- 1Department of Biomedical Sciences, Section of Anatomic Pathology, Cornell University College of Veterinary Medicine, Ithaca, NY, United States
- 2Department of Microbiology, Immunology, and Pathology, College of Veterinary Medicine and Biomedical Sciences, Colorado State University, Fort Collins, CO, United States
- 3Athens Veterinary Diagnostic Laboratory and Department of Pathology, University of Georgia College of Veterinary Medicine, Athens, GA, United States
- 4Department of Veterinary Pathobiology, College of Veterinary Medicine & Biomedical Sciences, Texas A&M University, College Station, TX, United States
Canine glioma is considered a potential model for human glioma, with recent studies of occurrence, therapy, and reclassification supporting the value of the canine model. The current diagnosis of canine glioma is based on morphologic criteria and immunohistochemistry (IHC), including oligodendrocyte transcription factor 2 (Olig2), glial fibrillary acidic protein (GFAP), and 2′, 3′ cyclic nucleotide phosphatase (CNPase). Microtubule-associated protein 2 (MAP2) is a proven marker of human glioma and is used to complement the diagnosis and its specific immunoreactivity pattern contributes to the differentiation of astrocytomas from other glial tumors. The objective of this study was to evaluate whether canine gliomas express MAP2 and to explore differences in the pattern of immunolabeling between different gliomas. Seventy-eight cases of canine glioma were evaluated for MAP2 expression by immunohistochemistry. A glial origin was supported by Olig2 IHC in all cases. MAP2 immunolabeling was evaluated on a semi-quantitative basis, including the percentage of immunolabeled neoplastic cells, as well as the signal intensity, distribution, and pattern of immunolabeling. MAP2 was expressed in all cases, with significant correlation between diagnosis and signal intensity (P = 0.04). MAP2 immunolabeling distribution was dominated by diffuse (34/78; 44%), followed by patchy (20/78; 26%), multifocal to coalescing (16/78; 21%), and scattered (8/78; 10%). All oligodendrogliomas (53/53; 100%) and undefined gliomas (12/12; 100%) revealed a combination of perinuclear and cytoplasmic immunolabeling, and all but 3 astrocytomas had a combination of perinuclear and cytoplasmic processes immunolabeling (10/13; 77%). Significant correlation between immunolabeling pattern and diagnosis was obtained (P = 0.001). The study demonstrates that MAP2 is expressed in canine gliomas and the pattern of expression can also be applied to help distinguish astrocytomas from oligodendrogliomas and undefined gliomas.
Introduction
Canine brain tumors are a potential naturally occurring model for their counterpart human disease and occur at a comparable incidence between the two species (1–5). Recent efforts to reclassify canine gliomas (6) have bridged the gap between canine and human glioma histopathology by employing a streamlined and more comprehensive tumor classification scheme. This reclassification has standardized the diagnoses and grading of canine glioma to oligodendroglioma, astrocytoma, or undefined representing either high- or low-grade neoplasms (6). Primary tumors of the central nervous system (CNS) in dogs are dominated by meningioma (~50%) (7), followed by gliomas (~40%) (8, 9), and choroid plexus tumors (~7%) (10, 11). Over 50% of gliomas occur in brachycephalic breeds, such as boxers, Boston terriers, and bulldogs. Even among the brachycephalic breeds, boxers, bulldogs, and Boston terriers have a reported higher incidence for astrocytomas in particular (12). The overall incidence of primary CNS tumors in dogs ranges from 3 to 5% of all neoplasms and is similar to their human counterparts (1, 13). The prognosis of dogs with primary CNS tumors is guarded to poor, and numerous cases are euthanized after the initial diagnosis. Therapeutic management of these cases, when elected, follows similar protocols as in humans, including surgical resection, radiation, and chemotherapy. Histologic characterization of the neoplasm is integral to deciding on the therapeutic course (14), but therapy is often attempted without fulfilling this step (15).
A variety of morphological and immunohistochemical (IHC) similarities between canine and human gliomas have been reported, particularly in astrocytomas (16–19). Olig2, a transcription factor, consistently labels canine glioma (20) with additional differentiation aided by expression of CNPase, for oligodendroglioma, and GFAP, for astrocytoma (6, 21). Furthermore, expression of growth factors and receptors, such as VEGF, EGFR-1, and PDGFR alpha, which correlate with higher grades for astrocytomas in humans, are also documented in dogs (18). In human neuropathology, molecular techniques are the norm for determining the diagnosis and prognosis; however, such techniques are not yet widely used in the veterinary field. Recent studies do indicate a wide variety of cell signaling abnormalities in canine glioma, many similar to those described in human glioma (22).
The cellular origin of gliomas is still a heavily debated topic (23, 24). Current theories are focused on neural stem cells (23); however, there is strong evidence that mature astrocytes can dedifferentiate and regain immature glial properties and be a source of neoplastic transformation (25, 26). While the cell of origin remains unclear in the dog, canine oligodendrogliomas do express a number of immunohistochemical markers of oligodendrocyte precursor cells including SOX10 and NG2, supporting a role for altered progenitor cells in the pathogenesis (27).
Microtubule-associated proteins (MAPs) are a group of cytoskeletal proteins of the MAP2/Tau family (28) that regulate homeostasis of microtubules. MAP2 binds to and increases the rigidity of the microtubules. Previous research has indicated that MAP2 plays an important role in forming dendritic protrusions in astrocytes and oligodendrocytes (28, 29). As cytoskeletal microtubules are essential for cell migration, interrupting microtubular dynamics would offer the potential of altering the infiltrative nature of glioma (30). MAP2 is found in two main forms, low molecular weight (MAP2c and MAP2d) and high molecular weight (MAP2a and MAP2b) protein (28). In the adult brain, MAP2a, 2b, and 2c are confined to neurons whereas MAP2d is associated with oligodendroglial precursors (31). The purpose of this study was to evaluate the expression of MAP2 in canine gliomas and determine if expression differences exist amongst oligodendroglioma, astrocytoma, and undefined gliomas.
Methods
Cases
A total of 78 canine gliomas were included in this study. Cases were retrieved from the pathology archives of Cornell University College of Veterinary Medicine, New York State Animal Health Diagnostic Center; University of Georgia College of Veterinary Medicine, Athens Diagnostic Laboratory; Colorado State University College of Veterinary Medicine; and Texas A&M College of Veterinary Medicine. All cases were fixed in 10% neutral buffered formalin, embedded in paraffin, sectioned at 5 μm, and stained with hematoxylin and eosin. All cases were classified and graded according to Koehler et al. (6).
Immunohistochemistry Protocols and Evaluation
Using an online sequence alignment tool (https://blast.ncbi.nlm.nih.gov/Blast.cgi), the MAP2 sequences for the dog were compared to sequences for rat and human and sequence homology indicated that an antibody for MAP2 that was derived in a rat would be predicted to label canine tissue. IHC for MAP2 (Sigma-Aldrich, St. Louis, MO; catalog number M4403) and Olig2 (Abcam Cambridge, MA, catalog number EPR2673) were performed using the Leica BOND Max IHC Staining System (Leica Systems, Buffalo Grove, IL). Pretreatment with heat-induced antigen retrieval was performed for 30 min using Tris/EDTA pH 9 (Bond Epitope Retrieval Solution 2, Leica, catalog #AR9640) for MAP2 and Olig2. Endogenous peroxidase activity was blocked with a 3% peroxide solution for 5 min (Leica, catalog #DS9800). The antibodies were diluted at 1:1,000 for 60 min (MAP2) and 1:200 for 60 min (Olig2). Biotin-free PowerVision poly-polymeric horseradish peroxidase anti-mouse (MAP2) (Leica, catalog #PV6114) or anti-rabbit (Olig2) (Leica, catalog #PV6119) IgG reagent was then applied to the slides for 30 min, followed by incubation with Bond Polymer Refine Detection for 10 min (Leica, catalog #DS9800). Tissues were developed with 3,3-diaminobenzidine (DAB) (Leica, catalog #DS9800) for 10 min. The slides were counterstained with hematoxylin (Leica, catalog #DS9800) for 5 min, dehydrated, cleared, and mounted. A tissue microarray composed of a number of canine tissues including kidney, lung, liver, spleen, brain, heart, small intestine, testis, lymph node, and pancreas was used to determine the specificity of the antibody to canine nervous tissue. In the tissue microarray, labeling was consistently present in the brain sections. Positive controls consisted of normal canine brain where MAP2 immunolabeling is found in the cytoplasm of neurons. Negative controls consisted of an isotype-matched antibody.
The degree of signal intensity was measured on a semi-objective scale of 0–3 according to the following criteria: 0 = no labeling; 1 = less than 25% of neoplastic cells with immunolabeling; 2 = 25–75% of neoplastic cells with immunolabeling; 3 = more than 75% of neoplastic cells with immunolabeling. Percentage of positive cells were determined based on the overall immunoreaction within the entirety of the neoplasm. The overall strength of the signal intensity was scored based on reasonable staining differences from 0 to 3, with 0 corresponding to no detectable signal, and 1, 2, and 3 corresponding to low, intermediate, and strongest signal intensity, respectively. The distribution of the immunolabeling within the neoplasm was evaluated as diffuse (throughout the neoplasm), multifocal to coalescing (large areas of immunolabeling within the mass that are confluent), patchy (small clusters of neoplastic cells with immunolabeling), and scattered (mostly individualized cells with immunolabeling). The cellular pattern of immunolabeling was also evaluated as nuclear (Nc), perinuclear (PNc), cytoplasmic (Ct), cytoplasmic processes (CtP), or any combinations of these. The IHC immunolabeling was scored independently by EAD and by a board certified veterinary pathologist (ADM). Intensity scores were then determined for each case through a combined review of EAD and ADM's original scoring. Olig2 expression was evaluated for percentage of neoplastic cells with immunolabeling based on the same scoring system employed for MAP2. All data were statistically analyzed using JMP™ software (www.jmp.com).
Results
The 78 cases included 34 different dog breeds, dominated by boxer (16/78; 21%), and followed by American bulldog (5/78; 6%), Boston terrier (4/78; 5%), and French bulldog (3/78; 4%), with individual cases corresponding to other breeds (Table 1). Spayed females made up 45% of all cases (35/78), followed by male castrated (36%; 28/78), male intact (8%; 6/78), and female intact (4%; 3/78), with 4/78 cases (5%) recorded as male not otherwise specified (NOS) and 2/78 (3%) cases with unknown sex. Ages ranged from 1 to 15 with an average of 7.6 years old and a median of 8 years old. The anatomic location of the neoplasm was dominated by forebrain NOS (20/78; 26%), followed by right forebrain (16/78; 21%), left forebrain (14/78; 18%), and cerebellum (5/78; 6%), with other anatomic locations represented in lower numbers (Table 1). The 78 glioma cases consisted of oligodendroglioma (Figure 1), astrocytoma (Figure 2), and undefined glioma (Figure 3). Of each diagnostic category, there were 53 oligodendrogliomas (68%; of which 32 were high-grade and 21 were low-grade; Figures 1A,B); 13 astrocytomas (17%; of which 2 were high-grade and 11 were low-grade; Figures 2A,C); and 12 undefined gliomas (15%; of which 7 were high-grade and 5 were low-grade; Figures 3A,B).
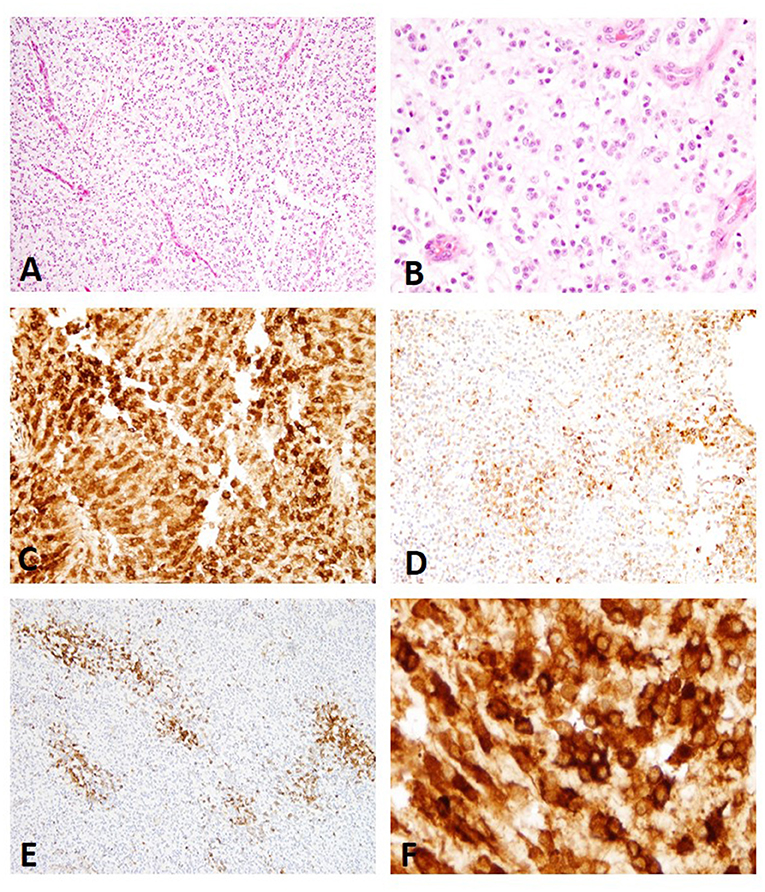
Figure 1. (A) Canine, Oligodendroglioma. Sheets of neoplastic oligodendrocytes with nuclear rowing and sparse stroma with branching capillaries (Hematoxylin and eosin (H&E) stain). (B) Canine, Oligodendroglioma. Round nuclei with coarse chromatin (H&E stain). (C) Canine, Oligodendroglioma. Diffuse immunolabeling with strong signal intensity (score 3) (Immunohistochemistry (IHC); MAP2). (D) Canine, Oligodendroglioma. Multifocal to coalescing distribution with moderate signal intensity (score 2) (IHC; MAP2). (E) Canine, Oligodendroglioma. Multifocal patchy immunolabeling with moderate signal intensity (score 2) (IHC; MAP2). (F) Canine Oligodendroglioma. Perinuclear and cytoplasmic immunolabeling pattern present in all cases of oligodendroglioma (IHC; MAP2).
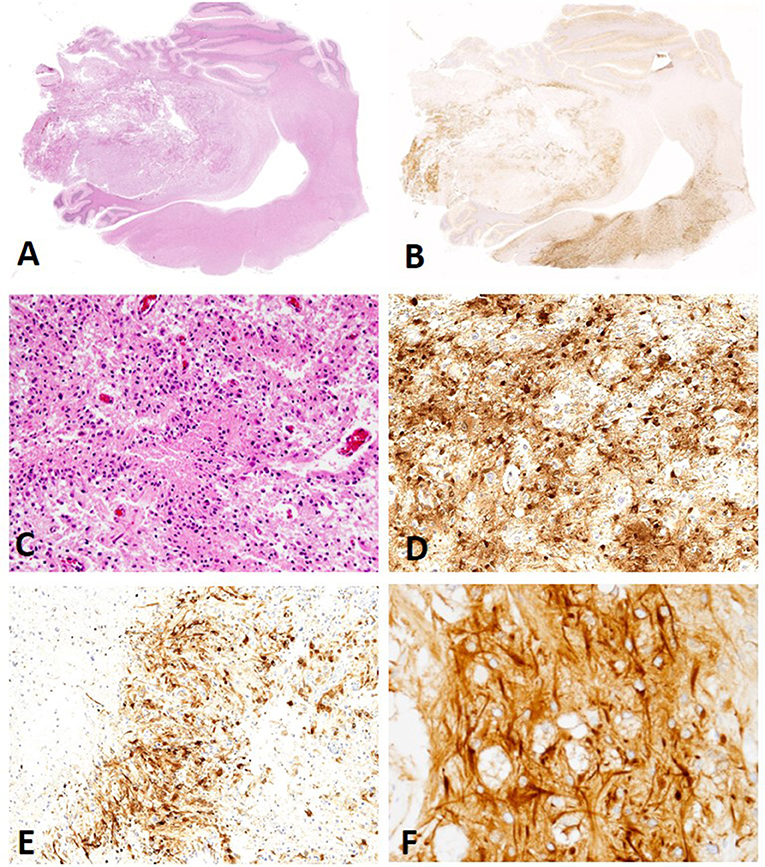
Figure 2. (A) Canine, Astrocytoma. Low-magnification of an astrocytoma (Hematoxylin and eosin (H&E) stain). (B) Canine, Astrocytoma. Low-magnification of MAP2 immunolabeling in an astrocytoma revealing multifocal to coalescing distribution with score 2 signal intensity (Immunohistochemistry (IHC); MAP2). (C) Canine, Astrocytoma. Low degree of cellular density, pleomorphism, and angular nuclei. (H&E stain). (D) Canine, Astrocytoma. Diffuse distribution of MAP2 immunolabeled cells in a case scored as a 3 for percent of neoplastic cells with immunolabeling (IHC; MAP2). (E) Canine, Astrocytoma. Patchy, multifocal distribution of MAP2 immunolabeled neoplastic cells (IHC; MAP2). (F) Canine, Astrocytoma. Immunolabeling of cytoplasmic processes (CtP) and perinuclear (PNc), a pattern noted in the majority of canine astrocytomas (IHC; MAP2.).
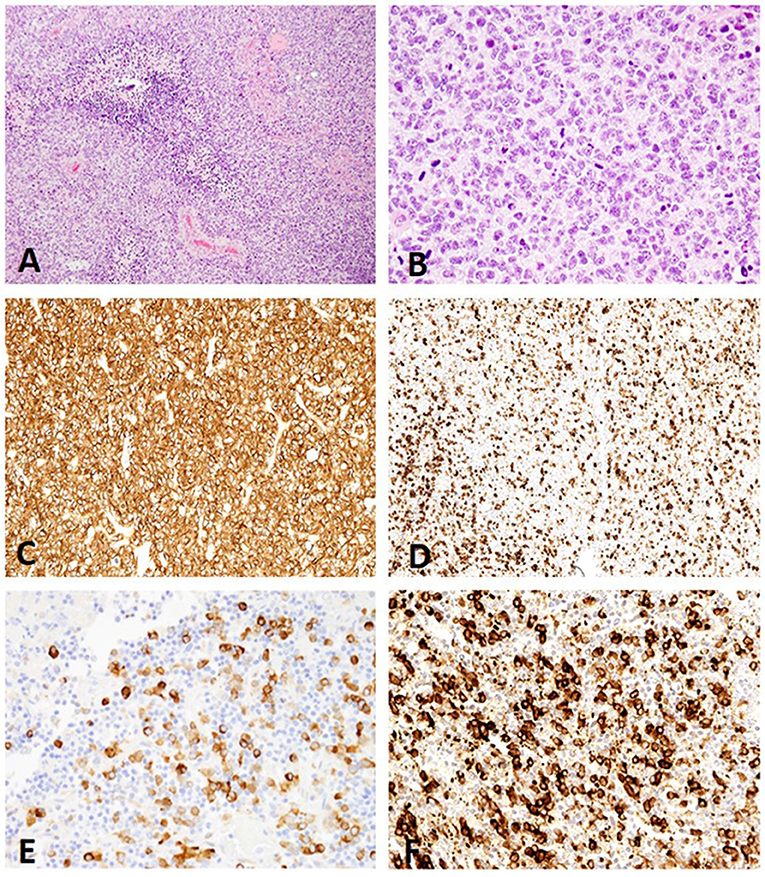
Figure 3. (A) Canine, Undefined glioma. Biphasic or biphenotypic morphology with palisading neoplastic cells around areas of necrosis. (Hematoxylin and eosin (H&E) stain). (B) Canine, Undefined glioma. Combination of nuclear rowing, pleomorphism, and high mitotic count (H&E stain). (C) Canine, Undefined glioma. MAP2 immunolabeling of canine undefined glioma revealing diffuse distribution with a score 3 signal intensity (Immunohistochemistry (IHC); MAP2). (D) Canine, Undefined glioma. Scattered distribution with a score 2 signal intensity (IHC; MAP2). (E) Canine, Undefined glioma. Scattered distribution with a score 1 signal intensity (IHC; MAP2). (F) Canine, Undefined glioma. The immunolabeling pattern, perinuclear (PNc) and cytoplasmic (Ct), noted in all undefined gliomas (IHC; MAP2).
MAP2 immunolabeling percentage: Equal numbers of gliomas were scored 3 (30/78; 38.5%) and 2 (30/78; 38.5%), while fewer scored 1 (18/78; 23%). There were no cases with 0 scoring on MAP2 scoring, allowing application of the null hypothesis (32), that reveals a 0.037% chance that a 0 score can happen with a canine glioma.
MAP2 immunolabeling signal intensity: Most cases had score 3 signal intensity (30/78; 38.5%), followed by 2 (28/78; 36%) and 1 (20/78; 26%). Significant correlation between type of glioma and signal intensity was found, regardless of the grade (P = 0.04).
MAP2 immunolabeling distribution: The distribution was dominated by diffuse immunolabeling (34/78; 44%), followed by patchy (20/78; 26%), multifocal to coalescing (16/78; 21%), and scattered (8/78; 10%).
MAP2 cellular pattern of immunolabeling: All oligodendrogliomas (53/53; 100%) and undefined gliomas (12/12; 100%) had a combination of PNc and Ct staining (Figures 1C–F, 3C–F). Three astrocytomas (3/13; 23%) had similar PNc and Ct staining; however, the remaining astrocytoma cases had a combination of PNc and CtP staining (10/13; 77%; Figures 2B,D–F). A significant correlation between staining pattern and diagnosis was obtained (P = 0.001). Specifically astrocytomas were more likely to stain with a combination of PNc and CtP, and oligodendrogliomas and undefined gliomas were more likely to stain with a PNc and Ct pattern (Supplemental Figures 1, 2).
MAP2 scoring and neoplasm grading (low vs. high-grade) comparison: Most high-grade gliomas (18/41; 44%) were assigned a score of 2 when analyzing the MAP2 immunolabeling percentage, followed by 3 (16/41; 39%), and 1 (7/41; 17%). A more even distribution was noted in the low-grade glioma group with most tumors assigned a score 3 (14/37; 38%), followed by score 2 (12/37; 32%), and score 1 (11/37; 30%). The majority of low-grade tumors had a PNc and Ct staining pattern (78%), and the remaining 21% had PNc and CtP staining. Ninety-five percent of the high-grade tumors had a PNc and Ct staining pattern, and only 5% had a PNc and CtP pattern. Overall, no correlation could be achieved between tumor grade and MAP2 signal intensity score or immunolabeling pattern. Olig2 expression was only used to score the percentage of neoplastic cells with immunolabeling. The majority of cases had a score of 3 (45/78; 58%), followed by 2 (28/78; 36%), and 1 (5/78; 6%) for Olig2 labeling.
Discussion
The classification of the gliomas included in this study was based on Koehler et al. (6). Based on these new criteria, glioma diagnoses are divided into oligodendroglioma, astrocytoma, and undefined, where the latter contains a roughly equal distribution of the two former glioma subtypes. Criteria considered for oligodendroglioma are: round nuclei, coarse chromatin, nuclear rowing, artifactual loss of cytoplasm, branching capillaries, and pseudo-rosettes. For astrocytoma, the criteria are: angular nuclei, open chromatin, pleomorphism, and a lower degree of cellular density than oligodendroglioma. The distribution of glioma subtypes in this study is similar to previous findings (6), with most cases being oligodendroglioma. Determining the exact prevalence and anatomic distribution of these tumors is challenging in veterinary medicine, due to data heterogeneity and inconsistent diagnostic criteria. While sample size is a limiting factor for strong statistical correlations, boxers were overrepresented in this study, similar to other previous reports (2, 10, 11). A susceptibility locus on canine chromosome 26 has been associated with a predilection for glioma in brachycephalic breeds (2, 22), but the routine molecular analyses necessary to confirm this predilection are nascent in veterinary medicine. The average age of the dogs in our study was 7.6 years, similar with previous reports, with 1 year old and 15 years old the minimum and maximum age, respectively.
Numerous monoclonal and polyclonal antibodies have been applied in the diagnosis of canine CNS tumors (8, 33), which allows for a more accurate correlation with their human counterparts. MAP2 is a proven marker in human gliomas, consistently used for diagnostic and grading purposes (31, 34–36), but its potential application in canine gliomas had not been explored to date. While sample size is a limiting factor, all cases included in this study had at least some degree of immunolabeling for MAP2. Furthermore, the majority of gliomas were scored as either a score 2 (38.5%) or a score 3 (38.5%), with significant correlation between diagnosis of glioma and immunolabel intensity (P = 0.04). MAP2 expression in human glioma reveals different immunolabeling patterns between oligodendroglioma (i.e., sparse processes, that in our study corresponds to lack of CtP immunolabeling and prominent PNc and Ct immunolabeling) and astrocytoma (i.e., densely ramified astrocytic elements, that correspond to predominately CtP staining in majority of the canine astrocytoma in this study) (37). All oligodendrogliomas (53/53; 100%) and undefined gliomas (12/12; 100%) in this study had a combination of PNc and Ct immunolabeling. Except for three astrocytomas (3/13; 23%) that had a combination of PNc and Ct immunolabeling, the majority of astrocytomas had a combination of PNc and CtP staining (10/13; 77%), with robust highlighting of cytoplasmic processes. Significant correlation between staining pattern and diagnosis was obtained (P = 0.001).
Based on these findings, MAP2 appears to be a suitable diagnostic immunohistochemical marker for canine glioma, with strong potential of aiding in the diagnosis of astrocytoma based on the pattern of immunolabeling. However, the number of cases exhibiting multifocal to coalescing (16/78; 20.5%) and patchy immunolabeling (20/78; 26%) illustrate the heterogeneity present in these tumors and raise a concern that the use of tissue microarray for immunohistochemical analysis in canine glioma may yield results that are not entirely representative of the totality of the neoplasm (33, 38). The three cases of astrocytoma with PNc and Ct staining were all from one location (the cerebellar white matter) and had an unusual pattern composed of sheets of gemistocyte-like morphology. Whether this corresponds to a specific subtype of astrocytoma remains to be determined; however, tumors of this morphology have been described at this location in the dog (6). No correlation could be demonstrated between tumor grade and MAP2 immunolabeling intensity score, and this may be due to the small size and heterogeneity of the sample. The distribution of the data is summarized in Supplemental Figures 1, 2.
The overall statistical significance is difficult to interpret due to a limited and somewhat heterogeneous sample size; however, the IHC results presented herein suggest that MAP2 can be a valuable marker in the evaluation of canine glioma. Furthermore, the staining pattern obtained in this study is similar with findings in human gliomas (37), making this marker a tool for better understanding protein expression in canine glioma, but also for differentiating astrocytomas from oligodendrogliomas and undefined gliomas. Future directions of study should be focused on understanding the role that neuronal differentiation has in the pathogenesis of canine glioma.
Data Availability Statement
All datasets generated for this study are included in the article/Supplementary Material.
Ethics Statement
This study protocol did not require ethics approval according to local legislation and national guidelines.
Author Contributions
This study was conceived by AM and ED. Case acquisition and interpretation was done by AM, ED, BP, CF, and DR. Experiments were performed by AM and ED. Data interpretation was done by AM and ED. Paper writing and editing was done by AM, ED, BP, CF, and DR.
Conflict of Interest
The authors declare that the research was conducted in the absence of any commercial or financial relationships that could be construed as a potential conflict of interest.
Acknowledgments
The authors acknowledge the assistance of the histology technicians at Cornell University College of Veterinary Medicine.
Supplementary Material
The Supplementary Material for this article can be found online at: https://www.frontiersin.org/articles/10.3389/fvets.2019.00395/full#supplementary-material
Supplemental Figure 1. Distribution of all data evaluated by JMP software.
Supplemental Figure 2. Distribution of diagnoses by the criteria considered for MAP2 immunolabeling evaluation (scoring, intensity, distribution, pattern) achieved with JMP software.
References
1. Dolecek TA, Propp JM, Stroup NE, Kruchko C. CBTRUS statistical report: primary brain and central nervous system tumors diagnosed in the United States in 2005–2009. Neuro Oncol. (2012) 14(Suppl. 5):1–49. doi: 10.1093/neuonc/nos218
2. Dickinson PJ. Advances in diagnostic and treatment modalities for intracranial tumors. J Vet Intern Med. (2014) 28:1165–85. doi: 10.1111/jvim.12370
3. Hicks J, Platt S, Kent M, Haley A, Canine brain tumors: a model for the human disease. Vet Comp Oncol. (2015) 15:252–72. doi: 10.1111/vco.12152
4. Herranz C, Fernández F, Martín-Ibáñez R, Blasco E, Crespo E, De la Fuente C, et al. Spontaneously arising canine glioma as a potential model for human glioma. J Comp Pathol. (2016) 154:169–79. doi: 10.1016/j.jcpa.2015.12.001
5. Hubbard ME, Arnold S, Bin Zahid A, McPheeters M, Gerard O'Sullivan M, Tabaran AF, et al. Naturally occurring canine glioma as a model for novel therapeutics. Cancer Invest. (2018) 36:415–23. doi: 10.1080/07357907.2018.1514622
6. Koehler JW, Miller AD, Miller CR, Porter B, Aldape K, Beck J, et al. A revised diagnostic classification of canine glioma: towards validation of the canine glioma patient as a naturally occurring preclinical model for human glioma. J Neuropathol Exp Neurol. (2018) 77:1039–54. doi: 10.1093/jnen/nly085
7. Motta L, Mandara MT, Skerritt GC. Canine and feline intracranial meningiomas: an updated review. Vet J. (2012) 192:153–65. doi: 10.1016/j.tvjl.2011.10.008
8. Johnson GC, Coates JR, Wininger F. Diagnostic immunohistochemistry of canine and feline intracalvarial tumors in the age of brain biopsies. Vet Pathol. (2014) 51:146–60. doi: 10.1177/0300985813509387
9. Higgins RJ, Bollen AW, Dickinson PJ, Sisó-Llonch S. Tumors of the nervous system. In: Meuten DJ, ed. Tumors in Domestic Animals. Ames, IA: John Wiley & Sons Inc. (2017). p. 834–49.
10. Snyder JM, Shofer FS, Van Winkle TJ, Massicotte C. Canine intracranial primary neoplasia: 173 cases (1986-2003). J Vet Intern Med. (2006) 20:669–75. doi: 10.1111/j.1939-1676.2006.tb02913.x
11. Song RB, Vite CH, Bradley CW, Cross JR. Postmortem evaluation of 435 cases of intracranial neoplasia in dogs and relationship of neoplasm with breed, age, and body weight. J Vet Intern Med. (2013) 27:1143–52. doi: 10.1111/jvim.12136
12. Stoica G, Levine J, Wolff J, Murphy K. Canine astrocytic tumors: a comparative review. Vet Pathol. (2011) 48:266–75. doi: 10.1177/0300985810389543
13. Dorn CR, Taylor DO, Schneider R, Hibbard HH, Klauber MR. Survey of animal neoplasms in Alameda and Contra Costa Counties, California. II Cancer morbidity in dogs and cats from Alameda County. J Natl Cancer Inst. (1968) 40:307–18.
14. Platt SR, Alleman AR, Lanz OI, Chrisman CL. Comparison of fine-needle aspiration and surgical-tissue biopsy in the diagnosis of canine brain tumors. Vet Surg. (2002) 31:65–9. doi: 10.1053/jvet.2002.29456
15. Evans SM, Dayrell-Hart B, Powlis W, Christy G, VanWinkle T. Radiation therapy of canine brain masses. J Vet Intern Med. (1993) 7:216–9. doi: 10.1111/j.1939-1676.1993.tb01010.x
16. Stoica G, Kim HT, Hall DG, Coates JR. Morphology, immunohistochemistry, and genetic alterations in dog astrocytomas. Vet Pathol. (2004) 41:10–9. doi: 10.1354/vp.41-1-10
17. Rossmeisl JH, Duncan RB, Huckle WR, Troy GC. Expression of vascular endothelial growth factor in tumors and plasma from dogs with primary intracranial neoplasms. Am J Vet Res. (2007) 68:1239–45. doi: 10.2460/ajvr.68.11.1239
18. Dickinson PJ, Roberts BN, Higgins RJ, Leutenegger CM, Bollen AW, Kass PH, et al. Expression of receptor tyrosine kinases VEGFR-1 (FLT-1), VEGFR-2 (KDR), EGFR-1, PDGFR alpha and c-Met in canine primary brain tumours. Vet Comp Oncol. (2006) 4:132–40. doi: 10.1111/j.1476-5829.2006.00101.x
19. Dickinson PJ, York D, Higgins RJ, LeCouteur RA, Joshi N, Bannasch D. Chromosomal aberrations in canine gliomas define candidate genes and common pathways in dogs and humans. J Neuropathol Exp Neurol. (2016) 75:700. doi: 10.1093/jnen/nlw042
20. Ligon KL, Alberta JA, Kho AT, Weiss J, Kwaan MR, Nutt CL, et al. The oligodendroglial lineage marker OLIG2 is universally expressed in diffuse gliomas. J Neuropathol Exp Neurol. (2004) 63:499–509. doi: 10.1093/jnen/63.5.499
21. Louis DN, Ohgaki H, Wiestler OD, Cavanee WK. WHO Classification of Tumours of the Central Nervous System. 4th ed. Lyon: IARC (2007).
22. Boudreau CE, York D, Higgins RJ, LeCouteur RA, Dickinson PJ. Molecular signalling pathways in canine gliomas. Vet Comp Oncol. (2017) 15:133–50. doi: 10.1111/vco.12147
23. Chen J, McKay RM, Parada LF. Malignant glioma: lessons from genomics, mouse models, and stem cells. Cell. (2012) 149:36–47. doi: 10.1016/j.cell.2012.03.009
24. Fraser AR, Bacci B, le Chevoir MA, Long SN. Epidermal growth factor receptor and Ki-67 expression in canine gliomas. Vet Pathol. (2016) 53:1131–7. doi: 10.1177/0300985816644301
25. Takahashi K, Yamanaka S. Induction of pluripotent stem cells from mouse embryonic and adult fibroblast cultures by defined factors. Cell. (2006) 126:663–76. doi: 10.1016/j.cell.2006.07.024
26. Uhrbom L, Kastemar M, Johansson FK, Westermark B, Holland EC. Cell type-specific tumor suppression by Ink4a and Arf in Kras-induced mouse gliomagenesis. Cancer Res. (2005) 65:2065–9. doi: 10.1158/0008-5472.CAN-04-3588
27. Kishimoto TE, Uchida K, Thongharb A, Shibato T, Chambers JK, Nibe K, et al. Expression of oligodendrocyte precursor cell markers in canine oligodendrogliomas. Vet Pathol. (2018) 55:634–44. doi: 10.1177/0300985818777794
28. Dehmelt L, Halpain S. The MAP2/Tau family of microtubule-associated proteins. Genome Biol. (2005) 6:204. doi: 10.1186/gb-2004-6-1-204
29. Roger B, Al-Bassam J, Dehmelt L, Milligan RA, Halpain S. MAP2c, but not tau, binds and bundles F-actin via its microtubule binding domain. Curr Biol. (2004) 14:363–71. doi: 10.1016/j.cub.2004.01.058
30. Zhou Y, Wu S, Liang C, Lin Y, Zou Y, Li K, et al. Transcriptional upregulation of microtubule-associated protein 2 is involved in the protein kinase A-induced decrease in the invasiveness of glioma cells. Neuro Oncol. (2015) 17:1578–88. doi: 10.1093/neuonc/nov060
31. Shafit-Zagardo B, Davies P, Rockwood J, Kress Y, Lee SG. Novel microtubule-associated protein 2 isoform is expressed early in human oligodendrocyte maturation. Glia. (2000) 29:233–45. doi: 10.1002/(SICI)1098-1136(20000201)29:3<233::AID-GLIA5>3.0.CO;2-U
32. van Belle G. Statistical Rules of Thumb. Chapter 2: Sample Size. 2nd ed. Hoboken, New Jersey: John Wiley & Sons Inc. (2008). doi: 10.1002/9780470377963
33. Wohlsein P, Recker T, Rohn K, Baumgartner W. Validation of usefulness of tissue microarray technology in primary tumors of the canine and feline central nervous system. J Comp Pathol. (2012) 146:320–6. doi: 10.1016/j.jcpa.2011.08.006
34. Wharton SB, Chan KK, Whittle IR. Microtubule-associated protein 2 (MAP-2) is expressed in low and high-grade diffuse astrocytomas. J Clin Neurosci. (2002) 9:165–9. doi: 10.1054/jocn.2001.1055
35. Blumcke I, Becker AJ, Normann S, Hans V, Riederer BM, Krajewski S, et al. Distinct expression pattern of microtubule-associated protein 2 in human oligodendrogliomas and glial precursor cell. J Neuropathol Exp Neurol. (2001) 61:74–9. doi: 10.1093/jnen/60.10.984
36. Suzuki SO, Kitai R, Llena J, Lee SC, Goldman JE, Shafit-Zagardo B. MAP-2e, a novel MAP-2 isoform, is expressed in gliomas and delineates tumor architecture and patterns of infiltration. J Neuropathol Exp Neurol. (2002) 61:403–12. doi: 10.1093/jnen/61.5.403
37. Blümcke I, Müller S, Buslei R, Riederer BM, Wiestler OD. Microtubule-associated protein-2 immunoreactivity: a useful tool in the differential diagnosis of low-grade neuroepithelial tumors. Acta Neuropathol. (2004) 108:89–96. doi: 10.1007/s00401-004-0873-8
Keywords: microtubule-associated protein 2, canine, glioma, oligodendroglioma, astrocytoma, immunohistochemistry
Citation: Demeter EA, Frank C, Rissi DR, Porter BF and Miller AD (2019) Microtubule-Associated Protein 2 Expression in Canine Glioma. Front. Vet. Sci. 6:395. doi: 10.3389/fvets.2019.00395
Received: 21 July 2019; Accepted: 24 October 2019;
Published: 15 November 2019.
Edited by:
David K. Meyerholz, University of Iowa, United StatesReviewed by:
Lauren E. Himmel, Vanderbilt University Medical Center, United StatesChristiane V. Löhr, Oregon State University, United States
Copyright © 2019 Demeter, Frank, Rissi, Porter and Miller. This is an open-access article distributed under the terms of the Creative Commons Attribution License (CC BY). The use, distribution or reproduction in other forums is permitted, provided the original author(s) and the copyright owner(s) are credited and that the original publication in this journal is cited, in accordance with accepted academic practice. No use, distribution or reproduction is permitted which does not comply with these terms.
*Correspondence: Andrew D. Miller, adm10@cornell.edu