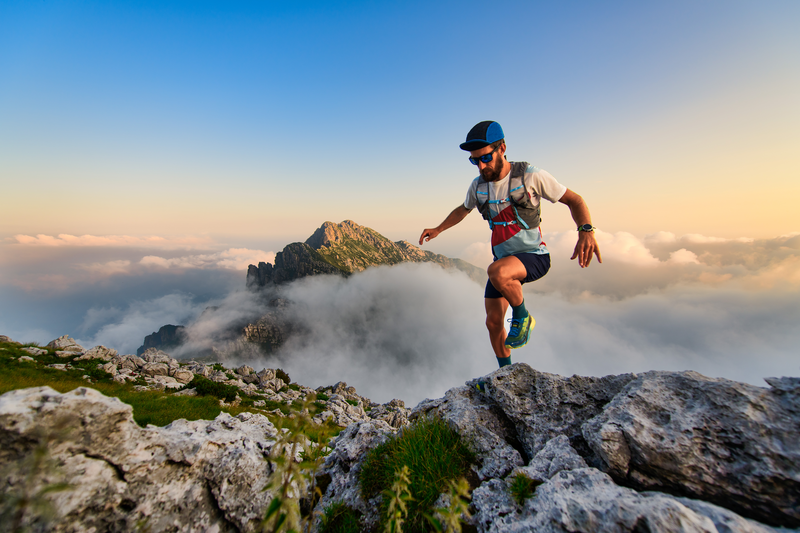
95% of researchers rate our articles as excellent or good
Learn more about the work of our research integrity team to safeguard the quality of each article we publish.
Find out more
ORIGINAL RESEARCH article
Front. Vet. Sci. , 05 September 2018
Sec. Animal Nutrition and Metabolism
Volume 5 - 2018 | https://doi.org/10.3389/fvets.2018.00201
The objective of this study was to evaluate the use of vegetable oils from plants grown in Brazil, first using the in vitro batch culture, and then evaluating the oil with methane (CH4) reducing potential in an in vivo experiment. The in vitro experiment was conducted as a completely randomized design using the seven contrasting oils. Treatments consisted of a control and 3 increasing concentrations (0, 1, 2, and 5% v/v) of oil added to a tifton 85 hay samples. All vegetable oils linearly decreased (P < 0.01) gas production after 24 h of incubation, with the greatest reduction when 5% of oil was included into the diet. Açaí and buriti had no effect of CH4 (% or mL/g DM incubated) however carrot, macaúba, basil, passionflower, and pequi oil all linearly decreased (P < 0.01) CH4 production with increasing inclusion rate of oil. Pequi oil resulted in the largest decrease in CH4 production (mL/g DM incubated) after 24 h of in vitro incubation. The objective of the in vivo experiment was to evaluate the effects of pequi oil on nutrient digestibility, CH4 production, and rumen fermentation parameters in wethers fed a hay-based diet. The experiment was conducted as a 2 × 2 Latin Square design using 4 Dorper wethers (63.4 ± 1.46 kg body weight). There were 2 experimental periods of 21 d each, with d 1–14 used for diet adaptation and d 15–21 for measurements and collections. The treatments consisted of a control diet and pequi oil fed at 70 g per animal per day. The addition of pequi oil to the diet had no effect on feed intake or the digestibility of nutrients, however there was a numerical decrease in the population of cellulolytic bacteria. There was a tendency (P = 0.06) for pequi oil addition to decrease CH4 production (g/d) by 17.5%. From this study, we can conclude that pequi oil may be used as a suitable oil for reducing CH4 production from ruminants, with no negative effects on intake or digestibility.
Brazil has the fifth largest land mass and is home to the third largest cattle population. However due to increasing populations, it has been estimated that beef production in Brazil still needs to increase by 22.8% and dairy production by 29.8% to meet the demands for beef and milk products (1). Due to its large land mass, Brazil has high potential to meet these production needs (2). Increasing environmental awareness has also pressured agricultural sectors to seek alternative methods to reduce enteric methane (CH4) production from ruminants.
With restrictions placed on using great proportions of concentrates as well antibiotic use, there is a focus on “natural” products to manipulate rumen fermentation, particularly using plant-based products. Lipid supplementation is considered a promising strategy for dietary manipulation, with the capacity to decrease CH4 emissions without effecting performance (3). Lipids can act in different ways to suppress methanogenesis; including suppression of ciliate protozoa and archaea, biohydrogenation of free unsaturated fatty acids, reduction in organic matter fermentation, and replacing fermentable carbohydrates (4–6). However, the potential of individual lipid sources in reducing enteric CH4 production is dependent on the fatty acid composition (7–9).
Plant oil and oilseeds have been shown to consistently lower methane emissions over time without reduction in performance characteristics (10), however total fat content of the diet can only be increased up to 6–7% until feed intake is decreased (11). Several studies have compared the effects of supplementing different vegetable oils on feed intake and animal performance with results differing based on the type of oil supplemented (7–9).
The Caryocar brasiliense Camb tree, common name pequi, produces a fruit which is high in oil. This tree is endemic to Brazil, however is spread throughout South America (12). Pequi oil is high in the unsaturated fatty acid, oleic acid, as well as the saturated fatty acid palmitic acid. The oil has also shown to have antibacterial properties possibly due to high carotenoids concentration (13). Additionally, research facilities are currently interested in the cultivation of this tree, as well as its possible use as a feed resource (12). The objective of this study was to first investigate the effects of non-conventional oils derived from açaí, buriti, carrot, macaúba, basil, passionfruit, and pequi on rumen fermentation and CH4 production using the in vitro gas production technique and select the most promising oil for an in vivo study. Subsequently, the objective of the second study was to evaluate the effect of pequi oil on rumen fermentation, apparent nutrient digestibility, enteric CH4 production, and microbial population in sheep fed a hay-based diet.
The experiments were conducted at the Bioenergetic Laboratory of Multi-use Complex on Livestock Bio-efficiency and Sustainability of the Brazilian Agricultural Research Corporation, Embrapa (Coronel Pacheco, Minas Gerais, Brazil), Latitude: 21° 55′67′ 'S, Longitude: 43° 26′ 90″ W and altitude: 414 meters. The climate is classified as tropical with an average temperature of 25.6°C; relative humidity of 77% and annual mean rainfall of 1,400 mm.
All animal management and handling procedures were approved by Embrapa Dairy Cattle Animal Care and Use Committee. For the in vitro trial - Protocol CEUA—EGL n° 29/2015 and for the in vivo—Protocol CEUA No. 7080090616.
The experiment was conducted as a completely randomized design with a control (100% Tifton 85 hay) and 3 increasing concentrations of vegetal oils [1, 2, and 5% (v/v)] sourced from Brazil (Table 1). The concentrations were chosen to obtain quadratic responses and calculated based on the volume of incubation media (e.g., 25 mL). Tifton 85 hay was used as the substrate and was ground to 1 mm and weighed into the incubation vials (0.5 g DM per vial) 1 d before incubation. The oils [açaí (Euterpe oleracea), buriti (Mauritia flexuosa), carrot (Daucus carota), macaúba (Acrocomia aculeata), basil (Ocimum basilicum), passionfruit (Passiflora edulis), and pequi (Caryocar brasiliense)] were obtained from Mundo dos Óleos (Brasilia, DF, Brazil), and were pipetted into each vial 1 h prior to incubation. The vegetable oils volumes added were 0.25, 0.5, and 1.25 mL for 1, 2, and 5% (v/v), respectively.
Table 1. Concentration of fatty acids (g/100 g of total fatty acids) identified in the oils used in in vitro batch cultures.
Rumen inoculum was pooled from 3 ruminally cannulated crossbred non-lactating dairy cows (Holstein × Gyr), 2 h after feeding. Cows were fed a total mixed ration (TMR) consisting of corn silage, Tifton 85 hay, concentrate (forage to concentrate; 53:47% DM) and a minerals mixture. Rumen contents were filtered by gauze. The fluid was then placed in a thermos heated to 39°C and immediately taken to the laboratory.
The in vitro incubations were conducted using bottles (50 mL) sealed with rubber stoppers. On the day of incubation, bottles with the substrate were kept at 39°C in an incubator and continuously carbonated with CO2 at the time of incubation. To begin the incubation, 25 mL of culture medium was dispensed into each vial, then immediately sealed and placed into the incubator. Culture medium consisted of ruminal fluid and buffer solution at a ratio of 1:2, respectively (14). The incubator was maintained at 39 ± 0.5°C and bottles were kept on a rotary shaker at 90 oscillation/min.
The gas volume was quantified at 8 and 24 h of incubation using water displacement technique (15) and the total production was calculated by adding the values obtained in the two readings. Gas samples were taken to quantify methane (CH4) production after 8 h of incubation, where 10 mL of gas were collected by inserting a 20 mL syringe through the septum, before gas pressure measurement. This gas was then transferred to previously evacuated 5.9 mL exetainers (Labco Ltd., High Wycombe, England). From each exetainer, 3 mL was removed for CH4 analysis (GC-FID Equipment Agilent Technologies 7820A-CH4: Gas Phase Chromatograph, Agilent Technologies, Model 7820A, Chromatography GC-FID EzChrom Elite interface software). After measuring the gas production at 24 h, the rubber stoppers were removed, and the pH was measured using a digital pH meter (Tecnopon, model MPa-210, Brazil) calibrated at 39°C. Immediately the vials were placed on ice to inhibit fermentation. Incubation media samples (1.5 mL) were taken after the 24 h of incubation and were placed in 0.3 mL of 20% metaphosphoric acid (w/v). Samples were kept at −20°C and then analyzed acetic, propionic, iso-butyric butyric acid, iso-valeric, and valeric acid using a high-performance liquid chromatography (HPLC; Waters, Model alliance separation module e2695 with a photodiode array detector; wavelength 210 nm; Waters Technologies of Brazil Ltd, Barueri, SP, Brazil) with a C18 ODS 80A (150 × 4.6 × 5 μm) column.
For the analytical quantification of fatty acids, the oils were previously lyophilized, with samples containing between 10 and 50 mg of total fatty acids and subjected to direct transesterification and extraction by acid catalysis (16). For the analysis a gas-phase chromatograph (CG-ECD Agilent Technologies 6890N-SF6: ChemStation interface software with capillary column; HP-FFAP, 25 m × 0.2 mm × 0.33 μm). The stationary phase of acid was modified with nitroterephthalic polyethylene glycol using H2 as a carrier gas at 1.0 mL/min.
The data were analyzed using the mixed model procedure of SAS (SAS Inc., 2018, SAS OnlineDoc 9.1.4 Cary, NC, USA). The univariate procedure of SAS was used to test for normal distribution of data. The statistical design was completely randomized with 3 vials used per treatment, with 3 incubations runs. Fixed effects were oil, oil concentration, and the interaction between these terms. Random effects were incubation run and treatment within incubation run. Each incubation run (n = 3) was considered as the experimental unit. Treatment means for each level were compared using the least squares mean linear hypothesis test. Linear and quadratic effects were evaluated by using planned orthogonal polynomial coefficients for each parameter when Type 3 tests for fixed effects were ≤ 0.05. Significance was declared at P ≤ 0.05 and tendencies 0.05 < P ≤ 0.10.
Due to its CH4 mitigation potential, in vitro, pequi oil was chosen to be fed in the metabolism trial. Four castrated Dorper wethers (63.4 ± 1.46 kg live weight) were used for the metabolism trial and for measurement of CH4 production using a respiration chamber designed to accommodate small ruminants. Animals were housed in metabolic crates throughout the experimental period. The experiment was conducted as a randomized 2 × 2 Latin square (2 × 2 periods, 2 animals per treatment). Animal were fed a total mixed ration (TMR) twice daily at 0730 h and 1530 h, ad libitum (5% orts), and allowed constant access to clean water (Table 2). The diets were formulated using the Small Ruminant Nutrition System (Version 1.9.6290.40564) to meet the nutritional requirements of wethers for maintenance. The diets were formulated to be iso-nitrogenous and iso-fibrous. Two diets were evaluated: control (without oil) and a diet with daily inclusion of pequi oil (Mundo dos Óleos) at 70 g oil per animal (75 mL oil/animal). The experiment periods consisted of a 14-d adaptation period, with 5 d of collection for digestibility parameters and 2 d of gas measurements using respiration chambers.
For determination of apparent digestibility of nutrients, wethers were housed in metabolic crates (0.6 m width × 1.82 m length) during the study. The cage had a tray separating feces and urine, with total feces being collected and measured and collected each day for 5 days. Samples of feed, orts and feces were collected daily, pooled, and stored in plastic bags and kept at −20°C. Samples were pooled for each animal in each period, obtaining equal representative samples. For analysis, samples were dried at 55°C to constant weight and ground (1-mm sieve) using a Wiley mill.
During chamber measurements, sheep were housed in metabolism crate inside the respiration chamber for 2 periods of 22 h. All animals were previously adapted to stay in the chamber for the data collection phase and weighed before and after measurements. The open circuit respiration chamber (Intergado® Ltda., Contagem, MG, Brazil) had volume of 6.39 m3 (2.48 × 1.48 × 1.74 m) and made from aluminum and transparent polyethylene terephthalate glycol (PETG) walls to enhance the visual contact between the animals inside and outside the chamber. Air inside the chamber was maintained at 55% RH (relative humidity) and 22°C by an air conditioning unit (LG TSNH122H4W0, Manaus, Brazil) and a fan (Ventisol VM20-01, China) was used to circulate air within the chamber.
Inside the chamber, the fresh air inlet has a valve and a T connection fitted with 2 horizontal PVC tubes (50 mm diameter × 134 mm) punctured at each 10 cm with 1 cm wholes to avoid laminar flow, immediately above the feed bin and next to recirculating air entrance. The chamber was fitted with an air outlet which has a filter box (CSL-849-100HC, Solberg Manufacturing Inc., Itasca, IL), in the rear section of the ceiling, where the air was continuously drawn out through a 51-mm-diameter flexible polyurethane connected directly to a mass flowmeter (Flow Kit model FK-500, Sable International Systems, Las Vegas, NV). The flow rate used was 1.4 L/min for each kg of body weight (BW). The gas analysis and data acquisition system (Sable Systems International), as well the calibration procedures, were as previously described by Machado et al. (16), to calculate the CH4 production. The recovery for CH4 from the respiration system was 112.5 ± 5.23%.
Daily heat production (HP, Kcal/d) was calculated according to Brouwer (17): HP (Kcal/d) = (3,866 × O2) + (1,200 × CO2) – (0,518 × CH4).
Rumen samples (~70 mL) were collected on d 19 of each period via the esophageal tubing. Rumen contents were squeezed through 4 layers of cheese cloth and pH was taken immediately (Model HI 8417, Hanna Instruments, Singapore). A 50 mL subsample was taken for DNA extraction and frozen at −20°C.
DNA extraction was performed using MOBIO PowerLyzer™ Power Soil (Geneworks, Australia). After extraction, DNA concentration and purity were checked using a ND-2000 spectrophotometer (Nanodrop Technologies). DNA samples were diluted to 10 ng/μL for standardization of concentration between samples. The quantification of the number of total bacterial genes, archaea, Clostridium sp., Fibrobacter succinogenes, anaerobic fungi, Ruminococcus flavefaciens, Ruminococcus albus, and Selenomonas ruminantium were estimated with specific primers (16S rRNA) by amplification of the ribosomal gene sequences and curves were generated for their quantification (Table 3). The efficiency of the amplification of each primer set between 84 and 100% and linear standard curve (R2) above 0.996 were accepted for subsequent calculation of gene copy number.
The StepOnePlus™ (Applied Biosystems) thermocycler was used to quantify detection by real-time fluorescent PCR (Qpcr) with SYBR Green I as fluorescent dye. The reaction in a final volume of 10 μL containing 5 μL of the SYR Green ROX qPCR Master Mix (Thermo Scientific), 5 pmol of each primer (forward and reverse), 1 μL of DNA, and sterilized ultrapure water was applied. For the populations of F. succinogenes, S. ruminantium, R. flavefaciens, R. albus, and Clostridium sp., the relative quantification of the number of copies of the 16S rRNA gene from the ruminal microbiota groups was performed in relation to the number of total bacteria. For the populations of total bacteria, protozoa, fungi, and archaea the calculation was performed by absolute quantification using the standard curve method and expressed as the number of copies of amplicon from 16S rRNA that have been amplified by primers specific to each microorganism per 10 ng of DNA in 2 ml of ruminal fluid.
The following AOAC (22) methods, were applied to quantify dry matter (DM; Method 967.03), ash (Method 942), ether extract (EE) content by extraction with petroleum ether using a Soxtherm Fat Extractor (Gerhardt Instruments, Königswinter, Germany—Method 920.39). Neutral detergent fiber (NDF) concentration was quantified using of sodium sulfite (Na2SO3) (23) adapted for Ankom 200/220 Fiber Analyzer (Ankom Technol. Corp., Fairport, NY, EU) and not corrected for ash or protein. For this analysis, a thermostable alpha-amylase (Termamyl 2x) was added. The Kjeldahl method (Vapodest 20S, Gerhardt Instruments) with crude protein (CP) content calculated as N × 6.25 was applied for nitrogen (N) concentration determination. Non-fibrous carbohydrates (NFC, % of DM) was calculated using the equation NFC = 100–[CP + NDF + EE + Ash].
The data were analyzed as a 2 × 2 Latin square (2 treatments × 2 periods, 2 animals per treatment), using the SAS mixed model (SAS Inc., 2018, SAS OnlineDoc 9.1.4 Cary, NC, USA). The means were compared using the LSMEANS/DIFF, considering period and treatment as fixed effect and animal located within each treatment as random effect. The method for computing denominator degrees of freedom was Kenwardroger (e.g., DDFM = KENWARDROGER) Significance was declared at P ≤ 0.05 and tendencies 0.05 < P ≤ 0.10.
There was an oil × concentration effect on gas production after 24 h incubation (mL or mL/g DM incubated; P = 0.02) for all oils, and a linear effect of concentration (P < 0.01) was observed (Table 4). Carrot, passionfruit and pequi had the lowest total gas production (ml/g DM incubated) after 24 h of incubation when oil was included at 5% of incubation media. There was an oil × concentration effect observed for CH4 data (% or mL/g DM incubated). Carrot, macaúba, basil, passionfruit, and pequi oils linearly decreased (P < 0.01) the % CH4 in the gas sample. When calculated based on mL/g DM incubated, buriti also decreased CH4 production. Pequi oil resulted in the largest reduction of CH4 production.
Table 4. Effect of different oils on accumulative gas and methane production in an in vitro batch culture using a hay-based diet.
There was an oil × concentration effect (P ≤ 0.05) on pH, propionate, butyrate, BCVFA percentages of total VFA and the acetate to propionate (A:P) ratio (Table 5). The pH was linearly increased (P < 0.01) by increasing concentrations of oils included in the diet for all oils. The was a quadratic response (P < 0.01) of propionate percentage of total VFA to increasing concentration of oil in the diet for açaí, carrot, and pequi oil where propionate percentage of total VFA was increased at 1 and 2 % inclusion of oil. There was a linear and quadratic effect (P < 0.01) of buriti, carrot, passionfruit, basil, and pequi oils on acetate percentage of total VFA, where for most of oils butyrate percentage of total VFA was decreased at greater oil inclusion. All oils resulted in a quadratic response (P < 0.01) to BCVFA with increasing inclusion of oil. There was effect of açaí and pequi oil on the A:P, in which the ratio was decreased from the control at 1% oil inclusion and increased at 5% inclusion.
Table 5. Effect of different oils on pH and the volatile fatty acids (VFA) concentrations in an in vitro batch culture using a hay-based diet.
Pequi oil addition had no effect (P ≥ 0.46) on the apparent digestibility of nutrients (Table 6). Feed intake was not affected by pequi oil (P ≥ 0.27; Table 7). There was a tendency (P = 0.06) for an effect of pequi oil on CH4 production (g/d) in which CH4 was reduced by 17.5%. There was also a tendency for decreased CH4 yield based on g/kg DMI (P = 0.10) and g/kg NDF intake (P = 0.10). There was no effect of pequi oil on heat production (P = 0.12).
Table 6. Effect of pequi oil on apparent digestibility of nutrients in wethers fed a hay-based diet.
Table 7. Effect of pequi oil on dry matter intake (DMI), methane production and heat production in wethers fed a hay-based diet.
Pequi oil had no effect (P ≥ 0.52) on the concentration of total bacteria, archaea, protozoa or fungi (Table 8). Except for R. albus (0.79), the relative expression in terms of fold-changes of specific groups of the rumen microbiota after supplementation of pequi oil showed reduction ranging from −0.037 to −0.882 (Table 8).
Table 8. Effect of pequi oil on rumen microbial diversitya in wethers fed a hay-based diet fold-changes for specific groups after supplementation of pequi oil.
Oil supplementation can exert suppressive effects on digestibility and VFA production due to inhibitory effects on rumen microbiota. In this study, total gas production was decreased with increasing concentration of oils added to the substrate. It is well established that high concentrations of oil to the diet can inhibit fermentation (23, 25) therefore the maximum fat inclusion in the diet is recommended at no greater than 6% (26). However, in this study, all vegetable oils decreased total gas production when included at only 5% v/v. The reduction in gas production can explain the increase in pH with increasing concentration of oil supplementation, as high concentrations of oils increase pH, due to inhibition of fermentation (27).
All oils decreased CH4 production (%), except for açai and buriti, on a linear scale. Pequi oil had the greatest suppressive effect on CH4 (mL/g DM incubated), decreasing production after 24 h incubation by 86.1% and for this reason it was selected to use in the animal trial. Methane reduction potential of fats has been associated with the concentrations of MUFA and PUFA (28) with C12:0 and C18:3 having a high inhibitory effects for methanogenesis. Pequi oil may have been the most effective in decreasing CH4 due to its high palmitic (C16:0) and oleic acid (C18:1) content, probably acting in cellulolytic bacteria and archaea, respectively (29).
However, buriti also has a high concentration of these fatty acid and only suppressed CH4 production (mL/g DM incubated) when included at 5%. Additionally, buriti also has a high concentration of the PUFA, alpha-linolenic acid [C18:3 (n-3)] and linoleic acid [C18:2 (n-6)] compared to the other examined oils, however, the same potential for CH4 reduction was not exhibited. The passionfruit and carrot oil were also notable in decreasing CH4 (%) inducing 55.0%, and 69.4% reductions. Of these CH4 suppressing oils, only carrot and pequi oil changed the VFA profile. Both carrot and pequi oil increased the percentage of propionate, however only pequi oil supplementation altered the acetate to propionate ratio.
Whilst gas production was reduced in vitro, dry matter intake and other digestibility parameters were not influenced in the metabolism component of the study. However, pequi oil did have a tendency to decrease CH4 production by 17.5% when fed at 70 g per animal per day.
In contrast to this, Duarte et al. (30, 31) demonstrated that pequi oil included at 1.5 mL/d, increasing the dietary fat content to 6.0%, had no effect on in vitro CH4 production using the rumen simulation technique (RUSITEC). However, authors state that this may have been due to the small concentration of pequi oil tested in relation to fermentation liquid. Polyphenolic compounds (e.g., tannins) originating from tropical leaves or shrubs negatively impact methanogenic production by direct reduction of archaea population (32). However, during extraction of the oil from pequi pulp polyphenolic compounds were also identified (33). Therefore, the lower methane emission observed in lambs fed pequi oil in this work could be a result of the associative effects of certain fatty acids and polyphenolic compounds present in the oil that could contribute to the change in microbial populations and consequently decrease enteric CH4 production.
Lipids can also act to suppress the protozoal populations. However, in the in vivo study, protozoa communities were not affected by pequi oil supplementation. Comparatively, in the in vitro study butyrate production was decreased with increasing supplementation with pequi oil. Butyrate production is associated with certain microbial populations, specifically protozoa (34, 35). A decrease in protozoa numbers are associated with a decrease in CH4 production as the main fermentation end products of protozoal activity are acetate and butyrate, two VFA which provide H2 for methanogenesis (36).
The lack of change of microbial diversity is supported by Duarte et al. (31) who found that the archaeal and bacterial microbiota structure was not affected by pequi oil supplementation using the rumen simulation technique. However they did report that pequi oil increased the relative abundance of Anaerovibrio, a genus that has reported to be involved in the metabolism of lipids in the rumen, enhancing propionate synthesis (37, 38). In our study, when we compared the changes in microbial communities due to changes in the diet, i.e., addition of pequi, within the same animal (n = 2), we observed that except for Ruminococcus albus, all other studied cellulolytic bacteria were reduced, as observed with the fungi, archaea, and protozoa populations (39, 40).
From the in vitro study it can be concluded that all the vegetable oils decreased gas production after 24 h incubation, however only carrot, macaúba, basil, passionfruit, and pequi oil decreased CH4 (% and mL/g DM incubated). Pequi oil had the greatest CH4 reducing potential and also decreased the acetate to propionate ratio.
Supplementing pequi oil into a hay-based diet of wethers had no effect on the apparent digestibility of nutrients, dry matter intake or microbial diversity. Pequi oil supplementation did however, numerically decrease CH4 production by 17.5%. Therefore, pequi oil may be daily fed at 70 g/animal to reduce enteric CH4 production without any negative effects on feed digestibility.
AC and LP study design. DF and RR conducting in vitro study. DF, RR, TT, and FM conducting in vivo study. DF, AA, LP, RM, and RR lab analysis. AA DNA extraction and PCR analysis. AC, ST, and AA statistical analysis. ST, DF, AC, RM, and LP writing the manuscript. LP, FM, MC, and RM leader of the grants. AC, ST, LP, RM, TT, AA, FM, and MC editing the final version of the manuscript. All authors read and approved the final manuscript.
The results of this article is part of PECUS RumenGases Brazil—Conceptual advance in diagnosis and estrategies of mitigating of enteric methane emissions by ruminants in Brazil research project. The authors are thankful to Conselho Nacional de Desenvolvimento Científico e Tecnológico (CNPq), Fundação de Amparo à Pesquisa do Estado de Minas Gerais (FAPEMIG), Coordenação de Aperfeiçoamento de Pessoal de Nível Superior (CAPES-PVE), Fundação de Amparo à Pesquisa do Estado da Bahia (FAPESB), Universidade Federal de São João del-Rei (UFSJ), Centro de Energia Nuclear na Agricultura (CENA-USP) and Empresa Brasileira de Pesquisa Agropecuária (EMPRAPA) for supporting this research. Also the authors acknowledge both Ph.D candidates Vanessa Amorim Teixeira and João Paulo Sacramento for their help with the animals during the in vivo study.
The authors declare that the research was conducted in the absence of any commercial or financial relationships that could be construed as a potential conflict of interest.
1. MAPA. Brasil 2013/2014 a 2023/2024 – Projeções de Longo Prazo”, feito pelo Ministério da Agricultura, Pecuária e Abastecimento (Mapa). in Projeções do Agronegócio. Available online at: http://www.agricultura.gov.br/assuntos/politica-agricola/todas-publicacoes-de-politica-agricola/projecoes-do-agronegocio/projecoes-do-agronegocio-brasil-2013-2014-a-2023-2024.pdf/view
2. McManus C, Barcellos JOJ, Formenton BK, Hermuche PM, De Carvalho OA, Guimarães R, et al. Dynamics of cattle production in Brazil. PLoS ONE (2016) 11:e0147138. doi: 10.1371/journal.pone.0147138
3. Beauchemin KA, McGinn SM, Petit HV. Methane abatement strategies for cattle: lipid supplementation of diets. Can J Anim Sci. (2007) 87:431–40. doi: 10.4141/CJAS07011
4. Eugène M, Massé D, Chiquette J, Benchaar C. Meta-analysis on the effects of lipid supplementation on methane production in lactating dairy cows. Can J Anim Sci. (2008) 88:331–7. doi: 10.4141/CJAS07112
5. Beauchemin KA, McGinn SM, Benchaar C, Holtshausen L. Crushed sunflower, flax, or canola seeds in lactating dairy cow diets: effects on methane production, rumen fermentation, and milk production. J Dairy Sci. (2009) 92:2118–27. doi: 10.3168/jds.2008-1903
6. Knapp JR, Laur GL, Vadas PA, Weiss WP, Tricarico JM. Invited review: enteric methane in dairy cattle production: quantifying the opportunities and impact of reducing emissions. J Dairy Sci. (2014) 97:3231–61. doi: 10.3168/jds.2013-7234
7. Hartanto R, Cai L, Yu J, Zhang N, Sun L, Qi D. Effects of supplementation with monensin and vegetable oils on in vitro enteric methane production and rumen fermentability of goats. Pakistan J Agric Sci. (2017) 54:693–8. doi: 10.21162/PAKJAS/17.4347
8. Mao HL, Wang JK, Zhou YY, Liu JX. Effects of addition of tea saponins and soybean oil on methane production, fermentation and microbial population in the rumen of growing lambs. Livest Sci. (2010) 129:56–62. doi: 10.1016/j.livsci.2009.12.011
9. Wanapat M, Mapato C, Pilajun R, Toburan W. Effects of vegetable oil supplementation on feed intake, rumen fermentation, growth performance, and carcass characteristic of growing swamp buffaloes. Livest Sci. (2011) 135:32–7. doi: 10.1016/j.livsci.2010.06.006
10. Grainger C, Beauchemin KA. Can enteric methane emissions from ruminants be lowered without lowering their production? Anim Feed Sci Technol. (2011) 166–7:308–20. doi: 10.1016/j.anifeedsci.2011.04.021
11. Johnson KA, Johnson DE. Methane emissions from cattle. J Anim Sci. (1995) 73:2483–92. doi: 10.2527/1995.7382483x
12. Guedes AMM, Antoniassi R, de Faria-Machado AF. Pequi: a Brazilian fruit with potential uses for the fat industry. OCL (2017) 24: D507. doi: 10.1051/ocl/2017040
13. Costa JGM, Brito SA, Nascimento EMM, Botelho MA, Rodrigues FFG, Coutinho HDM. Antibacterial properties of pequi pulp oil (Caryocar coriaceum - Wittm.). Int J Food Prop. (2011) 14:411–6. doi: 10.1080/10942910903207744
14. Ding S, Meale SJ, Alazzeh AY, He ML, Ribeiro GO, Jin L, et al. Effect of Propionibacterium freudenreichii in diets containing rapeseed or flaxseed oil on in vitro ruminal fermentation, methane production and fatty acid biohydrogenation. Anim Prod Sci. (2017) 57:2051–9. doi: 10.1071/AN15878
15. Fedorah PM, Hrudey SE. A simple apparatus for measuring gas production by methanogenic cultures in serum bottles. Environ Technol Lett. (1983) 4:425–32. doi: 10.1080/09593338309384228
16. Sukhija PS, Palmquist DL. Rapid method for determination of total fatty acid content and composition of feedstuffs and feces. J Agric Food Chem. (1988) 36:1202–6. doi: 10.1021/jf00084a019
17. Brouwer E. Report of sub-committee on constants and factors. In: Symposium of Energy Metabolism held at European Association for Animal Production. London: EAAP Academic (1965). p. 441–3.
18. Denman SE, McSweeney CS. Development of a real-time PCR assay for monitoring anaerobic fungal and cellulolytic bacterial populations within the rumen. FEMS Microbiol Ecol. (2006) 58:572–82. doi: 10.1111/j.1574-6941.2006.00190.x
19. Denman SE, Tomkins NW, McSweeney CS. Quantitation and diversity analysis of ruminal methanogenic populations in response to the antimethanogenic compound bromochloromethane. FEMS Microbiol Ecol. (2007) 62: 313–22. doi: 10.1111/j.1574-6941.2007.00394.x
20. Asanuma N, Iwamoto M, Kawato M, Hino T. Numbers of nitrate-reducing bacteria in the rumen as estimated by competitive polymerase chain reaction. Anim Sci J. (2002) 73:199–205. doi: 10.1046/j.1344-3941.2002.00028.x
21. Yu Y, Lee C, Kim J, Hwang S. Group-specific primer and probe sets to detect methanogenic communities using quantitative real-time polymerase chain reaction. Biotechnol Bioeng. (2005) 89:670–9. doi: 10.1002/bit.20347
22. AOAC. Official Methods of Analysis of AOAC International. 16th edn. Washington, DC: Association of Official Analytical Chemists (1995).
23. Van Soest PJ, Robertson JB, Lewis BA. Methods for dietary fiber, neutral detergent fiber, and nonstarch polysaccharides in relation to animal nutrition. J Dairy Sci. (1991) 74:3583–97. doi: 10.3168/jds.S0022-0302(91)78551-2
24. Vargas JE, Andrés S, Snelling TJ, López-Ferreras L, Yáñez-Ruíz DR, García-Estrada C, et al. Effect of sunflower and marine oils on ruminal microbiota, in vitro fermentation and digesta fatty acid profile. Front Microbiol. (2017) 8:1124. doi: 10.3389/fmicb.2017.01124
25. Fraser GR, Chaves AV, Wang Y, McAllister TA, Beauchemin KA, Benchaar C. Assessment of the effects of cinnamon leaf oil on rumen microbial fermentation using two continuous culture systems. J Dairy Sci. (2007) 90:2315–28. doi: 10.3168/jds.2006-688
26. Van Soest PJ. Nutritional Ecology of the Ruminants. 2nd Edn. Ithaca: Cornell University Press (1994).
27. Castillejos L, Calsamiglia S, Ferret A. Effect of essential oil active compounds on rumen microbial fermentation and nutrient flow in vitro systems. J Dairy Sci. (2006) 89:2649–58. doi: 10.3168/jds.S0022-0302(06)72341-4
28. Patra AK, Yu Z. Effects of coconut and fish oils on ruminal methanogenesis, fermentation, and abundance and diversity of microbial populations in vitro. J Dairy Sci. (2013) 96:1782–92. doi: 10.3168/jds.2012-6159
29. Nagaraja TG, Newbold CJ, Van Nevel CJ, Demeyer DI. Manipulation of Rumen Fermentation. Dordrecht: Springer (1997).
30. Duarte AC, Durmic Z, Vercoe PE, Chaves AV. Dose-response effects of dietary pequi oil on fermentation characteristics and microbial population using a rumen simulation technique (Rusitec). Anaerobe (2017) 48:59–65. doi: 10.1016/j.anaerobe.2017.06.013
31. Duarte AC, Holman DB, Alexander TW, Kiri K, Breves G, Chaves AV. Incubation temperature, but not pequi oil supplementation, affects methane production, and the ruminal microbiota in a rumen simulation technique (Rusitec) system. Front Microbiol. (2017) 8:1076. doi: 10.3389/fmicb.2017.01076
32. Malik PK, Kolte AP, Baruah L, Saravanan M, Bakshi B, Bhatta R. Enteric methane mitigation in sheep through leaves of selected tanniniferous tropical tree species. Livest Sci. (2017) 200:29–34. doi: 10.1016/j.livsci.2017.04.001
33. de Santana Magalhães F, Cardoso VL, Reis MHM. Sequential process with bioadsorbents and microfiltration for clarification of pequi (Caryocar brasiliense Camb.) fruit extract. Food Bioprod Process (2018) 108:105–116. doi: 10.1016/j.fbp.2018.02.003
34. Demeyer DI, Van Nevel CJ. Effect of defaunation on the metabolism of rumen micro-organisms. Br J Nutr. (1979) 42:515–24. doi: 10.1079/BJN19790143
35. Carberry CA, Kenny DA, Han S, McCabe MS, Waters SM. Effect of phenotypic residual feed intake and dietary forage content on the rumen microbial community of beef cattle. Appl Environ Microbiol. (2012) 78:4949–58. doi: 10.1128/AEM.07759-11
36. McAllister TA, Newbold CJ. Redirecting rumen fermentation to reduce methanogenesis. Aust J Exp Agric. (2008) 48:7–13. doi: 10.1071/EA07218
37. Jouany JP, Lassalas B, Doreau M, Glasser F. Dynamic features of the rumen metabolism of linoleic acid, linolenic acid and linseed oil measured in vitro. Lipids (2007) 42:351–60. doi: 10.1007/s11745-007-3032-x
38. Jenkins TC, Wallace RJ, Moate PJ, Mosley EE. Board-invited review: recent advances in biohydrogenation of unsaturated fatty acids within the rumen microbial ecosystem. J Anim Sci. (2008) 86:397–412. doi: 10.2527/jas.2007-0588
39. Yang SL, Bu DP, Wang JQ, Hu ZY, Li D, Wei HY, et al. Soybean oil and linseed oil supplementation affect profiles of ruminal microorganisms in dairy cows. Animal (2009) 3:1562–9. doi: 10.1017/S1751731109990462
Keywords: exotic oils, sheep, in vitro, methane, cerrado biome
Citation: Freitas DS, Terry SA, Ribeiro RS, Pereira LGR, Tomich TR, Machado FS, Campos MM, Corrêa PS, Abdalla AL, Maurício RM and Chaves AV (2018) Unconventional Vegetable Oils for a Reduction of Methanogenesis and Modulation of Ruminal Fermentation. Front. Vet. Sci. 5:201. doi: 10.3389/fvets.2018.00201
Received: 25 May 2018; Accepted: 02 August 2018;
Published: 05 September 2018.
Edited by:
Amlan Kumar Patra, West Bengal University of Animal and Fishery Sciences, IndiaReviewed by:
Seongwon Seo, Chungnam National University, South KoreaCopyright © 2018 Freitas, Terry, Ribeiro, Pereira, Tomich, Machado, Campos, Corrêa, Abdalla, Maurício and Chaves. This is an open-access article distributed under the terms of the Creative Commons Attribution License (CC BY). The use, distribution or reproduction in other forums is permitted, provided the original author(s) and the copyright owner(s) are credited and that the original publication in this journal is cited, in accordance with accepted academic practice. No use, distribution or reproduction is permitted which does not comply with these terms.
*Correspondence: Alexandre V. Chaves, YWxleC5jaGF2ZXNAc3lkbmV5LmVkdS5hdQ==
Disclaimer: All claims expressed in this article are solely those of the authors and do not necessarily represent those of their affiliated organizations, or those of the publisher, the editors and the reviewers. Any product that may be evaluated in this article or claim that may be made by its manufacturer is not guaranteed or endorsed by the publisher.
Research integrity at Frontiers
Learn more about the work of our research integrity team to safeguard the quality of each article we publish.