- 1Department of Small Animal Medicine and Surgery, University of Veterinary Medicine Hannover, Hanover, Germany
- 2Institute for Biometry, Epidemiology and Information Processing, University of Veterinary Medicine, Hanover, Germany
Neutrophil gelatinase-associated Lipocalin (NGAL) is a glycoprotein involved in inflammation acting as an acute phase protein and chemokine as well as a regulator of iron homeostasis. NGAL has been shown to be upregulated in experimental autoimmune encephalomyelitis (EAE) in mice. Increased NGAL concentration in cerebrospinal fluid (CSF) and expression in central nervous system (CNS) has been described in human neuroinflammatory disease such as multiple sclerosis and neuropsychiatric lupus as well as in bacterial meningitis. We aimed to investigate involvement of NGAL in spontaneous canine neuroinflammation as a potential large animal model for immune- mediated neurological disorders. A commercially available Enzyme-linked Immunosorbent Assay (ELISA) for detection of canine NGAL was validated for use in canine CSF. Concentration in CSF and serum of canine patients suffering from steroid- responsive meningitis- arteriitis (SRMA), Meningoencephalitis of unknown origin (MUO), different non- inflammatory CNS disease and control dogs were compared. Relationship between NGAL concentration in CSF and serum and inflammatory parameters in CSF and blood (IgA concentration, total nucleated cell count (TNCC), protein content) as well as association with erythrocytes in CSF, duration of illness, plasma creatinine and urinary leucocytes were evaluated. In dogs with SRMA and MUO, CSF concentration of NGAL was significantly higher than in dogs with idiopathic epilepsy, compressive myelopathy, intracranial neoplasia and SRMA in remission (p < 0.0001). Patients with acute SRMA had significantly higher levels of NGAL in CSF than neurologically normal controls (p < 0.0001). Serum NGAL concentrations were significantly higher in dogs with SRMA than in patients with myelopathy and intracranial neoplasia (p < 0.0001). NGAL levels in CSF were strongly positively associated with IgA concentration (rSpear= 0.60116, p < 0.0001), TNCC (rSpear= 0.65746, p < 0.0001) and protein content (rSpear= 0.73353, p < 0.0001) in CSF. It can be measured in CSF of healthy and diseased dogs. Higher concentrations in canine patients with SRMA as well as positive association with TNCC in CSF suggest an involvement in pro-inflammatory pathways and chemotaxis in SRMA. High serum levels of NGAL in serum of SRMA patients in different stages of disease might reflect the systemic character of the disease.
Introduction
Neutrophil gelatinase-associated Lipocalin (NGAL) or Lipocalin-2 (LCN2), is a versatile glycoprotein acting as an acute-phase protein as well as part of the innate immune system mainly by its function as a siderophore-binding protein (1). It is produced and stored in neutrophils (2), macrophages (3), but also in astrocytes (4, 5) and the choroid plexus (6) and many other tissues (7, 8). In the central nervous system (CNS), neuroprotective (9, 10), as well as detrimental impact during neuroinflammatory processes has been described (11–13). NGAL induces astrocytosis (13, 14), and enhances immune cell migration (15–17) especially via recruitment of neutrophils (18, 19).
Increased NGAL concentration was found in cerebrospinal fluid (CSF) of human patients with bacterial meningitis (20, 21) but also in patients with autoimmune CNS disease such as multiple sclerosis (22, 23) and neuropsychiatric lupus (24). It was also detected in brain tissue in experimental animal studies after application of lipopolysaccharides (25) and has been shown to be upregulated in mice with experimental autoimmune encephalomyelitis (EAE) which serves as a model for human multiple sclerosis (5).
Until now, data regarding concentration of NGAL in CSF of healthy dogs and canine patients with inflammatory and non-inflammatory CNS disease is lacking. Further investigation of spontaneous inflammation of the central nervous system in dogs (26–28) could be useful for closing the gap between experimental animal models and investigation of human spontaneous autoimmune neuroinflammation.
This study aimed to test the hypothesis that NGAL concentrations in CSF would be higher in steroid-responsive meningitis-arteriitis (SMRA) and MUO (Meningoencephalitis of unknown origin) than in non-inflammatory (idiopathic epilepsy, intracranial neoplasia, intervertebral disc disease) CNS disease and neurological healthy dogs. We also hypothesized that there would be a correlation with the total nucleated cell count (TNCC) in CSF, possibly due to chemotaxis.
The overall goal of the study is improvement of differentiation of CNS diseases and amelioration of the understanding of the etiopathology of suspected autoimmune canine CNS disease like SRMA and MUO by detection of a new biomarker in canine CSF. Better understanding of chemokine signaling pathways and modulation of neuroinflammation could help to develop more specific treatment strategies for aforementioned pathologies (5, 13, 29, 30).
Materials and Methods
Serum and Cerebrospinal Fluid Samples
Paired serum and CSF samples were collected for routine diagnostic workup between December 2011 and May 2018 at the Department for Small Animal Medicine and Surgery of the University of Veterinary Medicine, Hannover, Germany, from client–owned dogs presented to the neurology group and healthy, university-owned beagles (animal experiment number 33.9-42502-05-14A453). The specimens were aliquoted and stored at −20°C prior to measurement of NGAL concentrations. The study was conducted according to the University's ethical guidelines.
Animals
The dogs underwent general and neurological examination, complete blood cell count (CBC), blood chemistry, CSF analysis including IgA measurement and individual combination of further specific testing (urine analysis, orthopedic examination, radiographs, testing for infectious agents in CSF and/or blood, advanced diagnostic imaging such as magnetic resonance imaging or computed tomography, abdominal or heart ultrasound, electrodiagnostics, surgery, histopathology) to obtain a definitive or highly likely diagnosis (Table 2).
Dogs were retrospectively assigned to different groups regarding diagnosis: SRMA acute (untreated), SRMA recurrence, SRMA in remission (under therapy with glucocorticosteroids), MUO (including confirmed and presumed cases, see Supplementary Table 4), intracranial neoplasia, compressive myelopathy [intervertebral disc herniation (IVDH) or spondylomyelopathy], idiopathic epilepsy (IE), others (behavioral abnormalities, idiopathic vestibular syndrome, bacterial meningoencephalitis). The diagnoses were defined according to published data (Table 2).
Healthy dogs and dogs with final diagnosis of orthopedic disease with a normal general and neurological examination and laboratory values in the reference range served as control group (“healthy/orthopedic”).
Tissue samples were obtained following routine necropsy after spontaneous death or euthanasia on owner's request due to poor prognosis and were conducted in accordance with the German Animal Welfare Act within the law of animal welfare, Germany, and following the ethical guidelines of the University of Veterinary Medicine Hannover. No dogs were euthanized for this particular study; sample aliquots were obtained for clinical diagnostics or previously attained during other studies (animal experiment number 33.9-42502-05-14A453).
Data Collection
Information regarding duration of clinical signs, comorbidities, signalment, and course of disease as well as blood and urine parameters and if available biopsy or necropsy results were retrospectively evaluated using the digital clinical data software (easyVET, VetZ GmbH, Isernhagen, Germany). If possible, follow up was conducted via control appointments or phone calls to owners or local practitioners (see Supplementary Table 2 for signalement, urine, and renal parameters).
Quantification of Neutrophil Gelatinase-Associated Lipocalin
A commercially available Sandwich Enzyme-linked immunosorbent Assay (ELISA) established for canine NGAL in urine, plasma, serum, tissue extracts, or culture media (36–40) was used following manufacturer's instructions (Dog NGAL ELISA Kit 043, Bioporto, Hellerup, Denmark). It was validated for measurement of NGAL- concentration in pooled, aliquoted and frozen canine CSF by determination of intraassay and interassay reproducibility and analysis of recovery rate using CSF samples spiked with calibrator fluid. Intraassay reproducibility was calculated by measuring pooled CSF in seven replicates (each as duplet) and calculation of the coefficient of variance (CV). Interassay reproducibility was tested calculating CV for pooled and aliquoted CSF measured in duplicates on five different dates.
Concentration of NGAL in paired serum and CSF samples was afterwards measured following manufacturer's instructions. The mean minimum detectable value given by the manufacturer was 0.56 pg/mL. If the value exceeded the highest value of the standard curve (400 pg/mL), the sample was diluted and measured again. All samples were analyzed in duplicates and mean values were calculated.
Quantification of Immunoglobulin A
IgA in aliquoted serum and CSF samples was measured prior to this study as part of CSF analysis in-house using an ELISA as previously described (41).
Data Analysis
Data was analyzed using the statistical software SAS Enterprise Guide 9.3 (SAS Institute Inc., Cary, NC, USA). The Kolmogorov–Smirnov-Test was used to analyze the data for normal distribution. This was repeated after identification and exclusion of outliers. Because data did not conform to Gaussian distribution, non-parametric tests consisting of Kruskal–Wallis test, Dunn's-post hoc test and Wilcoxon two-sample test were performed. Wilcoxon two-sample test was used to compare all groups pairwise (see Supplementary Table 1). Values of p < 0.0001 were considered significant when comparing medians of the means. Spearman's rank correlation coefficients were calculated to analyze associations between NGAL concentration in serum and CSF, IgA concentration, and NGAL concentration in serum and CSF, NGAL concentration and nucleated cell count in CSF, NGAL concentration in CSF and erythrocyte count in CSF, NGAL concentration and duration of illness in inflammatory disease, NGAL concentration in serum and creatinine concentration in blood plasma, and NGAL concentration in serum and presence of leucocytes in urine detected by Combur stick (Roche Deutschland Holding GmbH, Mannheim, Germany). As stability of NGAL in frozen canine samples has not been reported, linear regression analysis by groups was conducted to identify possible influence of sampling year and storage period on NGAL concentration in canine CSF and serum. Scatter graphs from the obtained data were created using GraphPad software (GraphPad Prism™ ®, version 5, La Jolla, CA, USA).
Results
Validation of ELISA for Use of NGAL Measurement in Cerebrospinal Fluid
Recovery rate of the four CSF samples spiked with calibrator fluid is shown in Table 1. For intraassay reproducibility, the coefficient of variance (CV = 3.9%, median NGAL concentration 387.85 pg/ml) was calculated. Interassay reproducibility was tested calculating CV for pooled CSF (CV = 6.2%, median NGAL concentration 648.875 pg/ml).
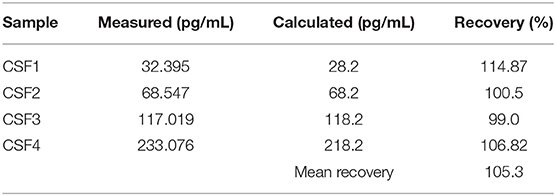
Table 1. Recovery rate of four CSF dilutions spiked with calibrator fluid. Recovery was calculated as (“Measured”/“Calculated”) × 100%.
Canine Cerebrospinal Fluid and Serum Samples
In total, 163 CSF and 157 paired serum samples of 140 individual patients were included (Table 2). Twenty-five of the patients in the acute SRMA group also had samples included in “SRMA therapy” and three had samples included in “SRMA recurrence.” One SRMA patient only had samples analyzed as a part of the SRMA recurrence group, but SRMA acute and SRMA control samples were not available. All other patients had only samples from one time point included.
Concentration of NGAL in Canine CSF and Serum
NGAL was detected in all available CSF and serum samples of patients of the different groups. NGAL concentrations in CSF and Serum of the disease groups and controls are shown in Figures 1, 2. Table 3 reveals median concentrations of NGAL (pg/ml) in canine CSF and serum as well as ranges. The highest concentrations of NGAL in CSF were found in dogs with acute SRMA and MUO. Canine NGAL serum concentration was overall higher than in CSF with highest concentration in dogs with acute SRMA, SRMA in remission under therapy and SRMA recurrences.
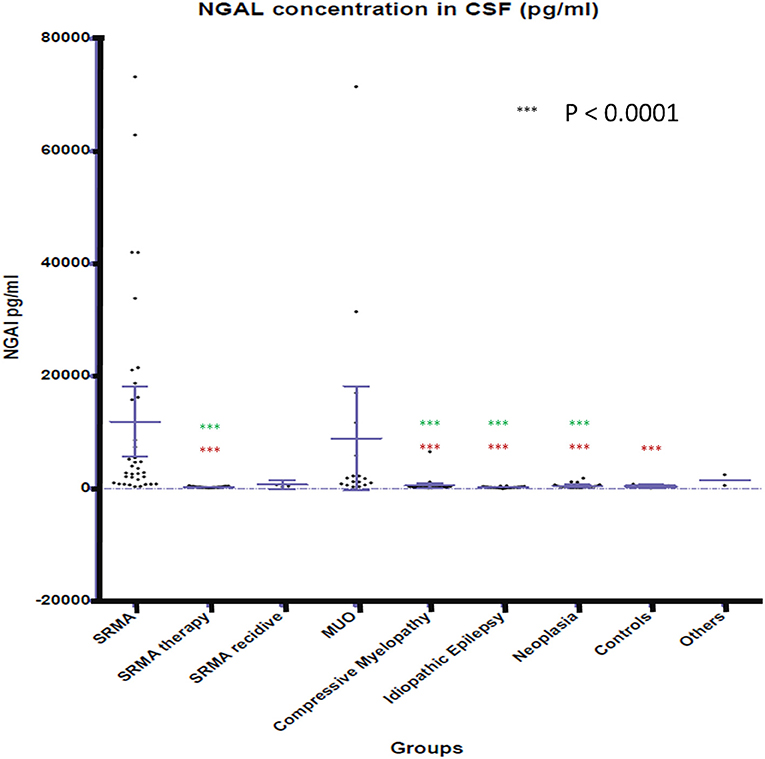
Figure 1. NGAL concentrations in CSF of the different groups. Boxes contain values from the first to the third quartile; lines inside the boxes indicate median values; end of vertical lines show minimum and maximum values; dots represent outliers. Red asterisks (***) represent groups differing significantly (p < 0.0001) from acute SRMA group. Green asterisks (***) represent groups differing significantly from MUO group. NGAL, neutrophil gelatinase-associated lipocalin; SRMA, steroid-responsive meningitis-arteriitis; CSF, cerebrospinal fluid; MUO, meningoencephalitis of unknown origin; IE, idiopathic epilepsy; “SRMA therapy” consisted of dogs under treatment without clinical signs, “SRMA recidive” consisted of dogs with relapse of clinical signs, “Myelopathy” group consisted of dogs with intervertebral disc herniation or compressive spondylomyelopathy, “Neoplasia” included dogs with primary and secondary brain tumors, “Controls” included patients without neurological disorders (healthy and orthopedic), “Others” included various neurological diseases (bacterial meningoencephalitis, idiopathic vestibular syndrome, behavioral abnormalities).
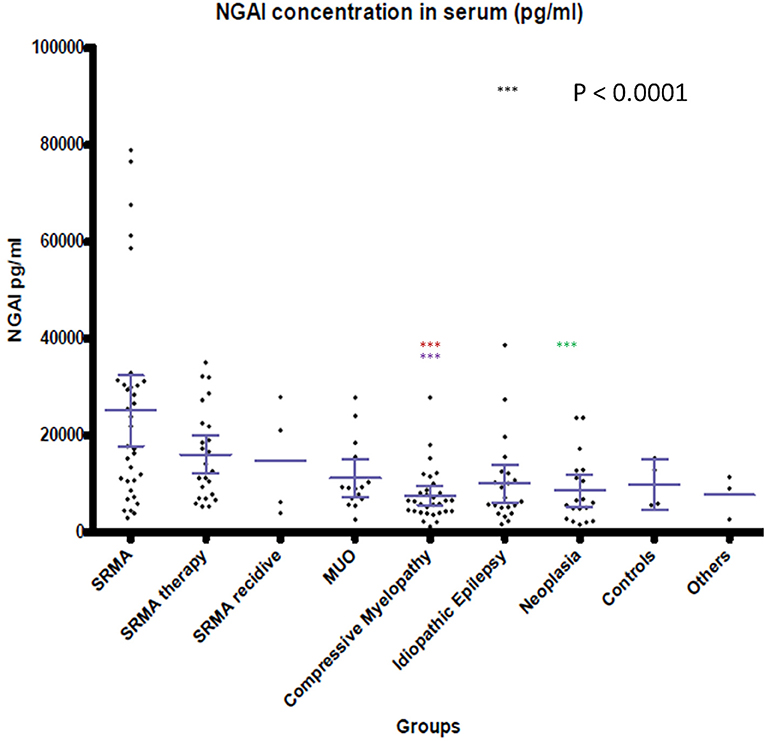
Figure 2. NGAL concentrations in serum of the different groups. Boxes contain values from the first to the third quartile; lines inside the boxes indicate median values; end of vertical lines show minimum and maximum values; dots represent outliers. Red asterisks (***) represent groups differing significantly (p < 0.0001) from acute SRMA group. Lilac arterisks (***) represent groups differing significantly from SRMA therapy group. Green arterisks (***) indicate groups differing significantly from SRMA acute group only in Dunn's post hoc test. NGAL, neutrophil gelatinase-associated lipocalin; SRMA, steroid-responsive meningitis-arteriitis; CSF, cerebrospinal fluid; MUO, meningoencephalitis of unknown origin; IE, idiopathic epilepsy; “SRMA therapy” consisted of dogs under treatment without clinical signs, “SRMA recidive” consisted of dogs with relapse of clinical signs, “Myelopathy” group consisted of dogs with intervertebral disc herniation or compressive spondylomyelopathy, “Neoplasia” included dogs with primary and secondary brain tumors, “Controls” included patients without neurological disorders (healthy and orthopedic), “Others” included various neurological diseases (bacterial meningoencephalitis, idiopathic vestibular syndrome, behavioral abnormalities).
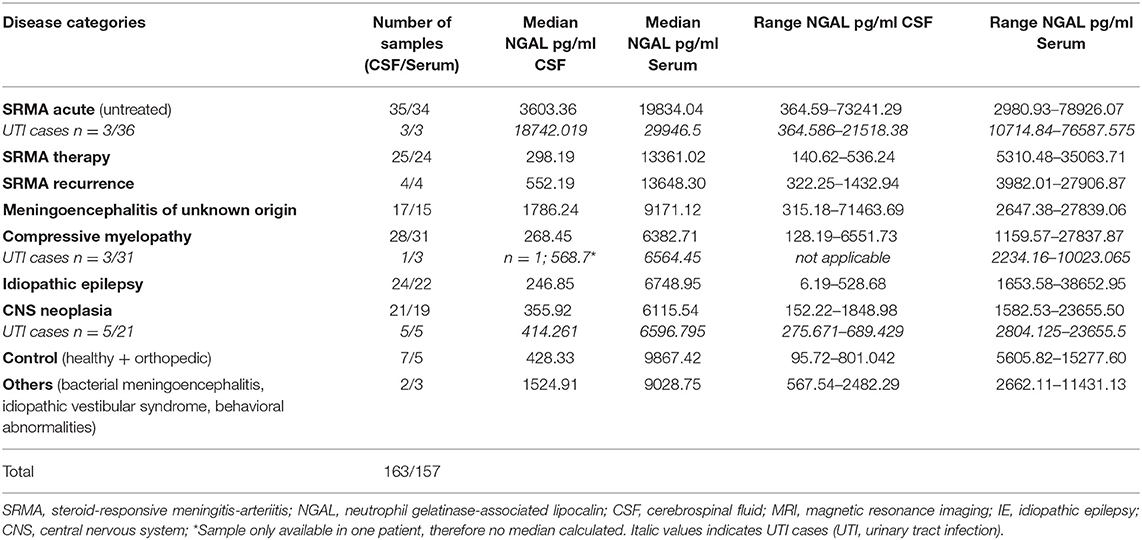
Table 3. Median concentrations and ranges of NGAL (pg/ml) in canine CSF and serum in different groups.
Kruskal–Wallis-Test indicated a significant difference in CSF NGAL levels [χ2(8) = 95.9672; p < 0.0.0001] between the different groups. Wilcoxon rank sum test results are shown in Supplementary Table 1. NGAL concentrations in CSF differed significantly (p < 0.0001) between acute SRMA patients and the group with dogs displaying SRMA in remission under corticosteroid therapy, the group with idiopathic epilepsy, intracranial neoplasia, compressive myelopathy and the control group. NGAL concentrations in CSF were significantly higher in dogs with MUO than in SRMA patients in remission under therapy, idiopathic epilepsy patients, the compressive myelopathy group as well as than in dogs suffering from intracranial neoplasia (p < 0.0001; Supplementary Table 1).
Kruskal- Wallis test identified significant differences for NGAL serum concentration when comparing the nine groups [χ2(8) = 36.4667; p < 0.0001]. Using Wilcoxon-ranked sum test, in dogs with acute SRMA and SRMA in remission, serum levels of NGAL were significantly higher than in serum of dogs with compressive myelopathy (p < 0.0001; Supplementary Table 1). Additionally, Kruskal–Wallis-Dunn's test identified a difference between NGAL serum levels in dogs with acute SRMA and intracranial neoplasia [difference = 93.42, SE = 12.51, q = 7.47, q(0.05) = 3.197].
Correlation Analysis
As shown in Table 4, NGAL levels in CSF and serum were weakly positively associated. NGAL concentration in CSF, but not in serum was strongly positively associated with IgA concentration in CSF and serum, respectively. There was a strong, positive association of NGAL concentration in CSF with total nucleated cell count (lc/3ul) in CSF and a strong, positive association with protein content in CSF. Erythrocyte count in CSF was weakly positively associated with NGAL concentration in canine CSF (see Supplementary Table 3 for an overview of amount of red blood cells, leucocytes, and NGAL in CSF).
There was a weak, negative association between duration of illness in inflammatory disease and level of NGAL in CSF. Duration of illness in inflammatory disease (SRMA, MUO) showed a moderate negative association with NGAL concentration in canine serum.
A weak, positive association was detected between NGAL serum levels and concentration of creatinine in blood plasma and leucocytes in urine.
Linear Regression Analysis to Identify Influence of Sample Year on NGAL Concentration
Linear regression analysis by group did not identify significant linear regression coefficients of NGAL in CSF or serum through year of sampling (p > 0.05).
Discussion
In the current study, a commercially available NGAL ELISA was validated for use in canine CSF and, for the first time, concentration of NGAL in CSF and serum in dogs with different inflammatory and non-inflammatory disease was described.
NGAL in canine CSF was shown to be elevated in dogs with inflammatory disease (SRMA and MUO) in comparison to non-inflammatory CNS disease categories (compressive myelopathy, neoplasia, IE). A positive association between NGAL concentration in CSF and TNCC in CSF was found, which could indicate enhanced chemotaxis as described in experimental studies (15–17) and increased permeability of the blood brain barrier, for example via disruption of tight junctions as described in rodent models with artificial stroke (42, 43). When assessing the different subgroups against each other, CSF levels of NGAL in MUO (confirmed and presumed cases) and acute SRMA were significantly higher than in dogs with idiopathic epilepsy, compressive myelopathy, intracranial neoplasia, and SRMA in remission. CSF levels in acute SRMA, but not SRMA recurrence and MUO were significantly higher than in control dogs without neurological disease. The group of dogs with SRMA recurrence was very small (n = 4) due to sample availability and rare occurrence of relapses in our SRMA population. Therefore, explanatory power is questionable for this group. MUO on the other hand represents a very heterogenous group because different entities are covered by this umbrella term (26, 28), as long as no histopathological diagnosis is available. In our study, confirmed and presumed MUO cases were included (see also Supplementary Table 4). For further studies, inclusion of histopathologically confirmed MUO cases only would be desirable.
The strong, positive association of NGAL levels in CSF and protein content in CSF could be caused by aforementioned blood brain barrier disruption and influx of proteins from the periphery or, on the other hand, be caused by increased intrathecal immunoglobulin synthesis as well as NGAL synthesis in neuroinflammation. The strong positive association of NGAL and IgA in CSF most probably reflects the local synthesis of immunoglobulins in the CNS (41) and of NGAL. Intrathecal production of NGAL in endothelial and glial cells as well as in the choroid plexus is suspected, as it was shown previously in rodent models and human samples (5, 6, 11, 19, 22, 23). Positive association of CSF NGAL and IgA levels could indicate overlapping signaling pathways. Chemokines and proteins which have been described to play an important role in SRMA are Il-6 (44), IL-8 (45), IL-17 (46), MMP2 and MMP9 (47). Those have also been described in cell culture and experimental murine or porcine studies to be induced by NGAL or to increase NGAL expression themselves (48–54). In a glial cell model and murine EAE, NGAL treated cells expressed higher levels of MMP9 and upregulated MMP9 was suspected to increase blood brain barrier damage (12). Concentration of NGAL in canine CSF and serum were only weakly positively associated, additionally supporting the theory of intrathecal NGAL synthesis in dogs.
NGAL in CSF of clinically normal dogs with SRMA in remission under corticosteroid therapy, which were presented for CSF examination several weeks after initiation of therapy, did show significant decrease of levels compared to acute, untreated SRMA. Interestingly, in those dogs, serum NGAL levels remained high in the course of the disease as it has also been described for IgA levels in dogs (55, 56). This finding could be worth further investigation in a prospective study with SRMA cases only and synchronized sampling intervals, as it might help in diagnosis of atypical or protracted SRMA cases. Our findings contradict an experimental study with pigs receiving hydrocortisone prior to iatrogenic induced endotoxemia in which hydrocortisone counteracted NGAL plasma increase (57). This might be due to different time points of measurement as plasma NGAL concentration in the experimental study was only sampled up to 6 h after hydrocortisone application whereas in our study, sampling took place before steroid treatment in the acute SRMA group and weeks or months after initiation of corticosteroid therapy in the dogs with SRMA in remission under therapy.
NGAL levels in serum differed significantly between acute SRMA cases and SRMA patients in remission when compared to compressive myelopathy patients, but this was not found in MUO patients. In contrast to MUO, SRMA is considered a systemic disease and is associated with neutrophilic pleocytosis in CSF and peripheral blood (26, 27, 41). Studies on murine and human cell cultures and experimental studies with lcn2 knockout mice revealed an especially high impact of NGAL on neutrophil function (16, 17). MUO is not a homogenous pathology as it includes different diseases like granulomatous meningoencephalitis or necrotizing encephalitis (26, 28), which is typically associated with mononuclear pleocytosis in CSF but not necessarily pleocytosis in peripheral blood (31). This might explain why median ranked values of serum NGAL levels in MUO patients did not differ significantly in statistical analysis compared to the controls and non-inflammatory disease groups. Dunn's Kruskal–Wallis post-hoc test also identified a significant difference between serum samples of acute SRMA and intracranial neoplasia. This could as well-represent the systemic nature of SRMA in contrast to intracranial neoplasia as only one of the included patients suffered from metastatic neoplastic disease (prostatic carcinoma). However, this result should be considered with caution, as there was detectable overlap of concentrations in those two groups as can be seen in Figure 2. Apart from that, no statistically significant differences between the remaining serum groups were detected. This could reflect heterogeneously composed groups not only in MUO patients but also in intracranial neoplasia and the compressive myelopathy group, where different entities, combined with possible underlying diseases as well as acute and more chronic myelopathies were included, possibly leading to different grades of disease severity at timepoint of sampling. Medians and ranges of serum levels were overall comparable to published data regarding serum NGAL levels in dogs (36, 58–60) but our results raise the suspicion, as postulated before (58, 61), that higher levels of NGAL in serum alone might not be specific for renal disease due to its complex role in a variety of other peripheral inflammatory and neoplastic pathologies. In the future, further studies comparing NGAL concentration in serum in systemic inflammatory, renal and other diseases could be useful. As NGAL is also acting as a regulator of iron metabolism (1) and might be increased in case of hemorrhage, which is possible in SRMA (62) because of necrotizing vasculitis, we investigated if there is an association of erythrocyte content and NGAL levels in CSF (see also Supplementary Table 3). For those parameters, only a weak, positive association with questionable relevance was detected, so NGAL levels might not always be influenced by hemorrhage depending for example on chronicity of disease or the erythrocytes detected in our CSF samples might have been present due to iatrogenic contamination. Further studies should include a group of patients with intracerebral hemorrhage or vascular incidents to investigate NGAL CSF levels in CSF and serum in those patients.
In humans and dogs, serum and urinary NGAL have been investigated as an early marker of renal dysfunction (36, 59, 63) and urinary NGAL was shown to be increased in dogs with urinary tract infection (37). Therefore, we also included data regarding renal parameters and urine analysis (see Supplementary Table 2) but found only a weak, positive association between NGAL concentration in serum and plasma creatinine levels or leucocytes in urine. Nevertheless, statistical evaluation could be biased as only a small number of dogs included in the study were diagnosed with renal disease (n = 2, diagnosed by histopathology) and none of the patients showed increased plasma creatinine at time of sampling for CSF and serum. Sixteen dogs had clinical signs of urinary tract infection (UTI) at time of sampling (five dogs with intracranial neoplasia, three dogs with acute SRMA and compressive myelopathy each, two dogs diagnosed with IE and one each in the groups of SRMA in remission, SRMA recurrence and MUO, see Supplementary Table 2).
Outliers, which showed markedly increased NGAL concentration in CSF were identified. In the acute SRMA group, one of the highest measured NGAL concentration belonged to a patient who later relapsed after reduction of prednisolone dosage, but the other three outliers either had a good outcome (follow up period 7 and 17 months, respectively) or were lost to follow up (n = 1). In the MUO group, the patient with highest NGAL concentration was euthanized directly after diagnosis on owner's request and an unusual, marked sterile pyogenic meningoencephalitis was found in necropsy. Nevertheless, the other two outliers had a good or satisfying outcome. One patient with very high NGAL CSF levels in the group with compressive myelopathy initially had a good outcome but developed another intervertebral disc herniation at another location 4 months later and the other outlier was a patient who had undergone hemilaminectomy 1 year prior. One of the patients with very high NGAL levels in CSF in the neoplasia group had a suspected choroid plexus tumor, an interesting fact, as the choroid plexus has been described as one of the main intrathecal areas of NGAL synthesis (6). No histopathological confirmation of tumor type was available in this dog. In one other patient with very high NGAL CSF levels, histopathology after euthanasia on owner's request identified meningioma with secondary surrounding encephalitis in the brainstem. The third outlier showed central vestibular signs and had an extraaxial cerebellar mass with suspicion of meningioma, but necropsy was not provided.
In serum, one very high NGAL concentration in the acute SRMA group belonged to a patient who relapsed under reduced prednisolone dosage, but the other two patients had a good outcome (in both patients follow up period 2 months). No remarkable features were detectable in the other outliers regarding NGAL in serum in the myelopathy and idiopathic epilepsy group, who had either a good outcome or were lost to follow up.
A correlation of high serum NGAL concentration with negative outcome in patients with sepsis as well as traumatic brain injury has been described (64, 65). Therefore, in the future we aim to investigate possible correlation of NGAL concentration in serum and CSF of dogs with diverse CNS disease and their clinical outcome. Moreover, as NGAL levels in CSF differed significantly between MUO and intracranial neoplasia, it might be a promising biomarker for differentiating categories of mass lesions in canines in addition to MRI, if biopsies are declined due to risks of the procedure (66). A limitation of the presented study is the retrospective nature and that necropsy or biopsy results were not provided for all cases either due to ethical concerns of the owners or good outcome mostly in SRMA cases. Interestingly, we found detectable NGAL concentration in CSF not only in glioma as described in human tumor tissue (67), but also in confirmed meningioma cases and a metastatic brain tumor, which might indicate secondary neuroinflammation in dogs with brain tumors.
Treatment of SRMA and MUO consists of long- term immunosuppressant monotherapy with glucocorticosteroids or with additional immunomodulatory or chemotherapeutic drugs. Treatment so far is unspecific and bears risk of adverse effects as well as insufficient response (28, 61). Therefore, adapted therapy for immune-mediated neuroinflammatory diseases targeting specific signaling pathways or via modulation of gene expression is urgently needed. Different drugs were shown to decrease NGAL levels in animal models including usage of nanotherapeutics for down regulation of NGAL synthesis (29), application of the iron chelator deferoxamine in a traumatic brain injury model in rats (30) and modulation of NGAL in EAE-mice treated with natalizumab which is currently used in human multiple-sclerosis (5). The current study might provide the foundation for future treatment studies of canine neuroinflammation.
In conclusion, NGAL can be detected in canine CSF using commercially available ELISA. It is increased in CSF of dogs with SRMA compared to controls and non-inflammatory disorders and also is higher in dogs with MUO compared to dogs with idiopathic epilepsy, compressive myelopathy and intracranial neoplasia. Concentration of NGAL in CSF of dogs with SRMA in remission under therapy with corticosteroids is significantly lower than in acute SRMA- and MUO- patients. NGAL levels in canine serum were significantly higher in patients with SRMA compared to those suffering from myelopathy reflecting unspecific systemic disease, but not necessarily indicating renal failure as proposed before. NGAL is a promising biomarker in canine CSF and serum, potentially adding another piece of the puzzle to the etiopathology of autoimmune neuroinflammation.
Data Availability
The datasets generated for this study are available on request to the corresponding author.
Ethics Statement
Ethical review and approval was not required for the animal study because it is a retrospective study using data and samples acquired previously. Tissue samples were obtained following routine necropsy after spontaneous death or euthanasia on owner's request due to poor prognosis and were conducted in accordance with the German Animal Welfare Act within the law of animal welfare, Germany, and following the ethical guidelines of the University of Veterinary Medicine Hannover. No dogs were euthanized for this particular study; sample aliquots were obtained for clinical diagnostics or previously attained during other studies (animal experiment number 33.9-42502-05-14A453). Written informed consent was obtained from the owners for the participation of their animals in this study.
Author Contributions
AT conceived, designed, and supervised the study. NM assisted with study design, collected and analyzed data, performed the experiments, and wrote the first draft of the manuscript. KR gave substantial contribution to the statistical analysis of data. RC conceived in part the study and gave substantial contributions to acquisition, analysis and interpretation of the data. All authors contributed to the critical revision of the manuscript for important intellectual content and have read and approved the final version.
Funding
This publication was supported by Deutsche Forschungsgemeinschaft and University of Veterinary Medicine Hannover, Foundation within the funding programme Open Access Publishing.
Conflict of Interest Statement
The authors declare that the research was conducted in the absence of any commercial or financial relationships that could be construed as a potential conflict of interest.
Acknowledgments
The authors wish to express their gratitude to the whole staff of the Neurology Service of the Department of Small Animal Medicine and Surgery, University of Veterinary Medicine, Hannover, Germany helping examining the animals and collecting the samples. Preliminary parts of the data included in this study have been presented as a research poster at 30th Annual Symposium of the ESVN-ECVN 2017 in Helsinki, Finland and at the 4th International Workshop of Veterinary Neuroscience, Bern, Switzerland 2018.
Supplementary Material
The Supplementary Material for this article can be found online at: https://www.frontiersin.org/articles/10.3389/fvets.2019.00315/full#supplementary-material
Supplementary Table 1. Wilcoxon rank sum test results.
Supplementary Table 2. Summary of the signalement, creatinine concentration, leucocytes in urine, urinary tract disease, duration of illnes prior to sample date in patients with inflammatory disease.
Supplementary Table 3. Overview of NGAl level, totan nucleated cell count, and erythrocyte count in CSF.
Supplementary Table 4. Overview of MUO cases (histopathology if available, follow- up period, outcome).
Abbreviations
CBC, complete blood count; CSF, cerebrospinal fluid; CNS, central nervous system; CV, coefficient of variance; EAE, experimental autoimmune encephalomyelitis; ELISA, enzyme-linked immunosorbent assay; Dept., department; IE, idiopathic epilepsy; IG, immunoglobulin; IL, interleukin; IVDH, intervertebral disc herniation; lc, leucocyte; LCN2, lipocalin 2; MMP, matrix- metalloproteinase; MRI, magnetic resonance imaging;MUO, meningoencephalitis of unknown origin; NGAL, neutrophil gelatinase- associated lipocalin; rSpear, Spearman's rank correlation coefficient; SRMA, steroid- responsive meningitis- arteriitis; TNCC, total nucleated cell count; UTI, urinary tract infection.
References
1. Goetz DH, Holmes MA, Borregaard N, Bluhm ME, Raymond KN, Strong RK. The neutrophil lipocalin NGAL is a bacteriostatic agent that interferes with siderophore-mediated iron acquisition. Mol Cell. (2002) 10:1033–43. doi: 10.1016/S1097-2765(02)00708-6
2. Kjeldsen L, Bainton DF, Sengelov H, Borregaard N. Identification of neutrophil gelatinase-associated lipocalin as a novel matrix protein of specific granules in human neutrophils. Blood. (1994) 83:799–807.
3. Jung M, Weigert A, Tausendschön M, Mora J, Ören B, Sola A, et al. Interleukin-10-induced neutrophil gelatinase-associated lipocalin production in macrophages with consequences for tumor growth. Mol Cell Biol. (2012) 32:3938–48. doi: 10.1128/MCB.00413-12
4. Chun BY, Kim JH, Nam Y, Huh MI, Han S, Suk K. Pathological involvement of astrocyte-derived lipocalin-2 in the demyelinating optic neuritis. Invest Ophthalmol Vis Sci. (2015) 56:3691–8. doi: 10.1167/iovs.15-16851
5. Marques F, Mesquita SD, Sousa JC, Coppola G, Gao F, Geschwind DH, et al. Lipocalin 2 is present in the EAE brain and is modulated by natalizumab. Front Cell Neurosci. (2012) 6:33. doi: 10.3389/fncel.2012.00033
6. Marques F, Rodrigues AJ, Sousa JC, Coppola G, Geschwind DH, Sousa N, et al. Lipocalin 2 is a choroid plexus acute-phase protein. J Cereb Blood Flow Metab. (2008) 28:450–5. doi: 10.1038/sj.jcbfm.9600557
7. Chakraborty S, Kaur S, Guha S, Batra SK. The multifaceted roles of neutrophil gelatinase associated lipocalin (NGAL) in inflammation and cancer. Biochim Biophys Acta. (2012) 1826:129–69. doi: 10.1016/j.bbcan.2012.03.008
8. Cowland JB, Borregaard N. Molecular characterization and pattern of tissue expression of the gene for neutrophil gelatinase-associated lipocalin from humans. Genomics. (1997) 45:17–23. doi: 10.1006/geno.1997.4896
9. Tang W, Ma J, Gu R, Ding X, Lei B, Wang X, et al. Lipocalin 2 suppresses ocular inflammation by inhibiting the activation of NF-κβ pathway in endotoxin-induced uveitis. Cell Physiol Biochem. (2018) 46:375–88. doi: 10.1159/000488472
10. Kang SS, Ren Y, Liu CC, Kurti A, Baker KE, Bu G, et al. Lipocalin-2 protects the brain during inflammatory conditions. Mol Psychiatry. (2018) 23:344–50. doi: 10.1038/mp.2016.243
11. Jha MK, Lee S, Park DH, Kook H, Park KG, Lee IK, et al. Diverse functional roles of lipocalin-2 in the central nervous system. Neurosci Biobehav Rev. (2015) 49:135–56. doi: 10.1016/j.neubiorev.2014.12.006
12. Nam Y, Kim JH, Seo M, Kim JH, Jin M, Jeon S, et al. Lipocalin-2 protein deficiency ameliorates experimental autoimmune encephalomyelitis: the pathogenic role of lipocalin-2 in the central nervous system and peripheral lymphoid tissues. J Biol Chem. (2014) 289:16773–89. doi: 10.1074/jbc.M113.542282
13. Suk K. Lipocalin-2 as a therapeutic target for brain injury: an astrocentric perspective. Prog Neurobiol. (2016) 144:158–72. doi: 10.1016/j.pneurobio.2016.08.001
14. Lee S, Park JY, Lee WH, Kim H, Park HC, Mori K, et al. Lipocalin-2 is an autocrine mediator of reactive astrocytosis. J Neurosci. (2009) 29:234–49. doi: 10.1523/JNEUROSCI.5273-08.2009
15. Lee S, Kim JH, Kim JH, Seo JW, Han HS, Lee WH, et al. Lipocalin-2 is a chemokine inducer in the central nervous system: role of chemokine ligand 10 (CXCL10) in lipocalin-2-induced cell migration. J Biol Chem. (2011) 286:43855–70. doi: 10.1074/jbc.M111.299248
16. Schroll A, Eller K, Feistritzer C, Nairz M, Sonnweber T, Moser PA, et al. Lipocalin-2 ameliorates granulocyte functionality. Eur J Immunol. (2012) 42:3346–57. doi: 10.1002/eji.201142351
17. Shashidharamurthy R, Machiah D, Aitken JD, Putty K, Srinivasan G, Chassaing B, et al. Differential role of lipocalin 2 during immune complex-mediated acute and chronic inflammation in mice. Arthritis Rheum. (2013) 65:1064–73. doi: 10.1002/art.37840
18. Asimakopoulou A, Borkham-Kamphorst E, Tacke F, Weiskirchen R. Lipocalin-2 (NGAL/LCN2), a “help-me” signal in organ inflammation. Hepatology. (2016) 63:669–71. doi: 10.1002/hep.27930
19. Ferreira AC, Dá Mesquita S, Sousa JC, Correia-Neves M, Sousa N, Palha JA, et al. From the periphery to the brain: lipocalin-2, a friend or foe? Prog Neurobiol. (2015) 131:120–36. doi: 10.1016/j.pneurobio.2015.06.005
20. Guiddir T, Deghmane AE, Giorgini D, Taha MK. Lipocalin 2 in cerebrospinal fluid as a marker of acute bacterial meningitis. BMC Infect Dis. (2014) 14:276. doi: 10.1186/1471-2334-14-276
21. Lippi G, Avanzini P, Calzetti C, Caleffi A, Pipitone S, Musa R, et al. The role of neutrophil gelatinase-associated lipocalin (NGAL) in cerebrospinal fluids for screening of acute bacterial meningitis. Clin Lab. (2014) 60:377–81. doi: 10.7754/Clin.Lab.2013.121239
22. Berard JL, Zarruk JG, Arbour N, Prat A, Yong VW, Jacques FH, et al. Lipocalin 2 is a novel immune mediator of experimental autoimmune encephalomyelitis pathogenesis and is modulated in multiple sclerosis. Glia. (2012) 60:1145–59. doi: 10.1002/glia.22342
23. Al Nimer F, Elliott C, Bergman J, Khademi M, Dring AM, Aeinehband S, et al. Lipocalin-2 is increased in progressive multiple sclerosis and inhibits remyelination. Neurol Neuroimmunol Neuroinflamm. (2016) 3:e191. doi: 10.1212/NXI.0000000000000191
24. Mike EV, Makinde HM, Gulinello M, Vanarsa K, Herlitz L, Gadhvi G, et al. Lipocalin-2 is a pathogenic determinant and biomarker of neuropsychiatric lupus. J Autoimmun. (2019) 96:59–73. doi: 10.1016/j.jaut.2018.08.005
25. Ip JP, Noçon AL, Hofer MJ, Lim SL, Müller M, Campbell IL. Lipocalin 2 in the central nervous system host response to systemic lipopolysaccharide administration. J Neuroinflammation. (2011) 8:124. doi: 10.1186/1742-2094-8-124
26. Vitale S, Foss K. Immune-mediated central nervous system disease—current knowledge and recommendations. Top Companion Anim Med. (2019) 34:22–9. doi: 10.1053/j.tcam.2018.11.003
27. Black VL, Whitworth FJS, Adamantos S. Pyrexia in juvenile dogs: a review of 140 referred cases. J Small Anim Pract. (2018) 60:116–20. doi: 10.1111/jsap.12938
28. Talarico LR, Schatzberg SJ. Idiopathic granulomatous and necrotising inflammatory disorders of the canine central nervous system: a review and future perspectives. J Small Anim Pract. (2010) 51:138–49. doi: 10.1111/j.1748-5827.2009.00823.x
29. Smith JA, Braga A, Verheyen J, Basilico S, Bandiera S, Alfaro-Cervello C, et al. RNA nanotherapeutics for the amelioration of astroglial reactivity. Mol Ther Nucleic Acids. (2018) 10:103–21. doi: 10.1016/j.omtn.2017.11.008
30. Zhao J, Xi G, Wu G, Keep RF, Hua Y. Deferoxamine attenuated the upregulation of lipocalin-2 induced by traumatic brain injury in rats. Acta Neurochir Suppl. (2016) 121:291–4. doi: 10.1007/978-3-319-18497-5_50
31. Granger N, Smith PM, Jeffery ND. Clinical findings and treatment of non- infectious meningoencephalomyelitis in dogs: a systematic review of 457 published cases from 1962 to 2008. Vet J. (2010) 184:290–7. doi: 10.1016/j.tvjl.2009.03.031
32. Jeffery ND, Levine JM, Olby NJ, Stein VM. Intervertebral disk degeneration in dogs: consequences, diagnosis, treatment, and future directions. J Vet Intern Med. (2013) 27:1318–33. doi: 10.1111/jvim.12183
33. Da Costa R. Cervical spondylomyelopathy (wobbler syndrome) in dogs. Vet Clin Small Anim. (2010) 40:881–913. doi: 10.1016/j.cvsm.2010.06.003
34. De Risio L, Bhatti S, Muñana K, Penderis J, Stein V, Tipold A, et al. International veterinary epilepsy task force consensus proposal: diagnostic approach to epilepsy in dogs. BMC Vet Res. (2015) 11:148. doi: 10.1186/s12917-015-0462-1
35. Dickinson PJ. Advances in diagnostic and treatment modalities for intracranial tumors. J Vet Intern Med. (2014) 28:1165–85. doi: 10.1111/jvim.12370
36. Ahn HJ, Hyun C. Evaluation of serum neutrophil gelatinase-associated lipocalin (NGAL) activity in dogs with chronic kidney disease. Vet Rec. (2013) 173:452. doi: 10.1136/vr.101829
37. Daure E, Belanger MC, Beauchamp G, Lapointe C. Elevation of neutrophil gelatinase-associated lipocalin (NGAL) in non-azotemic dogs with urinary tract infection. Res Vet Sci. (2013) 95:1181–5. doi: 10.1016/j.rvsc.2013.09.002
38. Kai K, Yamaguchi T, Yoshimatsu Y, Kinoshita J, Teranishi M, Takasaki W. Neutrophil gelatinase-associated lipocalin, a sensitive urinary biomarker of acute kidney injury in dogs receiving gentamicin. J Toxicol Sci. (2013) 38:269–77. doi: 10.2131/jts.38.269
39. Segev E, Palm C, LeRoy B, Cowgill LD, Westropp JL. Evaluation of neutrophil gelatinase-associated lipocalin as a marker of kidney injury in dogs. J Vet Intern Med. (2013) 27:1362–7. doi: 10.1111/jvim.12180
40. Nabity MB, Lees GE, Cianciolo R, Boggess MM, Steiner JM, Suchodolski JS. Urinary biomarkers of renal disease in dogs with X-linked hereditary nephropathy. J Vet Intern Med. (2012) 26:282–93. doi: 10.1111/j.1939-1676.2012.00891.x
41. Tipold A, Pfister H, Zurbriggen A, Vandevelde M. Intrathecal synthesis of major immunoglobulin classes in inflammatory diseases of the canine CNS. Vet Immunol Immunopathol. (1994) 42:149–59. doi: 10.1016/0165-2427(94)90004-3
42. Egashira Y, Hua Y, Keep RF, Iwama T, Xi G. Lipocalin 2 and blood-brain barrier disruption in white matter after experimental subarachnoid hemorrhage. J Cereb Blood Flow Metab. (2014) 34:1306–14.
43. Jin M, Kim JH, Jang E, Lee YM, Soo Han H, Woo DK, et al. Lipocalin-2 deficiency attenuates neuroinflammation and brain injury after transient middle cerebral artery occlusion in mice. J Cereb Blood Flow Metab. (2014) 34:1306–14. doi: 10.1038/jcbfm.2014.83
44. Maiolini A, Otten M, Hewicker-Trautwein M, Carlson R, Tipold A. Interleukin-6, vascular endothelial growth factor and transforming growth factor beta 1 in canine steroid responsive meningitis-arteriitis. BMC Vet Res. (2013) 9:23. doi: 10.1186/1746-6148-9-23
45. Burgener I, Van Ham L, Jaggy A, Vandevelde M, Tipold A. Chemotactic activity and IL-8 levels in the cerebrospinal fluid in canine steroid responsive meningitis-arteriitis. J Neuroimmunol. (1998) 89:182–90. doi: 10.1016/S0165-5728(98)00134-9
46. Freundt-Revilla J, Maiolini A, Carlson R, Beyerbach M, Rentmeister K, Flegel T, et al. Th17-skewed immune response and cluster of differentiation 40 ligand expression in canine steroid-responsive meningitis-arteriitis, a large animal model for neutrophilic meningitis. J Neuroinflammation. (2017) 14:20. doi: 10.1186/s12974-016-0784-3
47. Schwartz M, Puff C, Stein VM, Baumgartner W, Tipold A. Marked MMP-2 transcriptional up-regulation in mononuclear leukocytes invading the subarachnoidal space in aseptic suppurative steroid-responsive meningitis-arteriitis in dogs. Vet Immunol Immunopathol. (2010) 133:198–206. doi: 10.1016/j.vetimm.2009.08.007
48. Tveita AA, Rekvig OP, Zykova SN. Increased glomerular matrix metalloproteinase activity in murine lupus nephritis. Kidney Int. (2008) 74:1150–8. doi: 10.1038/ki.2008.308
49. Hamzic N, Blomqvist A, Nilsberth C. Immune-induced expression of lipocalin-2 in brain endothelial cells: relationship with interleukin-6, cyclooxygenase-2 and the febrile response. J Neuroendocrinol. (2013) 25:271–80. doi: 10.1111/jne.12000
50. Te Boekhorst BC, Bovens SM, Hellings WE, van der Kraak PH, van de Kolk KW, Vink A, et al. Molecular MRI of murine atherosclerotic plaque targeting NGAL: a protein associated with unstable human plaque characteristics. Cardiovasc Res. (2011) 89:680–8. doi: 10.1093/cvr/cvq340
51. Shao S, Cao T, Jin L, Li B, Fang H, Zhang J, et al. Increased lipocalin-2 contributes to the pathogenesis of psoriasis by modulating neutrophil chemotaxis and cytokine secretion. J Invest Dermatol. (2016) 136:1418–28. doi: 10.1016/j.jid.2016.03.002
52. Yan L, Borregaard N, Kjeldsen L, Moses MA. The high molecular weight urinary matrix metalloproteinase (MMP) activity is a complex of gelatinase B/MMP-9 and neutrophil gelatinase-associated lipocalin (NGAL). Modulation of MMP-9 activity by NGAL. J Biol Chem. (2001) 276:37258–65. doi: 10.1074/jbc.M106089200
53. Xu MJ, Feng D, Wu H, Wang H, Chan Y, Kolls J, et al. Liver is the major source of elevated serum lipocalin-2 levels after bacterial infection or partial hepatectomy: a critical role for IL-6/STAT3. Hepatology. (2015) 61:692–702. doi: 10.1002/hep.27447
54. Ferreira MC, Whibley N, Mamo AJ, Siebenlist U, Chan YR, Gaffen SL. Interleukin-17-induced protein lipocalin 2 is dispensable for immunity to oral candidiasis. Infect Immun. (2014) 82:1030–5. doi: 10.1128/IAI.01389-13
55. Lowrie M, Penderis J, McLaughlin M, Eckersall PD, Anderson TJ. Steroid responsive meningitis-arteriitis: a prospective study of potential disease markers, prednisolone treatment, and long-term outcome in 20 dogs (2006-2008). J Vet Intern Med. (2009) 23:862–70. doi: 10.1111/j.1939-1676.2009.0337.x
56. Maiolini A, Carlson R, Schwartz M, Gandini G, Tipold A. Determination of immunoglobulin A concentrations in the serum and cerebrospinal fluid of dogs: an estimation of its diagnostic value in canine steroid-responsive meningitis–arteritis. Vet J. (2012) 191:219–24. doi: 10.1016/j.tvjl.2010.12.018
57. Söderberg E, Eriksson M, Larsson A, Lipcsey M. The impact of hydrocortisone treatment on neutrophil gelatinase-associated lipocalin release in porcine endotoxemic shock. Intensive Care Med Exp. (2017) 5:4. doi: 10.1186/s40635-017-0117-6
58. Cobrin AR, Blois SL, Abrams-Ogg AC, Kruth SA, Dewey C, Holowaychuk MK, et al. Neutrophil gelatinase-associated lipocalin in dogs with chronic kidney disease, carcinoma, lymphoma and endotoxaemia. J Small Anim Pract. (2016) 57:291–8. doi: 10.1111/jsap.12481
59. Hsu WL, Lin YS, Hu YY, Wong ML, Lin FY, Lee YJ. Neutrophil gelatinase-associated lipocalin in dogs with naturally occurring renal diseases. J Vet Intern Med. (2014) 28:437–42. doi: 10.1111/jvim.12288
60. Jung HB, Kang MH, Park HM. Evaluation of serum neutrophil gelatinase-associated lipocalin as a novel biomarker of cardiorenal syndrome in dogs. J Vet Diagn Invest. (2018) 30:386–91. doi: 10.1177/1040638718758430
61. Mårtensson J, Bellomo R. The rise and fall of NGAL in acute kidney injury. Blood Purif. (2014) 37:304–10. doi: 10.1159/000364937
62. Tipold A, Schatzberg SJ. An update on steroid responsive meningitis-arteriitis. J Small Anim Pract. (2010) 51:150–4. doi: 10.1111/j.1748-5827.2009.00848.x
63. Haase-Fielitz A, Haase M, Devarajan P. Neutrophil gelatinase-associated lipocalin as a biomarker of acute kidney injury: a critical evaluation of current status. Ann Clin Biochem. (2014) 51(Pt 3):335–51. doi: 10.1177/0004563214521795
64. Wang B, Chen G, Zhang J, Xue J, Cao Y, Wu Y. Increased neutrophil gelatinase-associated lipocalin is associated with mortality and multiple organ dysfunction syndrome in severe sepsis and septic shock. Shock. (2015) 44:234–8. doi: 10.1097/SHK.0000000000000408
65. Shen LJ, Zhou J, Guo M, Yang CS, Xu QC, Lv QW, et al. Serum lipocalin-2 concentrations and mortality of severe traumatic brain injury. Clin Chim Acta. (2017) 474:130–5. doi: 10.1016/j.cca.2017.09.016
66. Flegel T, Oevermann A, Oechtering G, Matiasek K. Diagnostic yield and adverse effects of MRI-guided free-hand brain biopsies through a mini-burr hole in dogs with encephalitis. J Vet Intern Med. (2012) 26:969–76. doi: 10.1111/j.1939-1676.2012.00961.x
Keywords: neutrophil gelatinase-associated lipocalin, chemokine, neuroinflammation, autoimmune disease, biomarker, steroid-responsive meningitis-arteriitis, meningoencephalitis of unknown origin, canine
Citation: Meyerhoff N, Rohn K, Carlson R and Tipold A (2019) Measurement of Neutrophil Gelatinase-Associated Lipocalin Concentration in Canine Cerebrospinal Fluid and Serum and Its Involvement in Neuroinflammation. Front. Vet. Sci. 6:315. doi: 10.3389/fvets.2019.00315
Received: 05 July 2019; Accepted: 04 September 2019;
Published: 18 September 2019.
Edited by:
Andrea Fischer, Ludwig Maximilian University of Munich, GermanyReviewed by:
Theresa Elizabeth Pancotto, Virginia Tech, United StatesThomas Robert Harcourt-Brown, University of Bristol, United Kingdom
Copyright © 2019 Meyerhoff, Rohn, Carlson and Tipold. This is an open-access article distributed under the terms of the Creative Commons Attribution License (CC BY). The use, distribution or reproduction in other forums is permitted, provided the original author(s) and the copyright owner(s) are credited and that the original publication in this journal is cited, in accordance with accepted academic practice. No use, distribution or reproduction is permitted which does not comply with these terms.
*Correspondence: Nina Meyerhoff, nina.meyerhoff@tiho-hannover.de