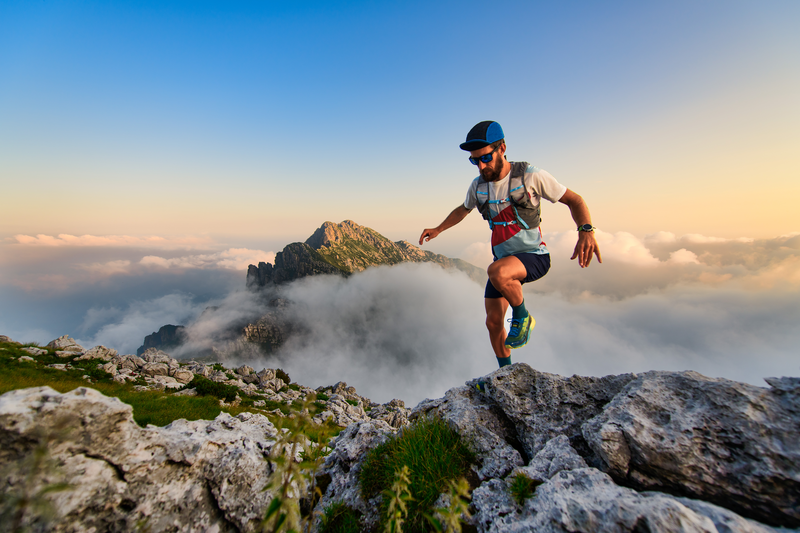
94% of researchers rate our articles as excellent or good
Learn more about the work of our research integrity team to safeguard the quality of each article we publish.
Find out more
ORIGINAL RESEARCH article
Front. Vet. Sci. , 19 November 2019
Sec. Zoological Medicine
Volume 6 - 2019 | https://doi.org/10.3389/fvets.2019.00405
This article is part of the Research Topic Health and Disease in Free-Ranging and Captive Wildlife View all 41 articles
Avian species experience extensive morbidity and mortality following large-scale oil spills, often resulting in oiled birds being rescued, and admitted to rehabilitation. Our objective was to experimentally establish time-specific, descriptive blood analyte data following sublethal oil exposure and subsequent rehabilitation. Thirty wild Ring-billed Gulls (Larus delawarensis) were randomly allocated to three treatment groups of 10 birds each. One treatment group served as controls and two treatment groups were externally oiled daily for 3 days with weathered MC252 oil collected from the Deepwater Horizon oil spill, mimicking the upper threshold of the US Fish and Wildlife Service's moderate oiling classification. Following external oiling, one oiled treatment group was cleaned via standard rehabilitation practices. Serial venous blood samples were collected for a month to measure packed cell volume, total solids, blood gas and select plasma biochemistry analytes, total white blood cell estimates and differentials, and reticulocyte estimates. We found that both sublethal oil exposure and aspects of captivity were associated with a mild non-regenerative anemia. No other differences in venous blood gas and biochemical analytes as well as white blood cell concentrations were observed among the three groups. These findings suggest that the mild anemia seen in oiled birds undergoing rehabilitation is possibly multifactorial and that moderately oiled gulls have subtle, but potentially not insignificant clinicopathological abnormalities following sublethal oil exposure. Oiled gulls did not develop any clinicopathological derangements post-rehabilitation, suggesting current standard practices for rehabilitation cause minimal morbidity in clinically stable, moderately oiled gulls.
The negative impact of oil spills on wildlife, particularly birds, has been widely recognized. Aquatic and semi-aquatic avian populations have experienced extensive morbidity and mortality following many large-scale oil spills, such as the Exxon Valdez (1), Prestige (2), and most recently, Deepwater Horizon (3, 4). However, crude oil's true impact on birds is likely larger than the reported acute mortality estimates (5, 6). Chronic oil exposure has been implicated in avian population declines long after the initial mass mortality following an oil spill, slowing population recovery despite clean up and environmental restoration (7). Thus, a stronger understanding of the effects of sublethal external oil exposure (i.e., external oiling that does not lead to acute death) on individual birds is warranted to better characterize avian oil toxicosis as well as the broader impacts of oil spills.
Sublethal oil exposure on birds causes a wide range of adverse effects including: hemolytic anemia; decreased nutrient absorption; altered physiological stress responses; renal, hematopoietic, and hepatic damage; and immunosuppression (8–12). Of these adverse effects, hemolytic anemia has been most frequently demonstrated in birds exposed to crude oil. Hemolytic anemia secondary to oil exposure is likely caused by oxidative damage mediated by metabolites of polycyclic aromatic hydrocarbons generated from cytochrome P450 enzymes in the livers of oiled birds (13, 14). Oil induced hemolytic anemia is characterized by red blood cell (RBC) regeneration, increased numbers of reticulocytes, and the development of Heinz bodies from oxidative damage to hemoglobin; however, the kinetics of these abnormalities is not well-characterized. Although the adverse effect of oil exposure on RBCs has been well-investigated, there are limited reports on the effect of oil on white blood cells (WBC) (15, 16). Previous studies have shown birds exposed to oil often exhibit reduction in total WBC counts and lymphocytes suggestive of immunosuppression as well as markedly elevated heterophil counts and heterophil/lymphocyte ratios (15, 16). There are currently no reports on the effect of oil exposure on the blood gas and acid-base status of birds.
Due to the development of protocol-driven response and recovery efforts, oiled birds are increasingly rescued and admitted to rehabilitation following oil spills (17). While on-going research continues to target aspects of emergency medicine and critical care for improvement, rehabilitation alone is an inherently stressful sequence of events for oiled birds. To date, no studies have evaluated the effect of current rehabilitation procedures on oiled birds. Knowledge of the clinicopathological abnormalities observed in birds following prolonged sublethal oil exposure, the effect rehabilitation has on these abnormalities, and the overall chronology of these abnormalities would be invaluable to veterinarians treating and managing oiled birds. However, given the temporal and spatial unpredictability of oil spills, this knowledge is difficult to acquire from wild birds in situ. While most experimental research on oil exposure has traditionally focused on oral dosing, less work has been conducted on sublethal external oiling. A recent suite of papers assessing avian exposure to Deepwater Horizon crude oil (18) has shown myriad impacts from sublethal external oiling, including flight impairment, reduced weight gain, altered metabolome, oxidative damage, changes in plasma biochemistry, changes in organ weights and histopathology, and cardiac dysfunction (19–24). Consequently, further exploration of the impacts of external oiling is warranted.
The objective of our study was to establish time-specific estimates of hematological, biochemical, and venous blood gas analytes to not only improve the point-of-care assessment of oiled birds, but also help better understand the pathogenesis of oil toxicosis from external oiling. Under experimental conditions and through serial blood sampling, our study characterizes post-exposure and post-rehabilitation changes in select blood analytes for birds externally oiled with weathered crude oil from the Deepwater Horizon oil spill. We also establish reference intervals for wild Ring-billed Gulls (Larus delawarensis), a common species found in coastal areas at risk of oil spills. We hypothesized that sublethal oil exposure would cause anemia and immunosuppression in externally oiled birds and that rehabilitated birds would return to pre-exposure values more quickly than post-exposure, non-rehabilitated birds.
All aspects of this study, including bird capture, transport, quarantine, husbandry, oiling, and sampling, were performed according to procedures approved by the Institutional Animal Care and Use Committee of the US Department of Agriculture, Animal and Plant Health Inspection Service, National Wildlife Research Center (NWRC; Approval 2806). Thirty-five Ring-billed Gulls (RBGU) were captured in Larimer County, CO using air cannon nets and net guns and transported to the NWRC, Fort Collins, CO in large ventilated crates. Birds were not captured in extreme weather conditions and transport time was <1 h. Capture was conducted under the authority of US Fish and Wildlife Service (USFWS) Migratory Bird Permit #MB019065 as well as Colorado Parks and Wildlife scientific collection license #18TRb2433.
Upon arrival at the NWRC, captured RBGUs were given a complete and thorough physical exam by the NWRC attending veterinarian, weighed, individually marked with a unique leg band, and a baseline blood sample was collected. Body temperature was also measured using a VetOne digital cloacal thermometer (MWI Animal Health, Aurora, CO). Given RBGUs are not sexually dimorphic, the sex distribution of the captured birds was unknown. Most birds were characterized as breeding adults based on plumage, but a few had markings that were consistent with either non-breeding adult or second winter plumage. Throughout the study, birds were group housed in large, netted outdoor flight pens (18.29 × 37.80 m) that contained artificial pools, heat lamps, as well as platforms and other objects that allowed for natural perching and loafing. During testing, RBGUs were offered a maintenance diet of commercial avian cubes (Mazuri Fish Analog 50/10 Frozen 5T8, St. Louis, MO), raw golden shiner minnows (Notemigonus crysoleucas, I.F. Anderson Farms, Inc., Lonoke, AR), and canned mackerel ad libutum two times per day. At the conclusion of this study, all surviving birds were transferred as study animals to other research projects or released near the capture site in Larimer County, CO.
MC252 oil collected during the Deepwater Horizon oil spill and artificially weathered (i.e., weathered in the laboratory prior to receipt for use in studies) was used to moderately externally oil two of the three treatments groups. Oil was artificially weathered by TDI-Brooks International, Inc. and B&B Laboratories (College Station, TX) and shipped to NWRC by Ecochem, Inc. (Tukwila, WA) under chain of custody.
After acclimation, 30 RBGUs were randomly allocated to three treatment groups of 10 birds each. A priori power analyses determined 10 birds per treatment group would adequately minimize a Type II statistical error (power = 0.996, given effect size = 0.8 and α = 0.05). Two treatment groups were externally oiled daily for 3 days with 5–7 mL of oil applied per day with a paintbrush to the breast, wing edges, and tail feathers mimicking the upper threshold of the USFWS moderate oiling classification for birds. This level of oiling is generally described as 14–40% surface area coverage. In general, oil was applied to body areas that would be exposed to an oil sheen for surface feeding birds such as RBGUs. After 3 days, oiled RBGUs received a cumulative total of 15–21 mL of oil. The third treatment group served as controls and were handled in the same manner as the other two groups but were painted with water instead of oil for 3 days. Nine days after initial oiling, one of the oiled treatment groups was cleaned following standard practices for rehabilitating oiled birds over a 48-h period. Briefly, oiled birds were washed in sequential tubs filled with diluted detergent (1-2%) in softened water (2–3 grains of hardness) heated to 106-110°F (41–43°C) until the water remained clear. Birds were then rinsed with softened water at a temperature of 106-112°F and allowed to rest in a clean and well-ventilated brooder box lined with warm towels and equipped with water for drinking while being dried with heat lamps. During the drying process, air temperature was maintained between 90 and 95°F (32-35°C), with the birds being carefully monitored for signs of hypothermia or hyperthermia. The second oiled treatment group remained externally oiled until the conclusion of the study and then was cleaned using the same methods as previously described.
In order to characterize changes over time, blood samples (200–300 μL), weights, and temperatures were collected from RBGUs immediately prior to the external oiling and then 7, 15, 22, and 31 days post-oiling (DPO). Venous blood was anaerobically and aseptically collected using a 1.0 inch, 22-gauge needle, and heparinized syringes from either the left or right brachial, distal metatarsal, or external jugular veins. Blood samples were immediately divided into two subsamples. The first subsample was stored in microtainers to later measure packed cell volume (PCV) and total solids using a PCV chart and refractometer as well as visually assess for presence of plasma hemolysis, following high-speed centrifugation of blood-filled microhematocrit tubes. The second subsample was used for instant venous blood gas and select plasma biochemistry analysis, using the Element POC Blood Gas & Electrolyte Analyzer (EPOC; Heska Corporation, Loveland, CO), a handheld veterinary point-of-care analyzer.
Venous blood gas and plasma biochemistry analytes of interest included: hematocrit; plasma concentrations of sodium, potassium, chloride, ionized calcium, lactate, glucose, and bicarbonate; pH; and venous pO2 and pCO2. Because the EPOC performed all analyses at 37°C, pH, pCO2, pO2, were temperature corrected (TC) to reflect the RBGU's respective body temperatures via the following equations:
where ΔT = 37°C−body temperature (25). The TC bicarbonate concentration was calculated via the Henderson-Hesselbach equation using TC pH and pCO2 values as well as the solubility coefficient for CO2 and pK (logarithmic acid dissociation constant) (25). Due to the effects of pH on ionized calcium, the following correction was applied (26):
Anion gap was calculated via the following equation:
Two duplicate blood smears were also made each time blood was drawn and subsequently submitted to the Colorado State University Veterinary Diagnostic Lab (Fort Collins, Colorado) for processing. Total WBC estimates were derived using an internal standard operating procedure for avian WBC estimates due to the unavailability of a whole blood sample. Briefly, all WBCs seen in ten 50× oil fields were counted and then divided by 10 to obtain the average WBC count per 50× oil field. This average was then used in the following equation:
This WBC estimate formula was developed from internal unpublished studies at the Colorado State University Veterinary Diagnostic Laboratory and has not been validated or verified. WBC differential was performed by calculating the percentage of each leukocyte type seen in a sample of 100 leukocytes under 1,000× oil immersion (27). Reticulocyte estimates were generated by enumerating aggregate reticulocytes and ring forms using previously described methods (28).
Physical, hematological, biochemical, and blood gas data collected from all 30 RBGUs immediately prior to the external oiling were aggregated to generate reference intervals. Given our small sample size and that some analytes had non-parametric distributions, robust estimation of the 90% confidence interval of reference limits was employed as suggested by Friedrichs et al. (29). Robust estimation of the 90% confidence interval was done using a bias corrected bootstrap method based on 10,000 bootstrap replicates. Median, minimum, and maximum values were also calculated and reported to allow informed clinical decision-making (29). For subsequent DPO, mean, median, standard deviation, minimum, and maximum were calculated for each treatment group separately. Data were also analyzed using multiple linear mixed models (LMM) for repeated measures with treatment group, DPO, and the interaction between treatment group and DPO as predictor variables, each of the various clinical analytes as the response variable, and the individual RBGU as a random effect. A separate LMM was run for each clinical analyte with the false discovery rate controlled via the Benjamini–Hochberg procedure (30). Hematocrit as determined by EPOC, hereafter HCT (EPOC), and PCV determined manually, hereafter PCV (spun), were compared using a paired t-test, Lin's concordance correlation coefficient (31), and a Bland-Altman plot (32). All statistics were performed in the statistical software program R version 3.5 (33) with the 90% confidence intervals being generated with the “boot” package and LMMs being fitted using the “lme4” package (34).
Physical, hematological, biochemical, and blood gas analyte values from baseline (0 DPO) sampling showed no obvious clinical abnormalities in the RBGUs (Table 1). Of the 30 RBGUs included in this study, 28 survived through its conclusion: one rehabilitated-oiled RBGU died between 7 and 15 DPO and one non-rehabilitated-oiled RBGU died between 22 and 31 DPO. Necropsies and histopathology of the deceased birds found the cause of death was not due to sublethal external oil exposure. LMMs were not run for monocytes, eosinophils, and basophils given their mean and median were 0 × 103/μL on 7, 15, 22, and 31 DPO.
Table 1. Reference intervals and summary statistics for body temperature and select venous blood analytes of wild Ring-billed Gulls (Larus delawarensis).
PCV (spun) and HCT (EPOC) of both the rehabilitated and non-rehabilitated-oiled groups were significantly lower when compared to the control group (Table 2). On average, non-rehabilitated-oiled RBGUs had 4 ± 0.8% lower PCV (spun) than controls and rehabilitated-oiled RBGUs had 3 ± 0.8% lower PCV (spun) than controls. Similarly, non-rehabilitated-oiled RBGUs had 5 ± 0.8% lower HCT (EPOC) than controls and rehabilitated-oiled RBGUs had 4 ± 0.8% lower HCT (EPOC) than controls on average. PCV (spun) and HCT (EPOC) were also significantly lower over time for all groups (Table 2). All treatment groups had lower PCV (spun) and HCT (EPOC) on 7, 15, 22, and 31 DPO compared to 0 DPO (Table 3). HCT (EPOC), but not PCV (spun), exhibited a significant interaction between non-rehabilitated-oiled group and time (Table 2); when compared to the control group, non-rehabilitated-oiled RBGUs' HCT (EPOC) decreased 0.2% more over time. Obvious plasma hemolysis was not visually observed following high-speed centrifugation of blood-filled microhematocrit tubes for any treatment group.
Table 2. Linear mixed model results for clinical analytes of Ring-billed Gulls (Larus delawarensis) in three treatment groups (Control gulls, Oiled/Non-Rehabilitated gulls, and Oiled/Rehabilitated gulls).
Table 3. Post-oiling summary statistics for Ring-billed Gull (Larus delawarensis) weight, body temperature, and select venous blood analytes stratified by treatment group (Control gulls, Oiled/Non-Rehabilitated gulls, and Oiled/Rehabilitated gulls) and days post-oiling (DPO).
Estimated reticulocytes significantly differed among treatment groups but did not change over time nor exhibit an interaction between group and time (Table 2). Non-rehabilitated-oiled RBGUs had a 1.3 ± 0.1% higher estimated reticulocyte percentage than both rehabilitated-oiled and control RBGUs. Body weight, body temperature, total solids, sodium, potassium, chloride, TC ionized calcium, lactate, glucose, TC anion gap, TC pH, TC pO2, TC bicarbonate, total WBC, heterophils, and lymphocytes did not differ among treatment groups, change over time, or exhibit an interaction between group and time.
HCT (EPOC) and PCV (spun) were significantly different [t(145) = 28.78, p < 0.001]—the HCT (EPOC) was on average 8.2% (95% CI: 1.5–14.9%) less than PCV (spun). The concordance correlation coefficient between HCT (EPOC) and PCV (spun) was 0.35 (95% CI: 0.28–0.41) suggesting low concordance in their results. A Bland-Altman plot of the relationship between HCT (EPOC) and PCV (spun) shows considerable variability in the discrepancy between the two methods, but no obvious trend (Figure 1).
Figure 1. A Bland-Altman plot of the diagnostic difference between hematocrit as measured by the Element POC Blood Gas and Electrolyte Analyzer—HCT (EPOC)—and PCV determined manually following high-speed centrifugation of microhematocrit tubes—PCV (spun). Values on the x-axis represent the mean HCT/PCV between the two methods for a given Ring-billed Gull (Larus delawarensis) and values on the y-axis represent the difference between the two methods for the same Ring-billed Gull. Levels of agreement (LoA) represent a 95% confidence interval around the average difference.
Brought to near extinction due to anthropogenic forces in the late nineteenth century, RBGUs have rebounded and become one of the most common and widespread gull species in North America. We are the first to report blood data reference intervals for wild RBGUs, a potential resource for future comparative studies or clinical work. RBGU PCV (spun) was similar to PCV data previously reported for other gull species (35–38). Total solids were lower than means reported in Kelp Gulls (Larus dominicanus) (37, 38), but birds sampled in both of these studies foraged on high-protein discards from the fishing industry which likely resulted in higher plasma protein concentrations. Glucose concentrations were within the range observed in Kelp Gulls and other seabirds (35, 37, 38). On average, RBGUs had a higher lymphocytes compared to heterophils, a finding seen in Great Black-backed Gulls (L. marinus) and Herring Gulls (L. argentatus) (39), but opposite of a pattern seen in Kelp Gulls (37).
Our results support our hypothesis that RBGUs develop some degree of anemia secondary to external oil exposure, similar to other avian species. The anemia seen in oiled RBGUs falls within the range of anemia found in externally oiled birds sampled shortly after the Deepwater Horizon oil spill which had on average a 4–19% lower PCV than non-oiled birds (40). Oiled RBGUs may have had a milder anemia compared to some of the birds sampled after Deepwater Horizon due to their easy access to nutritious, oil-free food, and limited need to expend energy foraging. Although control RBGUs had higher HCT (EPOC) than both non-rehabilitated and rehabilitated-oiled RBGUs over the study period, all RBGUs exhibited anemia after 0 DPO suggesting that both external oiling and aspects of captivity or repeated handling cause persistent mild anemia. The implication that captivity can induce anemia in wild birds over time supports previous experimental work; both orally exposed to oil and non-exposed Rhinoceros Auklets (Cerorhinca monocerata) had on average a 10% lower PCV after 3 weeks in captivity (41). Given our RBGUs were group housed in large outdoor flight pens that encouraged natural behaviors, the development of anemia secondary to captivity, regardless of oil exposure, is potentially due to alterations in physiological demands or the stress of repeated handling, rather than the sedentary nature of captivity.
While non-rehabilitated-oiled RBGUs had a higher estimated reticulocyte percentage than both rehabilitated-oiled RBGUs and control RBGUs, all three treatment groups failed to show increased RBC regeneration in the face of a persistent mild anemia, given reticulocyte percentages vary between 1 and 5% in healthy birds (27). This finding contrasts our hypothesis that rehabilitated birds would return to pre-exposure values quicker than non-rehabilitated birds as well as field observations made after the Deepwater Horizon oil spill that found externally oiled birds had on average 27-40% higher reticulocyte percentages (40). However, the absence of a regenerative response is similar to results from previous experimental studies. Rhinoceros Auklets orally exposed to oil as well as Double-crested Cormorants (Phalacocorax auritis) orally and externally exposed to oil showed regenerative, but inadequately compensated, responses to anemia with PCV never returning to levels recorded post-capture and pre-oiling (41, 42). The lack of compensatory RBC regeneration despite persistent anemia suggests a lack of production in hematopoietic tissue which may be caused by oil-induced bone marrow suppression or potential changes in physiological demands wild birds experience in captivity resulting in a “resetting” of homeostatic RBC levels.
Although sublethal oil exposure has long been suspected of causing immunosuppression in birds, the effects of oil toxicosis in rehabilitated or experimental birds is confounded with captivity-related stress. RBGUs showed low heterophil to lymphocyte ratios on 0 DPO, suggesting the birds were well-acclimated to captivity prior to external oiling given heterophils increase and lymphocytes decrease in response to stress (43). Following oil exposure, no difference in total WBC, heterophil, lymphocyte, monocyte, eosinophil, and basophil counts were found among treatment groups. This is inconsistent with our hypothesis and implies that moderate external oiling does not alter circulating WBCs. Laughing Gulls (Leucophaeus atricilla) offered fish injected with oil also showed no changes in circulating WBCs (44), whereas Common Terns (Sterna hirundo), sampled 14–21 days after the Buzzards Bay oil spill, exhibited severely depressed total WBC and lymphocyte counts as well as markedly elevated heterophil counts and heterophil/lymphocyte ratios (15). While further research is warranted, the acute stress of capturing and handling wild birds is potentially a factor in explaining the discrepancy between experimental studies showing no oil-induced immunosuppression and field observations showing some degree of immunosuppression. It is also possible that immunosuppression in experimentally oiled birds is mitigated by the adequate husbandry and nutrition provided during experimental studies. The WBC estimate methodology used herein was developed from unpublished studies and has not been validated or verified by independent review. Therefore, comparisons of our WBC data to other studies needs to be done cautiously.
The significant interaction between non-rehabilitated-oiled RBGUs' HCT (EPOC) suggests that chronic sublethal external oil exposure may result in ongoing anemia. While the control and rehabilitated-oiled RBGUs' HCT (EPOC) gradually increased toward baseline levels between 15 and 31 DPO, the non-rehabilitated-oiled RBGUs' HCT (EPOC) continued to decrease. The diagnostic disagreement between the EPOC and PCV determined manually is not surprising given the difference in methodologies. Veterinary point-of-care analyzers measure hematocrit via whole blood conductometry in which a higher proportion of RBCs decreases electrical conductivity. Consequently, many veterinary point-of-care analyzers likely underestimate avian hematocrit due to the difference in conductivity between avian nucleated RBCs and mammalian non-nucleated RBCs. Measurements of hematocrit performed by the VetScan i-STAT 1 Analyzer (Abaxis Inc., Union City, California) have been previously shown to be on average 15% lower than PCV determined manually in Bar-headed Geese (Anser indicus) (45) suggesting that the magnitude of underestimating hematocrit may vary amongst species or veterinary point-of-care analyzers. In addition to our limited sample size, possible limitations of our study include significant differences in weather across the study period, Element POC cartridge temperature, and/or observer effects, given sampling was conducted outside and multiple observers performed WBC differentials. Other limitations of our study include the RBGUs' aforementioned easy access to nutritious, oil-free food and limited need to expend energy foraging as well as the availability of heat lamps minimizing the adverse effects of oil on thermoregulation. Despite accounting for the heterogeneity of individual RBGUs in our LMMs as a random effect, the small sample size per treatment group could explain the significant interaction found with HCT (EPOC) but not PCV (spun) or the lack of differences among treatment groups for some analytes.
Our study is the first to report hematological, biochemical, and blood gas analytes in birds following sublethal oil exposure and rehabilitation. We found that both sublethal oil exposure and aspects of captivity caused a mild non-regenerative anemia, suggesting the etiology of anemia seen in oiled birds undergoing rehabilitation is possibly multifactorial. Given that moderately oiled gulls in our study had subtle, but potentially not insignificant clinicopathological abnormalities, gulls affected by sublethal external oiling are likely strong candidates for rescue and rehabilitation. Furthermore, oiled gulls did not develop any clinicopathological derangements post-rehabilitation, suggesting current standard practices for rehabilitation cause minimal morbidity in stable, moderately oiled gulls.
The datasets generated for this study are available on request to the corresponding author.
This animal study was reviewed and approved by the USDA/APHIS/WS National Wildlife Research Center Institutional Animal Care and Use Committee.
ND, KH, and SS developed this study's design and oversaw all aspects of the project. JE lead efforts to capture study animals and assisted with their routine husbandry. LW provided the veterinary point of care analyzer used in this study as well as veterinary expertise. ND was responsible for statistical analysis and primarily writing this manuscript. All authors assisted with data collection and played a significant role in editing/reviewing this manuscript.
This research was supported by the intramural research program of the US Department of Agriculture, Animal Plant Health Inspection Service.
The authors declare that the research was conducted in the absence of any commercial or financial relationships that could be construed as a potential conflict of interest.
The authors would like to thank Kevin Bentler, Katherine Dirsmith, and Mikaela Samsel for their assistance with data collection. The authors would also like to thank the animal care staff of the National Wildlife Research Center for their dedicated animal husbandry as well as the clinical pathology staff of the Colorado State University Veterinary Diagnostic Lab for processing the collected blood smears. Earlier versions of this manuscript were greatly improved through the thoughtful feedback from two reviewers and the editor.
1. Piatt JF, Lensink CJ, Butler W, Marshal K, Nysewander DR. Immediate impact of the 'Exxon Valdez' oil spill on marine birds. Auk. (1990) 107:387–97. doi: 10.2307/4087623
2. Munilla I, Arcos JM, Oro D, Álvarez D, Leyenda PM, Velando A. Mass mortality of seabirds in the aftermath of the Prestige oil spill. Ecosphere. (2011) 2:art83. doi: 10.1890/ES11-00020.1
3. Haney JC, Geiger HJ, Short JW. Bird mortality from the Deepwater Horizon oil spill. I. Exposure probability in the offshore Gulf of Mexico. Mar Ecol-Prog Ser. (2014) 513:225–37. doi: 10.3354/meps10991
4. Haney JC, Geiger HJ, Short JW. Bird mortality from the Deepwater Horizon oil spill. II. Carcass sampling and exposure probability in the coastal Gulf of Mexico. Mar Ecol-Prog Ser. (2014) 513:239–52. doi: 10.3354/meps10839
5. Velando A, Álvarez D, Mouriño J, Arcos F, Barros Á. Population trends and reproductive success of the European shag Phalacrocorax aristotelis on the Iberian Peninsula following the Prestige oil spill. J Ornithol. (2005) 146:116–20. doi: 10.1007/s10336-004-0068-z
6. Votier SC, Hatchwell BJ, Beckerman A, McCleery RH, Hunter FM, Pellatt J, et al. Oil pollution and climate have wide-scale impacts on seabird demographics. Ecol Lett. (2005) 8:1157–64. doi: 10.1111/j.1461-0248.2005.00818.x
7. Peterson CH, Rice SD, Short JW, Esler D, Bodkin JL, Ballachey BE, et al. Long-term ecosystem response to the Exxon Valdez oil spill. Science. (2003) 302:2082–6. doi: 10.1126/science.1084282
8. Alonso-Alvarez C, Pérez C, Velando A. Effects of acute exposure to heavy fuel oil from the Prestige spill on a seabird. Aquat Toxicol. (2007) 84:103–10. doi: 10.1016/j.aquatox.2007.06.004
9. Leighton FA. Morphological lesions in red blood cells from herring gulls and Atlantic puffins ingesting Prudhoe Bay crude oil. Vet Pathol. (1985) 22:393–402. doi: 10.1177/030098588502200415
10. Leighton FA. Clinical, gross, and histological findings in herring gulls and Atlantic puffins that ingested Prudhoe Bay crude oil. Vet Pathol. (1986) 23:254–63. doi: 10.1177/030098588602300305
11. Peakall DB, Norstrom RJ, Jeffrey DA, Leighton FA. Induction of hepatic mixed function oxidases in the herring gull (Larus argentatus) by Prudhoe Bay crude oil and its fractions. Comp Biochem Physiol C Toxicol Pharmacol. (1989) 94:461–3. doi: 10.1016/0742-8413(89)90098-4
12. Peakall DB, Tremblay J, Kinter WB, Miller DS. Endocrine dysfunction in seabirds caused by ingested oil. Environ Res. (1981) 24:6–14. doi: 10.1016/0013-9351(81)90126-2
13. Troisi GM, Bexton S, Robinson I. Polyaromatic hydrocarbon and PAH metabolite burdens in oiled common guillemots (Uria aalge) stranded on the east coast of England (2001–2002). Environ Sci Technol. (2006) 40:7938–43. doi: 10.1021/es0601787
14. Troisi G, Borjesson L, Bexton S, Robinson I. Biomarkers of polycyclic aromatic hydrocarbon (PAH)-associated hemolytic anemia in oiled wildlife. Environ Res. (2007) 105:324–9. doi: 10.1016/j.envres.2007.06.007
15. Nisbet ICT, Tseng FS, Fiorello CV, Apanius V. Changes in white blood cell parameters of common terns (Sterna hirundo) exposed to low levels of oil. Waterbirds. (2015) 38:415–9. doi: 10.1675/063.038.0405
16. Briggs KT, Gershwin ME, Anderson DW. Consequences of petrochemical ingestion and stress on the immune system of seabirds. ICES J Mar Sci. (1997) 54:718–25. doi: 10.1006/jmsc.1997.0254
17. Mazet JAK, Newman SH, Gilardi KVK, Tseng FS, Holcomb JB, Jessup DA, et al. Advances in oiled bird emergency medicine and management. J Avian Med Surg. (2002) 16:146–9. doi: 10.1647/1082-6742(2002)016[0146:AIOBEM]2.0.CO;2
18. Bursian SJ, Alexander CR, Cacela D, Cunningham FL, Dean KM, Dorr BS, et al. Overview of avian toxicity studies for the deepwater horizon natural resource damage assessment. Ecotox Environ Safe. (2017) 146:4–10. doi: 10.1016/j.ecoenv.2017.05.014
19. Dean KM, Cacela D, Carney MW, Cunningham FL, Ellis C, Gerson AR, et al. Testing of an oral dosing technique for double-crested cormorants, Phalacocorax auritus, laughing gulls, Leucophaeus atricilla, homing pigeons, Columba livia, and western sandpipers, Calidris mauri, with artificially weather MC252 oil. Ecotox Environ Safe. (2017) 146:11–8. doi: 10.1016/j.ecoenv.2017.07.003
20. Perez CR, Moye JK, Cacela D, Dean KM, Pritsos CA. Low level exposure to crude oil impacts avian flight performance: the Deepwater Horizon oil spill effect on migratory birds. Ecotox Environ Safe. (2017) 146:98–103. doi: 10.1016/j.ecoenv.2017.05.028
21. Perez CR, Moye JK, Cacela D, Dean KM, Pritsos CA. Homing pigeons externally exposed to Deepwater Horizon crude oil change flight performance and behavior. Environ Pollut. (2017) 230:530–9. doi: 10.1016/j.envpol.2017.07.008
22. Harr KE, Reavill DR, Bursian SJ, Cacela D, Cunningham FL, Dean KM, et al. Organ weights and histopathology of double-crested cormorants (Phalacrocorax auritus) dosed orally or dermally with artificially weathered Mississippi Canyon 252 crude oil. Ecotox Environ Safe. (2017) 146:52–61. doi: 10.1016/j.ecoenv.2017.07.011
23. Harr KE, Rishniw M, Rupp TL, Cacela D, Dean KM, Dorr BS, et al. Dermal exposure to weathered MC252 crude oil results in echocardiographically identifiable systolic myocardial dysfunction in double-crested cormorants (Phalacrocorax auritus). Ecotox Environ Safe. (2017) 146:76–82. doi: 10.1016/j.ecoenv.2017.04.010
24. Dorr BS, Hanson-Dorr KC, Assadi-Porter FM, Selen ES, Healy KA, Horak KE. Effects of repeated sublethal external exposure to Deepwater Horizon oil on the avian metabolome. Sci Rep. (2019) 9:371. doi: 10.1038/s41598-018-36688-3
25. Ashwood ER, Kost G, Kenny M. Temperature correction of blood-gas and pH measurements. Clin Chem. (1983) 29:1877–85.
26. Fogh-Andersen N. Ionized calcium analyzer with a built-in pH correction. Clin Chem. (1981) 27:1264–7.
27. Campbell TW. Exotic Animal Hematology and Cytology. Ames, IA: John Wiley & Sons (2015). doi: 10.1002/9781118993705
28. Johns JL, Shooshtari MP, Christopher MM. Development of a technique for quantification of reticulocytes and assessment of erythrocyte regenerative capacity in birds. Am J Vet Res. (2008) 69:1067–72. doi: 10.2460/ajvr.69.8.1067
29. Friedrichs KR, Harr KE, Freeman KP, Szladovits B, Walton RM, Barnhart KF, et al. ASVCP reference interval guidelines: determination of de novo reference intervals in veterinary species and other related topics. Vet Clin Path. (2012) 41:441–53. doi: 10.1111/vcp.12006
30. Benjamini Y, Hochberg Y. Controlling the false discovery rate: a practical and powerful approach to multiple testing. J R Stat Soc Series B Stat Methodol. (1995) 57:289–300. doi: 10.1111/j.2517-6161.1995.tb02031.x
31. Lin LI. A concordance correlation coefficient to evaluate reproducibility. Biometrics. (1989) 45:255–68. doi: 10.2307/2532051
32. Bland JM, Altman DG. Statistical methods for assessing agreement between two methods of clinical measurement. Lancet. (1986) 1:307–10. doi: 10.1016/S0140-6736(86)90837-8
33. Team RC. R: A Language and Environment for Statistical Computing. Vienna: R Foundation for Statistical Computing (2019).
34. Bates D, Mächler M, Bolker B, Walker S. Fitting linear mixed-effects models using lme4. J Stat Softw. (2015) 67:1–48. doi: 10.18637/jss.v067.i01
35. Newman SH, Piatt JF, White J. Hematological and plasma biochemical reference ranges of Alaskan seabirds: their ecological significance and clinical importance. Waterbirds. (1997) 20:492–504. doi: 10.2307/1521600
36. Garcia MTA, Hermosa Y, Aguirre JI. Does breeding status influence haematology and blood biochemistry of yellow-legged gulls? Acta Biol Hung. (2010) 61:391–400. doi: 10.1556/ABiol.61.2010.4.3
37. Doussang D, Merino V, Moreno L, Islas A, Barrientos C, Mathieu C, et al. Hematologic and biochemical parameters of kelp gulls (Larus dominicanus) captured in the city of Talcahuano, Chile. J Zoo Wildl Med. (2015) 46:447–55. doi: 10.1638/2012-0080.1
38. D'Amico VL, Fazio A, Palacios MG, Carabajal E, Bertellotti M. Evaluation of physiological parameters of kelp gulls (Larus dominicanus) feeding on fishery discards in Patagonia, Argentina. Waterbirds. (2018) 41:310–5. doi: 10.1675/063.041.0311
39. Averbeck C. Haematology and blood chemistry of healthy and clinically abnormal great black-backed gulls (Larus Marinus) and herring gulls (Larus Argentatus). Avian Pathol. (1992) 21:215–23. doi: 10.1080/03079459208418837
40. Fallon JA, Smith EP, Schoch N, Paruk JD, Adams EA, Evers DC, et al. Hematological indices of injury to lightly oiled birds from the Deepwater Horizon oil spill. Environ Toxicol Chem. (2018) 37:451–61. doi: 10.1002/etc.3983
41. Newman SH, Mazet JK, Ziccardi MH, Lieske CL, Fauquier DA, Gardner IA, et al. Haematological changes and anaemia associated with captivity and petroleum exposure in seabirds. Comp Clin Path. (1999) 9:60–7. doi: 10.1007/BF02585537
42. Harr KE, Cunningham FL, Pritsos CA, Pritsos KL, Muthumalage T, Dorr BS, et al. Weathered MC252 crude oil-induced anemia and abnormal erythroid morphology in double-crested cormorants (Phalacrocorax auritus) with light microscopic and ultrastructural description of Heinz bodies. Ecotox Environ Safe. (2017) 146:29–39. doi: 10.1016/j.ecoenv.2017.07.030
43. Davis AK. Effect of handling time and repeated sampling on avian white blood cell counts. J Field Ornithol. (2005) 76:334–8. doi: 10.1648/0273-8570-76.4.334
44. Horak KE, Bursian SJ, Ellis CK, Dean KM, Link JE, Hanson-Dorr KC, et al. Toxic effects of orally ingested oil from the Deepwater Horizon spill on laughing gulls. Ecotox Environ Safe. (2017) 146:83–90. doi: 10.1016/j.ecoenv.2017.07.018
Keywords: oil toxicity, wildlife rehabilitation, hemolytic anemia, immune suppression, blood gas, Ring-billed Gull
Citation: Dannemiller NG, Horak KE, Ellis JW, Barrett NL, Wolfe LL and Shriner SA (2019) Effects of External Oiling and Rehabilitation on Hematological, Biochemical, and Blood Gas Analytes in Ring-Billed Gulls (Larus delawarensis). Front. Vet. Sci. 6:405. doi: 10.3389/fvets.2019.00405
Received: 12 June 2019; Accepted: 31 October 2019;
Published: 19 November 2019.
Edited by:
Nicole Indra Stacy, University of Florida, United StatesReviewed by:
Lorelei Schwacke, National Marine Mammal Foundation, United StatesCopyright © 2019 Dannemiller, Horak, Ellis, Barrett, Wolfe and Shriner. This is an open-access article distributed under the terms of the Creative Commons Attribution License (CC BY). The use, distribution or reproduction in other forums is permitted, provided the original author(s) and the copyright owner(s) are credited and that the original publication in this journal is cited, in accordance with accepted academic practice. No use, distribution or reproduction is permitted which does not comply with these terms.
*Correspondence: Nicholas G. Dannemiller, ZGFubmVtaWxsZXJuQGdtYWlsLmNvbQ==
Disclaimer: All claims expressed in this article are solely those of the authors and do not necessarily represent those of their affiliated organizations, or those of the publisher, the editors and the reviewers. Any product that may be evaluated in this article or claim that may be made by its manufacturer is not guaranteed or endorsed by the publisher.
Research integrity at Frontiers
Learn more about the work of our research integrity team to safeguard the quality of each article we publish.