- MOE Joint International Research Laboratory of Animal Health and Food Safety, College of Veterinary Medicine, Nanjing Agricultural University, Nanjing, China
Intestinal microbiota is necessary for the guarantee of intestinal mucosal barrier. However, the detailed effect of probiotics on porcine intestinal development, especially in the early life, is still unclear. In this study, we treated 3-day-old newborn piglets with Lactobacillus reuteri (L. reuteri) D8 and observed its beneficial effect on piglets. The body weights, villus height, and crypt depth of jejunum were all significantly increased after L. reuteri treatment in piglets. L. reuteri also significantly increased the proliferation index of PCNA+ cells in the crypt, as well as c-Myc and Tcf4 expressions. Furthermore, L. reuteri also enhanced intestinal mucosal barrier with the increase of goblet cells and antimicrobial peptides (AMPs) expressions of Muc2, Lyz1, and pBD1. The well development of Peyer's patches and increased number of CD3+ T cells, combined with increased expression of IL-4 and IFN-γ, also demonstrated the immune stimulation effect of L. reuteri D8. This study demonstrated that L. reuteri promotes the development of intestine mucosal system and maintains intestinal mucosal barrier in newborn piglets.
Introduction
Piglets diarrhea with intestinal inflammation and epithelial damage is a common problem and thus contributes to morbidity and mortality among piglets, particularly in intensive farming systems (1). The development of the gastrointestinal tract in newborn piglets is a very sophisticated process, which starts during prenatal life and continues after birth. The intestinal development of newborn piglets is not perfect and vulnerable to the invasion of various pathogens (2). Numerous studies have shown high morbidity of diarrhea and intestinal inflammation in piglets, resulting in the death of piglets and directly causing great economic losses (3, 4). The addition of antibiotics to livestock and poultry feed can significantly improve the feed conversion rate, the growth rate, and reduce the mortality rate (5–7). However, this phenomenon will cause bacterial drug resistance, seriously destroy the immune mechanism of the body, and weaken the ability of anti-virus and anti-infection. Moreover, antibiotics accumulate in the body, endangers human health, and ecological environment (8, 9).
The intestine is not only a major digestive and absorptive organ for nutrients, but also an effective barrier against various infectious agents. The intestinal mucosal barrier mainly contains mucus layers, epithelial cells, and mucosal immune system (10). The intestinal epithelium bind tightly together to form a dense protective wall, separating the intestinal contents from the intestinal tissues, and maintaining the health of intestinal tract (11). Once the intestinal mucosal barrier is destroyed or the balance of intestinal microflora is broken, it will lead to an increase of intestinal epithelial permeability, the destruction of the intestinal structure, and the displacement of pathogens that turn into other organs such as the liver, thus causing a systemic pathological response (12, 13).
Probiotics are live microbial food supplements or components of bacteria which have been shown beneficial effects on health (14). At present, probiotics are becoming substitutes for antibiotics, such as Lactobacillus, Bifidobacterium, and Bacillus. In previous study, it has been found that Lactobacillus can reduce the alkaline environment in intestinal tract, increase mucus secretion and strengthen the tight connection, so as to maintain the homeostasis of the internal environment (15–17). However, the mechanism of Lactobacillus on intestinal development and mucosal barrier in newborn piglets is not clear. Lactobacillus reuteri (L. reuteri) is one of the dominant species in the GIT of pigs (18). In this study, we hypothesis whether L. reuteri can affect intestinal stem cells and mucosal immune responses of piglets, which may provide a powerful basis for the application of L. reuteri in pig raising.
Materials and Methods
Animals and Bacteria Strains
Twelve 3-day-old piglets were purchased from Meishan Pig original breeding Farm in Jiangsu Province. The piglets were fed with sows in the laboratory animal room of Nanjing Agricultural University. The initial weight and health status of piglets were equal and piglets were male. L. reuteri D8 was isolated from the duodenum of pigs in our laboratory and further confirmed as L. reuteri strain through 16s RNA sequencing (GenBank: MF850249), which are tetracycline resistance. L. reuteri D8 were grown in MRS ager medium at 37°C (19). Pigs were raised in the laboratory animal room of Nanjing Agricultural University and divided into control group (6 piglets) and L. reuteri treatment group (6 piglets). The piglets were treated with 2 mL aseptic PBS or L. reuteri D8 (109 CFU) suspended in 2 mL PBS by gavage for 5 days and then sacrificed (20–22). The body weights of the pigs were recorded daily. Harvested jejunum and ileum tissues were fixed with 4% paraformaldehyde. Animal procedure was carried out in accordance with the Nanjing Agricultural University of Medicine Animal Studies Committee, which approved the protocols.
Histological Analysis
Tissue samples from the small intestine were fixed in 4% paraformaldehyde for 48 h at room temperature. After fixation, the samples were sectioned to fit glass slides and then dehydrated in a graded alcohol series. Then the tissue block is transparent in xylene, soaked in paraffin for 2 h and embedded in paraffin. The sections were serially sliced into 5-μm-thick sections, and mounted on slides. The sections were dried horizontally on a warming tray overnight at 37°C, and stained with hematoxylin-eosin (HE) for examination by light microscopy. The villus height and crypt depth of jejunum were measured (single-blind) by an observer using computer-assisted morphometry. The area of Peyer's patches (PPs) in ileum was also measured.
Immunohistochemistry
Paraffin sections were dewaxed in xylene and rehydrated in decreasing concentrations of ethanol. The sections were permeabilized with 0.5% Triton X-100 for 15 min, followed by washing three times with HBSS, then dipped in H2O2 (3%) to remove catalase. For PCNA staining, sections were incubated with anti-porcine PCNA antibody (1:100 Abcam) overnight at 4°C in a humidified box. For CD3 positive T cells staining, sections were incubated with anti-porcine CD3 (1:200 Abcam) overnight at 4°C. PBS was used instead of antibody for the control. Sections were then incubated goat against Rabbit (1:200 Boster) for 1 h at 37°C and washed. DAB color development for 5-10 min. In this study, nearly 50 sections were observed per group. PCNA positive cells and CD3 positive T cells of per crypt in jejunum were measured.
Periodic Acid-Schiff (PAS) Stain
Jejunum sections were dewaxed in xylene and rehydrated in decreasing concentrations of ethanol. Deparaffinized and rehydrated sections were treated with periodic acid for 5 min at RT. Slides were washed in distilled water then stained with Schiff's reagent for 15 min at RT, followed by a 10 min wash in running tap water. The sections were then counterstained with hematoxylin for 2 min and acid differentiation solution for 3 s. The sections were washed in running tap water for 15 min, followed by dehydration (75, 85, 95, 100%, EtOH) and cover-slipped. The number of goblets cells were counted in the jejunum.
ELISA
The collected blood was centrifuged at 8,000 rpm for 10 min. The supernatants were stored at −20°C until use. Porcine LPS (SBJ-z255, Sbjbio) and IgG (SBJ-z124, Sbjbio) in serum were measured with ELISA kits according to the manufacturer's instructions.
Real-Time PCR Quantification
RNA was extracted from jejunum tissues, which were cut into 2 mm size and put in a lapping tube fitted with 500 μL RNAios Plus (Takara), respectively. The tubes were grinded for 1 min and centrifuged at 8,000 g for 10 min at 4°C. After centrifugation, the supernatant was transferred to other tubes and 500 μL RNAios Plus (Takara) was added. Next, 200 μL of chloroform was added and mixed by hand during 15 s and placed at room temperature for 10 min. The tubes were centrifuged at 12,000 g for 15 min at 4°C. After centrifugation, the supernatant (400 μL) containing RNA was transferred to other tubes, then the same volume of isopropanol was added. The tubes were mixed by hand for 15 s and incubated overnight at −20°C. The next day, the tubes were centrifuged at 12,000 g for 20 min at 4°C. The supernatant was discarded, 1 mL of 75% ethanol was added twice and centrifuged at 12,000 g for 10 min at 4°C. Finally, 75% ethanol was discarded and the tubes was dried. The RNA precipitate was dissolved in 20 μL of RNase free water. The CDNA was synthesized by RNA, according to the instructions for the use of manufacturer's enzymes (Takara). Gene expression was assessed with a SYBR master mix (Takara). Relative mRNA expression was calculated using the ΔΔCt method. Primer sequences for real-time RT-PCR were as follows in Table 1.
Statistical Analysis
All data are expressed as means ± SD. The data were analyzed by One-Way ANOVA and Student's t-test with SPSS17.0 Software. Significance levels are indicated as *P < 0.05 and ** P < 0.01.
Results
Effects of L. reuteri D8 Treatment on Body Weight and Jejunum Morphology of Piglets
The body weights of piglets in control group and L. reuteri D8 treatment group were observed during experimental period. Compared with control group, the body weights of L. reuteri D8 treatment showed a significant increase from day 1 to day 3 (Figure 1A). L. reuteri D8 didn't affect LPS levels in serum compared to the control group (Figure 1B). The villus length and crypt depth of the jejunum in the L. reuteri treatment group were also longer than that in the control group (Figures 1C–E). These results demonstrated that L. reuteri D8 treatment increased body weight of piglets and promoted growth of intestinal epithelium to maintain the intestinal mucosal barrier.
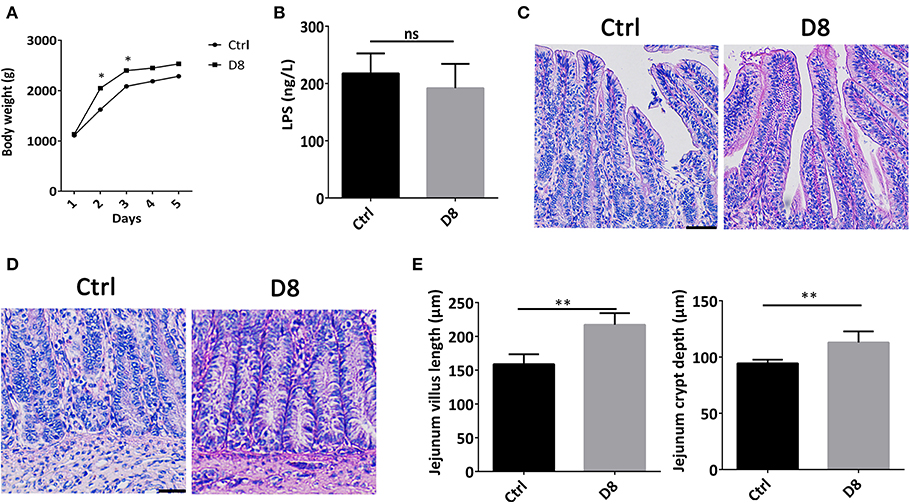
Figure 1. Effects of L. reuteri D8 treatment on body weight and jejunum morphology of piglets. (A) The body weight of piglets in the two groups were shown. The line chart indicated the body weight of piglets were measured by gram, n = 6. (B) The level of LPS in serum was detected, n = 6. (C,D) HE staining of piglet jejunum treated with PBS or L. reuteri D8, respectively, n = 50 villus or crypts per group. Scale bars, 50 μm. (E) Statistical graph of jejunal villus and crypt depth in piglets. Data were expressed as mean ± standard deviation (means ± SD). *P < 0.05, **P < 0.01.
Proliferation Status of the Jejunum After L. reuteri D8 Administration
The jejunum was stained by PCNA immune-histochemical staining, and observed under a confocal laser microscope. The proliferative index was significantly higher in L. reuteri D8 administration compared with control group (Figure 2A). At the same time, we also found that the mRNA level of c-Myc in jejunum was slightly up-regulated (Figure 2B). After L. reuteri D8 administration, Tcf4 mRNA levels had the expected increase (Figure 2C). β-Catenin plays essential roles in the Wnt pathway by interacting with T-cell factor 4 (TCF4) to transcribe oncogenes (23). Researches showed activation of wnt/β-catenin signaling pathway can promote the proliferation of intestinal stem cells (24, 25). The data indicate that L. reuteri D8 enhanced intestinal epithelial proliferation by activation of wnt/β-catenin pathway.
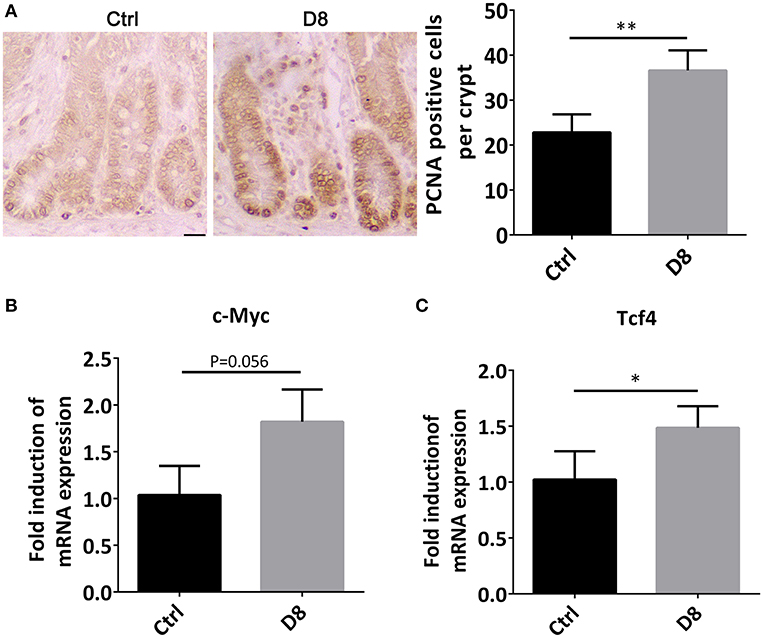
Figure 2. Proliferation status of jejunum after L. reuteri D8 administration. (A) Immunohistochemistry showed the number of anti-porcine PCNA-positive cells in the jejunal crypt of each group, n = 50 crypts per group. Scale bars, 50 μm. (B,C) Real-time PCR showed the expression of c-Myc and Tcf4 genes in the jejunum, n = 6. Data were expressed as mean ± standard deviation (means ± SD). *P < 0.05, **P < 0.01.
L. reuteri D8 Administration Improved Innate Immunity of Piglets
Intestinal stem cells can differentiate into secretory cells, such as goblet cells and Paneth cells, which can secrete antimicrobial peptides to play an important role in innate immunity against infection (26, 27). In order to verify the effect of L. reuteri D8 on immune response in piglets, we detected the number of goblet cells, the expression of Muc2 and Lyz-1. The study demonstrated that L. reuteri D8 administration significantly increased the number of goblet cells, the expression of Muc2 and Lyz-1 in jejunum (Figures 3A–C). L. reuteri D8 also significantly increased the mRNA expression level of porcine β-defensins 1 (pBD-1) in jejunum (Figure 3D). After L. reuteri D8 treatment, the expression of IL-22 mRNA was the quadruple of untreated group (Figure 3E). Based on these data, L. reuteri D8 promoted the differentiation of intestinal stem cells and stimulated innate immunity.
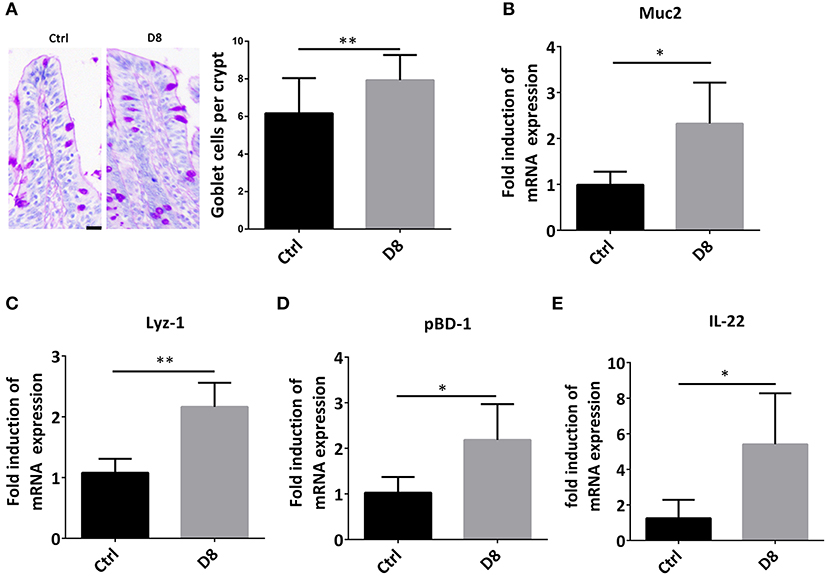
Figure 3. L. reuteri D8 administration improved innate immunity of piglets. (A) PAS staining of goblet cells in jejunum, n = 50 crypts per group. Scale bars, 25 μm. (B–E) Real-time PCR was used to determine the relative mRNA expression of muc2, Lyz-1, pBD1, and IL-22 in the jejunum treatment with PBS or L. reuteri D8, respectively, n = 6. Data were expressed as mean ± standard deviation (means ± SD). *P < 0.05, **P < 0.01.
Activation of Mucosal Immunity After L. reuteri D8 Administration in Piglets
Peyer's patch, also known as intestinal collecting lymph node, is an important part of intestinal mucosal immune system and a group of lymphoid follicles in small intestinal mucosa (28). In this study, the HE staining showed L. reuteri D8 treatment markedly increased the area of Peyer's Patches in ileum (Figure 4A). Moreover, immunohistochemistry showed that the number of CD3+ T cells observably increased in L. reuteri treatment group in jejunum (Figure 4B). Meanwhile, L. reuteri D8 treatment also increased the level of IgG antibodies in the serum (Figure 4C). IL-4 induces the differentiation of naïve CD4 T cells into Th2 cells, while IL-4 also drives the immunoglobulin (Ig) class switch to IgG1 and IgE (29). IFN-γ produced by activated Th1 cell, can regulate the immune system, resist viral and bacterial infections and tumorigenesis (30). IL-4 and IFN-γ mRNA levels had a pronounced increase, compared to the control group after L. reuteri D8 treatment (Figures 4D,E). These data suggested that L. reuteri D8 enhanced intestinal immune response.
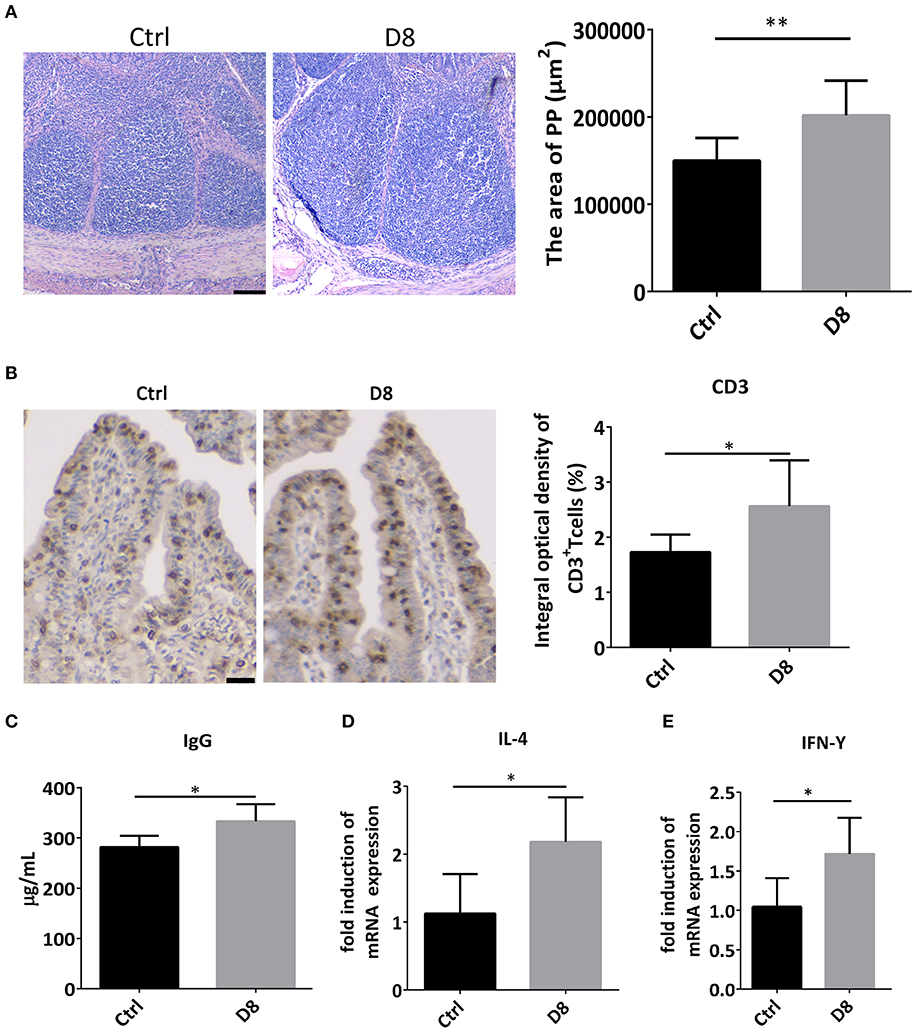
Figure 4. Expression of cytokines after L. reuteri D8 administration in piglets. (A) HE staining showed the area of the PPs of the piglet's in ileum treat with PBS or L. reuteri D8, respectively, n = 30 PPs per group. Scale bars, 200 μm. (B) Immunohistochemistry of CD3+ T cells in jejunum; n = 50 crypts per group. Scale bars, 25 μm. (C) The level of IgG antibody in serum; n = 6. (D,E) Real-time PCR detection of the gene expression levels of IL-4 and IFN-γ in jejunum, n = 6. Data were expressed as mean ± standard deviation (means ± SD). *P < 0.05, **P < 0.01.
Discussion
Probiotics has already been widely used in pig industry, accompanied with the restriction use of antibiotics. However, the detail mechanism between Lactobacillus and intestinal epithelial, especially for the intestine development in early life, is still covered. This study was conducted to investigate the effects of L. reuteri D8 in newborn pigs, with respect to growth performance, intestinal morphology, the proliferation of intestinal stem cell and development of innate and mucosal immunity. The formation of villi and crypt in the small intestine significantly enlarged the surface area of the intestinal mucosa, promoted the efficient absorption of nutrient and generated a protected stem cell niche (31–33). In neonate piglets, the integrity of the intestinal mucosal barrier has defectiveness in order to increase the absorption efficiency, especially for antibodies in milk. However, the imperfect intestine is also the infection target of pathogens, such as Escherichia coli and porcine epidemic diarrhea virus (34, 35). Stimulating the intestinal development and enhancing the intestinal mucosal ability are necessary for the health raising of pigs. In this study, we found that L. reuteri D8 could stimulate the body weight slightly with the supportive of increased villus height and crypt depth in jejunum of piglets. The stimulation effect of L. reuteri D8 on intestinal epithelial was further verified with the PCNA staining, as well as c-Myc and Tcf mRNA expressions. The increased villi height will provide more surface area of intestinal lumen to guarantee the absorption of more nutrients, which could also explain the increased body weight by L. reuteri D8 in early life.
Intestinal mucosal innate immunity constitutes the first line of defense, fundamental for the recognition and the initiation of an inflammatory response against microorganisms (36). The intestinal epithelial is composed of four cell lineages that come from a common stem cell progenitor: absorptive enterocytes, mucus-producing goblet cells, hormone-producing enteroendocrine cells, and Paneth cells, which produce antimicrobial peptides (37). In this study, we demonstrated that L. reuteri D8 successfully enhanced the number of goblet cells and the mRNA expression levels of Muc2 and Lyz-1, which are secreted by goblet cells and Paneth cells, respectively. The stimulatory effect of Lactobacillus on Muc-2 expression was consistent with previous study (38).
Besides Muc2 and lysozyme, pBD1, the first member of porcine β defensin, has a strong inhibitory activity against gram-negative bacteria (39). L. reuteri D8 also showed stimulatory effect on pBD1, as well as IL-22 expression. IL-22 serves as a communication line between the immune system and the epithelial cells in contact with the outside world (40). Previous study already demonstrated IL-22 secreted from LPLs could accelerate proliferation of intestinal epithelial, thus recovering damaged intestinal mucosa (19). Antimicrobial peptides are effector molecules of innate immunity with direct antimicrobial activity (41). Combined with this increased AMPs induced in early life of piglets, L. reuteri D8 showed a strong stimulation effect on enhancing the intestinal mucosal innate immunity. Antimicrobial factors produced by the intestinal mucosa limit the translocation of pathogenic microbes across the intestinal epithelial cell barrier (40).
The gut-associated lymphoid tissue (GALT) consists of both isolated and aggregated lymphoid follicles, while Peyer's Patches are composed by aggregated lymphoid follicles and PPs sampling of the lumen is crucial for protective mucosal immune responses (42). Here, L. reuteri D8 increased the area of PPs in ileum of piglets, which indicated the stimulation of immune tissue development and explained the enhanced serum IgG concentration. This phenomenon was further verified with the increased the number of CD3+ T cells and expression of IL-4 and IFN-γ, which are necessary for naive T cells differentiation to further increased the intestinal immunity (43, 44).
In summary, this study demonstrated that administration of L. reuteri is helpful to stimulate the intestinal development with increased intestinal villi and intestinal crypt, as well as PCNA expression. L. reuteri also increased AMPs expression and Peyer's Patches development to enhance the intestinal innate immunity for piglets in early life.
Data Availability Statement
All datasets generated for this study are included in the article/supplementary material.
Ethics Statement
The animal study was reviewed and approved by Nanjing Agricultural University of Medicine Animal Studies Committee.
Author Contributions
MW and HW were responsible for performing the experiments, data analysis, and writing the manuscript. LL and LJ provided help for the animal study. QY were responsible for the conception and design of the study, data collection, drafting the article, and final approval of the version submitted.
Funding
This study was supported by National Key R&D Program of China (2018YFE0127300), Fundamental Research Funds for the Central Universities (JCQY201906), National Natural Science Foundation of China (31972631 and 31502024), and the Priority Academic Program Development of Jiangsu Higher Education Institutions PAPD.
Conflict of Interest
The authors declare that the research was conducted in the absence of any commercial or financial relationships that could be construed as a potential conflict of interest.
References
1. Karlsson OE, Larsson J, Hayer J, Berg M., Jacobson M. The intestinal eukaryotic virome in healthy and diarrhoeic neonatal piglets. PLoS ONE. (2016) 11:e0151481. doi: 10.1371/journal.pone.0151481
2. Pluske JR, Turpin DL, Kim JC. Gastrointestinal tract(gut) health in the young pig. Anim Nutr. (2018) 4:187–96. doi: 10.1016/j.aninu.2017.12.004
3. Sorensen MT, Vestergaard EM, Jensen SK, Lauridsen C, Hojsgaard S. Performance and diarrhoea in piglets following weaning at seven weeks of age: challenge with E. coli O 149 and effect of dietary factors. Livestock Sci. (2009) 123:314–21. doi: 10.1016/j.livsci.2008.12.001
4. Rhouma M, Fairbrother JM, Beaudry F, Letellier A. Post weaning diarrhea in pigs: risk factors and non-colistin-based control strategies. Acta Vet Scand. (2017) 59:31. doi: 10.1186/s13028-017-0299-7
5. Allen HK, Levine UY, Looft T, Bandrick M, Casey TA. Treatment, promotion, commotion: antibiotic alternatives in food-producing animals. Trends Microb. (2013) 21:114–9. doi: 10.1016/j.tim.2012.11.001
6. Chattopadhyay MK. Use of antibiotics as feed additives: a burning question. Front Microbiol. (2014) 5:334. doi: 10.3389/fmicb.2014.00334
7. Mehdi Y, Létourneau-Montminy MP, Gaucher ML, Chorfi Y, Gayatri S, Rouissi T, et al. Use of antibiotics in broiler production: global impacts and alternatives. Anim Nutr. (2018) 4:170–8. doi: 10.1016/j.aninu.2018.03.002
8. Phillips I, Casewell M, Cox T, Groot BD, Friis C, Jones R, et al. Does the use of antibiotics in food animals pose a risk to human health? A critical review of published data. J Antimicrob Chemother. (2004) 53:28–52. doi: 10.1093/jac/dkg483
9. Tang KL, Caffrey NP, Nóbrega DB, Cork SC, Ronksley PE, Barkema HW, et al. Restricting the use of antibiotics in food-producing animals and its associations with antibiotic resistance in food-producing animals and human beings: a systematic review and meta-analysis. Lancet Planetary Health. (2017) 1:e316–27. doi: 10.1016/S2542-5196(17)30141-9
10. Turner JR. Intestinal mucosal barrier function in health and disease. Nat Rev Immunol. (2009) 9:799–809. doi: 10.1038/nri2653
11. Vindigni SM, Zisman TL, Suskind DL, Damman CJ. The intestinal microbiome, barrier function, and immune system in inflammatory bowel disease: a tripartite pathophysiological circuit with implications for new therapeutic directions. Therap Adv Gastroenterol. (2016) 9:606. doi: 10.1177/1756283X16644242
12. Pickert G, Neufert C, Leppkes M, Zheng Y, Wittkopf N, Warntjen M, et al. STAT3 links IL-22 signaling in intestinal epithelial cells to mucosal wound healing. J Exp Med. (2009) 206:1465–72. doi: 10.1084/jem.20082683
13. Amar J, Chabo C, Waget A, Klopp P, Vachoux C, Bermúdez-Humarán LG, et al. Intestinal mucosal adherence and translocation of commensal bacteria at the early onset of type 2 diabetes: molecular mechanisms and probiotic treatment. Embo Mol Med. (2011) 3:559–72. doi: 10.1002/emmm.201100159
14. Yu QH, Yuan LX, Deng J, Yang Q. Lactobacillus protects the integrity of intestinal epithelial barrier damaged by pathogenic bacteria. Front Cell Infect Microbiol. (2015) 5:26. doi: 10.3389/fcimb.2015.00026
15. Heeney DD, Gareau MG, Marco ML. Intestinal Lactobacillus in health and disease, a driver or just along for the ride? Curr Opin Biotechnol. (2017) 49:140. doi: 10.1016/j.copbio.2017.08.004
16. Wu H, Ye L, Lu X, Xie S, Yang Q, Yu Q. Lactobacillus acidophilus alleviated salmonella-induced goblet cells loss and colitis by notch pathway. Mol Nutr Food Res. (2018) 62:e1800552. doi: 10.1002/mnfr.201800552
17. Xie S, Zhao S, Jiang L, Lu L, Yang Q, Yu Q. Lactobacillus reuteri stimulates intestinal epithelial proliferation and induces differentiation into goblet cells in young chickens. J Agric Food Chem. (2019) 67:13758–66. doi: 10.1021/acs.jafc.9b06256
18. Oh PL, Benson AK, Peterson DA, Patil PB, Moriyama EN, Roos S, et al. Diversification of the gut symbiont Lactobacillus reuteri as a result of host-driven evolution. ISME J. (2010) 4:377–87. doi: 10.1038/ismej.2009.123
19. Hou QH, Ye LL, Liu HF, Huang LL, Yang Q, Turner JR, et al. Lactobacillus accelerates ISCs regeneration to protect the integrity of intestinal mucosa through activation of STAT3 signaling pathway induced by LPLs secretion of IL-22. Cell Death Different. (2018) 25:1657–70. doi: 10.1038/s41418-018-0070-2
20. Zhang JH, Deng J, Li YF, Yang Q. The effect of Lactobacillus on the expression of porcine beta-defensin-2 in the digestive tract of piglets. Livestock Sci. (2011) 138:259–65. doi: 10.1016/j.livsci.2011.01.001
21. Suo C, Yin Y, Wang X. Effects of lactobacillus plantarum ZJ316 on pig growth and pork quality. BMC Vet Res. (2012) 8:1746–6148. doi: 10.1186/1746-6148-8-89
22. Sayan H, Assavacheep P, Angkanaporn K, Assavacheep A. Effect of Lactobacillus salivarius on growth performance, diarrhea incidence, fecal bacterial population and intestinal morphology of suckling pigs challenged with F4+ enterotoxigenic Escherichia coli. Asian Austr J Anim Sci. (2018) 31:1308–14. doi: 10.5713/ajas.17.0746
23. Shin SH, Lim DY, Reddy K, Malakhova M, Liu FF, Wang T, et al. A small molecule inhibitor of the beta-catenin-TCF4 interaction suppresses colorectal cancer growth in vitro and in vivo. Ebiomedicine. (2017) 25:22–31. doi: 10.1016/j.ebiom.2017.09.029
24. Pinto D, Gregorieff A, Begthel H, Clevers H. Canonical Wnt signals are essential for homeostasis of the intestinal epithelium. Genes Dev. (2003) 17:1709–13. doi: 10.1101/gad.267103
25. MacDonald BT, Tamai K, He X. Wnt/β-catenin signaling: components, mechanisms, and diseases. Dev Cell. (2009) 17:9–26. doi: 10.1016/j.devcel.2009.06.016
26. Elphick DA, Mahida YR. Paneth cells: their role in innate immunity and inflammatory disease. Gut. (2005) 54:1802–9. doi: 10.1136/gut.2005.068601
27. Muniz LR, Knosp C, Yeretssian G. Intestinal antimicrobial peptides during homeostasis, infection, and disease. Front Immunol. (2012) 3:310. doi: 10.3389/fimmu.2012.00310
28. Da SC, Camille W, Johnny B, Jean-Pierre G, Hugues L. The Peyer's patch mononuclear phagocyte system at steady state and during infection. Front Immunol. (2017) 8:1254. doi: 10.3389/fimmu.2017.01254
29. Junttila IS. Tuning the cytokine responses: an update on interleukin (IL)-4 and IL-13 receptor complexes. Front Immunol. (2018) 9:888. doi: 10.3389/fimmu.2018.00888
30. Hu X, Ivashkiv LB. Cross-regulation of signaling pathways by interferon-γ: implications for immune responses and autoimmune diseases. Immunity. (2009) 31:539–50. doi: 10.1016/j.immuni.2009.09.002
31. Pott J, Hornef M. Innate immune signalling at the intestinal epithelium in homeostasis and disease. EMBO Rep. (2012) 13:684–98. doi: 10.1038/embor.2012.96
32. Kiela PR, Ghishan FK. Physiology of intestinal absorption and secretion. Best Pract Res Clin Gastroenterol. (2016) 30:145–59. doi: 10.1016/j.bpg.2016.02.007
33. Walton KD, Freddo AM, Wang S, Gumucio DL. Generation of intestinal surface: an absorbing tale. Development. (2016) 143:2261–72. doi: 10.1242/dev.135400
34. Curry SM, Schwartz KJ, Yoon KJ, Gabler NK, Burrough ER. Effects of porcine epidemic diarrhea virus infection on nursery pig intestinal function and barrier integrity. Vet Microbiol. (2017) 211:58–66. doi: 10.1016/j.vetmic.2017.09.021
35. Sun Y, Kim SW. Intestinal challenge with enterotoxigenic Escherichia coli in pigs, and nutritional intervention to prevent postweaning diarrhea. Anim Nutr. (2017) 3:322–30. doi: 10.1016/j.aninu.2017.10.001
36. Elia PP, Tolentino YFM, Bernardazzi C, de Souza HSP. The role of innate immunity receptors in the pathogenesis of inflammatory bowel disease. Mediat Inflamm. (2015) 2015:936193. doi: 10.1155/2015/936193
37. Santaolalla R, Abreu MT. Innate immunity in the small intestine. Curr Opin Gastroenterol. (2012) 28:124–9. doi: 10.1097/MOG.0b013e3283506559
38. Wang L, Cao H, Liu L, Wang B, Walker WA, Acra SA, et al. Activation of epidermal growth factor receptor mediates mucin production stimulated by p40, a Lactobacillus rhamnosus GG-derived protein. J Biol Chem. (2014) 289:20234–44. doi: 10.1074/jbc.M114.553800
39. Zhang GL, Wu H, Shi JS, Ganz T, Ross CR, Blecha F. Molecular cloning and tissue expression of porcine beta-defensin-1. Febs Lett. (1998) 424:37–40. doi: 10.1016/S0014-5793(98)00134-3
40. Kinnebrew MA, Pamer EG. Innate immune signaling in defense against intestinal microbes. Immunol Rev. (2012) 245:113–31. doi: 10.1111/j.1600-065X.2011.01081.x
41. Sechet E, Telford E, Bonamy C, Sansonetti PJ, Sperandio B. Natural molecules induce and synergize to boostexpression of the human antimicrobial peptide beta-defensin-3. Proc Natl Acad Sci USA. (2018) 115:E9869–78. doi: 10.1073/pnas.1805298115
42. Jung C, Hugot JP, Barreau F. Peyer's patches: the immune sensors of the intestine. Int J Inflamm. (2010) 2010:823710. doi: 10.4061/2010/823710
43. Bhat P, Leggatt G, Waterhouse N, Frazer IH. Interferon-gamma derived from cytotoxic lymphocytes directly enhances their motility and cytotoxicity. Cell Death Dis. (2017) 8:e2836. doi: 10.1038/cddis.2017.67
Keywords: Lactobacillus, piglets, intestinal development, mucosal immunity, proliferation
Citation: Wang M, Wu H, Lu L, Jiang L and Yu Q (2020) Lactobacillus reuteri Promotes Intestinal Development and Regulates Mucosal Immune Function in Newborn Piglets. Front. Vet. Sci. 7:42. doi: 10.3389/fvets.2020.00042
Received: 17 September 2019; Accepted: 16 January 2020;
Published: 07 February 2020.
Edited by:
Rajesh Jha, University of Hawaii at Manoa, United StatesReviewed by:
Xiao Xu, Wuhan Polytechnic University, ChinaAlessandra Pelagalli, University of Naples Federico II, Italy
Xin Jian Lei, Northwest A&F University, China
Copyright © 2020 Wang, Wu, Lu, Jiang and Yu. This is an open-access article distributed under the terms of the Creative Commons Attribution License (CC BY). The use, distribution or reproduction in other forums is permitted, provided the original author(s) and the copyright owner(s) are credited and that the original publication in this journal is cited, in accordance with accepted academic practice. No use, distribution or reproduction is permitted which does not comply with these terms.
*Correspondence: Qinghua Yu, eXVxaW5naHVhMTk4MUBuamF1LmVkdS5jbg==