- 1Zoetis Inc, Kalamazoo, MI, United States
- 2United States Department of Agriculture (USDA) Plum Island Animal Disease Center, Agricultural Research Services, USDA, Greenport, NY, United States
- 3United States Department of Agriculture (USDA) Office of National Programs, Agricultural Research Services, Beltsville, MD, United States
Inactivated, wild-type foot-and-mouth disease virus (FMDV) vaccines are currently used to control FMD around the world. These traditional FMD vaccines are produced using large quantities of infectious, virulent, wild-type FMD viruses, with the associated risk of virus escape from manufacturing facilities or incomplete inactivation during the vaccine formulation process. While higher quality vaccines produced from wild-type FMDV are processed to reduce non-structural antigens, there is still a risk that small amounts of non-structural proteins may be present in the final product. A novel, antigenically marked FMD-LL3B3D vaccine platform under development by Zoetis, Inc. and the USDA-ARS, consists of a highly attenuated virus platform containing negative antigenic markers in the conserved non-structural proteins 3Dpol and 3B that render resultant vaccines fully DIVA compatible. This vaccine platform allows for the easy exchange of capsid coding sequences to create serotype-specific vaccines. Here we demonstrate the efficacy of the inactivated FMD-LL3B3D-A24 Cruzeiro vaccine in cattle against wild-type challenge with A24 Cruzerio. A proprietary adjuvant system was used to formulate the vaccines that conferred effective protection at low doses while maintaining the DIVA compatibility. In contrast to wild-type FMDV, the recombinant FMD-LL3B3D mutant viruses have been shown to induce no clinical signs of FMD and no shedding of virus in cattle or pigs when inoculated as a live virus. The FMD-LL3B3D vaccine platform, currently undergoing development in the US, provides opportunities for safer vaccine production with full DIVA compatibility in support of global FMDV control and eradication initiatives.
Introduction
Foot and Mouth Disease Virus (FMDV) is the causative agent of a highly contagious disease that affects pigs, cattle, sheep, goats, buffalos, and other cloven-hoofed animals. The disease causes severe production losses and disrupts a wide range of agricultural, industrial, and social activities. The FMD status of a country represents the single largest barrier to trade in the agricultural sector. Estimates of the annual economic impact of FMD in endemic countries range from $6.5 to $21 billion, while the economic impact of an FMD incursion in an FMD-free country are >$1.5 billion per year (1–3).
An incursion of FMD in North America represents the single largest risk to the agricultural sector (1, 3, 4). According to the National Pork Producers Council (5), an FMD outbreak in the United States would immediately stop all export markets for U.S. pork and beef. As the export market represents ~25% of total US pork production, the outcome would be devastating to the US pork industry. Additional follow-on impacts would also result for the corn and soybean markets and have additional negative impacts to related industries (such as food processing plants, food distribution, and restaurants). The impact to the U.S. economy over 10 years is estimated to be over $128 billion for the beef and pork sectors, $44 billion for the corn sector, and $25 billion for the soybean sector with an additional loss of ~1.5 million jobs (5, 6).
Due to the risk of research on or FMD vaccine production with wild-type, virulent FMD strains, the United States has restricted the presence of the wild-type FMD viruses to only Plum Island Animal Disease Research Center in New York. This restriction has caused the United States to be reliant on overseas production of FMD vaccines. Roth and Spickler (7) stated that the United States should “seek USDA licensure of new technology FMD vaccines that could be safely manufactured in the U.S. and which are based on a platform that allows various capsid serotypes/topotypes to be inserted into the vaccine. These would then be candidates for vendor managed inventory of finished vaccine and of vaccine antigen concentrate (VAC)”.
There are currently seven immunologically distinct serotypes of FMDV that contain 60 topotypes. These serotypes, with the exception of serotype C which has not been detected in the field since 2004, circulate in seven recognized “pools” around the globe (8). The genetic and antigenic diversity of FMDV strains results in challenges with vaccine matching for effective FMD control (9). This situation leads to the necessity to maintain specific vaccines for each region. Traditional FMD vaccines (monovalent and multivalent) are comprised of virulent, wild-type viruses chemically inactivated and formulated with adjuvants. These vaccines confer protection from clinical signs of FMD caused by FMDVs closely related to the vaccine strain. However, the traditional FMD vaccines have several challenges and limitations (10–12).
There are four major drawbacks of traditional FMD vaccines that are currently commercially available. First, large quantities of infectious, virulent FMD virus are necessary to produce vaccine antigen, with the associated risk of virus escape from manufacturing facilities or incomplete inactivation during the vaccine formulation process. Therefore, traditional inactivated FMD vaccines must be manufactured in expensive biocontainment facilities utilizing virulent FMD strains. The typical volumes of culture fluids range between 1,000–5,000 liter. The associated risk of escape from the manufacturing facilities is a key reason why many countries restrict FMD vaccine production to only local endemic strains. There have been several examples of the virulent FMD viruses escaping from manufacturing facilities and causing widespread FMD outbreaks (13–16). Second, the vaccine strain must antigenically match to the wildtype FMDV responsible for the outbreak as standard vaccines may provide little or no cross-protection against different strains even within a serotype (17). High potency (emergency use) vaccines may remediate this issue somewhat (18, 19). Third, there are challenges associated with differentiating infected from vaccinated animals (DIVA) when using traditional FMD vaccines in order to serologically discern infected animals and vaccinated animals. Small amounts of residual non-structural proteins may still be present in traditional FMD vaccines, resulting in some animals with false positive results, especially if multiple revaccinations are required due to the inherent short duration of immunity of conventional vaccines (20–26). Fourth, traditional FMD vaccines may not fully protect animals from persistent infection (10, 27–32). In a 2016 study by Stenfeldt et al. (30), it was shown that neoteric [new or temporally acute (32)] subclinical infection or persistence resulted following challenge in similar percentage of vaccinated and non-vaccinated animals (62% in vaccinated cattle, 67% in non-vaccinated cattle), indicating that vaccination with traditional vaccines has little impact on the carrier state (30, 33, 34).
In the United States, FMDV is only one of two animal pathogens on the Select Agent List (34) requiring additional security measures. Furthermore, current U.S. law (21 U.S. Code § 113A) states that no live virus of foot-and-mouth disease may be introduced for any purpose into any part of the mainland of the United States. These U.S. regulations and restrictions create challenges for FMDV research along with the discovery, development, and manufacture of FMD vaccines.
For these reasons the search for alternative vaccines has been a focus of extensive research for decades. To address some of the above limitations of traditional FMD vaccines, Zoetis Inc. and the United States Department of Agriculture—Agricultural Research Services have jointly developed a safer next generation marker FMD Vaccine platform that utilizes a proprietary adjuvant system. The vaccine platform consists of an attenuated FMD A24 Cruzeiro virus that has been modified in three ways; (1) a 543-bp deletion of the FMDV leader sequence resulting in the complete attenuation of the FMD A24 Cruzeiro virus, (2) insertion of two unique restriction enzyme sites that flank the capsid coding region to accommodate swapping capsid coding cassettes, and (3) negative antigenic markers engineered into the non-structural proteins 3B and 3Dpol (35). In this study, we describe protective immune responses in cattle and DIVA capabilities after vaccination with the novel FMD-LL3B3D A24 Cruzeiro antigen formulated with a proprietary adjuvant. This vaccine platform allows for a rapid response capability by virtue of the easy exchange of capsid coding sequences using the unique restriction sites flanking the capsid coding region (Figure 1).
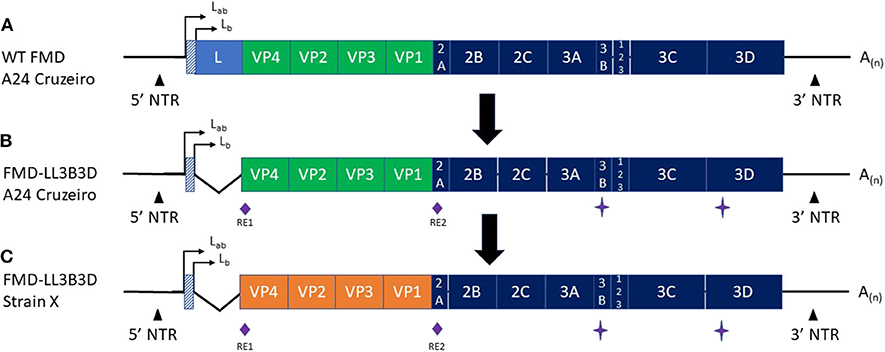
Figure 1. Graphic representation of the (A) Wild-type FMD A24 Cruzeiro, (B) FMD-LL3B3D A24 Cruzeiro, and (C) FMD-LL3B3D X genomes. The green (A,B) and orange (C) segments represent the capsid coding regions. RE1 and RE2 represent two unique restriction enzyme sites that were engineered into the genome to facilitate swapping of the capsid coding region cassettes. The star symbol represents mutations introduced into the 3B and 3D genes to negatively mark the virus rendering them fully-DIVA compatible. The angled lines (B,C) represent the 543-bp deletion of the leader gene rendering the resultant viruses fully attenuated. The orange region in (C) represents that the capsid coding region from any FMD strain may be cloned into the platform to generate a vaccine strain targeting the new strain.
In contrast to the conventional vaccines produced with wild-type FMD viruses, the recombinant FMDV-LL3B3D platform vaccine viruses are fully attenuated as they induce no clinical signs of FMD and no shedding of virus in cattle or pigs when inoculated as a live virus (35,36, Pflaum, in preparation). As a result, this vaccine platform may use existing FMD vaccine manufacturing technology without the concerns associated with current FMD vaccine production where the risk of wild-type virus escape from the manufacturing site may cause an FMD outbreak. The finished vaccine is formulated with a proprietary adjuvant system that induces robust humoral and cellular immune responses. The FMD-LL3B3D A24 Cruzeiro vaccine platform strain and a large number of capsid coding cassettes were excluded from the United States Select Agent Program regulations in April 2018 (37, 38). The FMDV-LL3B3D vaccine platform is currently under development with the goal of providing a high potency, fully DIVA compatible FMD vaccines manufactured in the United States. In this manuscript, we will discuss the preliminary vaccine safety and efficacy results and assessment of the DIVA compatibility.
Materials and Methods
FMD-LL3B3D Vaccine Virus and Cells
The FMD-LL3B3D A24 Cruzeiro (also named A24LL3BPVKV3DYR) vaccine virus was derived in baby hamster kidney cells (BHK-21) cell monolayers as previously described (35) following virus adaptation to BHK suspension cells (sBHK). The parental construct pA24Cru cDNA infectious clone, from which the FMD-LL3B3D A24 Cruzeiro strain was derived, was described by Rieder et al. (39). Suspension BHK (sBHK) cells in Celligen BLU bioreactor vessels (Eppendorf) were expanded in serum free media (Corning) with L-Glutamine and Gentamicin (Invitrogen). Parental A24 Cruzeiro FMDV challenge virus (39) was obtained from the Plum Island Animal Disease Center inventory and all uses of this select agent were in compliance with regulations detailing the requirements for possession, use, and transfer for USDA select agents mandated in 9 CFR parts 331 and 121. The cell line BHK-21 was maintained in MEM (Invitrogen) medium supplemented with 10% bovine serum (GE Healthcare), 10% Tryptose Phosphate (Teknova), 2 mM L-Glutamine (Invitrogen) and 1X Antimycotic/Antibiotic (Invitrogen).
The FMD-LL3B3D vaccine platform allows derivation of other relevant strains through the use of unique restriction enzyme sites flanking the capsid coding sequence. Standard molecular biology techniques are used to exchange the capsid coding region of the FMD-LL3B3D A24 Cruzeiro infectious plasmid with that of other FMD strains. The resultant infectious plasmids were linearized, transcribed into RNA, and then transfected into BHK-21 cells as previously described (35).
FMD-LL3B3D Antigen Production and Vaccine Formulation
Suspension BHK-21 cells were inoculated with the FMD-LL3B3D A24 Cruzeiro virus at a multiplicity of infection of 0.001 in a Celligen BLU bioreactor vessel (Eppendorf/New Brunswick) using optimal growth conditions determined previously and then virus was harvested when values of cell viability reached end points under 20%. Parameters such as pH, temperature, aeration rate and viable cell number were monitored.
The FMD-LL3B3D A24 Cruzeiro vaccine antigen was harvested from infected sBHK, clarified by filtering cell debris on successive capsule filters (Pall Corporation), concentrated on Hollow Fiber columns (GE Healthcare) and subjected to two chemical inactivation processes following the standard BEI inactivation protocol for vaccine preparation (40) using a 10 mM solution of 2-bromethylamine hydrobromide (BEA) in 0.7% NaOH. Virus was exposed to BEI at 25°C for up to 24 h per inactivation step. Neutralization of BEI was achieved by addition of 2% Sodium Thiosulfate (W/V). Inactivation kinetics were monitored by standard plaque assay in BHK-21 monolayers (data not shown). Complete inactivation of the bulk antigen was confirmed by a sterility assay in which three blind passages of undiluted material in BHK-21 cell monolayers were monitored for viral growth. For each passage, undiluted and diluted BEI-inactivated virus was used to infect BHK-21 monolayers followed by incubation for at least 72 h at 37°C. Vaccine antigens were stored in aliquots at −70°C until use. Specific concentrations of inactivated vaccine antigen were mixed with either Zoetis proprietary adjuvant or prepared as a water-in-oil-in-water (WOW) emulsion with Montanide ISA 206 (Seppic Paris) according to the manufacturer's instructions. The integrity of 146S particles and antigen concentration present in the formulated vaccines were determined by using 10–30% sucrose density gradients and 260 nm densitometry as previously described (35, 41, 42).
Preliminary Vaccine Efficacy Study
All cattle experiments were performed in the BSL3Ag FMDV research facility at the Plum Island Animal Disease Center (US Department of Agriculture, Agriculture Research Services). These studies were conducted in compliance with the Animal Welfare Act (AWA), the 2011 Guide for Care and Use of Laboratory Animals, the 2002 PHS Policy for the Humane Care and Use of Laboratory Animals, and U.S. Government Principles for Utilization and Care of Vertebrate Animals Used in Testing, Research and Training (43), as well as specific animal protocols reviewed and approved by the Institutional Animal Care and Use Committee (IACUC) of the Plum Island Animal Disease Center (USDA/APHIS/AC certificate number 21-F-0001).
Holstein steers, weighing between 250 and 300 kg were identified with ear tags and housed for a week of acclimation prior to vaccination. Seven bovines in treatment groups T02 and T03 were vaccinated with the indicated dose of BEI-inactivated FMD-LL3B3D virus formulated with either ISA 206 adjuvant (T02) or Zoetis proprietary adjuvant (T03) intramuscularly (IM) in the neck. Four bovine control animals were vaccinated with sterile phosphate-buffered saline (PBS). Immediately before and at indicated times after vaccination, blood was taken for serum analysis of FMDV-specific neutralizing antibodies. On day 21 post vaccination (dpv), all cattle were challenged with homologous, wild-type FMD A24 Cruzeiro intradermolingually (IDL) with 104 50% bovine tongue infectious doses (BTID50) according to OIE guidance. The animals were monitored at 0, 4, 7, and 10 days post-challenge (dpc) for the appearance of localized and generalized lesions. Sera and temperature were collected daily. Clinical signs were scored as 1 credit for each affected foot, and presence of vesicles in the head was not considered due to lingual inoculation of challenge. FMDV RNA was measured in sera, by rRT-PCR as previously described (35, 44).
Preliminary PD50 Study
To further investigate the protective immune responses to formulated FMD-LL3B3D A24 Cruzeiro vaccine formulated with the Zoetis proprietary adjuvant system, Holstein steers from 6 to 8 months of age were randomly assigned to one of four treatment groups (with four bovine per group): T01 received phosphate buffered saline (PBS) and served as the negative controls. Three separate vaccine dose volumes of the full dose vaccine formulated with 8 μg of hollow-fiber concentrated inactivated FMD-LL3B3D A24 Cruzeiro antigen and the Zoetis proprietary adjuvant system were applied intramuscularly (T02-Full dose = 2.0 ml, T03-1/4 dose = 0.5 ml, and T04-1/16 dose = 0.125 ml). Twenty-one days post-vaccination, all vaccinated and naïve animals were inoculated by the IDL route with 104 BTID50 of homologous, wild-type FMD A24 Cruzeiro. All cattle were followed for 3 weeks to assess development of clinical disease as expressed by fever, nasal secretion, salivation, loss of appetite and/or lameness and to examine the presence of viral RNA in probang samples.
Serology and Assessment of DIVA Compatibility
Serum samples from cattle in the naive (mock vaccinated) and vaccinated groups were tested for the presence of neutralizing antibodies against FMDV using a serum standard micro-neutralization test performed in 96-well plates (in quadruple replicas). End-point neutralizing titers were calculated as the reciprocal of the final serum dilution that neutralized 100 TCID50 of the corresponding FMDV in 50% of the wells (35, 45). The end point titer of the serum against homologous virus was calculated as the reciprocal of the last dilution of serum to neutralize 100 TCID50 in 50% of the wells (46). Serum samples at indicated time points were tested for the presence of antibodies against FMDV non-structural proteins (NSPs) using three commercially available competitive 3ABC Enzyme-Linked Imunosorbent Assay (cELISA) kits following the corresponding manufacturer's protocol. The three test kits were the PrioCHECK FMDV NS Antibody ELISA (Thermo Fisher Scientific) (47), VMRD FMDV Antibody Detection Kit (48), and the SERELISA FMDV NSP Antibody Competition ELISA (Zoetis Inc.). Values are cited as means ± Standard Deviations. Cellular immune responses were measured using a cell proliferation assay with PBMCs from both healthy naive and vaccinated steers (49).
Statistical Analysis
Statistical differences of serum neutralization comparing vaccination groups was determined by 2-way ANOVA using the Tukey's multiple comparisons test in GraphPad Prism. Statistical differences of 21-day post infection non-structural protein seroconversion was determined using the unpaired t-test in GraphPad Prism.
Results and Discussion
While traditional FMD vaccine formulations provide adequate safety and efficacy, there are still numerous challenges and gaps. Amongst these are use of virulent FMD strains, need for multiple doses, short duration of immunity, lack of prevention of persistence, and incomplete DIVA compatibility. In addition, timelines for development of traditional vaccines are not compatible with the need for rapid response to new or evolving FMD strains. To address these limitations, the USDA-ARS and Zoetis have developed the FMD-LL3B3D vaccine platform (Figure 1) that combines a safe and fully DIVA-compatible platform with rapid development of new inactivated FMD vaccines that are formulated with a proprietary adjuvant system which increases vaccine immunogenicity. The vaccine platform may be adapted to swap out the FMD capsid coding region while maintaining the safety and DIVA-compatibility capabilities.
Figure 1 depicts the generation of the FMD-LL3B3D vaccine platform from the wild-type FMD A24 Cruzeiro virus (Figures 1A,B) along with the ability to swap the capsid coding region (Figure 1C). The deletion of the leader gene renders the resultant FMD-LL3B3D vaccine viruses completely attenuated (35). Multiple FMD-LL3B3D vaccine viruses have been assessed for live virus safety in both pigs and cattle (Pflaum, in preparation). By utilizing a non-replicating, attenuated vaccine platform, events such as outbreaks associated with manufacturing facility escape (50) may be avoided. Traditional FMD manufacturers are typically limited to producing only those FMD strains that are endemic to the region. The FMD-LL3B3D vaccine platform affords the opportunity to produce global FMD vaccines from a single or a few manufacturing sites, thus improving the economics of vaccine production.
Animals that recover from natural infection are protected longer than traditionally vaccinated animals, and this is likely due to the replication of the virus in the host's cells inducing a more complete TH1 and TH2 immune response against the virus. This includes the presence of the complete repertoire of FMDV non-structural proteins, which are normally depleted in traditional vaccines or not included in recombinant FMDV vaccines. The presence of these NSPs has been shown to contain epitopes for T-cell mediated immunity, which may enhance the response to the viral infection. However, the most common assays to detect the difference between infected and vaccinated animals (DIVA) target the presence or absence of antibodies against the NSPs, making the addition of NSPs to vaccine preparation untenable. The FMD-LL3B3D vaccine platform carries mutations in the 3D polymerase (3Dpol) and 3B non-structural proteins which act as negative markers to distinguish vaccination with this platform from natural infection, thereby making the exclusion of NSP from vaccine preparations unnecessary.
The FMD-LL3B3D vaccine platform is used to generate vaccine bulk antigen targeting a wide variety of FMD strains. The bulk antigen is blended with a proprietary vaccine adjuvant system that increases the immunogenicity of the finished product. With FMD and other antigens, the adjuvant system has been demonstrated to increase the humoral and cellular immune responses, shorten the onset of immunity, and increase the duration of immunity (unpublished data).
The vaccine efficacy of the FMD-LL3B3D-based vaccine formulations was demonstrated in cattle using a laboratory-based vaccine/challenge study (Figure 2). Animals were vaccinated with a single vaccine dose on day 0 and challenged via the intradermal lingual route with virulent, wild-type FMD on day 21. Clinical observations and clinical samples (serum and swabs) were taken over the next 14 days. Probang samples were taken from day 38 through day 52 to assess persistence of the challenge virus.
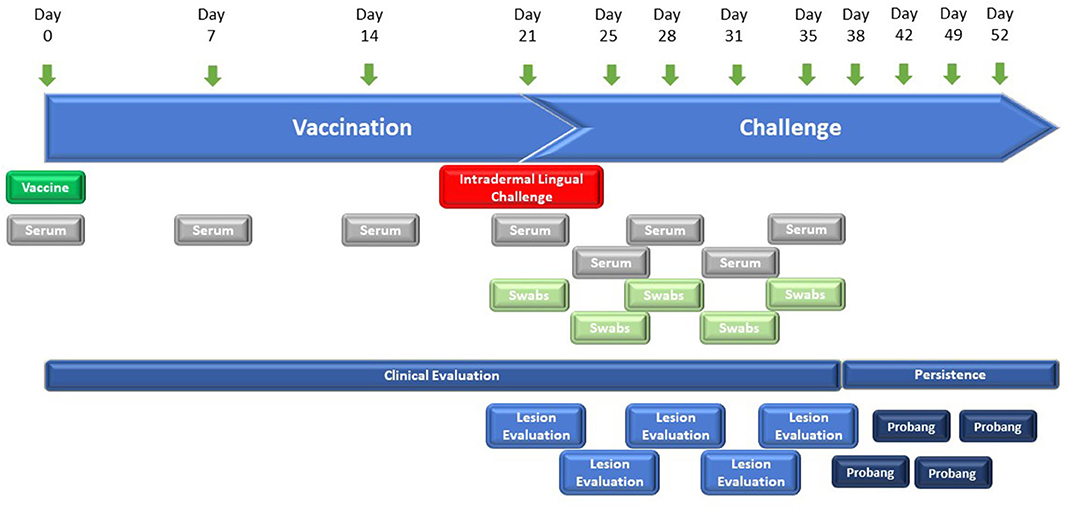
Figure 2. Graphic representation of the study designs for FMD-LL3B3D-based vaccine efficacy studies. Six–twelve-month-old steers were vaccinated on day 0 with the challenge occurring on day 21 where animals are intradermal-lingually challenged with 10,000 BTID50 of virulent, wild-type FMD virus. Serum, tonsillar swabs, and clinical observations were taken at the indicated days.
The serological and cellular immune responses to vaccination with a monovalent FMD-LL3B3D A24 Cruzeiro vaccine are shown on Figure 3. The serum neutralizing titer generated in response to vaccination with the FMD-LL3B3D A24 Cruzeiro vaccine formulated with the proprietary adjuvant system were statistically significantly higher than that generated using traditional commercial adjuvant at days 7, 14, and 21 (Figure 3A). In addition, the lymphocyte proliferation index, which represents the cellular immune response, were numerically increased in animals vaccinated using the proprietary adjuvant system vs. commercial-type adjuvant (Figure 3B).
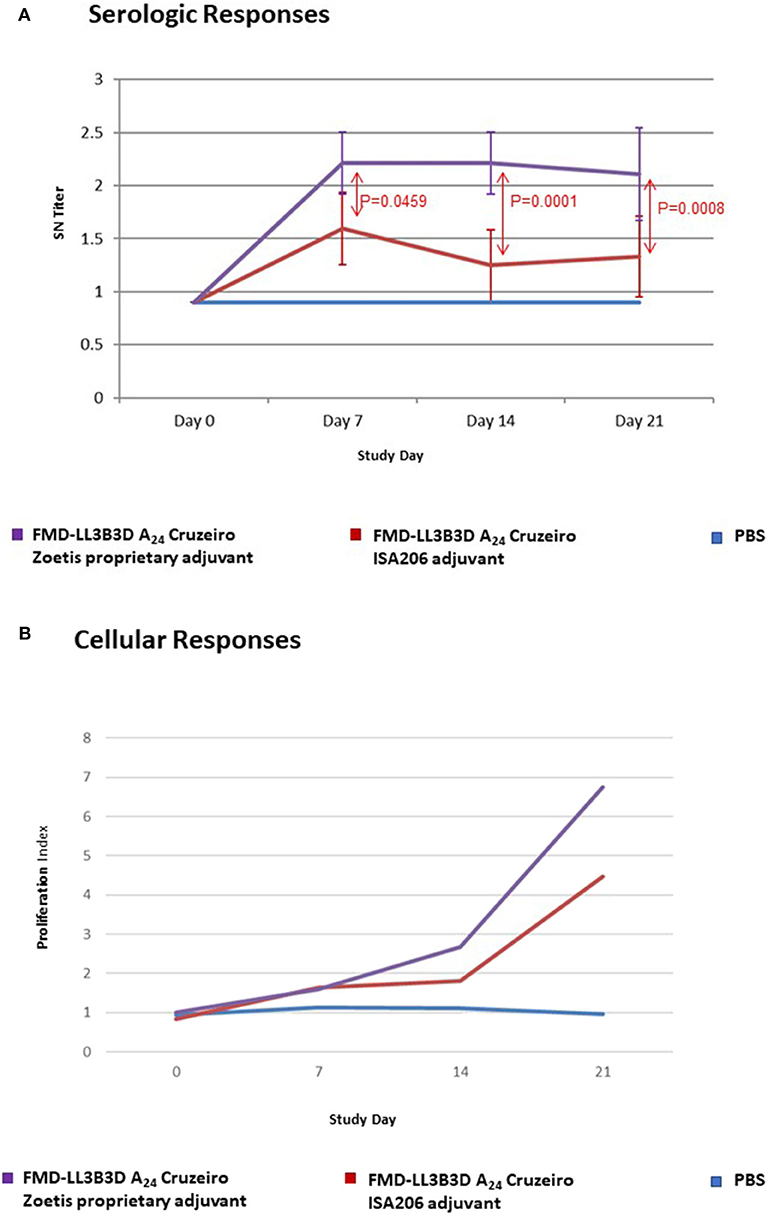
Figure 3. Immune responses of animals vaccinated in a preliminary vaccine efficacy study using FMD-LL3B3D A24 Cruzeiro antigen and a proprietary adjuvant system. (A) Serum neutralizing titers were measured for each vaccine group pre- and post- vaccination. FMD-LL3B3D-A24 vaccination induced dose-dependent levels of neutralizing antibodies. (B) Cellular immune responses were measured using cell proliferation assay (on PBMC from both healthy naive and vaccinated steers).
Following virulent challenge on day 21, clinical observations (temperature and clinical signs) were taken through day 31. Challenged animals that had been vaccinated with either PBS or commercial-type vaccine had elevated temperatures 1–4 days post challenge while animals vaccinated with the FMD-LL3B3D A24 Cruzeiro vaccine demonstrated no temperature elevations (Figure 4A). Cattle vaccinated with PBS began to show lesions consistent with FMD on their hooves and mouth starting at 3-days post challenge (Figure 4B). By day 10 post-challenge, all control animals had clinical score codes of 4. In contrast, animals vaccinated with either the commercial-type FMD A24 Cruzeiro vaccine or with the FMD-LL3B3D A24 Cruzeiro vaccine formulation did not show any lesions consistent with FMD.
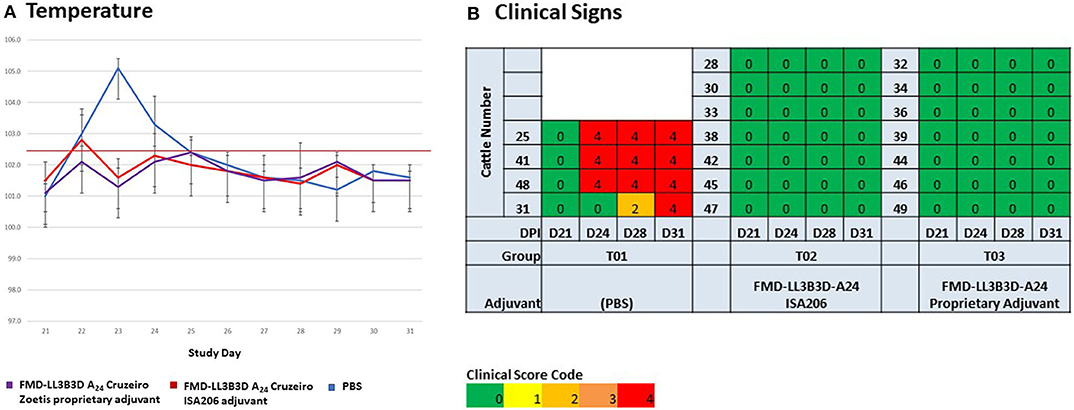
Figure 4. Clinical observations of animals vaccinated and challenged with virulent, wild-type FMD A24 Cruzeiro in an adjuvant selection study. All animals were vaccinated on day 0 and challenged on day 21. (A) Temperature was assessed on days 21 through 31. (B) Clinical observations were taken at days 21, 24, 28, and 31.
To determine the potency of the FMD-LL3B3D A24 Cruzeiro vaccine, we carried out an experiment in cattle, using full-dose, one-quarter dose, and one-sixteenth dose (2, 0.5, and 0.125 ml, respectively) of FMD-LL3B3D A24 Cruzeiro vaccine and challenged at 21 days post vaccination. Cattle in all three vaccinated groups challenged with virulent FMD A24 Cruzeiro were fully protected from both viremia (Figure 5A) and clinical signs of FMD (Figure 5B). In contrast, cattle vaccinated with PBS demonstrated a peak of viremia 3-days post challenge (Figure 5A) along with onset of lesions (Figure 5B). By day-6 post challenge, all four cattle vaccinated with PBS had clinical scores of 4 indicating that all four hooves had lesions.
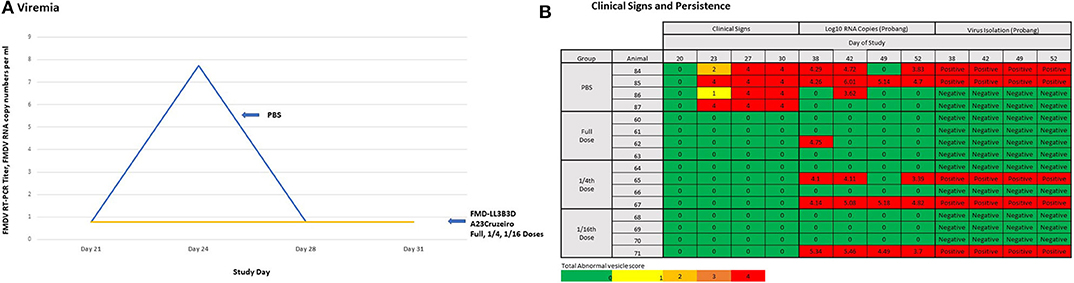
Figure 5. Preliminary Protective Dose 50 (PD50) study for the FMD-LL3B3D A24 Cruzeiro vaccine. Animals were vaccinated on day 0 with either a full vaccine dose of FMD-LL3B3D A24 Cruzeiro vaccine with a proprietary adjuvant, a quarter of a dose, or a sixteenth of a dose. All animals were then challenged on day 21. (A) Viremia was assessed by RT-PCR of serum samples on days 21, 24, 28, and 31. (B) Clinical observations were assessed on days 20, 23, 27, and 30 while challenge virus persistence was assessed by either viral isolation or RT-PCR of probang samples on days 38, 42, 49, and 52.
Persistent infection of the challenged virus following vaccination was assessed via probang samples taken 17-, 21-, 28-, and 31-days post challenge. Probang samples were tested for the presence of FMD A24 Cruzeiro by RT-PCR and virus isolation. With the exception of one RT-PCR positive sample, all probang samples from cattle vaccinated with the full-dose were RT-PCR negative for FMDV and all probang samples were negative for virus isolation (Figure 5B). Two of the four cattle vaccinated with the one-quarter dose were negative by both RT-PCR and by virus isolation (Figure 5B). Three of the four cattle vaccinated with the one-sixteenth dose were negative by both RT-PCR and by virus isolation (Figure 5B). Based on these results, we can only conclude that the full-dose FMD-LL3B3D A24 Cruzeiro vaccine contained in excess of 16 PD50 per dose.
Because traditional FMD vaccines are produced from wild-type FMDV, the bulk antigen must be purified to deplete the non-structural proteins present in the supernatant. This process is not 100% effective as there may still be some non-structural proteins present inside the intact viral particles. As such, vaccinated animals may still react to these trace non-structural proteins and present as positive results when tested by the available DIVA assays (20–26). In contrast, there is no need to deplete the non-structural proteins in the FMD-LL3B3D vaccine platform as the 3B coding region (encoding the non-structural protein) contains mutations which correspond to the epitope of the monoclonal antibodies used in the available commercial FMD DIVA diagnostic assays, thus rendering the FMD-LL3B3D vaccinated animals non-responsive (Figure 1) (35). Animals that were vaccinated with the full dose of FMD-LL3B3D A24 Cruzeiro vaccine were demonstrated to have reactivities similar to that of animals vaccinated with PBS in three different commercially available FMD DIVA diagnostic tests (Figure 6). This DIVA compatibility may facilitate a “vaccinate to live” policy instead of a “vaccinate to cull” as confidence in the immunologic status of the animals may be vastly increased.
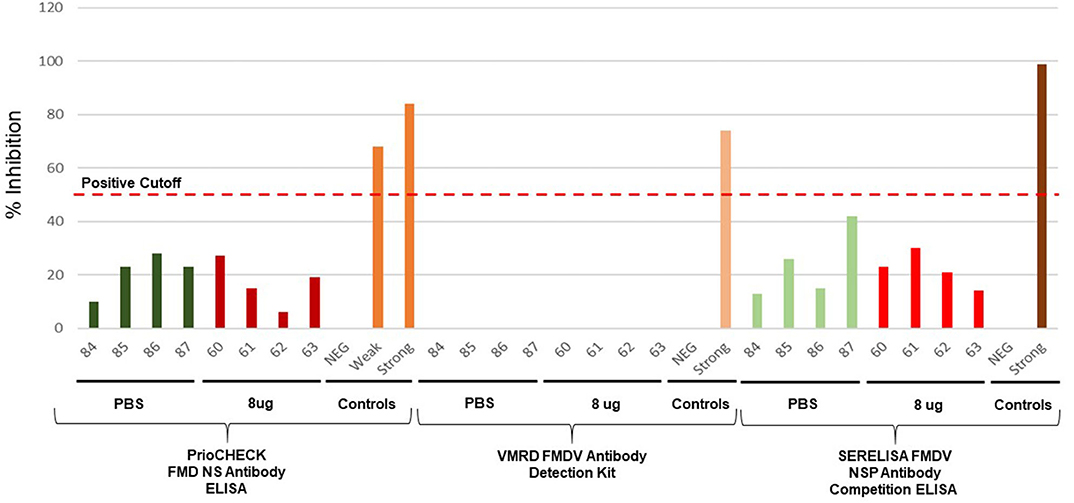
Figure 6. Assessment of DIVA Diagnostic compatibility of animals vaccinated with FMD-LL3B3D A24 Cruzeiro vaccine using the PrioCHECK FMDV NS Antibody ELISA (Thermo Fisher Scientific), Foot and Mouth Disease Virus Antibody Detection Kit cELISA (VMRD), or SERELISA® FMDV NSP Ab Competition (Zoetis).
In response to an outbreak of a novel FMD strain or incursion of a FMD strain that is not adequately covered by existing FMD vaccine banks, the FMD-LL3B3D vaccine platform is able to be utilized to rapidly generate new vaccine. A shuttle plasmid has been generated in E. coli in which the capsid coding region of the FMD-LL3B3D vaccine platform genome has been replace with the G-luc gene (Figure 7). This shuttle plasmid is the starting place for the rapid response capability. Upon obtaining a novel FMD strain, the capsid coding region is sequenced, and the capsid coding region is synthesized with the novel restriction sites flanking the capsid coding region. Traditional molecular biological techniques are utilized to clone the capsid coding region into the shuttle plasmid to generate a full-length plasmid construct. Following transcription, the full-length RNA is transfected into a manufacturing cell line to generate the new vaccine strain from which Premaster and Master Seeds may be derived. In this way, incursion of a new FMD strain into a FMD-free country may be initially addressed with the nearest matching vaccine and followed promptly with the specific FMD strain vaccine.
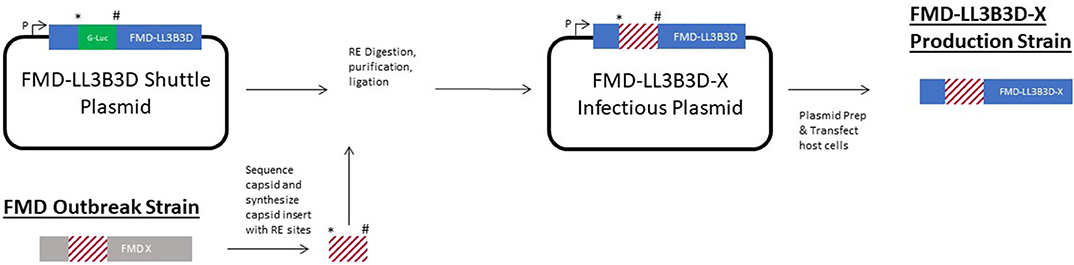
Figure 7. Graphic representation of the rapid response capability of the FMD-LL3B3D vaccine platform. The * and # symbols represent two unique restriction enzyme sites that were engineered into the genome to facilitate swapping of the capsid coding region cassettes.
Cattle immunized with a variety of chemically inactivated FMD-LL3B3D vaccine constructs were protected from challenge with parental virus (Figures 2–5). Three commercially available FMD DIVA companion assays were shown to be compatible with the negative markers built into the FMD-LL3B3D vaccine platform and facilitate the full DIVA capability (Figure 6). Taken together, the vaccine formulations containing FMD-LL3B3D-based antigens represent an improved product profile that addresses the limitations of existing FMD vaccines and create a rapid response capability that may be utilized to promptly address incursions of new FMDV serotypes (Figure 7). This new platform technology with high potency, safe antigen production, full DIVA compatibility, and single-dose application may revolutionize the FMD vaccine market and may provide a product profile in line with National efforts to eradicate FMD.
Data Availability Statement
The raw data supporting the conclusions of this article will be made available by the authors, without undue reservation.
Ethics Statement
Live animals used in these studies were owned by the United States Department of Agriculture—Agricultural Research Service and the animal experiments were performed under protocols approved by the Institutional Animal Care and Use Committee of the Plum Island Animal Disease Center.
Author Contributions
The studies were designed, directed, and coordinated by JH, ER, and LR. JH, ER, and LR provided conceptual and technical guidance for all aspects of the project. PK adapted virus to sBHK, generated preMaster seeds, determined the antigen content, and with PD formulated the vaccine. All animal experiments were conducted at the PIADC, USDA ARS BSL-3 animal facility. JP participated on the planning and conducted the animal experiment and with PK, VM, JT, ER, LR, and JH collected and analyzed the data. The manuscript was written by JH and ER with contributions and comments of all authors.
Conflict of Interest
JH, JT, PD, and VM are employed by Zoetis Inc.
The remaining authors declare that the research was conducted in the absence of any commercial or financial relationships that could be construed as a potential conflict of interest.
Acknowledgments
The following individuals contributed to the scientific content of this manuscript: Ignacio Correas (Zoetis Inc, Kalamazoo, MI, US), Murray Cameron (Zoetis Inc, Tullamore, Ireland), Sandra Dixon (Zoetis Inc, Kalamazoo, MI, US), Shelby Gould (Zoetis Inc, Kalamazoo, MI, US), Vickie King (Zoetis Inc, Kalamazoo, MI, US), Adam Lewis (Zoetis Inc, Kalamazoo, MI, US), Stephen Lyle (Zoetis Inc, Kalamazoo, MI, US), Nathalie Martinon (Zoetis Inc, Kalamazoo, MI, US), Nancee Oien (Zoetis Inc, Kalamazoo, MI, US), Wanda Isaacson (Zoetis Inc, Lincoln, NE, US), Ray Oçonnor (Zoetis Inc, Parsippany, NJ, US), Dusty Brown (Zoetis Inc, Parsippany, NJ, US), Katherine Pflaum (USDA Plum Island Animal Disease Center, Animal Research Services, USDA, Greeenport NY, US) and Michael Oldakowski (USDA Plum Island Animal Disease Center, Animal Research Services, USDA, Greeenport NY, US), Elizabeth Schafer (USDA Plum Island Animal Disease Center, Animal Research Services, USDA, Greeenport NY, US), Jonatan Arzt (USDA Plum Island Animal Disease Center, Animal Research Services, USDA, Greeenport NY, US).
References
1. Knight-Jones TJD, Rushton J. The economic impacts of foot and mouth disease – What are they, how big are they and where do they occur? Prev Vet Med. (2013) 112:161–73. doi: 10.1016/j.prevetmed.2013.07.013
2. Knight-Jones TJD, McLaws M, Rushton J. Foot-and-mouth disease impact on smallholders – what do we know, what don't we know and how can we find out more? Transbound Emerg Dis. (2017) 64:1079–94. doi: 10.1111/tbed.12507
3. Brown VR, Bevins SN. Potential role of wildlife in the USA in the event of a foot-and-mouth disease virus incursion. Vet Rec. (2019) 184:741. doi: 10.1136/vr.104895
4. Arzt J, White WR, Thomsen BV, Brown CC. Agricultural diseases on the move early in the third millennium. Vet Pathol. (2010) 47:15–27. doi: 10.1177/0300985809354350
5. National Pork Producers Council. Foot and Mouth Disease. (2020). Available online at: http://www.nppc.org (accessed April 18, 2020).
6. Lee B, Park J, Gordon P, Moore J, Richardson H. Estimating the state-by-state economic impacts of a foot-and-mouth disease attack. Int Reg Sci Rev. (2012) 35:26–47. doi: 10.1177/0160017610390939
7. Roth JA, Spickler AR. FMD Vaccine Surge Capacity for Emergency Use in the United States. Veterinary Microbiology and Preventive Medicine Reports (2014). p. 8. Available online at: https://lib.dr.iastate.edu/vmpm_reports/8 (accessed April 18, 2020).
8. World Reference Laboratory for Foot and Mouth Disease. Foot and Mouth Disease Occurrence. (2020). Available online at: https://www.wrlfmd.org/foot-and-mouth-disease/occurrence (accessed August 12, 2020).
9. Knowles NJ, Samuel AR. Molecular epidemiology of foot-and-mouth disease virus. Virus Res. (2003) 91:65–80. doi: 10.1016/S0168-1702(02)00260-5
10. Park JH, Lee KN, Ko YJ, Kim SM, Lee HS, Park JY, et al. Diagnosis and control measures of the 2010 outbreak of foot-and-mouth disease A type in the Republic of Korea. Transbound Emerg Dis. (2013) 60:188–92. doi: 10.1111/j.1865-1682.2012.01333.x
11. Robinson L, Knight-Jones TJ, Charleston B, Rodriguez LL, Gay CG, Sumption KJ, et al. Global foot-and-mouth disease research update and gap analysis: 7 - pathogenesis and molecular biology. Transbound Emerg Dis. (2016) 63(Suppl. 1):63–71. doi: 10.1111/tbed.12520
12. Kamel M, El-Sayed A, Castañeda Vazquez H. Foot-and-mouth disease vaccines: recent updates and future perspectives. Arch Virol. (2019) 164:1501–13. doi: 10.1007/s00705-019-04216-x
13. King AM, Underwood BO, McCahon D, Newman JW, Brown F. Biochemical identification of viruses causing the 1981 outbreaks of foot and mouth disease in the UK. Nature. (1981) 293:479–80. doi: 10.1038/293479a0
14. Bachrach HL. Foot-and-mouth disease and its antigens. Adv Exp Med Biol. (1985) 185:27–46. doi: 10.1007/978-1-4684-7974-4_2
15. Beck E, Strohmaier K. Subtyping of European foot-and-mouth disease virus strains by nucleotide sequence determination. J Virol. (1987) 61:1621–9. doi: 10.1128/JVI.61.5.1621-1629.1987
16. Cottam EM, Wadsworth J, Shaw AE, Rowlands RJ, Goatley L, Maan S, et al. Transmission pathways of foot-and-mouth disease virus in the United Kingdom in 2007. PLoS Pathog. (2008) 4:e1000050. doi: 10.1371/journal.ppat.1000050
17. Hyslop NSG, Davie J, Carter SP. Antigenic differences between strains of foot-and-mouth disease virus of type SAT 1. J Hyg. (1963) 61:217–30. doi: 10.1017/S0022172400020933
18. Brehm KE, Kumar N, Thulke H-H, Haas B. High potency vaccines induce protection against heterologous challenge with foot-and-mouth-disease virus. Vaccine. (2008) 26:1681–7. doi: 10.1016/j.vaccine.2008.01.038
19. Goris N, Maradei E, D'Aloia R, Fondevila N, Mattion NA, Perez A, et al. Foot-and-mouth disease vaccine potency testing in cattle using homologous and heterologous challenge strains: precision of the “Protection against Podal Generalisation” test. Vaccine. (2008) 26:3432–7. doi: 10.1016/j.vaccine.2008.04.034
20. Hedger RS, Barnett IT, Gradwell DV, Travassos, Dias P. Serological tests for foot-and-mouth disease in bovine serum samples. Problems of interpretation. Rev Sci Tech. (1982) 1:387–93. doi: 10.20506/rst.1.2.75
21. Mackay DK, Forsyth MA, Davies PR, Berlinzani A, Belsham GJ, Flint M, et al. Differentiating infection from vaccination in foot and mouth disease using a panel of recombinant non-structural proteins in ELISA. Vaccine. (1998) 16:446–59. doi: 10.1016/S0264-410X(97)00227-2
22. Bergmann IE, Malirat V, Neitzert E, Beck E, Panizzutti N, Sanchez C, et al. Improvement of a serodiagnostic strategy for foot and mouth disease virus surveillance in cattle under systematic vaccination: a combined system of an indirect ELISA 3ABC with an enzyme linked immunoelectrotransfer blot assay. Arch Virol. (2000) 145:473–89. doi: 10.1007/s007050050040
23. Lee F, Jong M, Yang D. Presence of antibodies to nonstructural proteins of foot-and-mouth disease virus in repeatedly vaccinated cattle. Vet Microbiol. (2006) 115:14–20. doi: 10.1016/j.vetmic.2005.12.017
24. Robiolo B, Seki C, Fondevilla N, Grigera P, Scodeller E, Periolo O, et al. Analysis of the immune response to FMDV structural and non-structural proteins in cattle in Argentina by the combined use of liquid phase and 3ABC-ELISA tests. Vaccine. (2006) 24:997–1008. doi: 10.1016/j.vaccine.2005.08.071
25. Mohapatra JK, Pandey LK, Sanyal A, Pattnaik B. Recombinant non-structural polyprotein 3AB-based serodiagnostic strategy for FMD surveillance in bovines irrespective of vaccination. J Virol Methods. (2011) 177:184–92. doi: 10.1016/j.jviromet.2011.08.006
26. Behura M, Mohapatra JK, Pandey LK, Das B, Bhatt M, Subramaniam S, et al. The carboxy-terminal half of nonstructural protein 3A is not essential for foot-and-mouth disease virus replication in cultured cell lines. Arch Virol. (2016) 161:1295–305. doi: 10.1007/s00705-016-2805-z
27. Alexandersen S, Zhang Z, Donaldson AI. Aspects of the persistence of foot-and-mouth disease virus in animals – the carrier problem. Microbes Infect. (2002) 4:1099–110. doi: 10.1016/S1286-4579(02)01634-9
28. Bergmann IE, Malirat V, Neitzert E. Non-capsid proteins to identify foot-and-mouth disease viral circulation in cattle irrespective of vaccination. Biologicals. (2005) 33:235–9. doi: 10.1016/j.biologicals.2005.08.013
29. Parthiban AB, Mahapatra M, Gubbins S, Parida S. Virus excretion from foot-and-mouth disease virus carrier cattle and their potential role in causing new outbreaks. PLoS ONE. (2015) 10:e0128815. doi: 10.1371/journal.pone.0128815
30. Stenfeldt C, Eschbaumer M, Rekant SI, Pacheco JM, Smoliga GR, Hartwig EJ, et al. The foot-and-mouth disease carrier state divergence in cattle. J Virol. (2016) 90:6344–64. doi: 10.1128/JVI.00388-16
31. Farooq U, Ahmed Z, Naeem K, Bertram M, Brito B, Stenfeldt C, et al. Characterization of naturally occurring, new and persistent subclinical foot-and-mouth disease virus infection in vaccinated Asian buffalo in Islamabad Capital Territory, Pakistan. Transbound Emerg Dis. (2018) 65:1836–50. doi: 10.1111/tbed.12963
32. Stenfeldt C, Arzt J. The carrier conundrum; A review of recent advances and persistent gaps regarding the carrier state of foot-and-mouth disease virus. Pathogens. (2020) 9:3. doi: 10.3390/pathogens9030167
33. Salt JS. The carrier state in foot and mouth disease–an immunological review. Br Vet J. (1993) 149:207–23. doi: 10.1016/S0007-1935(05)80168-X
34. Sutmoller P, Casas OR. Unapparent foot and mouth disease infection (sub-clinical infections and carriers): implications for control. Rev Sci Tech. (2002) 21:519–29. doi: 10.20506/rst.21.3.1366
35. Uddowla S, Hollister J, Pacheco JM, Rodriguez LL, Rieder E. A safe foot-and-mouth disease vaccine platform with two negative markers for differentiating infected from vaccinated animals. J Virol. (2012) 86:11675–85. doi: 10.1128/JVI.01254-12
36. Eschbaumer M, Dill V, Carlson JC, Arzt J, Stenfeldt C, Krug P, et al. Foot-and-mouth disease virus lacking the leader 2 protein and containing two negative DIVA markers 3 (FMDV LL3B3D A24) is fully attenuated in pigs. Pathogens. (2020) 17:1–8. doi: 10.3390/pathogens9020129
37. Federal Select Agent Program. Foot and Mouth Disease. (2020). Available online at: https://www.selectagents.gov/exclusions-usda.html#footmouth (accessed April 18, 2020).
38. GovDelivery (2020). Available online at: https://content.govdelivery.com/accounts/USDAAPHIS/bulletins/1ec50cb?reqfrom=share) (accessed April 20, 2020).
39. Rieder E, Henry T, Duque H, Baxt B. Analysis of a foot-and-mouth disease virus type A24 isolate containing an SGD receptor recognition site in vitro and its pathogenesis in cattle. J Virol. (2005) 79:12989–98. doi: 10.1128/JVI.79.20.12989-12998.2005
40. Bahnemann HG. Binary ethyleneimine as an inactivant for foot-and-mouth disease and its application for virus production. Arch Virol. (1975) 47:47–56. doi: 10.1007/BF01315592
41. Barteling SJ, Meloen RH. A simple method for the quantification of 140S particles of foot-and-mouth disease virus (FMDV). Arch Ges Virusforsh. (1974) 45:362–4. doi: 10.1007/BF01242879
42. Knipe T, Rieder E, Baxt B, Ward G, Mason PW. Characterization of synthetic foot-and-mouth disease virus provirions separates acid-mediated disassembly from infectivity. J Virol. (1997) 71:2851–6. doi: 10.1128/JVI.71.4.2851-2856.1997
43. IRAC. Federal Register. Vol. 50. Office of Science and Technology Policy [FR Doc. 85-12059] (1985).
44. Pacheco J, Arzt J, Rodriguez LL. Early events in the pathogenesis of foot-and-mouth disease in cattle after controlled aerosol exposure. Vet J. (2010) 183:46–53. doi: 10.1016/j.tvjl.2008.08.023
45. OIE Terrestrial Manual. Manual of Diagnostic Tests and Vaccines for Terrestrial Animals. (2017). Available online at: http://www.oie.int/fileadmin/Home/eng/Health_standards/tahm/2.01.08_FMD.pdf
46. Rweyemamu MM, Booth JC, Head M, Pay TW. Microneutralization tests for serological typing and subtyping of foot-and-mouth disease virus strains. J Hyg. (1978) 81:107–23. doi: 10.1017/S002217240005381X
47. Sørensen KJ, Madsen KG, Madsen ES, Salt JS, Nqindi J, Mackay DK. Differentiation of infection from vaccination in foot-and-mouth disease by the detection of antibodies to the non-structural proteins 3D, 3AB and 3ABC in ELISA using antigens expressed in baculovirus. Arch Virol. (1998) 143:1461–76. doi: 10.1007/s007050050390
48. Chung CJ, Clavijo A, Bounpheng MA, Uddowla S, Sayed A, Dancho B, et al. An improved, rapid competitive ELISA using a novel conserved 3B epitope for the detection of serum antibodies to foot-and-mouth disease virus. J Vet Diagn Invest. (2018) 30:699–707. doi: 10.1177/1040638718779641
49. Toka FN, Kenney MA, Golde WT. Rapid and Transient Activation of γδ T Cells to IFN-γ Production, NK Cell-Like Killing, and Antigen Processing during Acute Virus Infection. J Immunol. (2011) 186:4853–61. doi: 10.4049/jimmunol.1003599
Keywords: FMD (foot and mouth disease), DIVA, potency, efficacy, diagnostic, vaccine platform, rapid, effective
Citation: Hardham JM, Krug P, Pacheco JM, Thompson J, Dominowski P, Moulin V, Gay CG, Rodriguez LL and Rieder E (2020) Novel Foot-and-Mouth Disease Vaccine Platform: Formulations for Safe and DIVA-Compatible FMD Vaccines With Improved Potency. Front. Vet. Sci. 7:554305. doi: 10.3389/fvets.2020.554305
Received: 21 April 2020; Accepted: 13 August 2020;
Published: 25 September 2020.
Edited by:
Armanda Bastos, University of Pretoria, South AfricaReviewed by:
Francois Frederick Maree, Agricultural Research Council of South Africa (ARC-SA), South AfricaJong-Hyeon Park, Animal and Plant Quarantine Agency, South Korea
Copyright © 2020 Hardham, Krug, Pacheco, Thompson, Dominowski, Moulin, Gay, Rodriguez and Rieder. This is an open-access article distributed under the terms of the Creative Commons Attribution License (CC BY). The use, distribution or reproduction in other forums is permitted, provided the original author(s) and the copyright owner(s) are credited and that the original publication in this journal is cited, in accordance with accepted academic practice. No use, distribution or reproduction is permitted which does not comply with these terms.
*Correspondence: John M. Hardham, john.m.hardham@zoetis.com; Elizabeth Rieder, elizabeth.rieder@usda.gov
†Co-lead authors