), while A. tenuifolia is less affected by the temperature regimes. A. confertiflora plant height increases with increasing temperatures, and at lower temperatures develops a rosette. Root and rhizome biomass were less affected by the different temperatures regimes; A. artemisiifolia aboveground mass was not affected by temperature regimes while A. tenuifolia aboveground mass was reduced only at lower temperatures. A. confertiflora fast invasion and establishment are due to the combined effects of prolific seed dispersal, rapid sprouting and growth, and its phenotypic plasticity.
Introduction
Biological invasions are a main threat to biodiversity in terrestrial, freshwater, and marine ecosystems, requiring extensive research and management efforts (Mačić et al., 2018). Invasive species are defined as animals, plants, or other organisms introduced by man into places out of their natural range of distribution, where they become established and disperse, generating a negative impact on the local ecosystem and species. A. artemisiifolia is known throughout the world as an invasive weed and in many countries it causes severe damages to agriculture and substantial health effects to millions of people, due to its high allergenic properties (Makra et al., 2005, 2014; Bullock et al., 2012; Montagnani et al., 2017; Schaffner et al., 2020). In Israel there are five Ambrosia species, but only A. confertiflora is defined as an invasive species (Dufour-Dror, 2016; Yair et al., 2019).
The origin of most Ambrosia species is in the Americas and they spread mainly by global commerce to Europe, Asia and Australia. The genus Ambrosia belongs to the Asteraceae family (Compositae) and includes 42 species (Strother, 2006). Ambrosia plants are annual or perennial monoecious with wind-pollinated flowers producing achenes. The wind-borne pollen grains cause severe allergenic response in humans and animals. The perennial species propagate by achenes and by underground rhizomes.
There are five Ambrosia species in Israel, which were detected in the early 1990's (Danin, 1994; Yair et al., 2017). Species details and characteristics are summarized in Table 1. The invasive perennial species A. confertiflora DC (Burr ragweed), is the most abundant in Israel, mainly found in Central Israel; Three naturalized perennial species A. tenuifolia Spreng; (Lacy ragweed) is found in several locations but its spread is limited; A. psilotachya DC (Cuman ragweed or Perennial ragweed and A. greyi (wooly leaf bur ragweed) are restricted to one location only. The fifth species A. artemisiifolia L. (short ragweed, common ragweed) an annual casual plant that appears in several sites but manages to survive only in the Hula Lake Natural Reserve in northern Israel. The causes of Ambrosia spp. invasions in Israel are mainly anthropogenic and are believed to originate with shipments of grain for food, feed and oil production from the USA. A, confertiflora is hardly studied. This perennial species originated in South-West US to Central Mexico and is invasive in Australia (Watt, 1987) and Israel (Yair et al., 2019), and may have a spread potential in south Europe (Tanner et al., 2017). A. confertiflora is a highly variable and complex erect plant, reaching up to 3 m in height, (Payne, 1964; Whisenant, 2018), which produces both achenes and rhizomes that create dense sprouts. The plant produces numerous viable hooked prickled achenes (1-2 mm long, as described in Yair et al., 2019) that are easily dispersed by animals and humans. A. tenuifolia produces rhizomes and achenes but the latter are non-prickled, hardly viable and thus their low dispersion potential (Yair et al., 2019).

Table 2. Three-parameter logistic nonlinear regressions between A. confertiflora emergence and time (days).

Table 3. Three-parameter logistic nonlinear regressions between A. artemisiifolia emergence and time (days).
Research regarding biological invasions and traits is mainly related to the A. artemisiifolia species that produces many achenes, 3,000–62,000 per plant (Dickerson and Sweet, 1971; Fumanal et al., 2007). Germination of seeds is optimal at soil level, decreases between 2 and 8 cm depth while in 10 cm depth no germination is observed, thus seed burial by deep tillage can reduce seed germination (Bassett and Crompton, 1975; Guillemin and Chauvel, 2011). Seeds of A. artemisiifolia require stratification at 4°C for 8 weeks or more and for maximum germination there is a preference for alternating temperatures and light (Willemsen and Rice, 1972; Willemsen, 1975). The minimum temperature requirement for germination is 5°C (Baskin and Baskin, 1980) and at optimal conditions, germination begins at 2 days (Ortmans, 2016). Seeds possess secondary dormancy (Bazzaz, 1970, 1979) and can germinate even after 40 years (Baskin and Baskin, 1977; Telewski and Zeevaart, 2002).
The aim of this research was to study the effect of temperature on the development and growth of A. confertiflora compared to the other Ambrosia species present in the Israeli flora, to elucidate the environmental conditions and plant traits that may explain the rapid distribution and vast infestation of A. confertiflora in Israel.
Materials and Methods
Germination and Emergence Experiments
Plants and seeds of the three Ambrosia species A. confertiflora, A. artemisiifolia, and A. tenuifolia, were collected from various open field areas between the years 2009–2014 and kept dry in paper bags at 4°C until use. For germination experiments, seeds were germinated in Petri dish on various substrates: Whatman No. 1 filter paper, sea sand, vermiculite, perlite, Rehovot sandy soil, and commercial potting soil (Tuff Merom Hagolan). Following the finding that the best substrate for germination was Whatman No. 1 filter paper, all experiments were conducted on two layers of this filter paper in 9 cm Petri dish, moistened with 500 microliter tap water, sealed with Parafilm and placed in a 25/18°C 16d/8n growth chamber. No germination in Petri dishes wrapped with aluminum foil to prevent light penetration was recorded hence all other experiments were conducted under light condions. Seeds were determined germinated once the radicle length reached the length of the achene.
For depth emergence experiments, plastic boxes (18 × 14 × 10 cm L/W/H) filled with sieved Rehovot sandy soil (1.2% clay, 3.3 % silt, 95.5% sand, 0.2% organic matter, pH 7.5) were used. For each box seeds of fifty A. confertiflora, or 20 A. artemisiifolia or A. tenuifolia were separately sown in rows at 0, 1, 2, 3, 4 cm soil depth, and placed in the phytotron under the following temperature regimes: 10/16°C, 16/22°C, 22/28°C, and 28/34°C night/day temperatures which represent the four seasons in the Mediterranean region. Pots were randomly placed, watered by hand with no fertilizer for 55 days. Emerging seedlings were recorded on a weekly basis.
Plant Development
For Ambrosia biomass experiments, four to six leaf A. confertiflora, A. artemisiifolia, and A. tenuifolia seedlings from each species, were transplanted into 3.9 L plastic pots filled with potting soil and placed in the phytotron under four temperature regimes as described above. Eight pots (= 8 replicates) were transferred to each temperature regime. Pots were randomly placed and watered by hand for 121 days. Weekly measurements of plant height, sprout number, inflorescence development and final plant mass (shoot, root, and rhizomes) were conducted. The fresh weight of plants parts was determined at harvest and the dry weight after placing the plants in an oven for 3 days at a temperature of 60°C. For convenience all results are presented in fresh weight.
Rhizome Development
To determine rhizome sprouts development of the two perennial species A. confertiflora and A. tenuifolia, 10 replicates of a single 6–8 leaf stage plant were transferred into the center of 50 × 30 × 6.5 cm plant trays containing potting soil, trays placed in the Faculty of Agriculture net-house in Rehovot, Israel. The Number of sprouts was recorded weekly until 111 DAS.
We conducted two experiments in the net-house at the same time of year- August to November in two subsequent years. The average of the two experiments is presented in the results.
Statistical Analysis
All experiments were conducted in a complete random design, repeated at least twice with five to six replications. The results were combined and analyzed as 10–12 replications. Analysis of variance was computed and means were compared using Tukey Kramer HSD test (P ≤ 0.05) using JMP ver. 13.0 (SAS Institute Inc. USA). When data were naturally quantative, a three-parameter nonlinear logistic equation was fitted using Sigma Plot 10 (SYStat Software, Inc. California, USA) (Ephrath et al., 2012):
Where f (x) represents the number of Ambrosia spp. emergence, a represents the upper asymptote (maximum emergence), x50 represents the time when Y is 50% of maximum, and b represents the slope at x50.
Results
Germination and Emergence Rates
In our Petri dish study, both A. artemisiifolia and A. confertiflora did not show a dormancy period thus do not require stratification. Attempts to enhance seed germination by physically cracking the seed coat, soaking in sodium hypochlorite, ethanol or gibberellic acid, were not successful. Seed germination initiated 2–3 days after seeding (DAS) and reached the maximum rate at 10 DAS. The average germination rate of A. confertiflora in Petri dish in light conditions was 40 and 56% for A. artemisiifolia. In dark conditions, A. confertiflora germination rate was only 0.4% and for A. artemisiifolia only 22%, indicating that both Ambrosia species require light for germination as was found at (Baskin and Baskin, 1980).
The achenes of these species do not require stratification for germination and stratification did not increase germination rate. Achenes of the plant germinated immediately after maturing and harvest. Dry achenes maintained viability for a long period. Three out of four A. confertiflora achenes collected by A. Danin in Yamit, Sinai in 1981 and kept at the Jerusalem Herbarium, successfully germinated in our studies 35 years later and produced viable plants.
The emergence of A. confertiflora achenes (seeds) in the pot experiment started 6 days after seeding and the highest emergence rate of 30% was recorded in the 10/16°C regime when the achenes were placed on the soil surface exposed to light. At this temperature regime, the burial of seeds at 1 cm depth was sufficient to drastically reduce seed emergence to 2% and at 4 cm depth, no emergence was recorded at all, indicating the importance of light for A. confertiflora emergence (Figure 1).
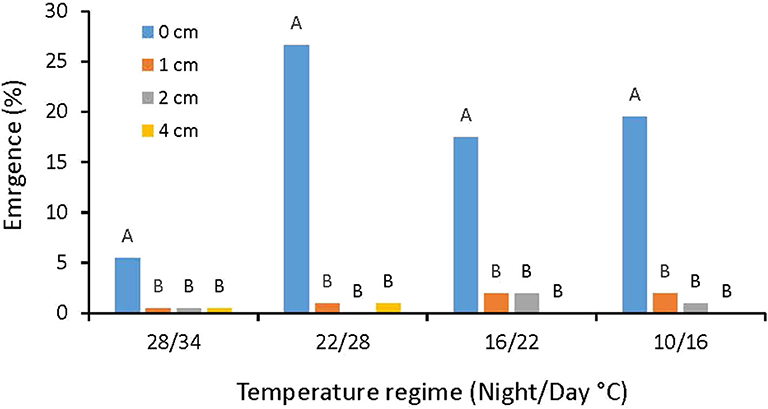
Figure 1. A. confertiflora emergence rate at four temperature regimes, 55 DAS. Columns within each temperature regime topped by different letters are significantly different according to Tukey-Kramer HSD, p = 0.05.
Seed emergence of A. confertiflora placed on the soil surface was inhibited at the highest temperature regime resulting in ca 5% emergence after 55 DAS, whereas at the lower temperature regimes the accumulated seed emergence rate reached a maximum of 28% at 55 DAS (Figure 2).
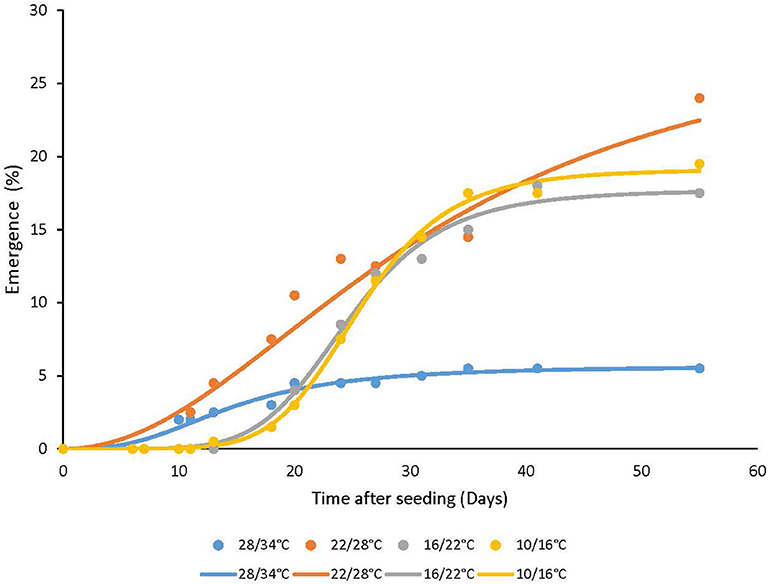
Figure 2. The effect of temperature regimes on the seedling emergence rate of A. confertiflora. Seed placed on the soil surface (0 cm seeding depth), 55 DAS. Three-parameter logistic nonlinear regressions between A. confertiflora emergence and time (days) were used. Parameters of the equations of four temperatures regimes are presented in Table 2.
The other perennial species, A. tenuifolia, that under field conditions produces a very low number of viable seeds, which in pots poorly germinated mainly from the soil surface (data not shown). A. artemisiifolia achenes which are bigger than those of other tested Ambrosia species, were less affected by the depth of seeding and germinated even from 4 cm depths (Figure 3). A. artemisiifolia achenes emergence rate from the soil surface was higher in high temperatures regimes (28/34°C, 22/28°C) as compared to lower temperatures regimes (10/16°C and 16/22°C), and started 6 days later than in the higher temperature regimes (Figure 4).
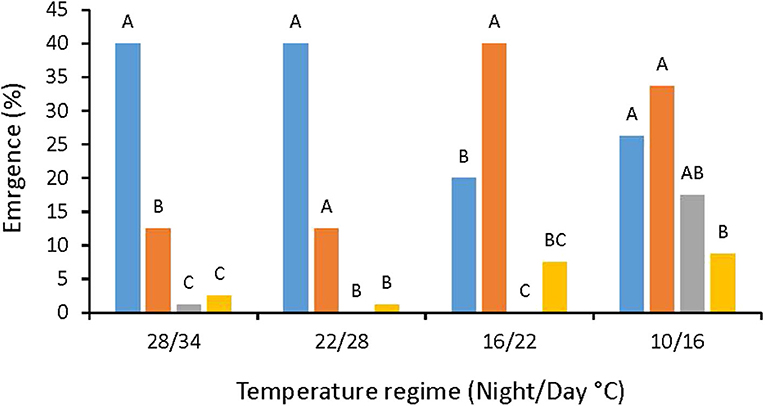
Figure 3. A. artemisiifolia emergence rates at four temperature regimes, 55 DAS. Columns within each temperature regime topped by different letters are significantly different according to Tukey-Kramer HSD, p = 0.05.
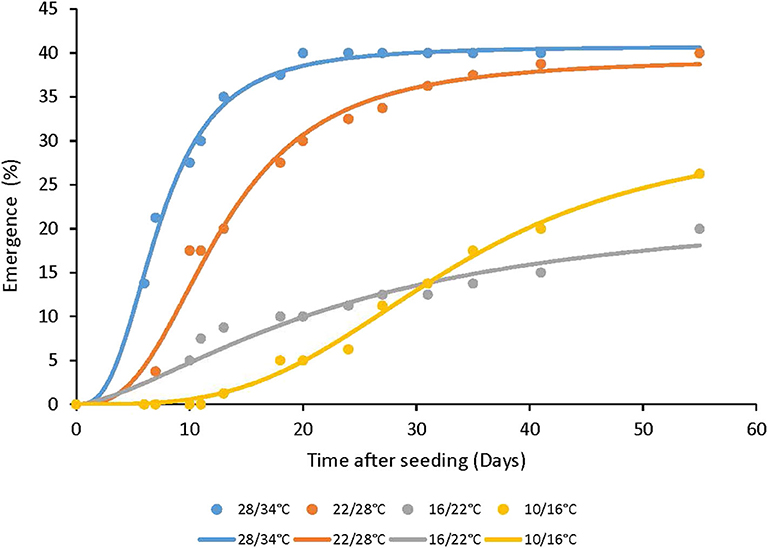
Figure 4. The effect of temperature regimes on the seedling emergence rate of A. artemisiifolia. Seeds were placed on the soil surface (0 cm seeding depth), 55 DAS. Three-parameter logistic nonlinear regressions between A. artemisiifolia emergence and time (days) were used. Parameters of the equations of four temperatures regimes are presented in Table 3.
Ambrosia Plant Development
Ambrosia species respond differently to different temperature regimes. Stem elongation differences were most evident in A. confertiflora as compared to A. tenuifolia and A. artemisiifolia, particularly at the two highest temperature regimes (Figure 5). Hence, at 100 DAS and 28/34°C regime A. confertiflora plants reached an impressing height of more than 180 cm (average height of 140 cm), whereas at the lowest temperature regime (10/16°C), the stem elongation was dramatically inhibited remaining at the rosette stage. Plant height of A. tenuifolia and A. artemisiifolia was less affected by the temperature regimes (Figure 5). A. tenuifolia plants were significantly shorter only at the lowest temperature regime, while in the A. artemisiifolia there was almost no effect to changes in temperature. A. confertiflora and A. artemisiifolia plants reach their maximal height at 60-80 DAS while A. tenuifolia maximal elongation was recorded at 100 DAS (data not shown).
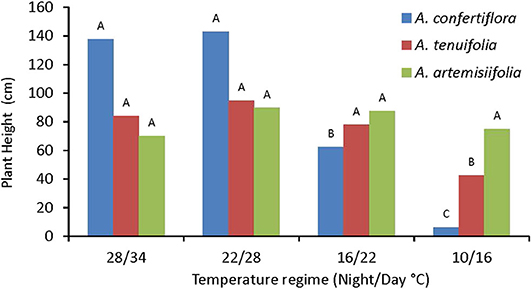
Figure 5. The effect of temperature regimes on plant height of A. confertiflora, A. tenuifolia and A. artemisiifolia at 121 DAS. Within a species (color), columns topped by different letters are significantly different according to Tukey Kramer HSD, p = 0.001.
The perennial species (A. confertiflora and A. tenuifolia), did not flower under long-day conditions throughout the current study. In contrary, flowering and fruit set were recorded in A. artemisiifolia as early as 25 DAS, in spite the fact that it is a short-day plant (Ziska et al., 2011), perhaps due to the reported short juvenile period in which it is not affected by day length (Deen et al., 1998).
Shoot biomass of the annual A. artemisiifolia was not affected by temperature, whereas the shoot biomass of A. confertiflora and A. tenuifolia was significantly reduced by the coldest temperature regime (Figure 6).
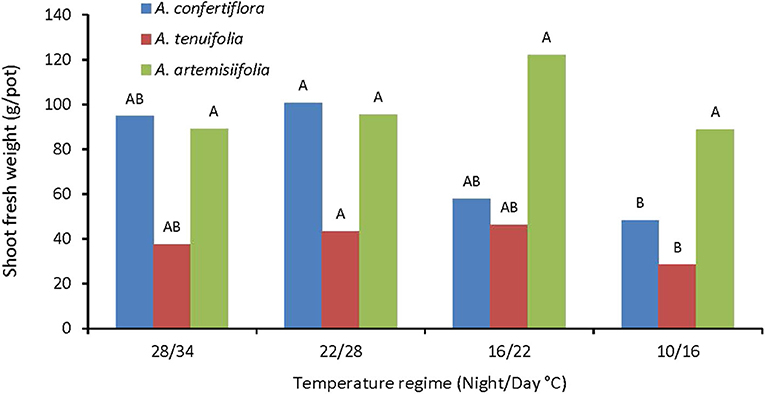
Figure 6. The effect of temperature regimes on the shoot fresh weight of A. confertiflora, A. tenuifolia, and A. artemisiifolia 121 DAS. Within a species (color), columns topped by different letters are significantly different according to Tukey Kramer HSD, p = 0.001.
In the perennial species, underground biomass (roots and rhizomes) was not affected by the temperature regimes, while the underground biomass (roots only) of the annual species was dramatically increased when grown under the coldest regime (10/16°C) (Figure 7). Thus, in order to elucidate the effect of temperature on resource allocation, we compared the effect of temperature on the ratio of underground/aboveground biomasses of the different species. The data presented in Figure 8 demonstrate that all tested species directed more resources to the underground organs as temperatures decline. This phenomenon was most evident in the annual A. artemisiifolia grown in the coldest conditions (10/16°C) that diverted 6-fold more resources to the roots than to the shoot. For A. confertiflora and A. tenuifolia the temperature effect was more moderate: the ratio was 2-3 in the coldest temperature regime and decreased to 0.5 in the warmest temperature regime (Figure 8).
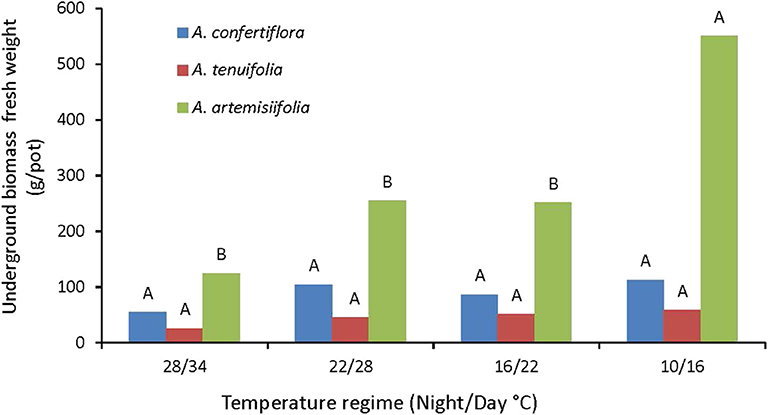
Figure 7. The effect of temperature regimes on the underground biomass accumulation of A. confertiflora, A. tenuifolia, and A. artemisiifolia 121 DAS. Within a species (color), columns topped by different letters are significantly different according to Tukey Kramer HSD, p = 0.001.
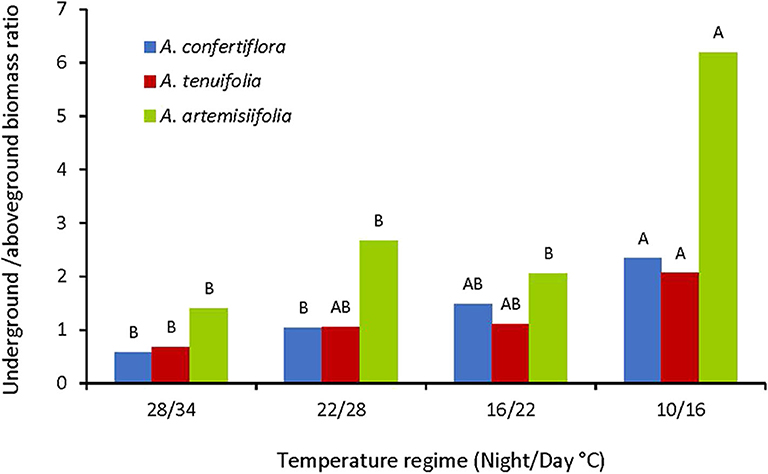
Figure 8. Effect of temperature regimes on underground/aboveground biomass ratio of A. artemisiifolia, A. confertiflora, and A. tenuifolia 121 DAS. Same colored bars topped with a same letter are not significantly different according to Tukey-Kramer HSD, p = 0.05.
Sprout Development
Sprouts develop from rhizomes in the perennial species A. confertiflora (Figure 9) and A. tenuifolia only, and not in the annual A. artemisiifolia. In the net-house experiments conducted during the summer and fall (August-November), sprouts first emerged from soil 25 DAS in both species. The average accumulated number of A. tenuifolia sprouts that developed from one mother plant at the end of the experiment (111 DAS) was five-fold higher than the number of sprouts that developed in A. confertiflora, 250 and 50, respectively (Figure 10).
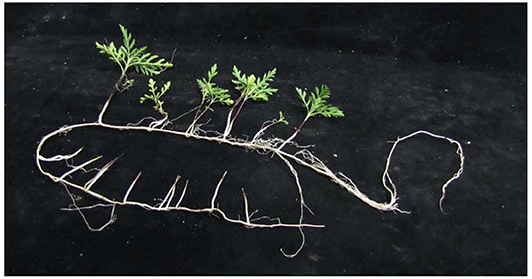
Figure 9. Sprouts of a single A. confertiflora rhizome that developed from a plant grown in a tray in the summer in the net-house 121 DAS.
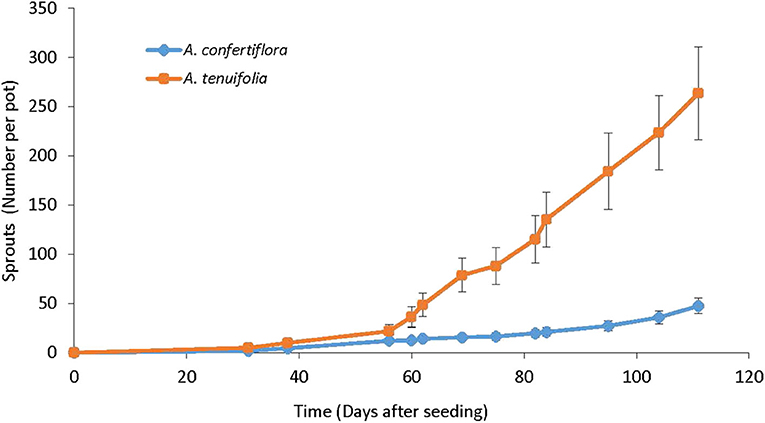
Figure 10. Accumulated number of A. confertiflora and A. tenuifolia rhizome sprouts that developed over time in pots in the net house from August to November, 111 DAS. Error bars represent the Standard Error of the means.
Discussion
This research elucidates the effect of temperature regimes on the early development and growth of three Ambrosia species that are in various distribution-status in Israel. The depth of seed placement in the soil strongly affects the germination and emergence of the three species: the seeds germinate well from the soil surface and up to 2 cm depth, as found also by Martínez et al. (2002). As these small seeds are placed deeper than 2 cm their emergence rate decreases accordingly (Farooq et al., 2019). The germination of A. confertiflora seeds are exceptionally sensitive to burial depth and they hardly emerge from more than 1 cm depth, while the bigger seeds of A. artemisiifolia possess more resources enabling them to emerge from deeper soil levels (2–4 cm), but as reported earlier they cannot emerge from depths >10 cm (Bassett and Crompton, 1975; Guillemin and Chauvel, 2011; Farooq et al., 2019). In contrast with other reports (Bazzaz, 1970, 1979; Willemsen and Rice, 1972; Willemsen, 1975; Baskin and Baskin, 1977, 1980; Leiblein-Wild et al., 2016), our germination studies showed that there is no need for stratification or other procedure to break the dormancy of A. artemisiifolia.
The two invasive species, A. confertiflora in Israel and A. artemisiifolia in Europe, demonstrate a higher phenotypic plasticity response to different temperatures, a common trait of invasive species. The effect of different temperatures on A. confertiflora and A. artemisiifolia seed germination is inverse: high temperatures reduce A. confertiflora germination rate and percentage, while the opposite occurs in A. artemisiifolia seeds as reported also by Gentili et al. (2019). As an annual plant, A. artemisiifolia is dependent mainly on the efficacy of seed germination and emergence for further favorable vegetative and reproductive growth (Gentili et al., 2019). The three examined Ambrosia species exhibit retarded growth in response to low temperatures, but A. confertiflora response to these low temperatures is the most extreme, leading to an increase in underground organs build-up, rosette formation and sprouting, while as temperature increases the stem elongates but the leaf number remains unchanged.
The two invasive A. artemisiifolia and A. confertiflora species have an interesting strategy of energy allocation following low temperatures to their underground plant parts (roots in A. artemisiifolia and rhizomes in A. confertiflora). In both A. confertiflora and A. artemisiifolia underground biomass increases 2 and 6-folds, respectively, in comparison to their above-ground mass. This ensures that plant resources are directed for supporting the establishment in soil under cold temperature periods. The annual short-day A. artemisiifolia plant grew rapidly, produced inflorescences and flowered 25 DAS, whereas A. confertiflora, did not flower throughout the four-month-long experiment. The high necessity to flower is not surprising as flowering is the sole way of reproduction of this annual plant, whereas the perennial Ambrosia species that reproduces also by sprouting is less dependent on seed production.
A. tenuifolia is not an invasive plant in Israel albeit the very high number of sprouts it rapidly produces. This is probably due to its less aggressive development in comparison to A. confertiflora and the fact that it produces a very low number of seeds, probably due to self-incompatibility (Friedman and Barrett, 2008). Furthermore, the seeds that are produced lack spines and are not dispersed by animals.
The naturalized A. artemisiifolia species is rapidly invading and spreading in Europe, Asia, and Australia. This annual invasive species successfully competes with local plant species due to its resistance to abiotic stresses, and its effective production and dispersion of viable achenes. Despite the sporadic detection of this species in Israel, A. artemisiifolia does not survive due to the dry summer in which the seedlings dry up and die. These plants can fit themselves to reduced moisture (Leiblein-Wild et al., 2014), but not to complete dryness such as the arid conditions in the Israeli summer. In highly moist conditions as in the reclaimed Hula Valley natural reserve in North-East of Israel, A. artemisiifolia grows rapidly and produces viable achenes, endangering the natural reserve and the neighboring irrigated agricultural fields (Yair et al., 2019).
The A. confertiflora species was first recorded in Israel in 1990 (Danin, 1994) and underwent a population explosion in less than 15 years (Yair et al., 2019), thus shifting from a naturalized to an invasive plant status. Several plant characteristics are responsible for this dramatic shift: fecundity, with the production of a vast number of viable achenes, easily caught on animal fur and other objects; ease of dispersion by man, farm equipment and the transportation of contaminated soil as well as rapid and long-distance seed transportation through water canals, streams and rivers (Yair et al., 2017). Additionally, rapid growth and fast sprouting contribute to its high competition with neighboring plants, resistance to temperature stress and growth plasticity may explain its rapid distribution and its establishment on river banks and fields adjacent to waterways.
Data Availability Statement
The raw data supporting the conclusions of this article will be made available by the authors, without undue reservation.
Author Contributions
BR, YY, and HE conceptualized and designed the overall study. YY, YG, and MS conducted the experimental work. YY, YG, MS, HE, and BR analyzed the data, wrote, and edited the manuscript. All authors contributed to the article and approved the submitted version.
Funding
The research was partially funded by fund No. 45-01-003 of the Chief Scientist of the Ministry of Agriculture.
Conflict of Interest
The authors declare that the research was conducted in the absence of any commercial or financial relationships that could be construed as a potential conflict of interest.
Acknowledgments
We wish to thank the Rubin lab staff in the Faculty of Agriculture, Food and Environment, The Hebrew University of Jerusalem for their support throughout this research program.
References
Baskin, J. M., and Baskin, C. C. (1977). Role of temperature in the germination ecology of three summer annual weeds. Oecologia 30, 377–382. doi: 10.1007/BF00399768
Baskin, J. M., and Baskin, C. C. (1980). Ecophysiology of secondary dormancy in seeds of Ambrosia artemisiifolia. Ecology 61, 475–480. doi: 10.2307/1937410
Bassett, I. J., and Crompton, C. W. (1975). The biology of Canadian weeds: 11. Ambrosia artemisiifolia L. and A. psilostachya DC. Can. J. Plant Sci. 55, 463–476. doi: 10.4141/cjps75-072
Bazzaz, F. (1970). Secondary dormancy in the seeds of the common ragweed Ambrosia artemisiifolia. B. Torrey. Bot. Club. 97, 302–310. doi: 10.2307/2483650
Bazzaz, F. A. (1979). Physiological ecology of plant succession. Annu. Rev. Ecol. Evol. Syst. 10, 351–371. doi: 10.1146/annurev.es.10.110179.002031
Bullock, J. M., Chapman, D., Schafer, S., Roy, D., Girardello, M., Haynes, T., et al. (2012). Assessing and controlling the spread and the effects of common ragweed in Europe, Final Report. Wallingford, UK: Natural Environment Research Council, 456.
Danin, A. (1994). Contributions to the flora of Israel and Sinai: VI Stipagrostis drarii and Ambrosia confertiflora and A tenuifolia, new records from Israel. Isr. J. Plant Sci. 42, 59–61. doi: 10.1080/07929978.1994.10676558
Deen, W., Hunt, T., and Swanton, C. J. (1998). Influence of temperature, photoperiod, and irradiance on the phenological development of common ragweed (Ambrosia artemisiifolia). Weed Sci. 46, 555–560. doi: 10.1017/S0043174500091098
Dickerson, C. T., and Sweet, R.D. (1971). Common ragweed ecotypes. Weed Sci. 19, 64–66. doi: 10.1017/S0043174500048281
Dufour-Dror, J. M. (2016). Improving the prevention of alien plant invasion in the EPPO region: the need to focus on highly invasive plants with (still) limited distribution–examples from Israel. EPPO Bul. 46, 341–347. doi: 10.1111/epp.12290
Ephrath, J. E., Hershenhorn, J., Achdari, G., Bringer, S., and Eizenberg, H. (2012). Use of logistic equation for detection of the initial parasitism phase of Egyptian broomrape (Phelipanche aegyptiaca) in tomato. Weed Sci. 60, 57–63. doi: 10.1614/WS-D-11-00070.1
Farooq, S., Onen, H., Ozaslan, C., Baskin, C. C., and Gunal, H. (2019). Seed germination niche for common ragweed (Ambrosia artemisiifolia L.) populations naturalized in Turkey. S. Afr. J. Botany 123, 361–371. doi: 10.1016/j.sajb.2019.03.031
Friedman, J., and Barrett, S. C. (2008). High outcrossing in the annual colonizing species Ambrosia artemisiifolia (Asteraceae). Ann. Bot. 101, 1303–1309. doi: 10.1093/aob/mcn039
Fumanal, B., Chauvel, B., and Bretagnolle, F. (2007). Estimation of pollen and seed production of common ragweed in France. Ann. Agric. Environ. Med. 14, 233–236. doi: 10.2307/23264356
Gentili, R., Asero, R., Caronni, S., Guarino, M., Montagnani, C., Mistrello, G., et al. (2019). Ambrosia artemisiifolia L. temperature-responsive traits influencing the prevalence and severity of pollinosis: a study in controlled conditions. BMC Plant Biol. 19:155. doi: 10.1186/s12870-019-1762-6
Guillemin, J. P., and Chauvel, B. (2011). Effects of the seed weight and burial depth on the seed behavior of common ragweed (Ambrosia artemisiifolia). Weed Biol. Manage. 11, 217–223. doi: 10.1111/j.1445-6664.2011.00423.x
Leiblein-Wild, M. C., Kaviani, R., and Tackenberg, O. (2014). Germination and seedling frost tolerance differ between the native and invasive range in common ragweed. Oecologia 174, 739–750. doi: 10.1007/s00442-013-2813-6
Leiblein-Wild, M. C., Steinkamp, J., Hickler, T., and Tackenberg, O. (2016). Modeling the potential distribution, net primary production and phenology of common ragweed with a physiological model. J. Biogeogr. 43, 544–554. doi: 10.1111/jbi.12646
Mačić, V., Albano, P. G., Almpanidou, V., Claudet, J., Corrales, X., Essl, F., et al. (2018). Biological invasions in conservation planning: a global systematic review. Front. Mar. Sci. 5:178. doi: 10.3389/fmars.2018.00178
Makra, L., Juhász, M., Béczi, R., and Borsos, E. (2005). The history and impacts of airborne Ambrosia (Asteraceae) pollen in Hungary. Grana 44, 57–64. doi: 10.1080/00173130510010558
Makra, L., Matyasovszky, I., Bodnár, K., and Tusnády, G. (2014). The origin and worldwide distribution of ragweed. Rev. Agric. Rural Develop. 3, 395–413.
Martínez, M. L., Vázquez, G., White, D. A., Thivet, G., and Brengues, M. (2002). Effects of burial by sand and inundation by fresh- and seawater on seed germination of five tropical beach species. Can. J. Bot. 80, 416–424. doi: 10.1139/b02-027
Montagnani, C., Gentili, R., Smith, M., Guarino, M. F., and Citterio, S. (2017). The worldwide spread, success, and impact of ragweed (Ambrosia spp.). Crit. Rev. Plant Sci. 36, 139–178. doi: 10.1080/07352689.2017.1360112
Ortmans, W. (2016). Effects of seed traits variation on seedling performance of the invasive weed, Ambrosia artemisiifolia L. Acta Oecol. 71, 39–46. doi: 10.1016/j.actao.2016.01.008
Payne, W. W. (1964). A re-evaluation of the genus Ambrosia (Compositae). J. Arnold Arbor. 65, 401–438.
Schaffner, U., Steinbach, S., Sun, Y., Skjøth, C. A., de Weger, L. A., Lommen, S. T., et al. (2020). Biological weed control to relieve millions from Ambrosia allergies in Europe. Nat. Commun. 11:1745. doi: 10.1038/s41467-020-15586-1
Strother, J. L. (2006). “Asteraceae,” in Flora of North America North of Mexico, Vol. 21. Magnoliophyta: Asteridae part 8, (New York, NY: Oxford University Press), 10–18.
Tanner, R., Branquart, E., Brundu, G., Buholzer, S., Chapman, D., Ehret, P., et al. (2017). The prioritisation of a short list of alien plants for risk analysis within the framework of the Regulation (EU) No. 1143/2014. NeoBiota. 35, 87–118. doi: 10.3897/neobiota.35.12366
Telewski, F. W., and Zeevaart, J. A. D. (2002). The 120-year period for Dr. Beal's seed viability experiment. Am. J. Bot. 89, 1285–1288. doi: 10.3732/ajb.89.8.1285
Watt, G. C. (1987). Ragweed dermatitis and the distribution of ragweed in Australia. Austr. J. Dermatol. 28, 27–29. doi: 10.1111/j.1440-0960.1987.tb00325.x
Whisenant, S. G. (2018). Common rangeland plants of west Central Texas by George Clendenin, USDA–natural resources conservation service. Great Plains Res. 28, 219–219. doi: 10.1353/gpr.2018.0043
Willemsen, R. W. (1975). Effect of stratification temperature and germination temperature on germination and the induction of secondary dormancy in common ragweed seeds. Am. J. Bot. 62, 1–5. doi: 10.1002/j.1537-2197.1975.tb12333.x
Willemsen, R. W., and Rice, E. L. (1972). Mechanism of seed dormancy in Ambrosia artemisiifolia. Am. J. Bot. 59, 248–257. doi: 10.1002/j.1537-2197.1972.tb10089.x
Yair, Y., Sibony, M., Goldberg, A., Confino-Cohen, R., Rubin, B., and Shahar, E. (2019). Ragweed species (Ambrosia spp.) in Israel: distribution and allergenicity. Aerobiologia 35, 85–95. doi: 10.1007/s10453-018-9542-6
Yair, Y., Sibony, M., and Rubin, B. (2017). Four Ambrosia species in Israel: invasive, naturalized and casual alien plants. Isr. J. Plant Sci. 64, 93–98. doi: 10.1080/07929978.2017.1288399
Keywords: Ambrosia artemisiifolia, A. confertiflora, A. tenuifolia, invasive weeds, plant biomass, plant emergence, ragweed, seed germination
Citation: Yair Y, Goldwasser Y, Sibony M, Eizenberg H and Rubin B (2020) Differential Germination and Growth Response to Temperature of Three Ambrosia Weed Species—Implications for Future Spread. Front. Agron. 2:573860. doi: 10.3389/fagro.2020.573860
Received: 18 June 2020; Accepted: 31 August 2020;
Published: 25 November 2020.
Edited by:
Mithila Jugulam, Kansas State University, United StatesReviewed by:
Roberta Masin, University of Padua, ItalySimerjeet Kaur, Punjab Agricultural University, India
Copyright © 2020 Yair, Goldwasser, Sibony, Eizenberg and Rubin. This is an open-access article distributed under the terms of the Creative Commons Attribution License (CC BY). The use, distribution or reproduction in other forums is permitted, provided the original author(s) and the copyright owner(s) are credited and that the original publication in this journal is cited, in accordance with accepted academic practice. No use, distribution or reproduction is permitted which does not comply with these terms.
*Correspondence: Baruch Rubin, cnViaW5AbWFpbC5odWppLmFjLmls