- 1Department of Veterinary Clinical Sciences, College of Veterinary Medicine, Iowa State University, Ames, IA, United States
- 2Department of Biomedical Sciences, SMART Pharmacology, College of Veterinary Medicine, Iowa State University, Ames, IA, United States
- 3Lloyd Veterinary Medical Center, College of Veterinary Medicine, Iowa State University, Ames, IA, United States
The study aimed to determine the impact of drop size on tear film pharmacokinetics and assess important physiological parameters associated with ocular drug delivery in dogs. Two separate experiments were conducted in eight healthy Beagle dogs: (i) Instillation of one drop (35 μl) or two drops (70 μl) of 1% fluorescein solution in each eye followed by tear collections with capillary tubes from 0 to 180 min; (ii) Instillation of 10 to 100 μl of 0.1% fluorescein in each eye followed by external photography with blue excitation filter (to capture periocular spillage of fluorescein) and tear collections from 1 to 20 min (to capture tear turnover rate; TTR). Fluorescein concentrations were measured in tear samples with a fluorophotometer. The TTR was estimated based upon non-linear mixed-effects analysis of fluorescein decay curves. Tear film pharmacokinetics were not superior with instillation of two drops vs. one drop based on tear film concentrations, residual tear fluorescence, and area under the fluorescein-time curves (P ≥ 0.163). Reflex TTR varied from 20.2 to 30.5%/min and did not differ significantly (P = 0.935) among volumes instilled (10–100 μl). The volumetric capacity of the canine palpebral fissure (31.3 ± 8.9 μl) was positively correlated with the palpebral fissure length (P = 0.023). Excess solution was spilled over the periocular skin in a volume-dependent manner, predominantly in the lower eyelid, medial canthus and lateral canthus. In sum, a single drop is sufficient for topical administration in dogs. Any excess is lost predominantly by spillage over the periocular skin as well as accelerated nasolacrimal drainage.
Introduction
Topical administration is the route of choice for treating diseases that affect the anterior segment of the eye (1, 2). This route is simple, convenient, non-invasive, and allows for the use of relatively high drug concentrations at the target tissue while minimizing systemic exposure (1, 2). One of the main challenges associated with topical administration, however, remains the poor bioavailability of therapeutic drugs to the inner tissues of the eye given rapid precorneal loss from reflex blinking and efficient nasolacrimal drainage (3–5). Optimization of eyedrop delivery can enhance therapeutic benefits for the patient (2, 6), regardless of the underlying pathology (e.g., dry eye, infectious keratitis, glaucoma), yet little consensus exists on fundamental concepts such as the number of eyedrops to apply. The label of ophthalmic products often recommends to “apply one to two drops” (e.g., Optixcare®, Lotemax®), while diverse publications in veterinary and human ophthalmology describe the use of either “1 drop” (7, 8), “1 to 2 drops” (9, 10), or “2 drops” (11, 12).
The volume of solution instilled through topical administration is known to influence the precorneal residence time, a key parameter in ocular pharmacology (5, 13). A prolonged contact time between the solution and the ocular surface is often desired, as it enhances drug bioavailability and permits longer intervals between instillations (6). In humans, best practices for ocular delivery often recommend a single drop of commercial preparations (~35 μl) per dosing session (2, 5, 6), as the maximum volume that the human palpebral fissure can hold without overflowing is estimated at 25–30 μl (1, 14). Any excess is rapidly lost via nasolacrimal drainage and spillage over the eyelashes and periocular skin (6, 15); therefore, a second drop does not provide any therapeutic advantage in humans and may in fact be counterproductive by increasing systemic absorption and the risk of associated adverse effects. In rabbits, a single drop is also sufficient as the lacrimal drainage rate is proportional to the volume of solution instilled (up to 50 μl), hence tear film drug concentrations decrease less rapidly with lower instilled volumes (13, 16). In fact, the smaller the instilled volume, the greater the fraction of applied dose that is absorbed inside the rabbit's eye (13, 16). Similar findings may be true in dogs, albeit direct extrapolation between species is not possible given important differences in ocular anatomy and physiology. In particular, the canine tear volume (65.3 μl) (17) is nearly 9-fold larger than humans (7.0 μl) (14) and rabbits (7.5 μl) (13), while the canine tear turnover rate is comparable to humans (12.2%/min vs. 10–20%/min, respectively) (17, 18) but faster than rabbits (7.1%/min) (13).
The primary objective of this study was to determine the influence of volume instilled via topical administration (i.e., one vs. two drops) on tear film kinetics of fluorescein in dogs. Given the aforementioned species differences in tear film dynamics, we originally hypothesized that the kinetic profile would be superior following instillation of two drops in canine eyes, a hypothesis that was proven to be wrong. Hence, to explain why a single eyedrop is deemed sufficient in dogs, a secondary objective was to determine the maximal volume that the canine palpebral fissure can hold, as well as the drainage rate relative to diverse volumes (10–100 μl) instilled onto the canine ocular surface. The present work focuses on canine-specific ocular physiology, providing valuable information to veterinary practitioners, pet owners, and scientists working with dogs as a translational animal model for ocular surface diseases.
Materials and Methods
Animals
Eight Beagle dogs (four neutered male, four spayed female) were included in the study, all confirmed to be healthy based on physical and ophthalmic examinations performed by a board-certified veterinary ophthalmologist (LS), including Schirmer tear test-1 (Eye Care Product Manufacturing, LLC, Tucson, AZ, USA), rebound tonometry (TonoVet, Icare Finland Oy, Espoo, Finland), slit-lamp biomicroscopy (SL-17; Kowa Company, Ltd., Tokyo, Japan), and indirect ophthalmoscopy (Keeler Vantage; Keeler Instruments, Inc., Broomall, PA, USA). All dogs were 3.0–3.5 years old and weighed 7.5–10 kg. The study was approved by the Institutional Animal Care and Use Committee of Iowa State University (IACUC #18-398) and was conducted in accordance with the Association for Research in Vision and Ophthalmology statement for the use of animals in ophthalmic and vision research.
Tear Film Fluorescein Following Instillation of One vs. Two Drops
A 1% fluorescein concentration was obtained by mixing 10% fluorescein solution (Akorn Inc., Lake Forest, IL, USA) with 1.4% polyvinyl alcohol lubricating eye drops (Artificial Tears, Rugby, Rockville Center, NY, USA). On Day 1, one eye in each dog was randomly selected (Excel software, Microsoft Corp., Redmond, WA, USA) to receive 35 μl (one drop) of 1% fluorescein solution while the contralateral eye received 70 μl (two drops) of the same solution, using a pipette (Eppendorf Reference® 2, 10–100 μl) for accuracy. On Day 2 (24 h later), the order of eyes was reversed and the experiment was repeated. Of note, the volume chosen for a single drop (35 μl) approximates the average drop size of commercial ophthalmic preparations used in veterinary and human medicine (35–39 μl) (19, 20) and is routinely described in previous scientific publications (4, 8, 21, 22). Following topical instillation, tear fluid was collected in each eye with a 2-μl capillary glass tube (Drummond Scientific Co., Broomhall, PA, USA) at the following time points: 0 min (i.e., immediately after instillation and spontaneous blinking), 1, 5, 10, 20, 30, 40, 50, 60, 90, 120, and 180 min. The capillary tube was placed against the inferior tear lake for ≤ 2 s, a duration sufficient to collect tear fluid by capillary action while minimizing the risk of inadvertent ocular irritation and reflex tearing. Given the rapid collection time (<2 s), the lack of blinking during collection (eyelids manually opened), and the relatively large tear volume in dogs (~65 μl) (17), the authors believe that it is unlikely for reflex tearing, if any, to affect tear fluorescein concentrations in a significant manner. The length of fluid contained within each capillary tube was measured to the nearest millimeter using a ruler, a value used to calculate the volume of fluid collected (as 32 mm equates to 2 μl). The collected fluid was then expelled into a 2-ml Eppendorf tube that contained 500 μl of phosphate buffered saline (Gibco® PBS, pH 7.2, Thermo Fisher Scientific, Rockford, IL, USA), vortexed for 30 s, and transferred to a cuvette for analysis. Fluorescein concentrations were measured (in ng/ml) with a computerized scanning ocular fluorophotometer (Fluorotron MasterTM, Coherent Radiation, Palo Alto, CA, USA) as previously described (17), with the exception that tear fluid was diluted with 0.5 ml of PBS herein (instead of 2 ml) to improve the sensitivity of fluorescein detection (data not shown); the cuvette was slightly raised in the device's cuvette holder to account for the lower total volume.
Volumetric Capacity of the Palpebral Fissure
A 0.1% fluorescein concentration was obtained by mixing 10% fluorescein solution with 1.4% polyvinyl alcohol lubricating eye drops. Each eye received the following volumes of 0.1% fluorescein solution via pipette administration, the order being selected at random (Excel software) in each dog: 10, 20, 30, 40, 50, 60, 70, 80, 90, and 100 μl. To minimize any carry-over effect from one session to another, the eyes and periocular skin were thoroughly rinsed with eye wash (Ocusoft® Eye Wash, OcuSOFT Inc., Richmond, TX, USA) at completion of each experiment, and a 1-h break was provided between repeated instillations in each eye to allow ample time for the physiological tear film dynamics to be restored (17). At each session, within 10 s of topical instillation and spontaneous blinking, an external photograph was taken with a Nikon D90 camera to capture each eye and associated periocular skin. To enhance detection of fluorescence, the camera was equipped with a screw-on Tiffen Wratten 15 deep yellow filter (Tiffen Manufacturing, Hauppauge, NY, USA) as well as an external flash (Nikon Speedlight SB-700) covered with a blue excitation filter (SJ-4 blue color). Of note, this photographic method better highlighted 0.1% than 1% fluorescein, hence the choice of 0.1% solution for this experiment.
Tear Turnover Rate at Various Instilled Volumes
In the experiment described above, following external photography (taken ~10 s after 0.1% fluorescein instillation), tear fluid was collected with 2-μl capillary glass tubes at the following time points in each eye: 1, 2, 4, 6, 10, 15, and 20 min (17). Tear film fluorescein concentrations were measured in all samples (see above for details) and recorded in ng/ml.
Data Analysis
Fluorophotometry
First, a fluorescein calibration curve was established by analyzing a dilution series of known fluorescein concentrations in triplicate (1–10,000 ng/ml). Fluorescein concentrations in tear samples were corrected based on the resulting calibration equation (y = 19.3 + 0.9 x – 3E-05 x2) (17). Fluorescein data of each animal were inputted to Monolix® version 2019R1 (Lixoft, Orsay, France), and tear turnover rate (TTR) was derived from a non-linear mixed effects model as previously described (17), assessing both reflex (rTTR) and basal (bTTR) tear turnover rates. Selected data points were censored in Monolix when a peak of fluorescence could not be identified on the fluorophotometer reading, or if the tear fluorescein concentration did not make physiologic sense (e.g., higher fluorescein at 2 min compared with baseline) (17). Overall, 11/640 (1.7%) of all data points were left censored.
External Photography
The volumetric capacity of the palpebral fissure was calculated in each eye as the average between the lowest instilled volume that led to periocular spillage of fluorescein solution and the highest instilled volume for which all fluorescence remained on the ocular surface. For instance, a volumetric capacity of 35 μl was calculated for an eye that had spillage first noted at 40 μl of instilled solution, but no spillage was noted at 30 μl (Figure 1). When present, the location of spillage was recorded (i.e., lower eyelid, upper eyelid, medial canthus, lateral canthus), and the area of fluorescence that extended beyond the eyelids margins was delineated with the “freehand selection” tool in ImageJ 1.52a software (National Institutes of Health, Bethesda, MD, USA). The area of fluorescein spillage was recorded in mm2 (Figure 2) using a length bar specific to each eye (i.e., palpebral fissure length measured in mm with calipers).
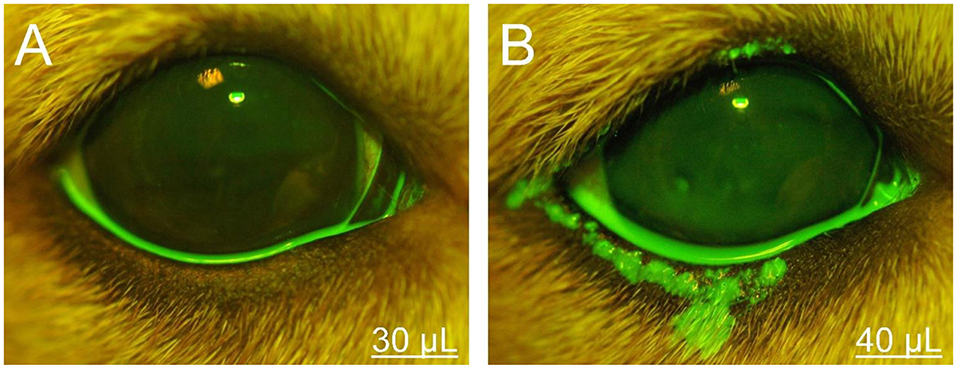
Figure 1. Photographs of the right eye and eyelids in a representative Beagle dog. The volumetric capacity of the palpebral fissure was calculated as 35 μl in this eye, based on the lack or presence of periocular spillage of 0.1% fluorescein with instillation of either 30 μl (A) or 40 μl (B), respectively.
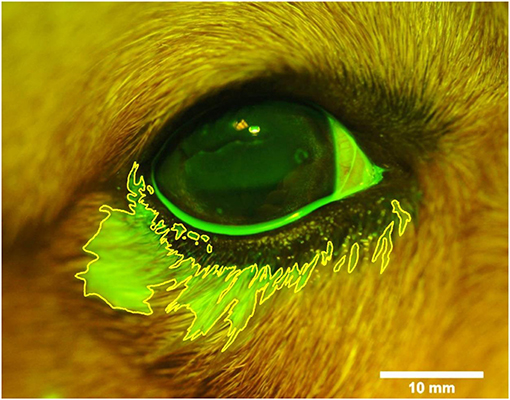
Figure 2. Photograph of the right eye and eyelids following topical instillation of 90 μl of 0.1% fluorescein in a representative Beagle dog. The area of periocular spillage was delineated with ImageJ software (version 1.52a, National Institute of Health), and recorded in mm2 based on a length bar (10 mm) specific to each eye.
Statistical Analysis
Normality of data was assessed with the Shapiro–Wilk test. A mixed model for repeated measures (MMRM) was fitted to the data using the R software version 3.6.0. In the model, fluorescein concentration was the response variable; the group (one or two drops), time (0–180 min), and group-by-time interaction were treated as fixed effects, and the animal and animal-by-group interaction were treated as random effects, using animal as block. After the model was fit, the fixed effects were tested, and comparisons between one vs. two drops were made for the following outcomes: (i) fluorescein concentration in tears at each time point, and (ii) percent of fluorescein remaining at each time point, using the baseline data of one drop for both groups in order to account for the different volumes instilled in both eyes. The R software was also used to calculate the area under the concentration-time curve (AUC), a parameter that was compared between groups (one vs. two drops) using the paired t-test. Differences among volume instilled in fluorescein periocular spillage and tear turnover rate were assessed with a one-way ANOVA, while the relationship between the volumetric capacity and the palpebral fissure length was assessed with the Pearson's correlation test. Statistical analyses were performed with SigmaPlot 14.0 (Systat software, Point Richmond, CA), and P < 0.05 were considered significant.
Results
Data were normally distributed (P > 0.05), therefore results are presented as mean ± standard deviation (range).
Volumetric Capacity of the Canine Palpebral Fissure
Mean ± SD (range) volumetric capacity of the canine palpebral fissure was 31.3 ± 8.9 μl (15–45 μl). A moderate positive correlation (r = 0.57, P = 0.023) was found between the length (in mm) and the volumetric capacity (in μl) of the palpebral fissure (Figure 3). Further, mean palpebral fissure length and volumetric capacity were slightly larger in male dogs (22.5 mm and 35 μl) compared to female dogs (22 mm and 27.5 μl), although these differences were not statistically significant (P ≥ 0.090).
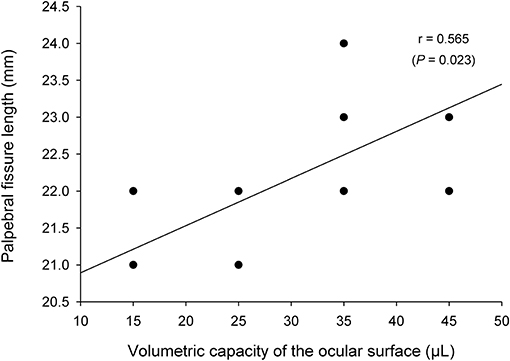
Figure 3. A positive association was found between the length and the volumetric capacity of the palpebral fissure (Pearson's correlation test).
Periocular Spillage of Instilled Solution
The lower eyelid represented the most common location (92%, Figures 1B, 2, 4A) covered by fluorescein spillage from the ocular surface, followed by the medial canthus (73%, Figure 4B), the lateral canthus (68%, Figure 4C), and the upper eyelid (32%, Figures 4E,F). Instillation of large volumes often resulted in excessive periocular spillage that covered multiple skin locations, although the amount and distribution of spillage varied within and between dogs; for instance, instillation of 100 μl of fluorescein onto the left eye of 3 different dogs resulted in either mild (Figure 4D) or pronounced (Figures 4E,F) periocular spillage. Overall, the area of periocular spillage increased as the volume of instilled solution increased (Figure 5), with statistical differences noted between 90 and 100 μl vs. 50 and 60 μl (P = 0.002), 90–100 μl vs. 30–40 μl (P < 0.001), and 70–80 μl vs. 30–40 μl (P = 0.003).
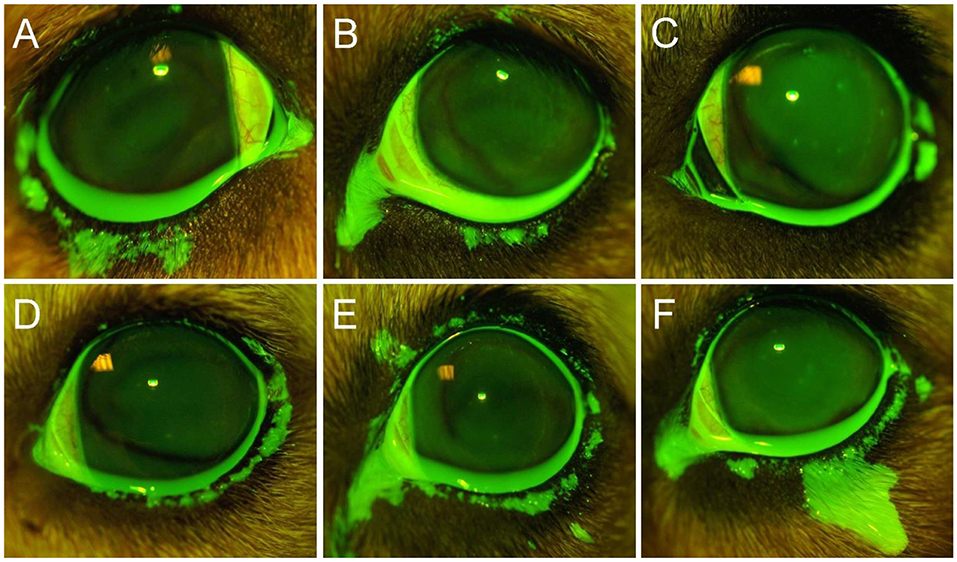
Figure 4. Representative ocular images following instillation of 60 μl (A), 80 μl (B), 40 μl (C), or 100 μl (D–F) of 0.1% fluorescein solution in different Beagle dogs. Notice the periocular spillage that predominantly affects the lower eyelid (A), medial canthus (B), lateral canthus (C), or multiple locations including the upper eyelid (D–F).
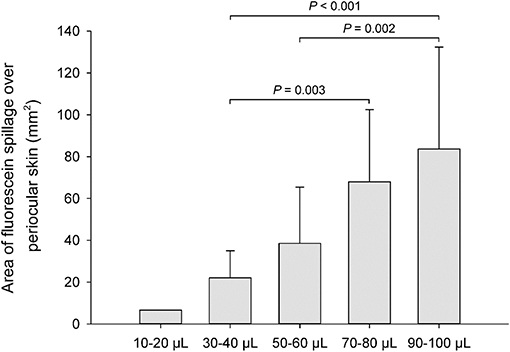
Figure 5. Bar chart depicting the mean area (+SD) of periocular spillage of 0.1% fluorescein solution, instilled at various volumes (10–100 μl) in 8 Beagle dogs (n = 16 eyes).
Tear Turnover Rate
Parameter estimation was performed using the stochastic approximation expectation maximization algorithm for non-linear mixed-effects models implemented in the Monolix Suite, as previously described for analysis of canine pharmacokinetic data (23, 24). Standard goodness-of-fit diagnostics were used to assess the validity of the model, including visual predictive checks, individual predictions vs. observations, individual weighted residuals plotted against tear fluorescein concentrations, and simulations of fluorescein vs. time disposition from 500 Monte Carlo simulations (Supplementary Appendix). Using the final mathematical model, a visual inspection of individual fluorescein decay curves showed a tendency for a “steeper” initial slope (i.e., a faster tear drainage) with increasing volumes of instilled fluorescein, as seen in a representative animal that received 10–100 μl of topical solution (Figure 6). However, the average rTTR (20.2–30.5%/min) did not vary significantly among groups (P = 0.935), nor did the bTTR (1.1–1.4%/min, P = 0.988) observed a few minutes following fluorescein instillation (Table 1).
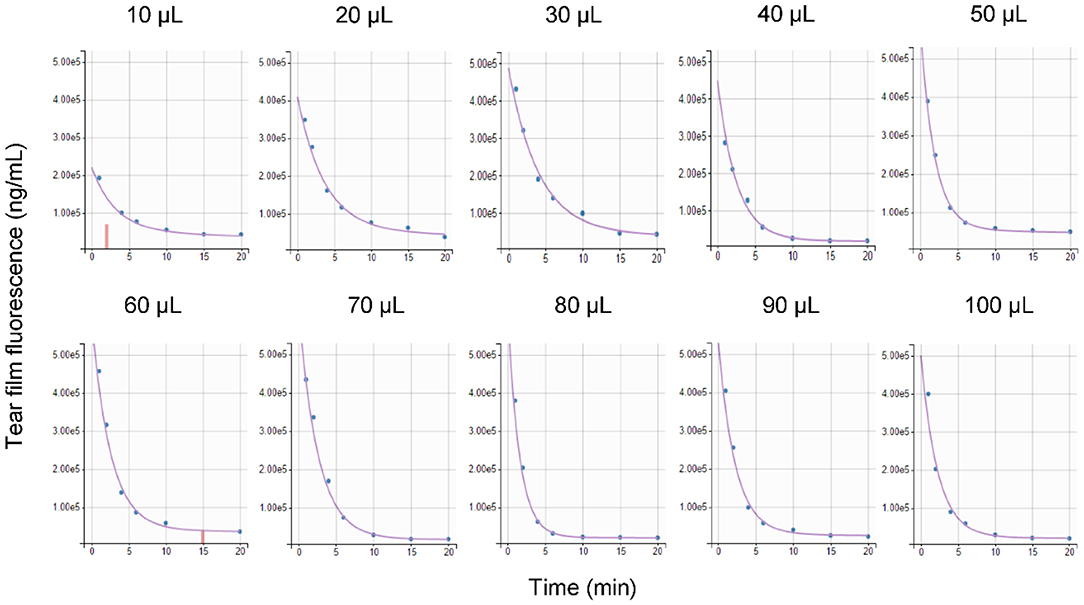
Figure 6. Comparison of predicted tear fluorescence over time (purple curve) with observed data (blue points) following topical instillation of 0.1% fluorescein solution (10–100 μl) in a representative Beagle dog. Censored data are shown as vertical red bars.

Table 1. Mean ± standard deviation of reflex tear turnover rate (rTTR) and basal tear turnover rate (bTTR) in 8 Beagle dogs following topical instillation of 10–100 μl of 0.1% fluorescein solution in each eye.
Tear Film Fluorescein Concentrations Following One vs. Two Drops
Tear film fluorescein concentrations in eyes receiving one vs. two drops are depicted in Figure 7. Immediately following instillation of fluorescein (t = 0 min), tear film concentrations were significantly higher (P = 0.046) in eyes receiving two drops (2,345 ± 237 μg/ml) compared to one drop (2,104 ± 403 μg/ml). However, no statistical differences in fluorescein concentrations were noted at t = 1 min (P = 0.163) or any subsequent time points (P ≥ 0.293). In fact, the overall exposure of the ocular surface to fluorescein (AUC of fluorescein concentration-time curve) was slightly higher in eyes receiving one drop (30,513 ± 21,530 μg*min/ml) compared to two drops (28,975 ± 17,410 μg*min/ml). However, this difference was not statistically significant (P = 0.742), and the overall effect of volume instilled on tear film fluorescein was non-significant (P = 0.619) when taking “time” into account in the model.
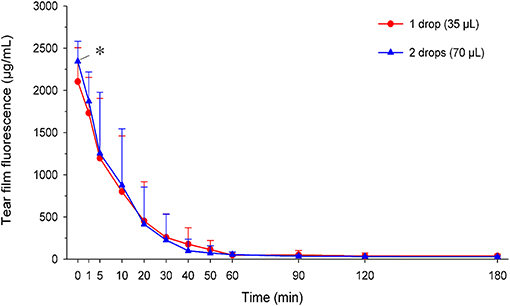
Figure 7. Scatter plot depicting the mean + SD of tear film fluorescence over time in canine eyes receiving either one drop (35 μl; red circles) or two drops (70 μl; blue triangles) of 1% fluorescein solution. Differences in tear fluorescence were noted at t = 0 min (P = 0.046) but no other time points (P ≥ 0.163).
The percentage of solution retained on the ocular surface following one vs. two drops is summarized in Figure 8. At t = 1 min, the percent retained was higher in the eyes receiving two drops (90.6 ± 16.7%) compared to one drop (81.8 ± 8.2%), a difference that approached statistical significance (P = 0.071). However, no significant differences were noted for any other time point (P ≥ 0.220) or in the overall effect of volume instilled on the percentage of solution retained (P = 0.731) when the variable “time” was taken into account.
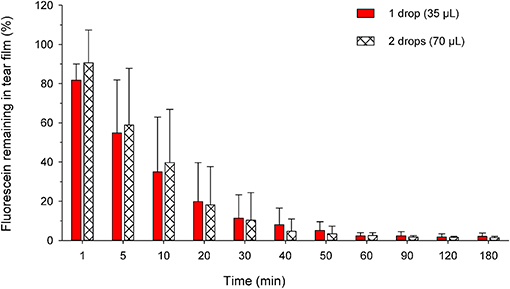
Figure 8. Bar chart depicting the mean + SD of residual tear film fluorescence at each time point in canine eyes receiving either one drop (35 μl; plain red bars) or two drops (70 μl; hatched white bars) of 1% fluorescein solution. For standardization, the residual fluorescence in both groups was compared to the tear fluorescence obtained at t = 0 min in eyes receiving a single drop of fluorescein. No statistical differences were noted between both groups at any time point (P ≥ 0.220).
Discussion
The present study supports the use of a single eyedrop in dogs, whether used therapeutically in canine patients with ocular disease or experimentally in canine models of translational research (25, 26). A second drop achieved higher tear film concentrations immediately after topical administration (t = 0 min), a finding that is partly explained by a lower dilution effect for two drops (1.9-fold) than one drop (2.9-fold) from the tear fluid present on the canine ocular surface (~65 μl) (17). However, the benefit of instilling two drops was short-lived (<1 min) and unlikely to be clinically important, although the present study focuses on fluorescein and cannot be directly extrapolated to ophthalmic drugs such as antibiotics, corticosteroids or anti-glaucoma medications. A second drop is wasted from an economic perspective and can potentially exacerbate local and/or systemic adverse effects by overflow on the periocular skin and drainage through the nasolacrimal duct, respectively. The latter was not evaluated herein as fluorescein is non-biologically active, albeit previous studies have shown that overwhelming the lacrimal system can increase the amount of drug that reaches the blood via the naso-buccal mucosa (27). In sum, the kinetic profile of fluorescein in tears was not superior with two drops vs. one drop, a finding that is often explained by an accelerated lacrimal drainage with increasing volume in both rabbits (13) and humans (3). However, this explanation is not valid in dogs as the rate of lacrimal drainage did not change significantly in our canine subjects despite a 10-fold increase in instilled volume (10–100 μl); rather, the present study shows that excessive periocular spillage is the main culprit limiting the benefit of using two topical drops in canine ophthalmology. The amount of solution that overflowed on the periocular skin was greater with larger volumes of instilled solution and primarily affected the lower eyelid, medial canthus, and lateral canthus. Such spillage can participate to local adverse effects, such as Malassezia sp. overgrowth in dogs receiving topical medications (28), or skin hyperpigmentation and lengthening of eyelashes in humans receiving topical prostaglandin analogs (29).
The volumetric capacity of the canine palpebral fissure is 31.3 μl. This value is somewhat similar to the volumetric capacity in humans (25–30 μl) (1, 14), and approximates the average volume of a single eyedrop of commercial preparations (~35 μl) (19, 20). As such, a single eyedrop is deemed sufficient in dogs and humans because their ocular surface is unable to accommodate volumes larger than ~30 μl, yet therapy with a single drop is relatively inefficient in both species given the short precorneal residence time and low ocular bioavailability (30). Several strategies can be implemented to enhance the benefits of eyedrop administration, including: (i) Eyelid closure and/or nasolacrimal punctal occlusion for several minutes following topical instillation (6, 31, 32); (ii) Higher drug concentration—Walters et al. showed that topical 1.5% levofloxacin in humans achieved tear concentrations that were 3–10 times higher than those seen with 0.3% ofloxacin at multiple time points over 24 h (11); (iii) Higher solution viscosity and/or use of mucoadhesive polymers (15, 33); (iv) Administration of a second drop ≥1 min apart from the first drop—Herring et al. showed that administration of two drops (1-min apart) of 0.5% proparacaine in dogs achieved significantly greater and longer anesthetic effect compared to eyes that received a single drop (34); and (v) Use of volumes smaller than the average commercial drop size (16, 35).
Strategies to improve ocular drug delivery should ideally be investigated in each species separately, as direct extrapolation between species is hindered by differences in ocular anatomy and physiological parameters such as blink and tear turnover rates (13, 14, 17, 36). Rabbits, for instance, have a much slower blink rate (3–6 blinks/h) (37, 38) and a slower tear drainage (7%/min) (13) compared to humans (17 blinks/min and 10–20%/min, respectively) (18, 39) as well as a different expression of mucins on the ocular surface that could affect the retention of mucoadhesive polymers (40). These differences explain why an instilled eyedrop is partially lost (20–30%) due to reflex blinking and periocular spillage in humans, but not in rabbits (15, 37), or why a solution's viscosity has a great impact on precorneal retention and drug ocular bioavailability in humans, but not in rabbits (15, 41). In a study from over four decades ago, it was recognized that “considerable reservations may be felt about comparing results from rabbits with those from humans because of the differences between the physiology of tear flow and mixing and general anatomy,” yet “the rabbit is the principal experimental animal in ophthalmology, so comparisons are needed” (42). Since then, rabbits continued to be the “species of choice” for ophthalmic studies given their availability and easy handling, yet the present study shows that dogs likely represent a more relevant model for translational research. Indeed, dogs and humans share many similarities that are relevant to ophthalmic drug delivery, although important differences exist (e.g., tear volume) (17) that should be accounted for in comparative studies. The similarities include the blink rate (14.2 vs. 17 blinks/min) (39, 43), basal TTR (12.2 10–20%/min) (17, 18), reflex TTR following eyedrop instillation (20–30 vs. 30%/min, respectively) (44), volumetric capacity of the palpebral fissure (31.3 vs. 25–30 μl) (1, 14), and periocular spillage of excess solution. The aforementioned similarities justify the use of dogs as a translational model in ophthalmic research, especially given the presence of spontaneous canine diseases that closely resemble human conditions including keratoconjunctivitis sicca (45, 46), herpetic keratitis (47), and neurotrophic keratopathy (48).
The main limitation of the study is the use of dogs from a single breed (Beagles), all being ophthalmoscopically healthy and relatively young (3–3.5 years). The tear film pharmacokinetics of two drops may be different in a larger canine breed, presumably due to differences in volumetric capacity and/or tear drainage, as shown in German Shepherd dogs using the fluorescein clearance test (20). Similarly, the ocular surface of older dogs may accommodate a larger volume due to laxity in the eyelids, and the instilled solution may be retained for longer durations due to reductions in tear volume, reflex tearing, and tear turnover rate (49, 50). In addition, the present findings do not fully represent the physiology of eyes with ocular surface disease, in which chemosis can reduce the volumetric capacity of the palpebral fissure (51), inflammation can affect tear drainage (52), and ocular absorption (53), and excessive tearing from ocular irritation can further dilute the administered solution (54). In particular, patients with inflamed nasolacrimal duct (dacryocystitis) may actually benefit from instillation of a second drop, as greater nasolacrimal drainage would theoretically be beneficial in such cases. A second limitation of the study is related to the use of sodium fluorescein as a marker for tear film kinetics. Fluorescein was shown to overestimate tear turnover in human subjects, as a portion of instilled fluorescein can be lost by conjunctival permeation and not nasolacrimal drainage (55). However, a common alternative described by other investigators (i.e., gamma scintigraphy) (56) is not applicable to dogs, in whom the general anesthesia required to hold still for the procedure would negatively impact the tear film dynamics.
The present study on drop size and tear film pharmacokinetics can be summarized as follows. Instillation of two drops provided tear film fluorescein concentrations that were higher than one drop at baseline, due to lower dilution effect from tears, although the benefits were short-lived (<1 min) and not clinically important. The kinetic profile of fluorescein in tear film was not superior in eyes receiving two drops vs. one drop as determined by the residual tear fluorescence at various time points and the overall exposure of the ocular surface (AUC) to the solution instilled. Therefore, a single standard size drop is sufficient for topical administration in dogs, a finding supported by the volumetric capacity of the canine palpebral fissure (31.3 μl). Any excess is lost predominantly by spillage over the periocular skin as well as accelerated nasolacrimal drainage.
Data Availability Statement
The datasets generated for this study are available on request to the corresponding author.
Ethics Statement
The animal study was reviewed and approved by Institutional animal care and use committee of Iowa State University.
Author Contributions
LS conceptualized and designed the study in consultation with RA and JM. LS, NK, and AR performed the experiments. LS and JM analyzed the data. All authors wrote the manuscript.
Conflict of Interest
The authors declare that the research was conducted in the absence of any commercial or financial relationships that could be construed as a potential conflict of interest.
Acknowledgments
The authors are thankful to Kimberly Phillips for proving invaluable technical support.
Supplementary Material
The Supplementary Material for this article can be found online at: https://www.frontiersin.org/articles/10.3389/fvets.2019.00457/full#supplementary-material
References
1. Davies NM. Biopharmaceutical considerations in topical ocular drug delivery. Clin Exp Pharmacol Physiol. (2000) 27:558–62. doi: 10.1046/j.1440-1681.2000.03288.x
2. Bartlett JD. Chapter 3: Ophthalmic drug delivery. In: Bartlett JD, Jaanus SD, Fiscella RG, Holdeman NR, Prokopich CL, editors. Clinical Ocular Pharmacology, 5th Edition. Saint Louism MO: Butterworth-Heinemann (2008). p. 39–52. doi: 10.1016/B978-0-7506-7576-5.50008-2
3. Friedrich SW, Cheng YL, Saville BA. Theoretical corneal permeation model for ionizable drugs. J Ocul Pharmacol. (1993) 9:229–49. doi: 10.1089/jop.1993.9.229
4. Palakuru JR, Wang J, Aquavella JV. Effect of blinking on tear volume after instillation of midviscosity artificial tears. Am J Ophthalmol. (2008) 146:920–4. doi: 10.1016/j.ajo.2008.06.020
5. Jünemann AGM, Choragiewicz T, Ozimek M, Grieb P, Rejdak R. Drug bioavailability from topically applied ocular drops. Does drop size matter? Ophthalmol J. (2016) 1:29–35. doi: 10.5603/OJ.2016.0005
6. Fraunfelder FT. Extraocular fluid dynamics: how best to apply topical ocular medication. Trans Am Ophthalmol Soc. (1976) 74:457–87.
7. Ledbetter EC, Nicklin AM, Spertus CB, Pennington MR, Van de Walle GR, Mohammed HO. Evaluation of topical ophthalmic ganciclovir gel for the treatment of dogs with experimentally induced ocular canine herpesvirus-1 infection. Am J Vet Res. (2018) 79:762–9. doi: 10.2460/ajvr.79.7.762
8. Seal JR, Robinson MR, Burke J, Bejanian M, Coote M, Attar M. Intracameral sustained-release bimatoprost implant delivers bimatoprost to target tissues with reduced drug exposure to off-target tissues. J Ocul Pharmacol Ther. (2019) 35:50–7. doi: 10.1089/jop.2018.0067
9. Hyndiuk RA, Eiferman RA, Caldwell DR, Rosenwasser GO, Santos CI, Katz HR, et al. Comparison of ciprofloxacin ophthalmic solution 0.3% to fortified tobramycin-cefazolin in treating bacterial corneal ulcers. Ciprofloxacin Bacterial Keratitis Study Group. Ophthalmology. (1996) 103:1854–62. discussion: 1862–53. doi: 10.1016/S0161-6420(96)30416-8
10. Simone JN, Pendelton RA, Jenkins JE. Comparison of the efficacy and safety of ketorolac tromethamine 0.5% and prednisolone acetate 1% after cataract surgery. J Cataract Refract Surg. (1999) 25:699–704. doi: 10.1016/S0886-3350(99)00023-1
11. Walters T, Rinehart M, Krebs W, Holdbrook M. Tear concentration and safety of levofloxacin ophthalmic solution 1.5% compared with ofloxacin ophthalmic solution 0.3% after topical administration in healthy adult volunteers. Cornea. (2010) 29:263–8. doi: 10.1097/ICO.0b013e3181b55fce
12. Leonardi A, Capobianco D, Benedetti N, Capobianco A, Cavarzeran F, Scalora T, et al. Efficacy and tolerability of ketotifen in the treatment of seasonal allergic conjunctivitis: comparison between ketotifen 0.025% and 0.05% eye drops. Ocul Immunol Inflamm. (2018) 27:1352–6. doi: 10.1080/09273948.2018.1530363
13. Chrai SS, Patton TF, Mehta A, Robinson JR. Lacrimal and instilled fluid dynamics in rabbit eyes. J Pharm Sci. (1973) 62:1112–21. doi: 10.1002/jps.2600620712
14. Mishima S, Gasset A, Klyce SD, Baum JL. Determination of tear volume and tear flow. Invest Ophthalmol. (1966) 5:264–76.
15. Zaki I, Fitzgerald P, Hardy JG, Wilson CG. A comparison of the effect of viscosity on the precorneal residence of solutions in rabbit and man. J Pharm Pharmacol. (1986) 38:463–6. doi: 10.1111/j.2042-7158.1986.tb04611.x
16. Chrai SS, Makoid MC, Eriksen SP, Robinson JR. Drop size and initial dosing frequency problems of topically applied ophthalmic drugs. J Pharm Sci. (1974) 63:333–8. doi: 10.1002/jps.2600630304
17. Sebbag L, Allbaugh RA, Wehrman RF, Uhl LK, Ben-Shlomo G, Chen T, et al. Fluorophotometric assessment of tear volume and turnover rate in healthy dogs and cats. J Ocul Pharmacol Ther. (2019) 35:497–502. doi: 10.1089/jop.2019.0038
18. Tomlinson A, Khanal S. Assessment of tear film dynamics: quantification approach. Ocul Surf. (2005) 3:81–95. doi: 10.1016/S1542-0124(12)70157-X
19. Van Santvliet L, Ludwig A. Determinants of eye drop size. Surv Ophthalmol. (2004) 49:197–213. doi: 10.1016/j.survophthal.2003.12.009
20. Oriá AP, Rebouças MF, Martins Filho E, Dórea Neto FA, Raposo AC, Sebbag L. Photography-based method for assessing fluorescein clearance test in dogs. BMC Vet Res. (2018) 14:269. doi: 10.1186/s12917-018-1593-y
21. Elibol O, Alçelik T, Yüksel N, Caglar Y. The influence of drop size of cyclopentolate, phenylephrine and tropicamide on pupil dilatation and systemic side effects in infants. Acta Ophthalmol Scand. (1997) 75:178–80. doi: 10.1111/j.1600-0420.1997.tb00119.x
22. Bandlitz S, Purslow C, Murphy PJ, Pult H. Time course of changes in tear meniscus radius and blink rate after instillation of artificial tears. Invest Ophthalmol Vis Sci. (2014) 55:5842–7. doi: 10.1167/iovs.14-14844
23. Mochel JP, Danhof M. Chronobiology and pharmacologic modulation of the renin-angiotensin-aldosterone system in dogs: what have we learned? Rev Physiol Biochem Pharmacol. (2015) 169:43–69. doi: 10.1007/112_2015_27
24. Pelligand L, Soubret A, King JN, Elliott J, Mochel JP. Modeling of large pharmacokinetic data using nonlinear mixed-effects: a paradigm shift in veterinary pharmacology. A case study with robenacoxib in cats. CPT Pharmacometrics Syst Pharmacol. (2016) 5:625–35. doi: 10.1002/psp4.12141
25. Gronkiewicz KM, Giuliano EA, Kuroki K, Bunyak F, Sharma A, Teixeira LB, et al. Development of a novel in vivo corneal fibrosis model in the dog. Exp Eye Res. (2016) 143:75–88. doi: 10.1016/j.exer.2015.09.010
26. Sebbag L, Allbaugh RA, Weaver A, Seo YJ, Mochel JP. Histamine-induced conjunctivitis and breakdown of blood-tear barrier in dogs: a model for ocular pharmacology and therapeutics. Front Pharmacol. (2019) 10:752. doi: 10.3389/fphar.2019.00752
27. Chavis RM, Welham RA, Maisey MN. Quantitative lacrimal scintillography. Arch Ophthalmol. (1978) 96:2066–8. doi: 10.1001/archopht.1978.03910060454013
28. Newbold GM, Outerbridge CA, Kass PH, Maggs DJ. Malassezia spp. on the periocular skin of dogs and their association with blepharitis, ocular discharge, and the application of ophthalmic medications. J Am Vet Med Assoc. (2014) 244:1304–8. doi: 10.2460/javma.244.11.1304
29. Johnstone MA, Albert DM. Prostaglandin-induced hair growth. Surv Ophthalmol. (2002) 47(Suppl. 1):S185–202. doi: 10.1016/S0039-6257(02)00307-7
30. Awwad S, Mohamed Ahmed AHA, Sharma G, Heng JS, Khaw PT, Brocchini S, et al. Principles of pharmacology in the eye. Br J Pharmacol. (2017) 174:4205–23. doi: 10.1111/bph.14024
31. Gelatt KN, MacKay EO, Widenhouse C, Widenhouse TS, Stopek JB. Effect of lacrimal punctal occlusion on tear production and tear fluorescein dilution in normal dogs. Vet Ophthalmol. (2006) 9:23–7. doi: 10.1111/j.1463-5224.2005.00430.x
32. Flach AJ. The importance of eyelid closure and nasolacrimal occlusion following the ocular instillation of topical glaucoma medications, and the need for the universal inclusion of one of these techniques in all patient treatments and clinical studies. Trans Am Ophthalmol Soc. (2008) 106:138–45; discussion: 145–38.
33. Zhu H, Chauhan A. Effect of viscosity on tear drainage and ocular residence time. Optom Vis Sci. (2008) 85:715–25. doi: 10.1097/OPX.0b013e3181824dc4
34. Herring IP, Bobofchak MA, Landry MP, Ward DL. Duration of effect and effect of multiple doses of topical ophthalmic 0.5% proparacaine hydrochloride in clinically normal dogs. Am J Vet Res. (2005) 66:77–80. doi: 10.2460/ajvr.2005.66.77
35. Patton TF. Pharmacokinetic evidence for improved ophthalmic drug delivery by reduction of instilled volume. J Pharm Sci. (1977) 66:1058–9. doi: 10.1002/jps.2600660746
36. Vézina M. Comparative ocular anatomy in commonly used laboratory animals. In: Weir AB, Collins M, editors. Assessing Ocular Toxicology in Laboratory Animals. Totowa, NJ: Humana Press (2013). p. 1–21. doi: 10.1007/978-1-62703-164-6_1
37. Maurice D. The effect of the low blink rate in rabbits on topical drug penetration. J Ocul Pharmacol Ther. (1995) 11:297–304. doi: 10.1089/jop.1995.11.297
38. Korb DR, Greiner JV, Glonek T, Whalen A, Hearn SL, Esway JE, et al. Human and rabbit lipid layer and interference pattern observations. In: Sullivan DA, Dartt DA, Meneray MA, editors. Lacrimal Gland, Tear Film, and Dry Eye Syndromes 2: Basic Science and Clinical Relevance. Boston, MA: Springer US (1998). p. 305–8. doi: 10.1007/978-1-4615-5359-5_42
39. Bentivoglio AR, Bressman SB, Cassetta E, Carretta D, Tonali P, Albanese A. Analysis of blink rate patterns in normal subjects. Mov Disord. (1997) 12:1028–34. doi: 10.1002/mds.870120629
40. Leonard BC, Yañez-Soto B, Raghunathan VK, Abbott NL, Murphy CJ. Species variation and spatial differences in mucin expression from corneal epithelial cells. Exp Eye Res. (2016) 152:43–8. doi: 10.1016/j.exer.2016.09.001
41. Saettone MF, Giannaccini B, Teneggi A, Savigni P, Tellini N. Vehicle effects on ophthalmic bioavailability: the influence of different polymers on the activity of pilocarpine in rabbit and man. J Pharm Pharmacol. (1982) 34:464–6. doi: 10.1111/j.2042-7158.1982.tb04762.x
42. Chrai SS, Robinson JR. Ocular evaluation of methylcellulose vehicle in albino rabbits. J Pharm Sci. (1974) 63:1218–23. doi: 10.1002/jps.2600630810
43. Carrington SD, Bedford PGC, Guillon JP, Woodward EG. Polarized light biomicroscopic observations on the pre-corneal tear film. 1. The normal tear film of the dog. J Small Anim Pract. (1987) 28:605–22. doi: 10.1111/j.1748-5827.1987.tb01276.x
44. Shell JW. Pharmacokinetics of topically applied ophthalmic drugs. Surv Ophthalmol. (1982) 26:207–18. doi: 10.1016/0039-6257(82)90081-9
45. Kaswan RL, Salisbury MA, Ward DA. Spontaneous canine keratoconjunctivitis sicca. A useful model for human keratoconjunctivitis sicca: treatment with cyclosporine eye drops. Arch Ophthalmol. (1989) 107:1210–6. doi: 10.1001/archopht.1989.01070020276038
46. Leonard BC, Stewart KA, Shaw GC, Hoehn AL, Stanley AA, Murphy CJ, et al. Comprehensive clinical, diagnostic, and advanced imaging characterization of the ocular surface in spontaneous aqueous deficient dry eye disease in dogs. Cornea. (2019) 38:1568–75. doi: 10.1097/ICO.0000000000002081
47. Ledbetter EC, Kice NC, Matusow RB, Dubovi EJ, Kim SG. The effect of topical ocular corticosteroid administration in dogs with experimentally induced latent canine herpesvirus-1 infection. Exp Eye Res. (2010) 90:711–7. doi: 10.1016/j.exer.2010.03.001
48. Sebbag L, Crabtree EE, Sapienza JS, Kim K, Rodriguez E. Corneal hypoesthesia, aqueous tear deficiency, and neurotrophic keratopathy following micropulse transscleral cyclophotocoagulation in dogs. Vet Ophthalmol. (2019). doi: 10.1111/vop.12705. [Epub ahead of print].
49. Sun WS, Baker RS, Chuke JC, Rouholiman BR, Hasan SA, Gaza W, et al. Age-related changes in human blinks. Passive and active changes in eyelid kinematics. Invest Ophthalmol Vis Sci. (1997) 38:92–9.
50. Van Haeringen NJ. Aging and the lacrimal system. Br J Ophthalmol. (1997) 81:824–6. doi: 10.1136/bjo.81.10.824
51. Kang YK, Im JC, Shin JP, Kim IT, Park DH. Short-term analysis of the residual volume of an eye drop following 23-gauge microincision vitrectomy surgery. Korean J Ophthalmol. (2017) 31:439–45. doi: 10.3341/kjo.2016.0090
52. Sorbara L, Simpson T, Vaccari S, Jones L, Fonn D. Tear turnover rate is reduced in patients with symptomatic dry eye. Cont Lens Anterior Eye. (2004) 27:15–20. doi: 10.1016/j.clae.2003.10.001
53. Behrens-Baumann W. Absorption of topically administered ciprofloxacin, ofloxacin and gentamicin in the inflamed rabbit eye. Ophthalmologica. (1996) 210:119–22. doi: 10.1159/000310687
54. Lux A, Maier S, Dinslage S, Süverkrüp R, Diestelhorst M. A comparative bioavailability study of three conventional eye drops versus a single lyophilisate. Br J Ophthalmol. (2003) 87:436–40. doi: 10.1136/bjo.87.4.436
55. Macdonald EA, Maurice DM. Loss of fluorescein across the conjunctiva. Exp Eye Res. (1991) 53:427–30. doi: 10.1016/0014-4835(91)90159-C
Keywords: tear film, drainage, canine, tear flow, fluorophotometry, eye drop, drug delivery
Citation: Sebbag L, Kirner NS, Allbaugh RA, Reis A and Mochel JP (2019) Kinetics of Fluorescein in Tear Film After Eye Drop Instillation in Beagle Dogs: Does Size Really Matter? Front. Vet. Sci. 6:457. doi: 10.3389/fvets.2019.00457
Received: 03 October 2019; Accepted: 28 November 2019;
Published: 19 December 2019.
Edited by:
Johanna Fink-Gremmels, Utrecht University, NetherlandsCopyright © 2019 Sebbag, Kirner, Allbaugh, Reis and Mochel. This is an open-access article distributed under the terms of the Creative Commons Attribution License (CC BY). The use, distribution or reproduction in other forums is permitted, provided the original author(s) and the copyright owner(s) are credited and that the original publication in this journal is cited, in accordance with accepted academic practice. No use, distribution or reproduction is permitted which does not comply with these terms.
*Correspondence: Lionel Sebbag, lsebbag@iastate.edu; Jonathan P. Mochel, jmochel@iastate.edu