- 1Facultad de Medicina Veterinaria y Zootecnia, Universidad Autónoma de Chiapas, Tuxtla Gutiérrez, Mexico
- 2Instituto Tecnológico de Tuxtla Gutiérrez, Tuxtla Gutiérrez, Mexico
- 3Instituto de Ciencias Biológicas, Universidad de Ciencias y Artes de Chiapas, Tuxtla Gutiérrez, Mexico
- 4Rollins School of Public Health, Emory University, Atlanta, GA, United States
- 5Department of Microbiology and Immunology, University of Mississippi Medical Center, Jackson, MS, United States
The green iguana appears to be a carrier for bacteria causing gastrointestinal infections in humans. The presence of diarrheagenic E. coli (DEC) pathotypes, however, has not been studied in this reptile. The aim of the current work was to investigate the prevalence of DEC in the intestines of 240 captive green iguanas, their phylogenetic groups, and the antibiotic susceptibility profile. E. coli strains were isolated from 41.7% (N = 100/240) of the intestinal content of green iguanas. DEC strains was identified in 25.9% of the screened population and were detected in the majority (62%, p = 0.009) of those reptiles carrying E. coli strains. Among DEC strains, STEC strains carrying the stx1 gene were the most prevalent pathotype isolated (38.7%), followed by EAEC and ETEC (27.4% each). Genetic markers of DEC strains belonging to the EHEC pathotype were not detected. More than a half of DEC strains were classified into the Clade I-II phylogroup (64.5%), followed by the phylogroup A (14.5%). The antibiotic susceptibility method demonstrated that a high proportion of DEC strains were resistance, or non-susceptible, to carbenicillin, amikacin, and ampicillin. We conclude that the green iguana kept in captivity is a carrier of DEC strains bearing resistance to first-line antibiotics, including penicillins. Given the increase presence of the green iguana in Latin American households, these reptiles represent a potential source of transmission to susceptible humans and therefore a potential source of gastrointestinal disease.
Introduction
Escherichia coli is a commensal bacteria found in the gastrointestinal tract of human and animals. Its ability to acquire virulence genes has originated strains that cause serious gastrointestinal infections in humans as well as animals (1). E. coli pathogenic strains are responsible for ~56 million cases of diarrhea causing 0.2 million annual deaths worldwide, most of it in children between 2 and 5 years of age (2).
Based on the virulence traits of this Gram-negative bacterium and the location of the infection within the human host, pathogenic E. coli strains are classified in Diarrheagenic E. coli (DEC) and Extra intestinal Escherichia coli (ExPEC). This last group includes uropathogenic E. coli (UPEC) and neonatal meningitis E. coli (NMEC). The DEC group includes Enterotoxigenic E. coli (ETEC), Enteropathogenic E. coli (EPEC), Enteroinvasive E. coli (EIEC), Shiga toxin-producing E. coli (STEC), Enteroagregative E. coli (EAEC), and diffusely adherent E. coli (DAEC) (3). EHEC (Enterohemorrhagic E. coli) is a subgroup of STEC strains associated with outbreaks and severe clinical illness in humans (4). Among the DEC pathotypes, STEC infections cause diarrhea, and hemorrhagic colitis but the infection occasionally progress to hemolytic uremic syndrome (HUS) that despite severe sequela can cause death (5). STEC virulence is mainly based on its ability to produce two isoforms of the Shiga toxin (Stx): Stx1 and Stx2, related to infections in human, mainly subtypes Stx2 (6). Multiple STEC serotypes have been reported in outbreaks of human disease, being the most frequent STEC O157, followed by non-O157 serotypes including O26, O103, O111, O21, O45, and O145 strains (7).
It have been reported that bovines, ovines, goats, pigs, dogs, and cats are reservoirs of different DEC strains (8). Ruminants, for example, are an important reservoir of DEC strains with their feces representing a key source of contamination of water and food that cause infections to humans. Several epidemiological studies have demonstrated the zoonotic potential of STEC strains. For instance, a study conducted in Belgium analyzing diarrheic calves found that 58% of STEC strains were capable of inducing the attaching/effacement (A/E) lesion, followed by EPEC strains (38%); the O26 and O111 serogroups were the most frequent (47.5 and 30%, respectively) (9). Another study found a prevalence of 0.7% of STEC serotype O103:H2 strains in feces of Norwegian sheep (10). A work conducted in Iran showed the presence of STEC strains in healthy calves and goats (26.3 and 27.5%, respectively) (6).
Recently, in Mexico, a high prevalence of STEC (40.7%) and ETEC (26.7%) was observed in dairy cows, besides reporting the presence of E. coli serotype O157:H7 and O104:H12 (11). The presence of DEC strains in wild animals seems to be low in comparison to their prevalence in humans and ruminants. EPEC (typical and atypical) and STEC strains have been isolated from captive wild birds in Brazil (12, 13). A study that surveyed animals from zoos in India demonstrated a low prevalence of DEC strains in wild ruminants (STEC 7.14%; EPEC 1.58%), in non-ruminant animals (STEC 3.48%; EPEC 5.81%) and wild birds (EPEC 5.84%) (14).
In Mexico and in other South American countries, the green iguana (Iguana iguana Linnaeus, 1758) has played an important role in the economy of some regions of these Latin America countries as these animals are sold for human consumption but also kept as pets. These factors led to a dramatic decline of iguana populations the last few years. Important efforts, however, to preserve the species have emerged in Mexico such as a special protection is in place according to the Mexican Official Norm NOM-059-SEMARNAT-2010 (15) as well as efforts to promote their conservation by means of the Wildlife Management Units (WMU) (16).
Iguana species can carry in their intestines Salmonella species (17, 18) and Escherichia coli (19–21). The prevalence of E. coli in iguana species ranges from 40 to 70%. For example, in the Ricord's iguana (Cyclura ricordi) the prevalence reported was 50% (22), while in the land iguana (Conolophus pallidus) from the Galapagos Island it was 70.83% (19). Regarding the green iguana, studies have reported an E. coli prevalence of 40% (21) and 53.2% (18). To the best of our knowledge, whether the green iguana can carry DEC pathotypes has not been previously investigated and it was the main motivation for this study.
Therefore, the goal of this study was to investigate the prevalence of DEC strains colonizing the intestines of captive I. iguana. To figure out whether a subset of DEC strains is associated to intestinal colonization of the green iguana, we also determined their phylogenetic group as well as their susceptibility to first-line antibiotics.
Materials and Methods
Green Iguana Population
This study was approved by the Committee for Animal Care of the University Autonomous of the Chiapas state (approval ID number 06/VET/RPR/269/16). From autumn 2015 to spring 2017, a total of 240 captive I. iguana were selected from the WMU as follows: 112 specimens from the Istmo-Costa region and 128 iguanas from the Metropolitan region of Chiapas, Mexico. Those regions of the State of Chiapas concentrate the majority of WMU for green iguana. The reptiles at WMU from the Metropolitan region were maintained in cages of 27 or 253 ft3, while reptiles from the Istmo-Costa region were placed in wired mesh confinements of 16 × 33 ft. Lettuce, carrots, and cabbage constitute the daily food source and potable water was available ad libitum from water fountains. The reptiles were safely retrieved from their respective cages and were classified in accordance to their age: 126 juvenile iguanas (6–18 months of age) and 114 adults (more than 36 months of age). As juvenile iguanas present physical characteristics that do not allow distinguishing males from females, sex was determined only within the adult population. Male iguanas presented swelling of the hemipenis (females lack of this characteristic) and bigger femoral pores than females (23). The study utilized 55 females and 59 males.
Isolation of E. coli and Identification of DEC Strains
Intestinal content was collected in accordance to approved guidelines (24). Briefly, a sterile swab was introduced into the cloaca and after rotating gently, it was placed in Stuart agar gel (Copan) for its transportation to the laboratory. Swabs were streaked immediately onto Eosin and Methylene Blue agar (BD-BBL) and incubated at 37°C for 24 h. Up to 10 lactose fermenting colonies were selected and analyzed by standard biochemical tests. MacConkey agar plates with sorbitol were used for the detection of EHEC serotype O157:H7 (25). E. coli strains were also genetically identified by PCR amplification of the uidA gene, that encodes for β-glucuronidase specific for E. coli (26).
Diarrheagenic E. coli Pathotypes, Serogroups, and Phylogenetic Group's Identification by Polymerase Chain Reaction (PCR)
The following reference strains were used: E. coli strain (ATCC® 25922™) as a negative control, while EAEC 042 (044:H18), ETEC H10407 (O78:H11), EPEC E2348-69 (O127:H6), STEC EDL933 (O157:H7), and EIEC E11 (O124NM) prototype strains were used as a positive control in PCR reactions. Strains were kindly provided by Dr. Teresa Estrada-García from CINVESTAV, Mexico, and deposited into the bacterial collection of the University of Sciences and Arts of the state of Chiapas (27).
To obtain bacterial DNA, bacterial lysates of each of the 10 previously selected colonies were prepared by resuspending it in 1 mL of deionized water and then boiling the preparation for 10 min. The bacterial lysate was centrifuged at maximum speed for 5 min and DNA-containing supernatant was separated and stored at −80°C until used. Specific gene-targets for Enteroagregative E. coli (EAEC) (aap, aggR, and AAprobe genes) were amplified by a technique described by Cerna et al. (28). For Enterotoxigenic E. coli (ETEC) (lt and st genes), Enteropathogenic E. coli (EPEC) (bfpA and eaeA genes), Shiga toxin-producing E. coli (STEC) (stx1 and stx2 genes), and Enteroinvasive E. coli (EIEC) (ial gene), we utilized targets and conditions described by López-Saucedo et al. (29). The eae and hlyA genes, molecular markers for Enterohemorragic E. coli (EHEC), a well-known STEC sub-group that cause disease in humans, were amplified as established by Oswald et al. (30) and Schmidt et al. (31), respectively. The STEC strains were further analyzed in order to detect the O26, O45, O103, O111, O113, O121 and O145 antigens. To this end, primers amplifying the wzx gene (O- antigen flippase) in the O-antigen gene clusters were utilized as described by DebRoy et al. (32). The O157 antigen was investigated by amplifying the rfb gene (O- specific polysaccharide), according to Paton and Paton (33).
E. coli strains were further classified, using a quadruplex PCR method, into seven phylogroups: A, B1, B2, C, D, E, F, and the cryptic Clade I (34). This method is based in the presence or absence of chuA, yjaA, arpA, trpA genes and the TSPE4 C2 DNA fragment. PCR conditions and primers are shown in Table 1. PCR reactions were run in a thermal cycler C1000 (BioRad) and PCR products were analyzed through electrophoresis in agarose gel (2%) ran at 80 V for 1 h. Agarose gels were stained with SyberGreen® (Invitrogen), and photographed with the Molecular Imager® Gel Doc™ XR System (BioRad). The lambda molecular weight markers (100 and 1,000 bp) were used (Invitrogen, USA).
Antimicrobial Susceptibility Analysis
The disk- diffusion method was utilized following recommendations by the Clinical and Laboratory Standards Institute (35). The following antimicrobial susceptibility test discs (BD BBL™ Sensi-Disc™) were utilized, according to different antimicrobial categories: Penicillins, ampicillin (AMP;10 μg) and carbenicillin (CAR;100 μg); aminoglycosides, amikacin (AMK;30 μg), netilmicin (NET;30 μg) and gentamicin (GEN;10 μg); cephalosporins, cephalothin (CEF;30 μg) and cefotaxime (CTX;30 μg); quinolones, ciprofloxacin (CIP;5 μg) and norfloxacin (NOR;10 μg); phenicols, chloramphenicol (CHL;30 μg); folate inhibitor, trimethoprim-sulfamethoxazole (SXT;25 μg) and furans, nitrofurantoin (NIT;300 μg). Strains resistant to β-lactams were further analyzed with amoxicillin-clavulanic acid discs (AMC; 20/10 μg). E. coli Non-susceptible strains (including intermediate and resistant phenotypes) to at least three antibiotics from different antimicrobial categories were recorded as multi-drug resistant (MDR) strains (36).
Statistical Analysis
Prevalence of E. coli pathotypes, serogroups, phylogenetic groups, and susceptibility to antibiotics were analyzed by descriptive statistics. Proportions of typical molecular markers among DEC strains were analyzed by binomial test. The analysis between categorical variables was performed using the two-tailed Fisher's Exact test or Chi- Square test (when the frequencies were higher than 5) establishing a significance level when p < 0.05. All statistical analyses were conducted using the IBM SPSS statistics software (Chicago, SPSS Inc.).
Results
Diarrheagenic E. coli Identification
We isolated 100 E. coli strains of fecal samples collected from 240 iguanas kept in captivity. DEC strains was identified in 25.9% of the screened population of I. iguana and were detected in the majority [62% (N = 62), p = 0.009] of those reptiles carrying E. coli strains (N = 100). Among DEC strains, STEC (40.3%) was the most prevalent category followed by EAEC (27.4%, with a significative frequency of the aap gene) and ETEC (27.4%). EPEC strains had the lowest prevalence (4.9%) (Table 2). EHEC O157:H7 gene markers were not amplified by PCR neither EHEC strains were detected on MacConkey/sorbitol agar plates.
Our PCR approach revealed a statistically significant higher frequency of STEC strains carrying the stx1 gene in comparison to those carrying stx2. The eae and hlyA virulence genes were not amplified in any of these STEC strains suggesting that they did not belong to the EHEC category. Accordingly, O- antigen genes carried by EHEC's most common serotypes (e.g., O26, O45, O103, O111, O113, O121, O145, and O157) were not amplified in any of these strains.
Investigation of Phylogenetic Groups
Given that these iguanas are in captivity we sought to investigate whether DEC strains were related by obtaining their phylogenetic group. Among these E. coli strains (N = 62), the clade I or II phylogroup was significantly the most predominant (64.5%), followed by phylogroups A and B2. Some of DEC strains (17.8%) could not be assigned to a known phylogroup (Table 3). Thus, DEC isolated from I. iguana may be related since the majority of strains were classified in clade I or II.
There was not association between the prevalence of E. coli pathotypes and the age or sex of the iguanas. Regarding the geographic region were these reptiles are kept in captivity, a statistically significant association was observed between STEC strains isolated from iguanas kept at the Metropolitan (i.e., urban) region in comparison to those from the Istmo-Costa (i.e., rural) (Table 4).
Antimicrobial Susceptibility of DEC Strains
Because these DEC strains represent a potential source of contamination for humans and therefore a source of gastrointestinal disease, we investigated their susceptibility to antibiotics utilized to treat gastrointestinal disease caused by Gram negative bacteria. More than a half of DEC strains were non-susceptible to antibiotics from the penicillin category, as well as two out of three antibiotics belonging to aminoglycosides. This trend was also observed when DEC strains were tested for their susceptibility to cephalothin and norfloxacin. 82.3% (N = 51) of DEC strains were non-susceptible to at least three antibiotics from different antimicrobial categories, and therefore strains were considered as multi-drug resistant (MDR). All strains non-susceptible to ampicillin were susceptible to amoxicillin-clavulanic acid (data not shown). In addition, the majority of strains were susceptible to ciprofloxacin and chloramphenicol (Figure 1).
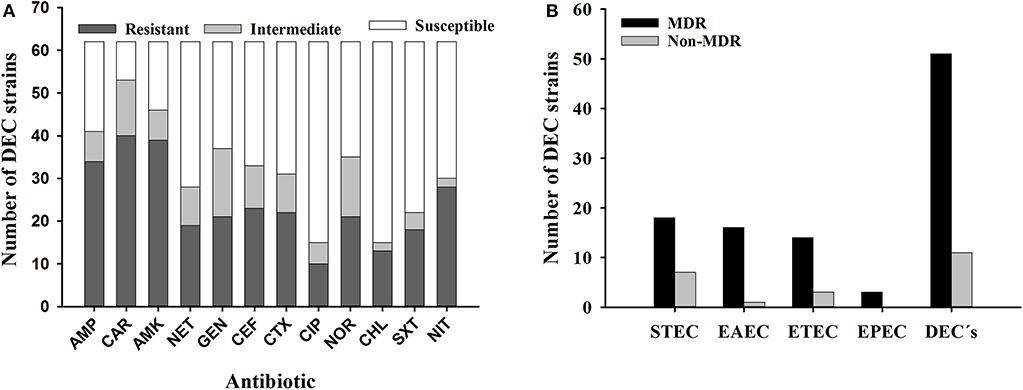
Figure 1. Antibiotic susceptibility of DEC strains (N = 62) isolated from I. iguana in Chiapas, Mexico. A, DEC strains with susceptibility, intermediate resistance or resistance against antibiotics. B, MDR phenotype among DEC strains. AMP; Ampicillin, AMK; Amikacin, CAR; Carbenicillin, GEN; Gentamicin, NET; Netilmicin, CEF; Cephalotin, CTX; Cefotaxime, CIP; Ciprofloxacin, NOR; Norfloxacin, CHL; Chloramphenicol, SXT; Trimethoprim-sulfamethoxazole, NIT; Nitrofurantoin; MDR, multidrug-resistant; STEC, Shiga toxin-producing E.coli; EAEC, Enteroaggregative E. coli; ETEC, Enterotoxigenic E. coli.; EPEC, Enteropathogenic E. coli; DEC, diarrheagenic E. coli.
Discussion
Escherichia coli is an ubiquitous Gram negative bacteria considered as one of the main constituent of the gastrointestinal tract of animals (37), humans (38), as well as reptiles such as turtles (39) and snakes (40). Nevertheless, there is almost no information about the presence and prevalence of E. coli pathotypes, such as DECs in the intestines of reptiles (41). This study revealed that the majority of E. coli strains isolated from I. iguana carried genes encoding virulence factors associated with diarrhea in humans (62%) such as those carried by STEC, EAEC, or ETEC pathotypes. We found similar carriage prevalence of the pathogenic variants of DEC strains in I. iguana regardless sex or age.
How do Iguanas acquire pathogenic DEC strains? Contamination of their food and water sources seems to be the main driver for iguanas to acquire DEC strains. Preliminary results from our group found a high prevalence of DEC strains in water and food samples (52 and 48%, respectively) from the Metropolitan region than that in the Istmo-Costa region (17 and 13%, respectively); STEC strains was the more frequently pathotype isolated. Besides that water offered to iguanas in the Metropolitan region might be contaminated with DEC strains, cleaning of their water fountains can take up to a week allowing further growth of DEC strains in that water source. Given that cattle farms are not commonly found in the metropolitan region, and cattle have been reported as a main reservoir for DEC strains, the source of DEC strains that contaminate water in the urban sites might be human feces.
Several studies have demonstrated that E. coli pathotypes are isolated from dairy cows (8), sheep (42), and pigs (43). In Mexico, a prevalence of 40.7 and 26.7% for the carriage of STEC or ETEC in bovine, respectively, was reported in the states of Jalisco, Sinaloa, and Sonora (11). The prevalence of STEC strains among livestock in southern Mexico is unknown but current studies in our laboratories are investigating the presence of DECs in livestock in the same geographical regions as those studied in this work. To the best of our knowledge, however, the presence of DEC strains in the intestine of iguanas had not been reported.
Acquisition of these DEC strains also can be a result of coprophagy, a behavior seen in iguanas because it is required for biochemical digestion of vegetables of their diet (44). Captivity in urban settings along with the mentioned coprophagy should also account for the presence of DEC strains in the intestine of iguanas. These observations are supported by the fact that a statistical significant association was observed in this study between intestinal carriage of STEC strains and iguanas kept in captivity at a metropolitan region of Chiapas. Reptiles may be in closer contact with human feces because of the crowdedness of an urban setting. Data supporting this hypothesis includes our own observations reporting the isolation of DEC's (13.8%) from the feces of 94 children from Chiapa de Corzo, a locality belonging to the metropolitan region (45). Our findings agree with those by Sylvester et al. (21) whom demonstrated an association between the prevalence of E. coli carriage and certain geographic regions. We hypothesize that factors such as the weather, season of the year (summer and autumn), such as well as a potential hygiene deficiency when handling iguanas (fecal contamination in water and food in cages) favors the acquisition of DEC bacteria (46, 47).
In the current study, the prevalence of STEC carrying the stx1 gene was higher than that of those carrying the stx2 gene (Table 1). However, these strains were not EHEC as none carried the eae and hlyA genes, neither genes that encode for the following O antigens associated to EHEC strains: O26, O45, O103, O111, O113, O121, O145, and O157. Stx toxins represent the key virulence factor for diarrheal disease caused by STEC strains (6). Stx2 toxin is more virulent than Stx1 because that toxin is responsible for causing Hemolytic Uremic Syndrome in humans (48). Similar to the current work, a study conducted in Isla Cabritos National Park (Dominican Republic) and Granada (West Indies) failed to isolate EHEC O157:H7 from I. iguana (21, 49). EHEC strains belonging to serotypes O157, O26, O145, and O111 have been isolated from cases of HUS in humans from USA and Europe (50, 51). Therefore, our data indicate that I. iguana kept in captivity carries Stx1-producing STEC strains but not EHEC strains.
The present study also revealed that the E. coli isolates were grouped mainly in Clade I-II. As such, our results agree with studies demonstrating that environmental E. coli strains belong to different cryptic clades (52). According to this technique, the majority of E. coli strains could be assigned to the phylogroups mentioned above, however, in order to analyze in deep the clonality, or not, more sophisticated molecular assays such as whole genome sequence are warranted (53).
Regarding susceptibility to antibiotics, the majority of DEC strains were non-susceptible to at least three antibiotics from the different categories assessed, including penicillins, aminoglycosides, and some of the broad-spectrum antibiotics cephalothin and norfloxacin. For all DEC's strains analyzed, the MDR phenotype was mainly observed among STEC strains (N = 18) (Figure 1). Studies recently published showed a similar prevalence Amezquita-Lopez et al. (54) investigated antibiotic resistance (i.e., aminoglycosides, cephalosporins, penicillins, and tetracyclines) among STEC strains recovered from healthy farm animals at North Western Mexico and showed that nearly half of those strains exhibited a MDR profile; strains were associated to human disease. A study of E. coli strains (N = 841) isolated from feces of healthy cattle from northwest and western Mexico, showed that STEC and ETEC pathotypes were the most prevalent (40.7 and 26.7%, respectively); in some STEC strains (25.7%), resistance to penicillins, cephalosporins, tetracycline, and trimethoprim/sulfamethoxazole was observed (11). Another work conducted in Brazil showed that 19/57 E. coli strains isolated from sheep were non-susceptible to sulfonamides (57.9%), followed by non-susceptibility to ampicillin as well as cephalosporins (47.3% each); among those strains the acquired antimicrobial resistance, the gene encoding beta-lactamases was the most prevalent (55). These data, along with those described in the current study, are worrisome since treatment failure is expected in the event of a zoonosis leading to outbreaks of gastrointestinal illness. While DEC strains had not been isolated from iguanas until this study, in other studies where normal flora E. coli were isolated from iguanas captured at a Caribbean island, authors reported susceptibility to different antibiotics of their iguana's E. coli isolates (21). The non-susceptibility to antibiotics observed in DEC strains isolated from iguanas in Chiapas might indicate that resistance to antibiotics is a consequence of the acquisition of strains from humans since these reptiles are not treated with antibiotics during their life-span. Moreover, antibiotic resistance is not a common trait in bacteria isolated from wildlife animals (19). Therefore, our findings suggest a microbiological impact caused by the conditions of captivity.
The green iguana is utilized as a pet in some Latin American countries, including in South Mexico. Animals used with this purpose are considered as a potential source of zoonosis and, as observed in this study, they may also bear strains with resistance to commonly used antibiotics that may complicate infections in the event of outbreaks of zoonotic origin.
Conclusions
The current study demonstrated the presence of diarrheagenic E. coli (mainly STEC) in I. iguana kept in captivity. The findings of the current work might be extremely useful for the sanitary efforts that are made during the green iguana handling, with the purpose of reducing its potential as a carrier for pathogenic bacteria. Additional studies about the genetic lineage of the DEC pathotypes found in the green iguana will allow us to understand the clonal origin and predict possible bacterial outbreaks in humans with DEC strains from reptiles kept in captivity, which may represent a risk for public health.
Data Availability Statement
The datasets generated for this study are available on request to the corresponding author.
Ethics Statement
This animal study was reviewed and approved by Committee for Animal Care of the University Autonomous of the Chiapas state (approval ID number 06/VET/RPR/269/16).
Author Contributions
GB-T, FG-M, and BR-S conceived and design of the study. LM-G, MO-L, CI-M, PM-N, and CT-C obtained fecal samples from iguanas, as well laboratory work. LP-V and JP-B determine virulence genes and phylogenetic analyses. GB-T, JV, and JG-J performed the statistical analysis and wrote the first draft of the manuscript. All authors contributed to manuscript revision, read, and approved the submitted version.
Funding
Financial support was partially granted to JG-J by the Program for Strengthening Educational Quality of the University of Science and Arts of the State of Chiapas (grant number C/PFCE-2016-07MSU0002G-13-43).
Conflict of Interest
The authors declare that the research was conducted in the absence of any commercial or financial relationships that could be construed as a potential conflict of interest.
Acknowledgments
We want to thank Dayanf Fernanda Saldaña-Molina, Eglantina Corzo-Cobos, Paola Gómez-Velázquez, Brenda OvandoDiaz, Gabriel Nucamendi-Moguel, and Jessica Estrada-Vázquez, for their contribution in the data collection. We also thanks the assistance provided by personnel of the Wildlife Management Units of the State of Chiapas, Mexico. This manuscript has been released as a Pre-Print at bioRxiv [http://dx.doi.org/10.1101/624544 (56)].
References
1. Kambire O, Adingra AA, Yao KM, Koffi-Nevry R. Prevalence of virulence genes associated with diarrheagenic pathotypes of Escherichia coli isolates from water, sediment, fish, and crab in Aby Lagoon, Cote d'Ivoire. Int J Microbiol. (2017) 2017:9532170. doi: 10.1155/2017/9532170
2. Nyanga PL, Onyuka J, Webale MK, Were T, Budambula V. Escherichia coli pathotypes and Shigella sero-groups in diarrheic children in Nairobi city, Kenya. Gastroenterol Hepatol Bed Bench. (2017) 10:220–8.
3. Croxen MA, Law RJ, Scholz R, Keeney KM, Wlodarska M, Finlay BB. Recent advances in understanding enteric pathogenic Escherichia coli. Clin Microbiol Rev. (2013) 26:822–80. doi: 10.1128/CMR.00022-13
4. Torres AG, Amaral MM, Bentancor L, Galli L, Goldstein J, Krüger A, et al. Recent advances in Shiga toxin-producing Escherichia coli research in Latin America. Microorganisms. (2018) 6:E100. doi: 10.3390/microorganisms6040100
5. Oh KH, Shin E, Jung SM, Im J, Cho SH, Hong S, et al. First isolation of a hybrid shigatoxigenic and enterotoxigenic Escherichia coli strain harboring the stx2 and elt genes in Korea. Jpn J Infect Dis. (2017) 70:347–8. doi: 10.7883/yoken.JJID.2016.237
6. Taghadosi R, Shakibaie MR, Alizade H, Hosseini-Nave H, Askari A, Ghanbarpour R. Serogroups, subtypes and virulence factors of shiga toxin-producing Escherichia coli isolated from human, calves and goats in Kerman, Iran. Gastroenterol Hepatol Bed Bench. (2018) 11:60–7.
7. Tang Y, Kim H, Singh AK, Aroonnual A, Bae E, Rajwa B, et al. Light scattering sensor for direct identification of colonies of Escherichia coli serogroups O26, O45, O103, O111, O121, O145 and O157. PLoS ONE. (2014) 9:e105272. doi: 10.1371/journal.pone.0105272
8. Singh P, Sha Q, Lacher DW, Del Valle J, Mosci RE, Moore JA, et al. Characterization of enteropathogenic and Shiga toxin-producing Escherichia coli in cattle and deer in a shared agroecosystem. Front Cell Infect Microbiol. (2015) 5:29. doi: 10.3389/fcimb.2015.00029
9. Fakih I, Thiry D, Duprez JN, Saulmont M, Iguchi A, Pierard D, et al. Identification of Shiga toxin-producing (STEC) and enteropathogenic (EPEC) Escherichia coli in diarrhoeic calves and comparative genomics of O5 bovine and human STEC. Vet Microbiol. (2017) 202:16–22. doi: 10.1016/j.vetmic.2016.02.017
10. Sekse C, Sunde M, Hopp P, Bruheim T, Cudjoe KS, Kvitle B, et al. Occurrence of potentially human-pathogenic Escherichia coli O103 in norwegian sheep. Appl Environ Microbiol. (2013) 79:7502–9. doi: 10.1128/AEM.01825-13
11. Navarro A, Cauich-Sánchez PI, Trejo A, Gutiérrez A, Díaz SP, Díaz C, et al. Characterization of diarrheagenic strains of Escherichia coli Isolated from cattle raised in three regions of Mexico. Front Microbiol. (2018) 9:2373. doi: 10.3389/fmicb.2018.02373
12. Sanches LA, Gomes MDS, Teixeira RHF, Cunha MPV, Oliveira MGX, Vieira MAM, et al. Captive wild birds as reservoirs of enteropathogenic E. coli (EPEC) and Shiga-toxin producing E coli (STEC). Braz J Microbiol. (2017) 48:760–3. doi: 10.1016/j.bjm.2017.03.003
13. Lopes ES, Maciel WC, Medeiros PHQ, Bona MD, Bindá AH, Lima SV, et al. Molecular diagnosis of diarrheagenic Escherichia coli isolated from psittaciformes of illegal wildlife trade. Pesquisa Vet Bra. (2018) 38:762–6. doi: 10.1590/1678-5150-pvb-5083
14. Milton AAP, Agarwal RK, Priya GB, Aravind M, Athira CK, Rose L, et al. Captive wildlife from India as carriers of Shiga toxin-producing, enteropathogenic and enterotoxigenic Escherichia coli. J Vet Med Sci. (2019) 81:321–7. doi: 10.1292/jvms.18-0488
15. Procuraduría Federal de Protección al Ambiente (PROFEPA). Mexican Official Norm NOM-059-SEMARNAT-2010. Protección Ambiental-Especies Nativas de México de Flora y Fauna Silvestres-Categorías de Riesgo y Especificaciones Para su Inclusión, Exclusión o Cambio-Listade Especies en Riesgo. Ciudad de México (2016). Available online at: https://www.gob.mx/profepa/documentos/norma-oficial-mexicana-nom-059-semarnat-2010 (accessed April 1, 2019).
16. Sisk TD, Castellanos VAE, Koch GW. Ecological impacts of wildlife conservation units policy in Mexico. Front Ecol Environ. (2007) 5:209–12. doi: 10.1890/1540-9295(2007)5[209:EIOWCU]2.0.CO;2
17. Sylvester W, Amadi V, Pinckney R, Macpherson C, McKibben J, Bruhl-Day R, et al. Prevalence, serovars and antimicrobial susceptibility of Salmonella spp. from wild and domestic green iguanas (Iguana iguana) in Grenada, West Indies. Zoo Pub Health. (2014) 61:436–41. doi: 10.1111/zph.12093
18. Romero SB, CíŽek A, Masaríková M, Knotek Z. Choanal and cloacal aerobic bacterial flora in captive green iguanas: a comparative analysis. Acta Vet Brno. (2015) 84:19–24. doi: 10.2754/avb201584010019
19. Thaller MC, Migliore L, Marquez C, Tapia W, Cedeno V, Rossolini GM, et al. Tracking acquired antibiotic resistance in commensal bacteria of galapagos land iguanas: no man, no resistance. PLoS ONE. (2010) 5:e8989. doi: 10.1371/journal.pone.0008989
20. Chaprazov T, Dimitrov R, Stamatova-Yovcheva K. Oral abscess associated with cranial tooth loss in green iguana (Iguana iguana). Turk J Vet Animal Sci. (2013) 37:615–7. doi: 10.3906/vet-1302-18
21. Sylvester W, Amadi V, Hegamin-Younger C, Pinckney R, Macpherson C, McKibben J, et al. Occurrence of antibiotic resistant Escherichia coli in green iguanas (Iguana iguana) in Grenada, West Indies. Int J Vet Med Res Rep. (2014) 2014:1–8. doi: 10.5171/2014.260412
22. Maria R, Ramer J, Reichard T, Tolson PJ, Christopher MM. Biochemical reference intervals and intestinal microflora of free-ranging ricord's iguanas (Cyclura ricordii). J Zoo Wildl Med. (2007) 38:414–9. doi: 10.1638/1042-7260(2007)38[414:BRIAIM]2.0.CO;2
23. Arcos-García JL, Reynoso Rosales VH, Mendoza Martínez GD, Hernández Sánchez D. Identificación del sexo y medición del crecimiento en iguana negra (ctenosaura pectinata) en las etapas de cría y juvenil. Vet México. (2005) 36:53–62.
24. American Society of Ichthyologists and Herpetologists (ASIH). Guidelines for Live Amphibians and Reptiles in Field and Laboratory Research. Lawrence, KS (2004). Available online at: https://www.asih.org/animal-care-guidelines (accessed April 1, 2019).
25. Tarr PI, Gordon CA, Chandler WL. Shiga-toxin-producing Escherichia coli and haemolytic uraemic syndrome. Lancet. (2005) 365:1073–86. doi: 10.1016/S0140-6736(05)71144-2
26. Tsai YL, Palmer CJ, Sangermano LR. Detection of Escherichia coli in sewage and sludge by polymerase chain reaction. Appl Environ Microbiol. (1993) 59:353–7. doi: 10.1128/AEM.59.2.353-357.1993
27. Gutiérrez-Jiménez J, Luna-Cazárez LM, Mendoza-Orozco MI, Díaz-Marina GJ, Burguete-Gutiérrez JC, Feliciano-Guzmán JM. Organization, maintenance, and preservation of the bacterial culture collection of the biological sciences institute, University of Science and Arts of Chiapas (UNICACH), Mexico. Revista Soc Venezolana Microbiol. (2015) 35:95–102.
28. Cerna JF, Nataro JP, Estrada-Garcia T. Multiplex PCR for detection of three plasmid-borne genes of enteroaggregative Escherichia coli strains. J Clin Microbiol. (2003) 41:2138–40. doi: 10.1128/JCM.41.5.2138-2140.2003
29. López-Saucedo C, Cerna JF, Villegas-Sepulveda N, Thompson R, Velazquez FR, Torres J, et al. Single multiplex polymerase chain reaction to detect diverse loci associated with diarrheagenic Escherichia coli. Emerg Infect Dis. (2003) 9:127–31. doi: 10.3201/eid0901.010507
30. Oswald E, Schmidt H, Morabito S, Karch H, Marches O, Caprioli A. Typing of intimin genes in human and animal enterohemorrhagic and enteropathogenic Escherichia coli: characterization of a new intimin variant. Infect Immun. (2000) 68:64–71. doi: 10.1128/IAI.68.1.64-71.2000
31. Schmidt H, Beutin L, Karch H. Molecular analysis of the plasmid-encoded hemolysin of Escherichia coli O157:H7 strain EDL 933. Infect Immun. (1995) 63:1055–61. doi: 10.1128/IAI.63.3.1055-1061.1995
32. DebRoy C, Roberts E, Valadez AM, Dudley EG, Cutter CN. Detection of Shiga toxin-producing Escherichia coli O26, O45, O103, O111, O113, O121, O145, and O157 serogroups by multiplex polymerase chain reaction of the wzx gene of the O-antigen gene cluster. Foodborne Pathog Dis. (2011) 8:651–2. doi: 10.1089/fpd.2010.0769
33. Paton AW, Paton JC. Detection and characterization of Shiga toxigenic Escherichia coli by using multiplex PCR assays for stx1, stx2, eaeA, enterohemorrhagic E. coli hlyA, rfbO111, and rfbO157. J Clin Microbiol. (1998) 36:598–602. doi: 10.1128/JCM.36.2.598-602.1998
34. Clermont O, Christenson JK, Denamur E, Gordon DM. The clermont Escherichia coli phylo-typing method revisited: improvement of specificity and detection of new phylo-groups. Environ Microbiol Rep. (2013) 5:58–65. doi: 10.1111/1758-2229.12019
35. CLSI. Performance Standards for Antimicrobial Disk Susceptibility Tests, Approved Standard. Wayne, IL: Clinical and Laboratory Standards Institute (2015).
36. Magiorakos AP, Srinivasan A, Carey RB, Carmeli Y, Falagas ME, Giske CG, et al. Multidrug-resistant, extensively drug-resistant and pandrug-resistant bacteria: an international expert proposal for interim standard definitions for acquired resistance. Clin Microbiol Infect. (2012) 18:268–81. doi: 10.1111/j.1469-0691.2011.03570.x
37. Smati M, Clermont O, Bleibtreu A, Fourreau F, David A, Daubie AS, et al. Quantitative analysis of commensal Escherichia coli populations reveals host-specific enterotypes at the intra-species level. Microbiologyopen. (2015) 4:604–15. doi: 10.1002/mbo3.266
38. García-Mazcorro JF, Garza-González E, Marroquín-Cardona AG, Tamayo JL. Caracterización, influencia y manipulación de la microbiota gastrointestinal en salud y enfermedad. Gastroenterol Hepatol. (2015) 38:445–66. doi: 10.1016/j.gastrohep.2015.01.004
39. Benites NR, Pessoa C, Bandini L, Saidenberg A, Moreno A, Sakata S, et al. Microbiota bacteriana e fúngica presentes na cloaca de jabutis-piranga (Geochelone carbonaria) criados em domicílio. Vet Zoo. (2013) 20:102–110.
40. Bastos HM, Lopes LFL, Gattamorta MA, Matushima ER. Prevalence of enterobacteria in bothrops jararaca in São Paulo State: microbiological survey and antimicrobial resistance standards. Acta Sci Biol Sci. (2008) 30:321–6. doi: 10.4025/actascibiolsci.v30i3.536
41. Nagy B, Fekete PZ. Enterotoxigenic Escherichia coli (ETEC) in farm animals. Vet Res. (1999) 30:259–84.
42. Martins FH, Guth BE, Piazza RM, Leao SC, Ludovico A, Ludovico MS, et al. Diversity of Shiga toxin-producing Escherichia coli in sheep flocks of Parana State, Southern Brazil. Vet Microbiol. (2015) 175:150–6. doi: 10.1016/j.vetmic.2014.11.003
43. Abubakar RH, Madoroba E, Adenubi O, Morar-Leather D, Fasina FO. Bacterial pathogens of pigs with particular reference to Escherichia coli: a systematic review and meta-analysis. J Vet Med Animal Health. (2017) 9:159–85. doi: 10.5897/JVMAH2017.0594
44. Mayer J, Bays TB. Reptile behavior In: Bays TB, Lightfoot T, Mayer J, editors. Exotic Pet Behavior. Saint Louis, MO: WB Saunders (2006). p. 103–62.
45. Gutierrez-Jimenez J, Luna-Cazares LM, Cruz LM, De Aquino-Lopez JA, Sandoval-Gomez D, Leon-Ortiz AT, et al. Children from a rural region in the Chiapas Highlands, Mexico, show an increased risk of stunting and intestinal parasitoses when compared with urban children. Bol Med Hosp Infant Mex. (2019) 76:18–26. doi: 10.24875/BMHIME.M19000051
46. Koteswar B, Reddy AD, Ravi G, Karunasagar I. Occurrence of pathotypes of Escherichia coli in aquatic environment. Int J Curr Microbiol App Sci. (2017) 6:3266–75. doi: 10.20546/ijcmas.2017.609.402
47. Zhou Y, Zhu X, Hou H, Lu Y, Yu J, Mao L, et al. Characteristics of diarrheagenic Escherichia coli among children under 5 years of age with acute diarrhea: a hospital based study. BMC Infect Dis. (2018) 18:63. doi: 10.1186/s12879-017-2936-1
48. Orth D, Grif K, Khan AB, Naim A, Dierich MP, Wurzner R. The Shiga toxin genotype rather than the amount of Shiga toxin or the cytotoxicity of Shiga toxin in vitro correlates with the appearance of the hemolytic uremic syndrome. Diagn Microbiol Infect Dis. (2007) 59:235–42. doi: 10.1016/j.diagmicrobio.2007.04.013
49. Adesiyun AA. Absence of Escherichia coli O157 in a survey of wildlife from Trinidad and Tobago. J Wildl Dis. (1999) 35:115–20. doi: 10.7589/0090-3558-35.1.115
50. Perelle S, Dilasser F, Grout J, Fach P. Detection by 5'-nuclease PCR of Shiga-toxin producing Escherichia coli O26, O55, O91, O103, O111, O113, O145 and O157:H7, associated with the world's most frequent clinical cases. Mol Cell Probes. (2004) 18:185–92. doi: 10.1016/j.mcp.2003.12.004
51. Valilis E, Ramsey A, Sidiq S, DuPont HL. Non-O157 Shiga toxin-producing Escherichia coli-A poorly appreciated enteric pathogen: systematic review. Int J Infect Dis. (2018) 76:82–7. doi: 10.1016/j.ijid.2018.09.002
52. Kumar N, Nahid F, Zahra R. Association of virulence factors, phylogenetic groups and antimicrobial resistance markers in Escherichia coli from Badin city, Pakistan. J Chemother. (2017) 29:8–13. doi: 10.1080/1120009X.2016.1154682
53. Kwong JC, McCallum N, Sintchenko V, Howden BP. Whole genome sequencing in clinical and public health microbiology. Pathology. (2015) 47:199–210. doi: 10.1097/PAT.0000000000000235
54. Amezquita-Lopez BA, Quinones B, Soto-Beltran M, Lee BG, Yambao JC, Lugo-Melchor OY, et al. Antimicrobial resistance profiles of Shiga toxin-producing Escherichia coli O157 and Non-O157 recovered from domestic farm animals in rural communities in Northwestern Mexico. Antimicrob Resist Infect Control. (2016) 5:1. doi: 10.1186/s13756-015-0100-5
55. Furlan JPR, Gallo IFL, de Campos A, Navarro A, Kobayashi RKT, Nakazato G, et al. Characterization of non-O157 Shiga toxin-producing Escherichia coli (STEC) obtained from feces of sheep in Brazil. World J Microbiol Biotechnol. (2019) 35:134. doi: 10.1007/s11274-019-2712-z
Keywords: diarrheagenic Escherichia coli, prevalence, Iguana, Chiapas, antibiotic resistance, zoonosis
Citation: Bautista-Trujillo GU, Gutiérrez-Miceli FA, Mandujano-García L, Oliva-Llaven MA, Ibarra-Martínez C, Mendoza-Nazar P, Ruiz-Sesma B, Tejeda-Cruz C, Pérez-Vázquez LC, Pérez-Batrez JE, Vidal JE and Gutiérrez-Jiménez J (2020) Captive Green Iguana Carries Diarrheagenic Escherichia coli Pathotypes. Front. Vet. Sci. 7:99. doi: 10.3389/fvets.2020.00099
Received: 10 July 2019; Accepted: 10 February 2020;
Published: 26 February 2020.
Edited by:
Paul Wigley, University of Liverpool, United KingdomReviewed by:
Steven Lee Foley, National Center for Toxicological Research (FDA), United StatesKenneth James Genovese, United States Department of Agriculture, United States
Copyright © 2020 Bautista-Trujillo, Gutiérrez-Miceli, Mandujano-García, Oliva-Llaven, Ibarra-Martínez, Mendoza-Nazar, Ruiz-Sesma, Tejeda-Cruz, Pérez-Vázquez, Pérez-Batrez, Vidal and Gutiérrez-Jiménez. This is an open-access article distributed under the terms of the Creative Commons Attribution License (CC BY). The use, distribution or reproduction in other forums is permitted, provided the original author(s) and the copyright owner(s) are credited and that the original publication in this journal is cited, in accordance with accepted academic practice. No use, distribution or reproduction is permitted which does not comply with these terms.
*Correspondence: Javier Gutiérrez-Jiménez, javier.gutierrez@unicach.mx