- National Animal Disease Center, Agricultural Research Service (ARS), United States Department of Agriculture (USDA), Ames, IA, United States
Bovine leukemia virus (BLV) affects the health and productivity of cattle. The virus causes abnormal immune function and immunosuppression. MicroRNAs (miRNAs) are involved in gene expression, having been associated with stress and immune response, tumor growth, and viral infection. The objective of this study was to determine the expression of circulating miRNAs produced by BLV in animals exposed to the virus. Sera from 14 animals were collected to establish IgG reactivity to BLV by ELISA, where seven animals were seropositive and seven were seronegative for BLV exposure. White blood cells (WBC) from each animal were also collected and miRNAs were identified by sequencing from sera and WBC. The seropositive group had higher counts of BLV miRNAs when compared to seronegative group in sera and WBC. Blv-miR-1-3p, blv-miR-B2-5p, blv-miR-B4-3p, and blv-miR-B5-5p were statistically significant (P < 0.00001) in serum with an average of 7 log2 fold difference between seropositive and seronegative groups. Blv-miR-B1-3p, blv-miR-B1-5p, blv-miR-B3, blv-miR-B4-3p, blv-miR-B4-5p, blv-miR-B5-5p were statistically significant (P < 1.08e−9) in WBC with an average of 7 log2 fold difference between the seropositive and the seronegative groups. Blv-miR-B2-3p and blv-miR-B2-5p were also statistically significant in WBC (P < 2.79e-17), with an average of 27 log2 fold difference between the seropositive and the seronegative groups. There were 18 genes identified as being potential targets for blv-miR-B1-5p, and 3 genes for blv-miR-B4-5p. Gene ontology analysis indicated that the target genes are mainly involved in the response to stress and in the immune system process. Several of the identified genes have been associated with leukemia development in humans and cattle. Differential expression of genes targeted by BLV miRNAs should be evaluated to determine their effect in BLV replication.
Introduction
Bovine Leukemia virus (BLV) belongs to the Retroviridae family, produces enzootic bovine leukosis, and is associated with subclinical infections in cattle (1–3). The disease has been characterized as having three stages: asymptomatic or aleukemic, persistent lymphocytosis, and leukemia or lymphoma (3, 4). Approximately 83% of the cows in dairy herds, and 39% of cattle at slaughter are infected with BLV (2, 3). No commercial vaccine is available and control of the virus is by culling positive animals (3). Infection with BLV has been associated with higher culling rates and shorter herd life, contributing to the cost of the production system. It has been estimated that the annual cost of subclinical BLV infection is more than $6,400, with a 50% prevalence in a 100 herd of cows in the mid-Atlantic region (1).
MicroRNAs (miRNAs) are small non-coding RNA molecules ranging in size from 21 to 25 nucleotides in length, which regulate gene expression by altering translation (5–7). Host miRNAs have been proposed as biomarkers for exposure to pathogens in humans (8, 9), and in livestock (10–12). Cattle exposed to different pathogens has shown differential expression of various miRNAs (13–15).
MiRNAs have been identified in what was thought to be an inactive region of the BLV genome (16, 17), and their activity in developing tumoral cells has also been identified (18–21). However, the expression of BLV miRNAs has not been established; therefore, the objective of this study was to determine the expression of circulating miRNAs produced by BLV in animals exposed to the virus.
Materials and Methods
Animals and laboratory techniques have been previously described (22). Following is a brief description of the procedures used.
Animals
Fourteen Holstein female cattle were used to collect samples at the National Animal Disease Center, in Ames, IA, United States. All animals included in the study were considered healthy according to the attending veterinarian. Samples came from 3 heifers and 11 cows with at least one calving and at mid-lactation. Jugular venipuncture was used to collect blood samples in PAXgene tubes (PreAnalytiX GmbH, Hombrechtikon, Zurich, Switzerland), from which white blood cells (WBCs) were obtained. Samples were kept at room temperature for 2 h and refrigerated (4°C) before centrifugation (3,000 g for 10 min) to collect serum and WBC. Management of animals was according to the protocol approved by the Institutional Animal Care and Use Committee of the National Animal Disease Center, in Ames, IA, United States.
ELISA
An additional blood sample was collected via jugular venipuncture in serum separator vacutainer tubes (SST, TM BD, Franklin Lakes, NJ, United States). Tubes were incubated (37°C for 30 min) and centrifuged (1,250 g for 30 min). Sera were stored at −80°C until processed. Sera were used to determine IgG response to BLV, using the IDEXX Leukosis Serum X2 Ab kit (Idexx Laboratories, Westbrook, ME, United States). Samples were determined positive according to manufacturer's directions. A positive was established if the sample to positive ratio (S/P) was >115%. The sample to positive ratio was the ratio of the difference between the optical density 450 nm of the sample minus the optical density 450 nm of the negative control, divided by the difference between the optical density 450 nm of the mean positive control minus the optical density 450 nm of the negative control. Seven animals were seronegative (negative group), and seven were seropositive (positive group).
Small Non-coding RNA Isolation
Total RNA was extracted from serum and WBC samples using the MagMAXTM mirVanaTM Total RNA Isolation Kit (Life Technologies, Carlsbad, CA, United States) and total RNA was eluted in 100 μL of RNase-free water. The concentration and quality of small RNAs was determined using an Agilent 2100 Bioanalyzer Small RNA chip (Agilent Technologies, Santa Clara, CA, United States).
Library Preparation and Sequencing
Six microliters (6 μL) of small RNA from each sample was used to prepare each library using the NEBNext Multiplex Small RNA Library Prep Kit (New England BioLabs, Ipswich, MA, United States) and 14 indexed primers, which gave all samples a unique identifier. Concentration of libraries and purification was done using the QIAquick PCR purification kit (QIAGEN, Germantown, MD, United States). Each library was loaded on an Agilent 2100 Bioanalyzer High Sensitivity DNA chip (Agilent Technologies, Santa Clara, CA, United States) to establish quality and quantity of each library between 135 and 170 base pairs. Thirty nanogram of each library was pooled (14 libraries in the pool) and size selected using AMPure XP beads (Beckman Coulter, Indianapolis, IN, United States). After selecting for size, the pools were concentrated using the QIAquickPCR purification kit (QIAGEN, Germantown, MD, United States) and eluted in RNase-free water. To determine the concentration of each pool, an Agilent 2100 Bioanalyzer High Sensitivity DNA chip (Agilent Technologies, Santa Clara, CA, United States) was used. Each pool was stored at −20°C until sequencing. The pool was sequenced as single-end 50 base pair reads using the Illumina HiSeq 3000 System (Illumina, San Diego, CA, United States).
Data Analysis
The BLV reference genome (GenBank assembly accession: GCA_000853665.1) was downloaded from NCBI (ViralProj14916). The BLV miRNA precursor and mature sequences were downloaded from miRBase (Release 22.1). The quality of raw sequencing reads were evaluated by FastQC (23), and were cleaned with cutadapt (24). The raw and normalized BLV miRNA reads were counted by using miRDeep2 (version 2.0.0.8) (25). The counted BLV miRNA raw reads were then used to identify differentially expressed miRNAs by running DESEQ2 package (26). Target genes of the differentially expressed miRNAs were separately predicted by miRanda (Total score ≥450 and max energy ≤-20 kcal/Mol) and PITA (Energetic score ≤-20 kcal/Mol) (27, 28). Gene ontology of the target genes were analyzed by using OmicsBox (www.biobam.com). Sequences are available on the NCBI SRA under BioProject accession number PRJNA378560.
Results
A total of 144,343,706 reads were mapped to bovine miRNAs from serum and WBC. There were 313,261 sequences that mapped to viral miRNAs (Table 1). Of these, 32,538 mapped to miRNAs in serum and 280,723 to miRNAs in WBC. Sequences were identified for all known mature BLV miRNAs, with the exception of blv-miR-B3-5p. The remaining sequences were mapped to bovine miRNAs and excluded from further analysis.
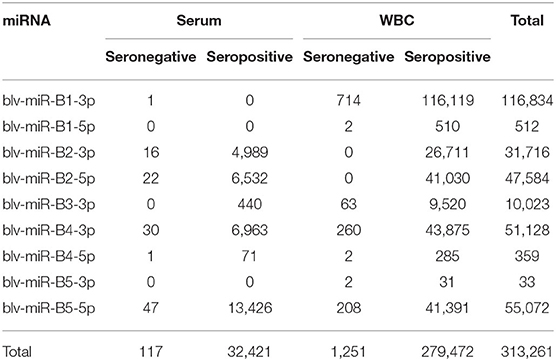
Table 1. Number of sequences for each miRNA identified in serum and white blood cells (WBC), in the seronegative and seropositive groups.
Figure 1 shows the significant (P < 0.05) log2 fold differences between the positive and the negative groups. For all significant BLV miRNAs, the positive group had greater number of sequences than the negative group. Blv-miR-B5-3p was not significant in serum or in WBC. In serum, blv-miR-B2-3p, blv-miR-B2-5p, blv-miR-B4-3p, and blv-miR-B5-5p, were significant and had an expression difference between the positive and the negative groups ranging from 7 to 8 log2 fold. In WBC, blv-miR-B2-3p and blv-miR-B2-5p had a 27 and 29 log2 fold difference between the two groups, respectively. The other significant BLV miRNAs in WBC had a difference in expression ranging from 7 to 8 log2 fold, which is similar to those observed in serum.
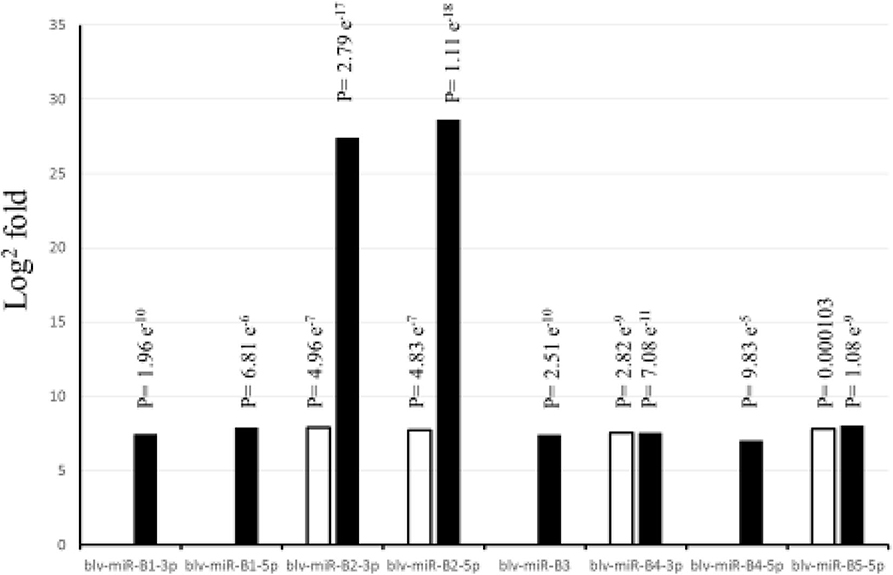
Figure 1. Differences in expression (Log2 fold) between cows positive and negative to exposure to bovine leukemia virus. White bars indicate expression in serum, and black bars indicate expression in white blood cells. P-values are adjusted and equivalent to false discovery rate (FDR).
The gene ontology analysis of the genes targeted by blv-miR-B1-5p and blv-miR-B4-5p, as involved in response to stress and immune system process pathways (Table 2). No other BLV miRNA was involved in these pathways. Both BLV miRNAs target different genes in both pathways. Blv-miR-B1-5p targets 15 different genes in the response to stress pathway and 9 genes in the immune system process pathway; however, C4A, COLEC12, GPS2, and TLR9, are involved in both pathways. Blv-miR-B1-5p targets a total of 20 genes in both pathways. Blv-miR-B4-5p targets 3 genes, ZFYVE26 in the response to stress pathway, FAM20C and MADCAM1 in the immune system process pathway.
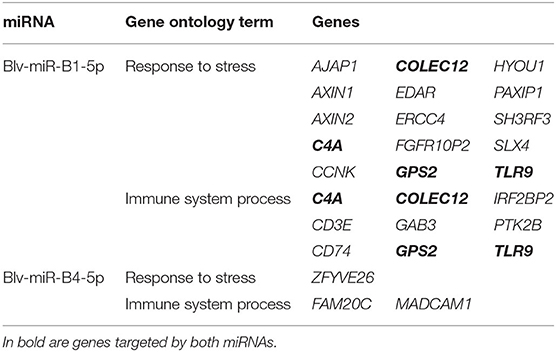
Table 2. Putative genes targeted in the host by blv-miR-B1-5p and blv-miR-B4-5p, identified as being involved in the response to stress and immune system processes.
Discussion
In a previous study, we identified differentially expressed transfer RNA fragments produced by the animal as a response to the exposure to BLV (22).
In the present study, the same animals were used to detect differentially expressed microRNAs produced by the BLV in cattle.
It has been established that BLV miRNAs modify gene expression of the host, promoting pathogenicity (19, 21). The comparison in expression of these miRNAs between positive and negative cattle groups is absent. Therefore, it was important to establish their expression to ascertain the potential role these miRNAs may play in establishing a BLV infection.
Blv-miR-B2-3p and blv-miR-B2-5p had the greatest differences in expression in WBC, compared to other BLV miRNAs. These miRNAs were not found to be involved in the response to stress or the immune system process pathways. Blv-miR-B2-5p was uncorrelated with other miRNAs in a study where BLV seroconversion status was compared (21). Blv-miR-B2-5p was found to be associated with the expression of RPTN. This gene is involved in cell differentiation and is down-regulated in patients with human papillomavirus (29). It is probable that blv-miR-B2-5p may down-regulate RPTN during a BLV infection. Despite being the BLV miRNAs with the greatest differences in expression between the positive and negative groups, they seem to be involved in processes other than the response of the animal to a BLV infection. Blv-miR-B2-3p has not been evaluated (21).
Blv-miR-B1-5p and blv-miR-B4-5p were associated with response to stress and immune systems processes, and were only significantly differentially expressed in WBC. It is possible that these BLV miRNAs are released in serum due to conclusion of their function within the cell. Additional studies would need to establish the reason for the release of BLV miRNAs in serum.
Genes found to be influenced by blv-miR-B1-5p have been observed to be related with leukemia in humans. The genes C4A, GPS2, and COLEC12, are involved in response to stress and in immune system processes. In addition, dysregulation of C4A, and GPS2, are also known to be involved in the development of leukemia in humans when they are dysregulated (30, 31). The gene COLEC12 produces a receptor that mediates the cross-talk between dendritic cells and gastric stromal cells and has been associated with the development of gastric cancer patients when deregulated (32). BLV miRNAs seem to dysregulate the expression genes to proliferate in cattle.
Toll-like receptors are a family of conserved glycoproteins that play a role in the innate immune system. The function is to sense the presence of a pathogen and initiate the immune response (33). The Toll-like receptor-9 (TLR9) was identified as being a target for blv-miR-B1-5p. The TLR9 gene is upregulated in cattle with a BLV high proviral load, compared to animals with a low proviral load, as well as an increase in messenger RNA for the protein produced by this gene (34, 35). It is unclear if the role of the blv-miR-B1-5p is to down-regulate the expression of this gene, given that in a BLV infection, TLR9 is overexpressed in cattle (34, 35). Further studies would need to be pursued to understand the role of blv-miR-B1-5p in a BLV infection in cattle.
The Wnt signaling pathway is composed of intercellular signaling proteins that regulate proliferation, cell division, and other processes relevant to cellular survival (36). The AXIN2 gene is the universal target of this pathway (37) and is dysregulated in leukemia patients (37, 38). It is probable that blv-miR-B1-5p dysregulates AXIN2 to produce leukemia in cattle.
Additional genes identified as being target of blv-miR-B1-5p have also been associated with different types of leukemia in humans. Structural chromosome rearrangements, specifically chromosome translocations that create fusion genes encoding chimeric proteins, characterize different types of leukemia. Genes involved in these fusion genes were identified as targets for blv-miR-B1-5p. Patients with a subtype of acute myeloid leukemia (AML), known as acute promyelocytic leukemia, show the fusion of the retinoic acid receptor alpha (RARA), with the promyelocytic leukemia gene. The gene IRF2BP2 is a target for blv-miR-B1-5p. In acute lymphoblastic leukemia (ALL) cases, the gene PTK2B, which is a target gene for blv-miR-B1-5p, has been identified as forming a fusion gene with KDM6A, STAG2, or TMEM2 (39, 40). In the case of stem cell leukemia-like syndrome, a fusion gene between FGFR1 and FGFR1OP2 characterize this condition in humans (41–43). The gene FGFR1OP2 was also identified as a target for blv-miR-B1-5p. The role of blv-miR-B1-5p is unclear in the fusion of the genes including IRF2BP2, PTK2B, and FGFR1OP2, if any. Further studies will need to establish if blv-miR-B1-5p plays a part in the development of fusion genes in leukemia cases in cattle.
The product of the gene CD74 is known to be involved in the defense mechanism of AML cells. This gene is a signaling receptor for the major histocompatibility complex type II, involved in antigen presentation, and its expression has been linked to cell survival pathways. Its expression has been associated with poor survival in patients with AML (44, 45). Expression of this gene assists in the defense mechanism of leukemia cells in the case of AML. This gene was identified as a target for blv-miR-B1-5p. It is possible the miRNA may influence the expression of CD74 in AML patients.
Overexpression of the SLX4 gene has been observed in the B-cell chronic lymphocytic leukemia (BCLL). B-cell chronic lymphocytic leukemia is characterized by lengthening of the telomeres. SLX4 is involved in the process known as alternative telomere lengthening, which characterizes BCLL (46). Similarly, SLX4 has also been associated with onset of Fanconi anemia, which is also characterized by telomere lengthening (47). This gene is a target by blv-miR-B1-5p, and has the potential to be associated with the development of both diseases.
Limited literature is available indicating the association of SH3RF3, PAXIP1, and CD3E, targeted by blv-miR-B1-5p. As part of a panel of 6 genes, SH3RF3 is used to establish the prognosis of patients affected with ALL. When SH3RF3 is expressed, in conjunction with CAMSAP1 and PCGF6, the outcome of ALL is favorable; however, when this genes is not expressed in ALL patients, the outcome of the disease is less than desirable (48). Blv-miR-B1-5p, may have a role in inhibiting expression of SH3RF3 to establish leukemia in cattle. The hematopoietic stem cells (HSC) are responsible in providing the organism with mature blood cells. The HSC reside in bone marrow pockets in the interphase of the bone and bone marrow. The PAXIP1 or PTIP genes are epigenetic regulators responsible for maintaining the bone marrow pockets. Downregulation of PAXIP1 results in downregulation of HSC. Downregulation of PAXIP1 has also been associated with downregulation of leukemia cells (49). The role of blv-miR-B1-5p in the regulation of PAXIP1 is unclear given that downregulation of PAXIP1 would result in a downregulation of leukemia cells. It has been established that CD3E is overexpressed in patients with T-cell ALL (50), and that patients with this condition and that a remission of the disease can be obtained with an anti-CD3E recombinant immunotoxin (51). Potentially, blv-miR-B1-5p has a role in the overexpression of CD3E in T-cell ALL patients; however, further studies would be needed to establish this effect. A similar process could be occurring for BLV infection in cattle.
Genes targeted by blv-miR-B4-5p have not been identified as playing a role in the development of leukemia in humans. Variants in the ZFYVE26 gene have been associated with colorectal cancer (52), variants in the MADCAM1 gene have been associated with multiple sclerosis (53), and variants in the FAM20C gene have been associated with development of Raine syndrome in humans (54). Although they are classified as being involved in the response to stress and immune system process pathways, there is no evidence suggesting their role in the development of leukemia. The role of these genes in processes associated with leukemia, and their relationship with BLV miRNAs, should be determined.
The sequence count for each BLV miRNA was greater in the positive group, compared to the negative group as expected; however, there were BLV miRNA sequences found in animals considered negative. This is possible given that animals were classified based on an ELISA. It is probable that animals with a negative ELISA, resulted with a low count of BLV miRNAs because they were exposed to the virus but had yet to develop an immune response high enough for a positive ELISA result. A longitudinal analysis could have established if animals considered seronegative at sampling seroconvert in future samplings.
There are differences in count number of sequences between serum and WBC. MiRNAs are produced in the nucleus of the cell, and due to their function they remain in the cell (6). Results from other studies have observed the difference in number of bovine miRNA counts, where there are greater number of bovine miRNA sequences when different tissues are compared (15). It is possible the low number of miRNA sequences in serum may be due to communication with other cells, or because they are excess from cellular processes.
It was established that BLV miRNAs were differentially expressed in serum and WBC, between seropositive and seronegative animals. Of the differentially expressed BLV miRNAs, seropositive animals had a higher expression of BLV miRNAs, when compared to seronegative animals. Most differentially expressed miRNAs had a consistent differential expression with an average of 7 log2 fold, with the exception of blv-miR-B2-3p and blv-miR-B2-5p, which had a differential expression averaging 27 log2 fold. These highly differentially expressed miRNAs target host genes unrelated to response to host stress or immune systems process. Blv-miR-B1-5p and blv-miR-B4-5p targeted host genes associated with response to stress and immune systems processes. It is unclear if BLV miRNAs have any role in the development of leukemia in cattle. However, blv-miR-B1-5p, produced by the BLV targets several genes related with development of leukemia in humans. MiRNAs involved in the development of leukemia in humans could also be responsible for the development of the condition in cattle.
Data Availability Statement
The datasets generated for this study can be found in the NCBI SRA under BioProject accession number PRJNA378560.
Ethics Statement
This animal study was reviewed and approved by Institutional Animal Care and Use Committee of the National Animal Disease Center, ARS, USDA.
Author Contributions
EC and JL conceived and designed the experiment. HM analyzed the data. EC and HM interpreted the results. EC wrote the manuscript. HM and JL reviewed and edited the manuscript. All authors contributed to the article and approved the submitted version.
Funding
This research was part of an intramural project of the USDA/ARS National Animal Disease Center. The USDA had no role in study design, data collection, analysis, interpretation of results, or preparation of manuscript.
Conflict of Interest
The authors declare that the research was conducted in the absence of any commercial or financial relationships that could be construed as a potential conflict of interest.
Acknowledgments
The authors would like to thank Randy Atchison and Duane Zimmerman for excellent technical assistance. Mention of trade name, proprietary product, or specified equipment does not constitute a guarantee or warranty by the USDA and does not imply approval to the exclusion of other products that may be suitable. USDA in an Equal Opportunity Employer.
References
1. Rhodes JK, Pelzer KD, Johnson YJ. Economic implications of bovine leukemia virus infection in mid-Atlantic dairy herds. J Am Vet Med Assoc. (2003) 223:346–52. doi: 10.2460/javma.2003.223.346
2. Frie MC, Coussens PM. Bovine leukemia virus: a major silent threat to proper immune responses in cattle. Vet Immunol Immunopathol. (2015) 163:103–14. doi: 10.1016/j.vetimm.2014.11.014
3. Bauermann FV, Ridpath JF, Dargatz DA. Bovine leukemia virus seroprevalence among cattle presented for slaughter in the United States. J Vet Diagn Invest. (2017) 29:704–6. doi: 10.1177/1040638717702183
4. Bartlett PC, Norby B, Byrem TM, Parmelee A, Ledergerber JT, Erskine RJ. Bovine leukemia virus and cow longevity in Michigan dairy herds. J Dairy Sci. (2013) 96:1591–7. doi: 10.3168/jds.2012-5930
5. Coutinho LL, Matukumalli LK, Sonstegard TS, Van Tassell CP, Gasbarre LC, Capuco AV, et al. Discovery and profiling of bovine microRNAs from immune-related and embryonic tissues. Physiol Genomics. (2007) 29:35–43. doi: 10.1152/physiolgenomics.00081.2006
6. McDaneld TG. MicroRNA: mechanism of gene regulation and application to livestock. J Anim Sci. (2009) 87(Suppl. 14):E21–8. doi: 10.2527/jas.2008-1303
7. Wahid F, Shehzad A, Khan T, Kim YY. MicroRNAs: synthesis, mechanism, function, and recent clinical trials. Biochim Biophys Acta. (2010) 1803:1231–43. doi: 10.1016/j.bbamcr.2010.06.013
8. Angelini TG, Emanueli C. MicroRNAs as clinical biomarkers? Front Genet. (2015) 6:240. doi: 10.3389/fgene.2015.00240
9. Wang J, Chen J, Sen S. MicroRNA as biomarkers and diagnostics. J Cell Physiol. (2016) 231:25–30. doi: 10.1002/jcp.25056
10. Samir M, Pessler F. Small non-coding RNAs associated with viral infectious diseases of veterinary importance: potential clinical applications. Front Vet Sci. (2016) 3:22. doi: 10.3389/fvets.2016.00022
11. Samir M, Vaas LA, Pessler F. MicroRNAs in the host response to viral infections of veterinary importance. Front Vet Sci. (2016) 3:86. doi: 10.3389/fvets.2016.00086
12. Correia CN, Nalpas NC, McLoughlin KE, Browne JA, Gordon SV, MacHugh DE, et al. Circulating microRNAs as potential biomarkers of infectious disease. Front Immunol. (2017) 8:118. doi: 10.3389/fimmu.2017.00118
13. Casas E, Cai G, Kuehn LA, Register KB, McDaneld TG, Neill JD. Association of MicroRNAs with antibody response to mycoplasma bovis in beef cattle. PLoS ONE. (2016) 11:e0161651. doi: 10.1371/journal.pone.0161651
14. Taxis TM, Bauermann FV, Ridpath JF, Casas E. Circulating MicroRNAs in serum from cattle challenged with bovine viral diarrhea virus. Front Genet. (2017) 8:91. doi: 10.3389/fgene.2017.00091
15. Taxis TM, Casas E. MicroRNA expression and implications for infectious diseases in livestock. CAB Reviews. (2017) 12:1–20. doi: 10.1079/PAVSNNR201712026
16. Kincaid RP, Burke JM, Sullivan CS. RNA virus microRNA that mimics a B-cell oncomiR. Proc Natl Acad Sci USA. (2012) 109:3077–82. doi: 10.1073/pnas.1116107109
17. Rosewick N, Momont M, Durkin K, Takeda H, Caiment F, Cleuter Y, et al. Deep sequencing reveals abundant noncanonical retroviral microRNAs in B-cell leukemia/lymphoma. Proc Natl Acad Sci USA. (2013) 110:2306–11. doi: 10.1073/pnas.1213842110
18. Durkin K, Rosewick N, Artesi M, Hahaut V, Griebel P, Arsic N, et al. Characterization of novel Bovine Leukemia Virus (BLV) antisense transcripts by deep sequencing reveals constitutive expression in tumors and transcriptional interaction with viral microRNAs. Retrovirology. (2016) 13:33. doi: 10.1186/s12977-016-0267-8
19. Gillet NA, Hamaidia M, de Brogniez A, Gutierrez G, Renotte N, Reichert M, et al. Bovine leukemia virus small noncoding RNAs are functional elements that regulate replication and contribute to oncogenesis in vivo. PLoS Pathog. (2016) 12:e1005588. doi: 10.1371/journal.ppat.1005588
20. Van Driessche B, Rodari A, Delacourt N, Fauquenoy S, Vanhulle C, Burny A, et al. Characterization of new RNA polymerase III and RNA polymerase II transcriptional promoters in the bovine leukemia virus genome. Sci Rep. (2016) 6:31125. doi: 10.1038/srep31125
21. Frie MC, Droscha CJ, Greenlick AE, Coussens PM. MicroRNAs Encoded by Bovine Leukemia Virus (BLV) are associated with reduced expression of B cell transcriptional regulators in dairy cattle naturally infected with BLV. Front Vet Sci. (2017) 4:245. doi: 10.3389/fvets.2017.00245
22. Taxis TM, Kehrli ME Jr, D'Orey-Branco R, Casas E. Association of transfer RNA fragments in white blood cells with antibody response to bovine leukemia virus in holstein cattle. Front Genet. (2018) 9:236. doi: 10.3389/fgene.2018.00236
23. Wingett SW, Andrews S. FastQ Screen: a tool for multi-genome mapping and quality control. F1000Res. (2018) 7:1338. doi: 10.12688/f1000research.15931.2
24. Martin M. Cutadapt removes adapter sequences from high-throughput sequencing reads. EMBnetjournal. (2018) 17:10–2. doi: 10.14806/ej.17.1.200
25. Friedlander MR, Mackowiak SD, Li N, Chen W, Rajewsky N. miRDeep2 accurately identifies known and hundreds of novel microRNA genes in seven animal clades. Nucleic Acids Res. (2012) 40:37–52. doi: 10.1093/nar/gkr688
26. Love MI, Huber W, Anders S. Moderated estimation of fold change and dispersion for RNA-seq data with DESeq2. Genome Biol. (2014) 15:550. doi: 10.1186/s13059-014-0550-8
27. Enright AJ, John B, Gaul U, Tuschl T, Sander C, Marks DS. MicroRNA targets in Drosophila. Genome Biol. (2003) 5:R1. doi: 10.1186/gb-2003-5-1-r1
28. Kertesz M, Iovino N, Unnerstall U, Gaul U, Segal E. The role of site accessibility in microRNA target recognition. Nat Genet. (2007) 39:1278–84. doi: 10.1038/ng2135
29. Kang SD, Chatterjee S, Alam S, Salzberg AC, Milici J, van der Burg SH, et al. Effect of productive human papillomavirus 16 infection on global gene expression in cervical epithelium. J Virol. (2018) 92:e01261. doi: 10.1128/JVI.01261-18
30. Yu L, Kim HT, Kasar S, Benien P, Du W, Hoang K, et al. Survival of Del17p CLL depends on genomic complexity and somatic mutation. Clin Cancer Res. (2017) 23:735–45. doi: 10.1158/1078-0432.CCR-16-0594
31. Guo L, Ren H, Zeng H, Gong Y, Ma X. Proteomic analysis of cerebrospinal fluid in pediatric acute lymphoblastic leukemia patients: a pilot study. Onco Targets Ther. (2019) 12:3859–68. doi: 10.2147/OTT.S193616
32. Chang LL, Hsu WH, Kao MC, Chou CC, Lin CC, Liu CJ, et al. Stromal C-type lectin receptor COLEC12 integrates H. pylori, PGE2-EP2/4 axis and innate immunity in gastric diseases. Sci Rep. (2018) 8:3821. doi: 10.1038/s41598-018-20957-2
33. Turin L, Riva F. Toll-like receptor family in domestic animal species. Crit Rev Immunol. (2008) 28:513–38. doi: 10.1615/critrevimmunol.v28.i6.30
34. Farias MVN, Lendez PA, Marin M, Quintana S, Martinez-Cuesta L, Ceriani MC, et al. Toll-like receptors, IFN-gamma and IL-12 expression in bovine leukemia virus-infected animals with low or high proviral load. Res Vet Sci. (2016) 107:190–5. doi: 10.1016/j.rvsc.2016.06.016
35. Martinez Cuesta L, Nieto Farias MV, Lendez PA, Rowland RRR, Sheahan MA, Cheuquepan Valenzuela FA, et al. Effect of bovine leukemia virus on bovine mammary epithelial cells. Virus Res. (2019) 271:197678. doi: 10.1016/j.virusres.2019.197678
36. Staal FJ, Clevers HC. WNT signalling and haematopoiesis: a WNT-WNT situation. Nat Rev Immunol. (2005) 5:21–30. doi: 10.1038/nri1529
37. Ng OH, Erbilgin Y, Firtina S, Celkan T, Karakas Z, Aydogan G, et al. Deregulated WNT signaling in childhood T-cell acute lymphoblastic leukemia. Blood Cancer J. (2014) 4:e192. doi: 10.1038/bcj.2014.12
38. Taskesen E, Staal FJ, Reinders MJ. An integrated approach of gene expression and DNA-methylation profiles of WNT signaling genes uncovers novel prognostic markers in acute myeloid leukemia. BMC Bioinformatics. (2015) 16(Suppl. 4):S4. doi: 10.1186/1471-2105-16-S4-S4
39. Tasian SK, Loh ML, Hunger SP. Philadelphia chromosome-like acute lymphoblastic leukemia. Blood. (2017) 130:2064–72. doi: 10.1182/blood-2017-06-743252
40. Pallares V, Hoyos M, Chillon MC, Barragan E, Prieto Conde MI, Llop M, et al. Focal adhesion genes refine the intermediate-risk cytogenetic classification of acute myeloid leukemia. Cancers. (2018) 10:436. doi: 10.3390/cancers10110436
41. Jin Y, Zhen Y, Haugsten EM, Wiedlocha A. The driver of malignancy in KG-1a leukemic cells, FGFR1OP2-FGFR1, encodes an HSP90 addicted oncoprotein. Cell Signal. (2011) 23:1758–66. doi: 10.1016/j.cellsig.2011.06.010
42. Ren M, Cowell JK. Constitutive Notch pathway activation in murine ZMYM2-FGFR1-induced T-cell lymphomas associated with atypical myeloproliferative disease. Blood. (2011) 117:6837–47. doi: 10.1182/blood-2010-07-295725
43. Qin H, Wu Q, Cowell JK, Ren M. FGFR1OP2-FGFR1 induced myeloid leukemia and T-cell lymphoma in a mouse model. Haematologica. (2016) 101:e91–4. doi: 10.3324/haematol.2015.137695
44. Ruvolo PP, Hu CW, Qiu Y, Ruvolo VR, Go RL, Hubner SE, et al. LGALS3 is connected to CD74 in a previously unknown protein network that is associated with poor survival in patients with AML. EBioMedicine. (2019) 44:126–37. doi: 10.1016/j.ebiom.2019.05.025
45. Yasinska IM, Sumbayev VV. Alliance of galectin-3 and CD74 biochemical networks as a crucial component of survival machinery operated by human acute myeloid leukaemia cells. EBioMedicine. (2019) 44:2–3. doi: 10.1016/j.ebiom.2019.05.051
46. Medves S, Auchter M, Chambeau L, Gazzo S, Poncet D, Grangier B, et al. A high rate of telomeric sister chromatid exchange occurs in chronic lymphocytic leukaemia B-cells. Br J Haematol. (2016) 174:57–70. doi: 10.1111/bjh.13995
47. Spinella JF, Healy J, Saillour V, Richer C, Cassart P, Ouimet M, et al. Whole-exome sequencing of a rare case of familial childhood acute lymphoblastic leukemia reveals putative predisposing mutations in Fanconi anemia genes. BMC Cancer. (2015) 15:539. doi: 10.1186/s12885-015-1549-6
48. Wang J, Mi JQ, Debernardi A, Vitte AL, Emadali A, Meyer JA, et al. A six gene expression signature defines aggressive subtypes and predicts outcome in childhood and adult acute lymphoblastic leukemia. Oncotarget. (2015) 6:16527–42. doi: 10.18632/oncotarget.4113
49. Das P, Veazey KJ, Van HT, Kaushik S, Lin K, Lu Y, et al. Histone methylation regulator PTIP is required to maintain normal and leukemic bone marrow niches. Proc Natl Acad Sci USA. (2018) 115:E10137–E10146. doi: 10.1073/pnas.1806019115
50. Saussoy P, Vaerman JL, Straetmans N, Deneys V, Cornu G, Ferrant A, et al. Differentiation of acute myeloid leukemia from B- and T-lineage acute lymphoid leukemias by real-time quantitative reverse transcription-PCR of lineage marker mRNAs. Clin Chem. (2004) 50:1165–73. doi: 10.1373/clinchem.2003.030114
51. Frankel AE, Woo JH, Ahn C, Foss FM, Duvic M, Neville PH, et al. Resimmune, an anti-CD3epsilon recombinant immunotoxin, induces durable remissions in patients with cutaneous T-cell lymphoma. Haematologica. (2015) 100:794–800. doi: 10.3324/haematol.2015.123711
52. Yu L, Yin B, Qu K, Li J, Jin Q, Liu L, et al. Screening for susceptibility genes in hereditary non-polyposis colorectal cancer. Oncol Lett. (2018) 15:9413–9. doi: 10.3892/ol.2018.8504
53. Dardiotis E, Panayiotou E, Siokas V, Aloizou AM, Christodoulou K, Hadjisavvas A, et al. Gene variants of adhesion molecules predispose to MS: a case-control study. Neurol Genet. (2019) 5:e304. doi: 10.1212/NXG.0000000000000304
Keywords: BLV, cattle, microRNA, serum, white blood cells
Citation: Casas E, Ma H and Lippolis JD (2020) Expression of Viral microRNAs in Serum and White Blood Cells of Cows Exposed to Bovine Leukemia Virus. Front. Vet. Sci. 7:536390. doi: 10.3389/fvets.2020.536390
Received: 19 February 2020; Accepted: 17 August 2020;
Published: 22 September 2020.
Edited by:
Göran Andersson, Swedish University of Agricultural Sciences, SwedenReviewed by:
Shaojun Liu, Hunan Normal University, ChinaStefan Vilcek, University of Veterinary Medicine in Kosice, Slovakia
Copyright © 2020 Casas, Ma and Lippolis. This is an open-access article distributed under the terms of the Creative Commons Attribution License (CC BY). The use, distribution or reproduction in other forums is permitted, provided the original author(s) and the copyright owner(s) are credited and that the original publication in this journal is cited, in accordance with accepted academic practice. No use, distribution or reproduction is permitted which does not comply with these terms.
*Correspondence: Eduardo Casas, eduardo.casas@usda.gov