- 1Department of Anesthesiology, The Ohio State University Wexner Medical Center, Columbus, OH, United States
- 2Department of Anesthesiology, School of Medicine, Stony Brook University, Health Sciences Center, Stony Brook, NY, United States
Fluid management has been widely recognized as an important component of the perioperative care in patients undergoing major procedures including spine surgeries. Patient- and surgery-related factors such as age, length of the surgery, massive intraoperative blood loss, and prone positioning, may impact the intraoperative administration of fluids. In addition, the type of fluid administered may also affect post-operative outcomes. Published literature describing intraoperative fluid management in patients undergoing major spine surgeries is limited and remains controversial. Therefore, we reviewed current literature on intraoperative fluid management and its association with post-operative complications in spine surgery.
Introduction
During the last decade, the role of fluid therapy as an essential component of perioperative management has been given more emphasis in large part due to the Enhanced Recovery After Surgery pathway (1, 2). The impact of intraoperative fluid therapy on hemodynamics during major surgery has been widely studied (3, 4). However, established guidelines for intraoperative fluid therapies are limited, particularly in specific surgical populations such as patients undergoing major spine surgery.
Fluid management during major spine surgery is determined by procedure- (e.g., length of surgery, risk of increased intraoperative blood loss, and prone positioning) and patient-related factors (e.g., American Society of Anesthesiologists –ASA physical status, comorbidities, and sequelae from long-term spinal deformities) (5–8). Over the past decade, the number of elective spine surgeries has continuously increased, especially in surgical populations at high risk of perioperative complications such as the elderly. A reduced physiological reserve is one of the factors that has a significant impact on the response to intraoperative fluid therapy in this patient setting (9). Likewise, the volume and type of fluid administered during the surgery may be associated with the onset of post-operative complications (10–15).
The spectrum of complex or major spine surgery comprises correction of deformities (e.g., scoliosis), vertebral infection (e.g., abscess), and spinal stabilization procedures following trauma, neoplastic disease or degenerative changes. Major spinal surgery may be performed at any spinal segment, from cervical to lumbosacral, and involves multiple vertebral levels.
Open and decompressive laminotomies and discectomies, anterior cervical discectomy and fusion, posterior cervical laminectomy and fusion, posterior cervical laminoplasty, multilevel anterior vertebrectomies, and fusion with instrumentation, decompressive laminectomies with posterior instrumented fusions, combined anterior/posterior approaches, and adult spinal deformity surgeries are some of the major spine procedures discussed in this review.
The use of spinal instrumentation devices may result in prolonged surgical times and significant intraoperative blood loss (16). Decompressive and corrective procedures involve multiple osteotomies releasing affected structures at localized spine levels. Spinal stabilization procedures entail instrumentation above and below the unstable vertebral levels in a combined posterior and anterior approach. Moreover, acute perioperative blood loss is a widely known complication of multilevel spine surgeries and is commonly exacerbated by the number of levels fused, osteotomies performed, and in pedicle subtraction osteotomies or vertebral column resections (17, 18). Blood transfusion in the setting of acute blood loss has been associated with increased risk of coagulation impairment, bloodborne infectious disease transmission, post-operative hematoma formation, shock, and pulmonary edema (16, 19).
Prone positioning is widely used in patients undergoing major spine surgeries and has been associated with significant hemodynamic changes such as decreased cardiac output, stroke volume (SV), and heart rate (20–22). Intraoperative hemodynamic stability and adequate tissue perfusion remain as some of the main challenges anesthesiologists face. Several trials suggest that goal-directed fluid therapy (GDFT) can help guide fluid administration to optimize intravascular volume status of patients undergoing major surgery. This hemodynamic strategy utilizes titration of fluids, vasopressors, and inotropes to achieve target values of hemodynamic variables tailored to the patient's cardiovascular physiology to improve post-operative outcomes (20, 23, 24).
Objectives
In this narrative review, we intend to discuss published literature describing outcomes and correlation between intraoperative fluid management and post-operative complications (e.g., perioperative visual loss –POVL, delayed post-operative extubation, post-operative urinary retention –POUR, post-operative pulmonary complications, and prolonged intensive care unit –ICU and hospital length of stay –LOS) in patients undergoing spine surgeries. Pathophysiological changes associated with surgical positioning, the use of GDFT, and volume replacement during massive blood loss in spine surgery will also be addressed.
Physiologic Changes During Prone Positioning
With the use of tools such as pulmonary arterial catheter and transesophageal echocardiography (TEE), significant reductions in cardiac output (CO) and SV have been reported in patients undergoing lumbar spine surgery in prone position (20, 21, 25). In an early study, Yokoyama et al. (21) analyzed several hemodynamic variables in anesthetized patients undergoing lumbar spine surgery in the prone position. Changes in systemic arterial pressure, right arterial pressure, pulmonary arterial pressure, CO, heart rate (HR), mean arterial pressure, pulmonary artery mean pressure, cardiac index (CI), stroke volume index (SVI), systemic vascular resistance index (SVRI), and pulmonary vascular resistance index (PVRI) were assessed. Authors reported a significant reduction in CI from 3.1 ± 0.5 to 2.5 ± 0.3 (p < 0.01) during prone positioning but no changes were observed in other hemodynamic variables (21). In healthy patients, these hemodynamic variations per se may have a minimum or null cardiopulmonary impact. However, some mechanical changes such as abdominal compression with subsequent increase in intrathoracic pressures also occur during prone positioning. Therefore, this may have a substantial impact on important anatomic structures (e.g., inferior vena cava), resulting in significant circulatory changes (i.e., decreased venous return, increased impedance of blood flow to the left ventricle and obstruction of the IVC) (22, 26). In addition, a greater intra-abdominal pressure generated during prone position may increase surgical bleeding by engorging collateral veins, compromising the spinal cord perfusion which can lead to lead to spinal cord ischemia (27–29). Likewise, changes in peak airway pressure and respiratory compliance can also contribute to decreasing venous return and CO (30) (Figure 1).
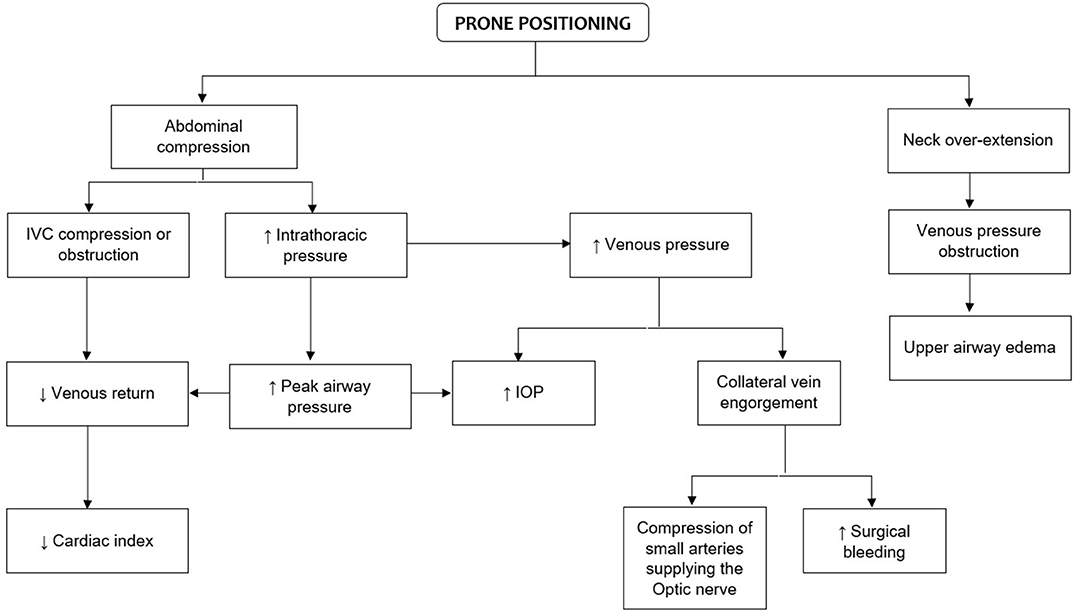
Figure 1. Physiologic changes of prone positioning that are relevant to spine surgery. IVC, inferior vena cava; IOP, intraocular pressure.
Surgical Tables
The main goals of surgical positioning in patients undergoing spine procedures are to decrease venous pressures at the surgical levels and to reduce the burden on peripheral nerves and face while establishing an optimal surgical approach (31). In a randomized clinical trial, Dharmavaram et al. (20) sought to compare the hemodynamic effects between five different prone positioning variants (a bolster system, Siemens'—George and Tom Upholstery-, Andrews', Wilson's, and Jackson's frames) in patients undergoing lumbar surgery. Left ventricular areas, CO, CI, SV, and contractility score were some of the TEE variables measured in these patients. A significant decrease in CO was reported in all tested variants of which the Wilson frame position showed the greatest reduction (19%). Similarly, considerable changes in CI and SV were observed when the Andrews, Wilson, and Siemens systems were used (20). Nevertheless, the incidence of procedure-inherent complications such as acute kidney injury may be comparable regardless of the frame used (32). NICOM® and FloTrac™/Vigileo™ systems are some of the non-invasive and minimally invasive (respectively), alternative techniques used to monitor hemodynamic changes and fluid response in patients undergoing spine surgeries in different prone variants (33, 34).
Goal-Directed Fluid Therapy
The combined physiologic effects of prone positioning and the risk of substantial blood loss pose patients undergoing multilevel spine surgery at high risk for intraoperative hemodynamic instability. GDFT is described as an approach to guide perioperative fluid administration in order to avoid fluid overload, organ hypoperfusion, hypoxia, and hypovolemia (35, 36). This volume replacement strategy has been associated with more positive post-operative outcomes such as enhanced post-operative recovery and reduced length of hospital stay, occurrence of acute renal injury and infections (37–44). On the other hand, fluid overload has been linked to delayed post-operative extubation, perioperative visual loss, and increased length of hospital stay in patients undergoing spine surgery (45–52).
Published literature describing the use of GDFT in spine surgeries is limited (6, 13, 53, 54). Dynamic indices such as pulse pressure variation, stroke volume variation, corrected flow time, and plethysmography index variability have been commonly used to predict fluid responsiveness among GDFT trials (Table 1) (33, 34, 55, 56). Significant reduction in blood loss and blood transfusion, fewer post-operative respiratory complications, faster return of bowel function after surgery, shorter length of stay, reduced number, and duration of hypotensive episodes, and decreased incidence of nausea, vomiting and post-operative delirium have been reported with the use of GDFT in patients undergoing spine surgeries (6, 13, 53). The last finding is attributed to a reduction of peripheral inflammatory markers IL-6 and S100β and becomes of particular interest as fluid balance has been described as a modifiable risk factor of post-operative delirium in this patient setting (14). Additionally, GDFT plays an important role in optimizing intraocular pressure during prone positioning when compared to other fluid therapies or medications (e.g., α2 agonist Brimonidine) (54).
Fluid Replacement During Massive Blood Loss
Despite an enormous amount of evidence supporting the substantial risk of greater perioperative blood loss during major spine and orthopedic surgeries, there is no universal consensus as to the optimal management of intraoperative fluid replacement for these procedures (18, 57, 58). In spine surgery, stripping muscles off bone and osteotomies are associated with an increased bleeding of exposed surfaces (17). Multi-level spine surgeries involve greater trauma and exposure, which means bleeding can be more insidious and profuse. Research efforts addressing massive perioperative blood loss in spine surgery are ongoing including preventive measures to reduce blood loss (e.g., tranexamic acid) and point-of-care devices (e.g., rotational thromboelastometry) that can be used to assess transient coagulopathies and therefore, to guide hemostatic therapy (59–62).
Crystalloids are the first-line resuscitative fluid in surgical patients (63–65). However, concerns about its overuse have been raised and studied (66–68). The high chloride content of 0.9% saline has led to hyperchloremic acidosis and increased risk of acute kidney injury (69–71). In addition, 0.9% saline use has been associated with more post-operative infections and blood transfusions compared to balanced salt solution in patients undergoing open abdominal surgery (72). This finding is consistent with in vitro and pre-clinical studies that show an association between hyperchloremic acidosis and changes in the immune response due to modifications in cytokine expression (73). Moreover, saline-induced acute kidney injury may be associated with neutrophil dysfunction and decreased bacterial clearance (74). In contrast, colloids are commonly preferred over crystalloids during massive bleeding based on their ability to persist longer within the intravascular space, enabling hemodynamic stability with a lower total volume of fluids (63). Nevertheless, colloids are costly and have been associated with hypersensitivity reactions, coagulopathy, and kidney dysfunction (75). Emerging evidence suggest that third-generation colloids in balanced salt solution are potentially safer with no significant effect on coagulation when compared to crystalloids (76, 77).
Intraoperative hypovolemia and vasodilation may result in hypotension and subsequent spinal cord ischemia. A decreased amplitude and increased latency in transcranial motor and somatosensory evoked potentials (TcMEPs and SSEPs, respectively) are some of the electrophysiological changes reported during spine ischemic episodes. In hypovolemic-hypotensive animal models, these electrophysiological variations return back to baseline values after blood volume was restored. However, the length of the hypotensive shock episodes remains as one of the main variables with a significant impact on these outcomes (78). Likewise, Lieberman et al. (79) also reported an association between hemorrhagic hypotension and vasodilatory hypotension with decreased amplitude and increased latency of TcMEPs in pig models. Nevertheless, reestablishment of the mean arterial pressure (MAP) during hemorrhagic hypotension (i.e., use of phenylephrine or preload optimization with colloids), did not restore TcMEP parameters as effectively as in the vasodilatory group (79). These findings suggest that augmentation of cardiac output and oxygen delivery (DO2) is more efficacious at restoring electrophysiological state after hemorrhagic hypotension than solely increasing MAP. A recent retrospective study conducted by Acharya et al. (80) including 61 patients who underwent spine deformity surgery with TcMEPs monitoring, identified hypotension as the most common cause of TcMEP's alterations. These findings were timely correlated with spinal cord ischemia as hypotension correction resulted in reversal of TcMEPs changes (80). While the authors followed a protocol to increase MAP > 100 mmHg, they did not specify how this was achieved.
Fluid Management and Postoperative Complications in Spine Surgery
Perioperative Visual Loss
Perioperative visual loss (POVL) in spine surgery is rare; however, it is one of the most devastating complications leading to greater functional disability and general medical burden (81). According to a nationwide population-based database, the incidence of POVL in spine fusion varied between 0.89 to 1.02 events per 10,000 surgeries during the period 1998–2012 (81). In another study that analyzed 167, 972 spinal deformity cases from 2009 to 2012, post-operative visual complication rate was 12.5 events per 100,000, with an average of 0.01% per year (82).
Ischemic optic neuropathy (ION), retinal arterial occlusion and cortical blindness are the most commonly reported causes of POVL (83), with patients undergoing spine surgeries involving thoracic or lower vertebrae fusions at higher risk (84).
A proposed pathophysiology for POVL in spine surgery suggests prone positioning may cause extravasation of fluids with subsequent interstitial fluid accumulation and elevated intraocular pressure. This elicits a local compartment syndrome within the optic nerve resulting in acute axon damage (85, 86). In addition, placing the patient in the prone position increases venous pressures resulting in venous engorgement. This in turn provokes a critical decrease in blood flow to the optic nerve by compressing the small supplying arteries, the posterior segment becoming especially vulnerable to ischemia due to poor collateral flow (49).
Several risk factors have been attributed to the development of POVL (83, 87) with volume and/or type of fluid administered being one of the foremost causes (48, 88, 89). In a case-control study of visual loss after cardiac surgery, a significant post-operative weight gain was commonly identified among patients, suggesting that large volumes of fluid may predispose a patient to visual complications (90). According to the ASA POVL Registry, mean intravenous crystalloid replacement was 9.7 ± 4.71 L among cases with 30% of them receiving colloids for fluid replacement therapy (84). Moreover, cell saver and packed red blood cells (PRBC) were used in approximately half of the cases (84).
In an attempt to reduce edema formation and to increase perfusion pressure of the optic nerve, several recommendations advocate the preferential use of colloids for intraoperative fluid replacement which traditionally have been taught to remain longer intravascularly due to their molecular size (49, 90–92). However, the general perception stating three times less colloid as crystalloid would be required to achieve similar hemodynamic effects in hypovolemic patients has become obsolete with this ratio being as low as 1:1.4-1.6 (93–95). Moreover, colloid administration may have little or no effect on IOP during prolonged spine surgeries in prone position. In a factorial randomized trial, Farag et al. (54) reported similar IOP in 60 patients undergoing spine surgery in the prone position receiving either crystalloid (lactated ringer) or colloid (5% albumin). Authors randomized patients into 4 groups: 2 of them receiving albumin and the other 2 receiving crystalloid, each combined with either an ophthalmic topical eye drop or topical Brimonidine, respectively. A significant increase in IOP was observed during prone positioning (mean of 12 ± 6 mmHg) in studied patients. This effect was significantly reduced with Brimonidine (P = 0.023) but neither the use of crystalloid nor colloid had any effect on IOP (P = 0.34) (54).
The correlation between the degree of blood loss, hypotension, and fluid therapy with ION remains controversial as these and other surgical-related risk factors have been linked to the development of POVL (96–98). Restricted intraoperative fluid therapies in the setting of large blood loss may result in anemia and hypotension. Anemia reduces oxygen carrying capacity and delivery to neural tissue whereas hypotension (i.e., hypovolemia or deliberate intraoperative hypotension) decreases arterial perfusion pressure, both potentially resulting to perioperative ION (97, 99). However, anemia and hypotension are common in many surgical procedures in which the incidence of POVL is basically null arguing further against their exclusive role in the pathogenesis of POVL (85). In a multivariate analysis, the POVL Study Group found that anemia or hypotension (defined as blood pressure > 40% below baseline for 30 min) alone were not independent risk factors of POVL, encouraging researchers to determine whether the effect of these factors remain significant when other variables (e.g., volume administration) are analyzed (49). Even though there is evidence supporting the administration of colloid over crystalloid to prevent POVL (10, 86, 100, 101), several safety and efficacy concerns about its use have been also reported (102, 103). In contrast, some authors support that either type of fluid can be used as long as adequate volume replacement is maintained and fluid overload is avoided (87, 104, 105).
Delayed Post-operative Extubation
Perioperative fluid overload may prolong the time it takes for airway to return to baseline and delay post-operative extubation in patients undergoing major spine surgeries, especially when multiple levels are involved (45, 46, 106–108). Prone positioning and large fluid shifts can also result in facial and upper airway edema potentially precluding safe extubation (45–47, 109). Delayed extubation may occur in up to 44% of adult patients undergoing multilevel spine surgeries and in 20–25% of those involving thoracic or lumbar levels (45–47, 110). Likewise, the overall incidence of airway adverse events including re-intubation, failed extubation in the ICU or delayed extubation (i.e., inability to extubate the patient at emergence from anesthesia) have been reported to be as high as 27% after posterior occipito-cervical spinal fusion (111).
The administration of high volumes of IV fluids, regardless of the type, is commonly associated with an increased risk of post-operative respiratory complications (51, 112). In a retrospective study, Siemionow et al. (51) analyzed data from 1,307 patients undergoing spine surgery in order to assess the impact of fluid therapy on length of stay and pulmonary complications. After a multivariable logistic regression, a significant correlation between total fluids administered (>4,165 mL) and longer hospital stay was identified (P < 0.0001). This correlation remained significant when either crystalloids or colloids were administered (51). Moreover, Hart et al. (12) reported a lower incidence of prolonged post-operative intubation or reintubation among patients who underwent cervical spine surgery in the prone position after the implementation of a restricted fluid therapy protocol.
Similarly, prolonged duration of anesthesia and surgery, increased levels of vertebrae fused, and higher intraoperative blood loss and transfusions have been also associated with delayed extubation in patients undergoing spine surgeries (45–47, 109, 111, 113). A recent propensity score-matched analysis indicated that patients who received higher volumes of non-cell saver blood product transfusions and did not have a proportionate decrease in the volume of crystalloids administered. These patients were identified to be at risk for delayed extubation even after controlling for patient demographics, comorbidities, and complexity of the spine surgery (106). Research has shown that since most of the transfused blood products remain intravascular, there should be a reciprocal reduction of crystalloid fluid administration. Therefore, in the setting of increased intraoperative blood loss, immediate post-operative extubation may be feasible when the proportion of crystalloids administered is reduced based on the total fluids infused.
Post-operative Urinary Retention
Postoperative urinary retention (POUR) is defined as an acute inability to void when the bladder is full after surgery, leading to patient's anxiety, discomfort, urinary tract infections, delayed hospital discharge and/or increased readmission rates (114–119). Most cases of POUR are associated with surgeries performed on the lumbar spine (15, 116, 119–121). Lumbar spine surgeries commonly involve the use of a Foley catheter which may cause urethral irritation and edema or reactive increase in tone of the bladder outlet, thereby contributing to POUR (120). In addition, post-operative pain in the lower lumbar area may trigger a reflex involving afferent and efferent fibers that ultimately inhibit detrusor muscle activity causing urinary retention (120, 122).
In the setting of posterior lumbar spine surgery, patient-related rather than surgery-related factors are likely to be the main contributors in the development of POUR (117, 119). Even though surgical manipulation of spinal nerves may be associated with the onset of POUR, no significant differences in POUR occurrence were reported between patients with and without overt post-operative neurologic deficit (119).
Despite some reports supporting a correlation between the volumes of IV fluid administered during surgery and the incidence of POUR in patients undergoing major surgeries (122–125), this association has not been observed in patients undergoing spine surgeries (15, 111). In a retrospective study including 397 patients who had undergone elective cervical, thoracic and lumbar surgery, Altschul et al. (116) reported an increased incidence of POUR in those patients with longer operative times. However, no correlation between POUR occurrence and intraoperative IV fluid administration was found (116). Similarly, Aiyer et al. (15) reported that a higher IV fluid volume administered was not an independent risk factor among patients who developed POUR after posterior lumbar spine surgery without intraoperative urinary catheterization. In summary, intraoperative bladder catheterization, previous spine surgery, operative level (L2−5), lumbar fusion, and duration of indwelling Foley catheterization are considered the main risk factors for POUR after major spine surgery (120).
Prolonged Length of Stay
In a retrospective study, Nahtomi-Shick et al. (52) identified total fluid administration as one of the intraoperative factors with a significant impact on length of ICU stay in patients who underwent spine surgery. Greater volumes of crystalloid fluids administered during surgery were associated with longer ICU length of stay. However, total fluid administration is closely related to the type of procedure which limits its specific predictive value for ICU length of stay (52). In contrast, functional presurgical and post-surgical variables may be stronger predictors of length of stay in patients undergoing spine surgery (126). These variables tend to be heterogeneous and interrelated, making it difficult to determine each of their individual impacts on prolonging length of hospital or ICU stay (126, 127). For instance, complex procedures or higher ASA physical status scores are commonly associated with increased length of surgery with subsequent increased fluid administration (52, 127, 128). Likewise, longer surgery time has been associated with increased blood loss and transfusion; both of which are significantly correlated with longer hospital stay (128).
Cerebrospinal Fluid Leak
Cerebrospinal fluid (CSF) leak is a well-known entity associated with a variety of neurosurgical procedures including spine surgeries. Whether planned or unplanned, CSF leak management may significantly impact patients' outcomes. Traditionally, specific surgical techniques such as the transoral approach for the anterior craniocervical junction pathologies, were linked to an increased risk of CSF leak and meningitis (129). However, the endoscopic endonasal approach has progressively replaced this and other highly invasive techniques for intra and extradural diseases, which resulted in enhanced recovery and a significant decreased risk of CSF leak (129).
Intentional or accidental durotomy triggers intracranial hypotension which, if uncorrected, may pose an increased risk of hemorrhage and ischemia. These life-threatening complications have been widely studied in patients undergoing intracranial procedures but may also be seen (in a lower proportion) after spine surgeries (130). In addition to surgical repair, optimizing intravenous fluid has been a traditional measure among practitioners (131). Nevertheless, specific guidelines on fluid replacement strategies and their impact on CSF leak resolution are limited.
Other Complications
Deep vein thrombosis (DVT) and pulmonary embolism (PE) are life-threatening post-operative complications reported after spine and other neurosurgical procedures associated with prolonged surgical times (132). In addition, fluid and electrolyte imbalance have been identified as an independent risk factor for DVT and PE in patients undergoing lumbar spine surgeries (133). However, the impact of perioperative fluid management in the onset or prevention of DVT and PE in spine surgeries remains unclear. In a retrospective analysis of 207 patients undergoing cervical spine surgery, Dube et al. (134) noticed an incidence of pulmonary complications close to 40%. An univariate analysis showed a relative risk of pulmonary complications of 2.3 in patients who received > 2,000 mL of intravenous fluid when compared to those receiving ≤ 2,000 mL (p = 0.004) (134). Impaired breathing, pneumothorax, pleural effusion, acute respiratory distress syndrome and pneumonia were some of the pulmonary complications reported (134). Therefore, a fluid management strategy should be considered along with other interventions (such as the use of inferior vena cava filters), in patients at high risk of post-operative pulmonary complications after spine surgery (133, 134).
Limitations
Considering the descriptive nature of our review, we intended to include relevant literature from several kinds of scientific journals. These highly variable sources of information allowed us to summarize different points of view describing the pathophysiology and outcomes associated with fluid management in adult patients undergoing spine surgeries. However, due to their particular complexity, we considered that including certain spine procedures (e.g., occipito-cervical junction and sacro-iliac surgeries) and populations (e.g., pregnant women, pediatric) in our narrative review, would have required a different approach from the one we initially proposed in order to better elucidate the heterogeneity of published literature.
Conclusion
Perioperative fluid therapy in patients undergoing major spine surgeries represents one of the main challenges for anesthesia care providers. To our knowledge, no trials addressing fluid optimization strategies in spine surgery have been published. Prospective randomized clinical studies comparing different fluid therapy strategies are required in order to establish evidence-based guidelines for perioperative fluid management in patients undergoing major spine surgery. Future trials may also need to explore which methods of monitoring fluid responsiveness are most suitable to guide perioperative fluid administration in patients undergoing spine surgery in the prone position. Until more clinical evidence is collected, short- and long-term complications derived from intraoperative fluid overload can presumably be prevented by a better understanding of the physiologic changes in patients undergoing spine surgery.
Author Contributions
CO performed literature search, worked on the structural design, and authored the manuscript. JF-D contributed to the structural design and critically revised the manuscript. OD and AM-M provided editorial advice. ME-V performed literature search, provided conception and structural design of the paper, and co-authored the main text. SB provided the publication concept and editorial advice. All authors reviewed the final manuscript before submission. All authors contributed to the article and approved the submitted version.
Conflict of Interest
The authors declare that the research was conducted in the absence of any commercial or financial relationships that could be construed as a potential conflict of interest.
References
1. Simmons JW, Dobyns JB, Paiste J. Enhanced recovery after surgery: intraoperative fluid management strategies. Surg Clin North Am. (2018) 98:1185–200. doi: 10.1016/j.suc.2018.07.006
2. Makaryus R, Miller TE, Gan TJ. Current concepts of fluid management in enhanced recovery pathways. Br J Anaesth. (2018) 120:376–83. doi: 10.1016/j.bja.2017.10.011
3. Weinberg L, Ianno D, Churilov L, Chao I, Scurrah N, Rachbuch C, et al. Restrictive intraoperative fluid optimisation algorithm improves outcomes in patients undergoing pancreaticoduodenectomy: a prospective multicentre randomized controlled trial. PLoS ONE. (2017) 12:e0183313. doi: 10.1371/journal.pone.0183313
4. Giustiniano E, Procopio F, Ruggieri N, Grimaldi S, Torzilli G, Raimondi F. Impact of the FloTrac/VigileoTM monitoring on intraoperative fluid management and outcome after liver resection. Dig Surg. (2018) 35:435–41. doi: 10.1159/000481406
5. Gandhi SD, Verma K, Aguwa O, Shah SA, Lonner BS. Management of operative blood loss in spinal deformity surgery. Curr Orthopaedic Pract. (2015) 26:291–8. doi: 10.1097/BCO.0000000000000227
6. Bacchin MR, Ceria CM, Giannone S, Ghisi D, Stagni G, Greggi T, et al. Goal-directed fluid therapy based on stroke volume variation in patients undergoing major spine surgery in the prone position: a cohort study. Spine (Phila Pa 1976). (2016) 41:E1131–7. doi: 10.1097/BRS.0000000000001601
7. White SJW, Cheung ZB, Ye I, Phan K, Xu J, Dowdell J, et al. Risk factors for perioperative blood transfusions in adult spinal deformity surgery. World Neurosurg. (2018) 115:e731–7. doi: 10.1016/j.wneu.2018.04.152
8. Schoenfeld AJ, Ochoa LM, Bader JO, Belmont PJ Jr. Risk factors for immediate postoperative complications and mortality following spine surgery: a study of 3475 patients from the National surgical quality improvement program. J Bone Joint Surg Am. (2011) 93:1577–82. doi: 10.2106/JBJS.J.01048
9. Rothrock RJ, Steinberger JM, Badgery H, Hecht AC, Cho SK, Caridi JM, et al. Frailty status as a predictor of 3-month cognitive and functional recovery following spinal surgery: a prospective pilot study. Spine J. (2019) 19:104–12. doi: 10.1016/j.spinee.2018.05.026
10. Epstein NE. Perioperative visual loss following prone spinal surgery: a review. Surg Neurol Int. (2016) 7(Suppl. 13):S347–60. doi: 10.4103/2152-7806.182550
11. Roth S. Perioperative visual loss: what do we know, what can we do? Br J Anaesth. (2009) 103(Suppl. 1):i31–40. doi: 10.1093/bja/aep295
12. Hart RA, Dupaix JP, Rusa R, Kane MS, Volpi JD. Reduction of airway complications with fluid management protocol in patients undergoing cervical decompression and fusion across the cervicothoracic junction. Spine (Phila Pa 1976). (2013) 38:E1135–40. doi: 10.1097/BRS.0b013e31829914ed
13. Zhang N, Liang M, Zhang DD, Xiao YR, Li YZ, Gao YG, et al. Effect of goal-directed fluid therapy on early cognitive function in elderly patients with spinal stenosis: a case-control study. Int J Surg. (2018) 54(Pt A):201–5. doi: 10.1016/j.ijsu.2018.04.007
14. Brown CH, LaFlam A, Max L, Wyrobek J, Neufeld KJ, Kebaish KM, et al. Delirium after spine surgery in older adults: incidence, risk factors, and outcomes. J Am Geriatr Soc. (2016) 64:2101–8. doi: 10.1111/jgs.14434
15. Aiyer S, Kumar NA, Kanna RM, Shetty A, Rajasekaran S. Factors influencing post-operative urinary retention following elective lumbar spine surgery - a prospective study. Glob Spine J. (2018) 8:170S. doi: 10.31616/asj.2018.12.6.1100
16. Johnson RG, Murphy M, Miller M. Fusions and transfusions. An analysis of blood loss and autologous replacement during lumbar fusions. Spine (Phila Pa 1976). (1989) 14:358–62. doi: 10.1097/00007632-198904000-00002
17. Hu SS. Blood loss in adult spinal surgery. Eur Spine J. (2004) 13(Suppl. 1):S3–5. doi: 10.1007/s00586-004-0753-x
18. Elgafy H, Bransford RJ, McGuire RA, Dettori JR, Fischer D. Blood loss in major spine surgery: are there effective measures to decrease massive hemorrhage in major spine fusion surgery? Spine (Phila Pa 1976). (2010) 35(Suppl. 9):S47–56. doi: 10.1097/BRS.0b013e3181d833f6
19. Naik BI, Nemergut EC, Durieux ME. Complexities of bleeding during spine surgery: it'll take your (mechanical) breath away. Anesthesiology. (2017) 126:195. doi: 10.1097/ALN.0000000000001411
20. Dharmavaram S, Jellish WS, Nockels RP, Shea J, Mehmood R, Ghanayem A, et al. Effect of prone positioning systems on hemodynamic and cardiac function during lumbar spine surgery: an echocardiographic study. Spine (Phila Pa 1976). (2006) 31:1388–93; discussion 94. doi: 10.1097/01.brs.0000218485.96713.44
21. Yokoyama M, Ueda W, Hirakawa M, Yamamoto H. Hemodynamic effect of the prone position during anesthesia. Acta Anaesthesiol Scand. (1991) 35:741–4. doi: 10.1111/j.1399-6576.1991.tb03382.x
22. Toyota S, Amaki Y. Hemodynamic evaluation of the prone position by transesophageal echocardiography. J Clin Anesth. (1998) 10:32–5. doi: 10.1016/S0952-8180(97)00216-X
23. Luostarinen T, Lindroos AC, Niiya T, Silvasti-Lundell M, Schramko A, Hernesniemi J, et al. Prone versus sitting position in neurosurgery-differences in patients' hemodynamic management. World Neurosurg. (2017) 97:261–6. doi: 10.1016/j.wneu.2016.10.005
24. Saugel B, Kouz K, Scheeren TWL. The '5 Ts' of perioperative goal-directed haemodynamic therapy. Br J Anaesth. (2019) 123:103–7. doi: 10.1016/j.bja.2019.04.048
25. McNulty SE, Weiss J, Azad SS, Schaefer DM, Osterholm JL, Seltzer JL. The effect of the prone position on venous pressure and blood loss during lumbar laminectomy. J Clin Anesthesia. (1992) 4:220–5. doi: 10.1016/0952-8180(92)90070-H
26. Pearce DJ. The role of posture in laminectomy. Proc R Soc Med. (1957) 50:109–12. doi: 10.1177/003591575705000209
27. Mathai KM, Kang JD, Donaldson WF, Lee JY, Buffington CW. Prediction of blood loss during surgery on the lumbar spine with the patient supported prone on the Jackson table. Spine J. (2012) 12:1103–10. doi: 10.1016/j.spinee.2012.10.027
28. Bhardwaj A, Long DM, Ducker TB, Toung TJ. Neurologic deficits after cervical laminectomy in the prone position. J Neurosurg Anesthesiol. (2001) 13:314–9. doi: 10.1097/00008506-200110000-00006
29. Koh JC, Lee JS, Han DW, Choi S, Chang CH. Increase in airway pressure resulting from prone position patient placing may predict intraoperative surgical blood loss. Spine (Phila Pa 1976). (2013) 38:E678–82. doi: 10.1097/BRS.0b013e31828cb3e5
30. Schonauer C, Bocchetti A, Barbagallo G, Albanese V, Moraci A. Positioning on surgical table. Eur Spine J. (2004) 13(Suppl. 1):S50–5. doi: 10.1007/s00586-004-0728-y
31. Pajewski TN. Anesthesia for anterior/posterior spine surgery. Neuroanesthesia. (2018) 2018:87. doi: 10.1093/med/9780190850036.003.0011
32. Jin SJ, Park YS, Kim SH, Kim D, Shim WH, Jang DM, et al. Effect of prone positional apparatus on the occurrence of acute kidney injury after spine surgery. World Neurosurg. (2019) 128:e597–602. doi: 10.1016/j.wneu.2019.04.216
33. Min JJ, Lee JH, Hong KY, Choi SJ. Utility of stroke volume variation measured using non-invasive bioreactance as a predictor of fluid responsiveness in the prone position. J Clin Monitor Comput. (2016) 31:397–405. doi: 10.1007/s10877-016-9859-z
34. Biais M, Bernard O, Ha JC, Degryse C, Sztark F. Abilities of pulse pressure variations and stroke volume variations to predict fluid responsiveness in prone position during scoliosis surgery. Br J anaesthesia. (2010) 104:407–13. doi: 10.1093/bja/aeq031
35. Ripolles J, Espinosa A, Casans R, Tirado A, Abad A, Fernández C, et al. Colloids versus crystalloids in objective-guided fluid therapy, systematic review and meta-analysis. Too early or too late to draw conclusions. Braz J Anesthesiol. (2015) 65:281–91. doi: 10.1016/j.bjane.2014.07.018
36. Manning MW, Dunkman WJ, Miller TE. Perioperative fluid and hemodynamic management within an enhanced recovery pathway. J Surg Oncol. (2017) 116:592–600. doi: 10.1002/jso.24828
37. Pearse R, Dawson D, Fawcett J, Rhodes A, Grounds RM, Bennett ED. Early goal-directed therapy after major surgery reduces complications and duration of hospital stay. A randomised, controlled trial [ISRCTN38797445]. Crit Care. (2005) 9:R687–93. doi: 10.1186/cc3887
38. Dave C, Shen J, Chaudhuri D, Herritt B, Fernando SM, Reardon PM, et al. Dynamic assessment of fluid responsiveness in surgical ICU patients through stroke volume variation is associated with decreased length of stay and costs: a systematic review and meta-analysis. J Intensive Care Med. (2020) 35:14–23. doi: 10.1177/0885066618805410
39. Habicher M, Balzer F, Mezger V, Niclas J, Müller M, Perka C, et al. Implementation of goal-directed fluid therapy during hip revision arthroplasty: a matched cohort study. Perioper Med. (2016) 5:31. doi: 10.1186/s13741-016-0056-x
40. Thomson R, Meeran H, Valencia O, Al-Subaie N. Goal-directed therapy after cardiac surgery and the incidence of acute kidney injury. J Crit Care. (2014) 29:997–1000. doi: 10.1016/j.jcrc.2014.06.011
41. Chong MA, Wang Y, Berbenetz NM, McConachie I. Does goal-directed haemodynamic and fluid therapy improve peri-operative outcomes?: a systematic review and meta-analysis. Eur J Anaesthesiol. (2018) 35:469–83. doi: 10.1097/EJA.0000000000000778
42. Dalfino L, Giglio MT, Puntillo F, Marucci M, Brienza N. Haemodynamic goal-directed therapy and postoperative infections: earlier is better. A systematic review and meta-analysis. Crit Care. (2011) 15:R154. doi: 10.1186/cc10284
43. Resalt-Pereira M, Munoz JL, Miranda E, Cuquerella V, Perez A. Goal-directed fluid therapy on laparoscopic colorectal surgery within enhanced recovery after surgery program. Rev Esp Anestesiol Reanim. (2019) 66:259–66. doi: 10.1016/j.redare.2019.01.005
44. Phan TD, Ismail H, Heriot AG, Ho KM. Improving perioperative outcomes: fluid optimization with the esophageal Doppler monitor, a metaanalysis and review. J Am Coll Surg. (2008) 207:935–41. doi: 10.1016/j.jamcollsurg.2008.08.007
45. Anastasian ZH, Gaudet JG, Levitt LC, Mergeche JL, Heyer EJ, Berman MF. Factors that correlate with the decision to delay extubation after multilevel prone spine surgery. J Neurosurg Anesthesiol. (2013) 26:167–71. doi: 10.1097/ANA.0000000000000028
46. Li F, Gorji R, Tallarico R, Dodds C, Modes K, Mangat S, et al. Risk factors for delayed extubation in thoracic and lumbar spine surgery: a retrospective analysis of 135 patients. J Anesth. (2013) 28:161–6. doi: 10.1007/s00540-013-1689-2
47. Kwon B, Yoo JU, Furey CG, Rowbottom J, Emery SE. Risk factors for delayed extubation after single-stage, multi-level anterior cervical decompression and posterior fusion. J Spinal Disord Tech. (2006) 19:389–93. doi: 10.1097/00024720-200608000-00002
48. Kla KM, Lee LA. Perioperative visual loss. Best Pract Res Clin Anaesthesiol. (2016) 30:69–77. doi: 10.1016/j.bpa.2015.11.004
49. Lee LA. Risk factors associated with ischemic optic neuropathy after spinal fusion surgery. Anesthesiology. (2012) 116:15–24. doi: 10.1016/j.yane.2012.02.039
50. Siemionow K, Pelton MA, Hoskins JA, Singh K. Predictive factors of hospital stay in patients undergoing minimally invasive ansforaminal lumbar interbody fusion and instrumentation. Spine (Phila Pa 1976). (2012) 37:2046–54. doi: 10.1097/BRS.0b013e31825c6688
51. Siemionow K, Cywinski J, Kusza K, Lieberman I. Intraoperative fluid therapy and pulmonary complications. Orthopedics. (2012) 35:e184–91. doi: 10.3928/01477447-20120123-06
52. Nahtomi-Shick O, Kostuik JP, Winters BD, Breder CD, Sieber AN, Sieber FE. Does intraoperative fluid management in spine surgery predict intensive care unit length of stay? J Clin Anesth. (2001) 13:208–12. doi: 10.1016/S0952-8180(01)00244-6
53. Picard J, Bedague D, Bouzat P, Ollinet C, Albaladejo P, Bosson JL, et al. Oesophageal doppler to optimize intraoperative haemodynamics during prone position. a randomized controlled trial. Anaesth Crit Care Pain Med. (2016) 35:255–60. doi: 10.1016/j.accpm.2015.12.011
54. Farag E, Sessler DI, Kovaci B, Wang L, Mascha EJ, Bell G, et al. Effects of crystalloid versus colloid and the alpha-2 agonist brimonidine versus placebo on intraocular pressure during prone spine surgery: a factorial randomized trial. Anesthesiology. (2012) 116:807–15. doi: 10.1097/ALN.0b013e3182475c10
55. Yang SY, Shim JK, Song Y, Seo SJ, Kwak YL. Validation of pulse pressure variation and corrected flow time as predictors of fluid responsiveness in patients in the prone position. Br J Anaesth. (2013) 110:713–20. doi: 10.1093/bja/aes475
56. Kim DH, Shin S, Kim JY, Kim SH, Jo M, Choi YS. Pulse pressure variation and pleth variability index as predictors of fluid responsiveness in patients undergoing spinal surgery in the prone position. Ther Clin Risk Manag. (2018) 14:1175–83. doi: 10.2147/TCRM.S170395
57. Rankin D, Zuleta-Alarcon A, Soghomonyan S, Abdel-Rasoul M, Castellon-Larios K, Bergese SD. Massive blood loss in elective spinal and orthopedic surgery: retrospective review of intraoperative transfusion strategy. J Clin Anesth. (2017) 37:69–73. doi: 10.1016/j.jclinane.2016.10.017
58. Huang YH, Ou CY. Significant blood loss in lumbar fusion surgery for degenerative spine. World Neurosurg. (2015) 84:780–5. doi: 10.1016/j.wneu.2015.05.007
59. Slattery C, Kark J, Wagner T, Verma K. The use of Tranexamic acid to reduce surgical blood loss: a review basic science, subspecialty studies, and the evolution of use in spine deformity surgery. Clin Spine Surg. (2019) 32:46–50. doi: 10.1097/BSD.0000000000000808
60. Carabini LM, Moreland NC, Vealey RJ, Bebawy JF, Koski TR, Koht A, et al. A randomized controlled trial of low-dose Tranexamic acid versus placebo to reduce red blood cell transfusion during complex multilevel spine fusion surgery. World Neurosurg. (2018) 110:e572–9. doi: 10.1016/j.wneu.2017.11.070
61. Buell TJ, Taylor DG, Chen CJ, Dunn LK, Mullin JP, Mazur MD, et al. Rotational thromboelastometry-guided transfusion during lumbar pedicle subtraction osteotomy for adult spinal deformity: preliminary findings from a matched cohort study. Neurosurg Focus. (2019) 46:E17. doi: 10.3171/2019.1.FOCUS18572
62. Naik BI, Durieux ME, Knisely A, Sharma J, Bui-Huynh VC, Yalamuru B, et al. SEER sonorheometry versus rotational thromboelastometry in large volume blood loss spine surgery. Anesth Analg. (2016) 123:1380–9. doi: 10.1213/ANE.0000000000001509
63. Krausz MM. Initial resuscitation of hemorrhagic shock. World J Emerg Surg. (2006) 1:14. doi: 10.1186/1749-7922-1-14
64. Spahn DR, Bouillon B, Cerny V, Duranteau J, Filipescu D, Hunt BJ, et al. The European guideline on management of major bleeding and coagulopathy following trauma: fifth edition. Crit Care. (2019) 23:98. doi: 10.1186/s13054-019-2347-3
65. Misir A, Mehrotra S. Fluid and medication considerations in the Traumatized patient. Curr pediatr Rev. (2018) 14:9–27. doi: 10.2174/1573396313666170815101504
66. Hahn RG. Adverse effects of crystalloid and colloid fluids. Anaesthesiol Intensive Ther. (2017) 49:303–8. doi: 10.5603/AIT.a2017.0045
67. Harrell BR, Miller S. Abdominal compartment syndrome as a complication of fluid resuscitation. Nurs Clin North Am. (2017) 52:331–8. doi: 10.1016/j.cnur.2017.01.010
68. Jones DG, Nantais J, Rezende-Neto JB, Yazdani S, Vegas P, Rizoli S. Crystalloid resuscitation in trauma patients: deleterious effect of 5L or more in the first 24h. BMC Surg. (2018) 18:93. doi: 10.1186/s12893-018-0427-y
69. Song JW, Shim JK, Kim NY, Jang J, Kwak YL. The effect of 0.9% saline versus plasmalyte on coagulation in patients undergoing lumbar spinal surgery; a randomized controlled trial. Int J Surg. (2015) 20:128–34. doi: 10.1016/j.ijsu.2015.06.065
70. Bhaskaran K, Arumugam G, Vinay Kumar PV. A prospective, randomized, comparison study on effect of perioperative use of chloride liberal intravenous fluids versus chloride restricted intravenous fluids on postoperative acute kidney injury in patients undergoing off-pump coronary artery bypass grafting surgeries. Ann Card Anaesth. (2018) 21:413–8. doi: 10.4103/aca.ACA_230_17
71. Madani SJ, Araste Fard F, Karimi-Sari H, Khosravi MH, Delavari A. Comparison of acid–base and electrolyte imbalances between normal saline and 1.4% sodium bicarbonate intravenous fluids therapy during cervical and lumbar laminectomy. Polish Ann Med. (2017) 24:117–22. doi: 10.1016/j.poamed.2016.03.005
72. Shaw AD, Bagshaw SM, Goldstein SL, Scherer LA, Duan M, Schermer CR, et al. Major complications, mortality, and resource utilization after open abdominal surgery: 0.9% saline compared to Plasma-Lyte. Ann Surg. (2012) 255:821–9. doi: 10.1097/SLA.0b013e31825074f5
73. Kellum JA, Song M, Almasri E. Hyperchloremic acidosis increases circulating inflammatory molecules in experimental sepsis. Chest. (2006) 130:962–7. doi: 10.1378/chest.130.4.962
74. Zarbock A, Schmolke M, Spieker T, Jurk K, van Aken H, Singbartl K. Acute uremia but not renal inflammation attenuates aseptic acute lung injury: a critical role for uremic neutrophils. J Am Soc Nephrol. (2006) 17:3124–31. doi: 10.1681/ASN.2006040358
75. Niemi TT, Miyashita R, Yamakage M. Colloid solutions: a clinical update. J Anesthesia. (2010) 24:913–25. doi: 10.1007/s00540-010-1034-y
76. Jang MS, Han JH, Lee S, Kim SE. Postoperative blood loss and coagulation changes after balanced 6% hydroxyethyl starch 130/0.4 administration during spine surgery: a retrospective study. Clin Spine Surg. (2019) 32:E65–70. doi: 10.1097/BSD.0000000000000727
77. Joosten A, Delaporte A, Mortier J, Ickx B, van Obbergh L, Vincent JL, et al. Long-term impact of crystalloid versus colloid solutions on renal function and disability-free survival after major abdominal surgery. Anesthesiology. (2019) 130:227–36. doi: 10.1097/ALN.0000000000002501
78. Haghighi SS, Oro JJ. Effects of hypovolemic hypotensive shock on somatosensory and motor evoked potentials. Neurosurgery. (1989) 24:246–52. doi: 10.1227/00006123-198902000-00015
79. Lieberman JA, Feiner J, Lyon R, Rollins MD. Effect of hemorrhage and hypotension on transcranial motor-evoked potentials in swine. Anesthesiology. (2013) 119:1109–19. doi: 10.1097/ALN.0b013e31829d4a92
80. Acharya S, Palukuri N, Gupta P, Kohli M. Transcranial motor evoked potentials during spinal deformity corrections-safety, efficacy, limitations, and the role of a checklist. Front Surg. (2017) 4:8. doi: 10.3389/fsurg.2017.00008
81. Rubin DS, Parakati I, Lee LA, Moss HE, Joslin CE, Roth S. Perioperative visual loss in spine fusion surgery: ischemic optic neuropathy in the United States from 1998 to 2012 in the nationwide inpatient sample. Anesthesiology. (2016) 125:457–64. doi: 10.1097/ALN.0000000000001211
82. Shillingford JN, Laratta JL, Sarpong NO, Swindell H, Cerpa M, Lehman RA, et al. Visual loss following spine surgery: what have we seen within the scoliosis research society morbidity and mortality database? Spine (Phila Pa 1976). (2018) 43:1201–7. doi: 10.1097/BRS.0000000000002592
83. Li A, Swinney C, Veeravagu A, Bhatti I, Ratliff J. Postoperative visual loss following lumbar spine surgery: a review of risk factors by diagnosis. World Neurosurg. (2015) 84:2010–21. doi: 10.1016/j.wneu.2015.08.030
84. Lee LA, Roth S, Posner KL, Cheney FW, Caplan RA, Newman NJ, et al. The American society of anesthesiologists postoperative visual loss registry: analysis of 93 spine surgery cases with postoperative visual loss. Anesthesiology. (2006) 105:652–9. doi: 10.1097/00000542-200610000-00007
85. Nandyala SV, Marquez-Lara A, Fineberg SJ, Singh R, Singh K. Incidence and risk factors for perioperative visual loss after spinal fusion. Spine J. (2014) 14:1866–72. doi: 10.1016/j.spinee.2013.10.026
86. Farag E, Doyle DJ. Vision loss after spine surgery: a new hypothesis. Can J Anaesth. (2006) 53:420. doi: 10.1007/BF03022516
87. Apfelbaum JL, Roth S, Rubin D, Connis RT, Agarkar M, Arnold PM. Practice advisory for perioperative visual loss associated with spine surgery 2019: an updated report by the American society of anesthesiologists task force on perioperative visual loss, the North American neuro-ophthalmology society, and the society for neuroscience in anesthesiology and critical care. Anesthesiology. (2019) 130:12–30. doi: 10.1097/ALN.0000000000002503
88. Yoshioka S, Takano T, Ryujin F, Takeuchi Y. A pediatric case of reversible cerebral vasoconstriction syndrome with cortical subarachnoid hemorrhage. Brain Dev. (2012) 34:796–8. doi: 10.1016/j.braindev.2012.01.001
89. Özkiriş M, Akin I, Özkiriş A, Adam M, Saydam L. Ischemic optic neuropathy after carotid body tumor resection. J Craniofac Surg. (2014) 25:e58–61. doi: 10.1097/SCS.0b013e3182a306cc
90. Shapira OM, Kimmel WA, Lindsey PS, Shahian DM. Anterior ischemic optic neuropathy after open heart operations. Ann Thorac Surg. (1996) 61:660–6. doi: 10.1016/0003-4975(95)01108-0
91. Su AW, Lin SC, Larson AN. Perioperative vision loss in spine surgery and other orthopaedic procedures. J Am Acad Orthopaedic Surg. (2016) 24:702–10. doi: 10.5435/JAAOS-D-15-00351
92. Lee LA. Perioperative visual loss and anesthetic management. Curr Opin Anaesthesiol. (2013) 26:375–81. doi: 10.1097/ACO.0b013e328360dcd9
93. Milner HE, Wilson RJT, Davies SJ, Yates DRA. Crystalloid or colloid for goal-directed fluid therapy in colorectal surgery. Br J Anaesth. (2013) 112:281–9. doi: 10.1093/bja/aet307
94. James MF, Michell WL, Joubert IA, Nicol AJ, Navsaria PH, Gillespie RS. Resuscitation with hydroxyethyl starch improves renal function and lactate clearance in penetrating trauma in a randomized controlled study: the FIRST trial (Fluids in Resuscitation of Severe Trauma). Br J Anaesth. (2011) 107:693–702. doi: 10.1093/bja/aer229
95. Finfer S, Bellomo R, Boyce N, French J, Myburgh J, Norton R, et al. A comparison of albumin and saline for fluid resuscitation in the intensive care unit. N Engl J Med. (2004) 350:2247–56. doi: 10.1056/NEJMoa040232
96. Fandino W. Strategies to prevent ischemic optic neuropathy following major spine surgery: a narrative review. J Clin Anesth. (2017) 43:50–8. doi: 10.1016/j.jclinane.2017.09.009
97. Foroozan R, Golnik KC. Are anemia and hypotension causally related to perioperative ischemic optic neuropathy? J Neuro Ophthalmol. (2017) 37:81–6. doi: 10.1097/WNO.0000000000000479
98. Pandey VP, Dasgupta A, Aggarwal A, Jain S. Perioperative visual deficit in a patient undergoing dorsolumbar spine surgery. J Neuroanaesthesiol Crit Care. (2018) 5:195–7. doi: 10.1055/s-0038-1667211
99. Buono LM, Foroozan R. Perioperative posterior ischemic optic neuropathy: review of the literature. Surv Ophthalmol. (2005) 50:15–26. doi: 10.1016/j.survophthal.2004.10.005
100. Price TP, Ivashchenko A, Schurr MJ. Perioperative visual loss after excision and autografting of a thermal burn to the back. Burns. (2014) 40:e31–4. doi: 10.1016/j.burns.2013.12.022
101. Roth S, Moss HE. update on perioperative ischemic optic neuropathy associated with non-ophthalmic surgery. Front Neurol. (2018) 9:557. doi: 10.3389/fneur.2018.00557
102. Kitaba A, Martin DP, Gopalakrishnan S, Tobias JD. Perioperative visual loss after nonocular surgery. J Anesth. (2013) 27:919–26. doi: 10.1007/s00540-013-1648-y
103. Reinhart K, Perner A, Sprung CL, Jaeschke R, Schortgen F, Groeneveld ABJ, et al. Consensus statement of the ESICM task force on colloid volume therapy in critically ill patients. Intensive Care Med. (2012) 38:368–83. doi: 10.1007/s00134-012-2472-9
104. Larson CP Jr. Excessive crystalloid infusion may contribute to ischemic optic neuropathy. Anesthesiology. (2007) 106:1249. doi: 10.1097/01.anes.0000267622.64914.a1
105. Baig MN, Lubow M, Immesoete P, Bergese SD, Hamdy EA, Mendel E. Vision loss after spine surgery: review of the literature and recommendations. Neurosurg Focus. (2007) 23:E15. doi: 10.3171/FOC-07/11/15
106. Ramchandran S, Day LM, Line B, Buckland AJ, Passias P, Protopsaltis T, et al. The impact of different intraoperative fluid administration strategies on postoperative extubation following multilevel thoracic and lumbar spine surgery: a propensity score matched analysis. Neurosurgery. (2018) 85:31–40. doi: 10.1093/neuros/nyy226
107. Heitz JW, Audu PB. Asymmetric postoperative visual loss after spine surgery in the lateral decubitus position. Br J Anaesth. (2008) 101:380–2. doi: 10.1093/bja/aen163
108. Sawyer F, Phan T, Moreno Duarte I, Bonney I, Schumann R. Perioperative airway changes and their return to baseline in patients undergoing elective surgery in the prone and trendelenburg positions: early data. Anesth Analg. (2016) 122:S6. doi: 10.1213/01.ane.0000482829.38701.68
109. Sagi HC, Beutler W, Carroll E, Connolly PJ. Airway complications associated with surgery on the anterior cervical spine. Spine (Phila Pa 1976). (2002) 27:949–53. doi: 10.1097/00007632-200205010-00013
110. Cata JP, Noguera EM, Parke E, Ebrahim Z, Kurz A, Kalfas I, et al. Patient-controlled epidural analgesia (PCEA) for postoperative pain control after lumbar spine surgery. J Neurosurg Anesthesiol. (2008) 20:256–60. doi: 10.1097/ANA.0b013e31817ffe90
111. Sheshadri V, Moga R, Manninen P, Goldstein CL, Rampersaud YR, Massicotte EM, et al. Airway adverse events following posterior occipito-cervical spinal fusion. J Clin Neurosci. (2017) 39:124–9. doi: 10.1016/j.jocn.2016.12.036
112. Niescery J, Huhmann N, Dasch B, Bullmann V, Weber TP, Bellgardt M, et al. Effects of liberal vs. conventional volume regimen on pulmonary function in posterior scoliosis surgery. Middle East J Anesthesiol. (2013) 22:165–71.
113. Modes K, Li F, Gorji R, Zhong J. Risk factors for delayed extubation in major thoracolumbar spine surgery. J Neurosurg Anesthesiol. (2011) 23:415–6. doi: 10.1097/ANA.0b013e31822d8464
114. Baldini G, Bagry H, Aprikian A, Carli F. Postoperative urinary retention: anesthetic and perioperative considerations. Anesthesiology. (2009) 110:1139–57. doi: 10.1097/ALN.0b013e31819f7aea
115. Jackson J, Davies P, Leggett N, Nugawela MD, Scott LJ, Leach V, et al. Systematic review of interventions for the prevention and treatment of postoperative urinary retention. BJS open. (2018) 3:11–23. doi: 10.1002/bjs5.50114
116. Altschul D, Kobets A, Nakhla J, Jada A, Nasser R, Kinon MD, et al. Postoperative urinary retention in patients undergoing elective spinal surgery. J Neurosurg Spine. (2017) 26:229–34. doi: 10.3171/2016.8.SPINE151371
117. Golubovsky JL, Ilyas H, Chen J, Tanenbaum JE, Mroz TE, Steinmetz MP. Risk factors and associated complications for postoperative urinary retention after lumbar surgery for lumbar spinal stenosis. Spine J. (2018) 18:1533–9. doi: 10.1016/j.spinee.2018.01.022
118. Boulis NM, Mian FS, Rodriguez D, Cho E, Hoff JT. Urinary retention following routine neurosurgical spine procedures. Surg Neurol. (2001) 55:23–7; discussion 7–8. doi: 10.1016/S0090-3019(01)00331-7
119. Gandhi SD, Patel SA, Maltenfort M, Anderson DG, Vaccaro AR, Albert TJ, et al. Patient and surgical factors associated with postoperative urinary retention after lumbar spine surgery. Spine (Phila Pa 1976). (2014) 39:1905–9. doi: 10.1097/BRS.0000000000000572
120. Lee S, Kim CH, Chung CK, Park SB, Yang SH, Kim SH, et al. Risk factor analysis for postoperative urinary retention after surgery for degenerative lumbar spinal stenosis. Spine J. (2017) 17:469–77. doi: 10.1016/j.spinee.2016.03.017
121. Keskinen H, Helenius L, Pajulo O, Helenius IJ. Postoperative urinary retention or difficulties to empty the bladder in young patients undergoing posterior spinal fusion for adolescent idiopathic scoliosis. J Pediatr Surg. (2018) 53:1542–6. doi: 10.1016/j.jpedsurg.2017.09.023
122. Toyonaga T, Matsushima M, Sogawa N, Jiang SF, Matsumura N, Shimojima Y, et al. Postoperative urinary retention after surgery for benign anorectal disease: potential risk factors and strategy for prevention. Int J Colorectal Dis. (2006) 21:676–82. doi: 10.1007/s00384-005-0077-2
123. Keita H, Diouf E, Tubach F, Brouwer T, Dahmani S, Mantz J, et al. Predictive factors of early postoperative urinary retention in the postanesthesia care unit. Anesth Analg. (2005) 101:592–6. doi: 10.1213/01.ANE.0000159165.90094.40
124. Petros JG, Rimm EB, Robillard RJ, Argy O. Factors influencing postoperative urinary retention in patients undergoing elective inguinal herniorrhaphy. Am J Surg. (1991) 161:431–3; discussion 4. doi: 10.1016/0002-9610(91)91105-R
125. Sherburne E, Sawin K. Investigating time to void after lower-extremity orthopedic surgery in a pediatric population. J Spec Pediatr Nurs. (2008) 13:36–47. doi: 10.1111/j.1744-6155.2008.00132.x
126. Kanaan SF, Waitman LR, Yeh HW, Arnold PM, Burton DC, Sharma NK. Structural equation model analysis of the length-of-hospital stay after lumbar spine surgery. Spine J. (2015) 15:612–21. doi: 10.1016/j.spinee.2014.11.001
127. Gruskay JA, Fu M, Bohl DD, Webb ML, Grauer JN. Factors affecting length of stay after elective posterior lumbar spine surgery: a multivariate analysis. Spine J. (2015) 15:1188–95. doi: 10.1016/j.spinee.2013.10.022
128. Zheng F, Cammisa FP, Sandhu HS, Girardi FP, Khan SN. Factors predicting hospital stay, operative time, blood loss, and transfusion in patients undergoing revision posterior lumbar spine decompression, fusion, and segmental instrumentation. Spine (Phila Pa 1976). (2002) 27:818–24. doi: 10.1097/00007632-200204150-00008
129. Chibbaro S, Cebula H, Aldea S, Baussart B, Tigan L, Todeschi J, et al. Endonasal endoscopic odontoidectomy in ventral diseases of the craniocervical junction: results of a multicenter experience. World Neurosurg. (2017) 106:382–93. doi: 10.1016/j.wneu.2017.06.148
130. Pham MH, Tuchman A, Platt A, Hsieh PC. Intracranial complications associated with spinal surgery. Eur Spine J. (2016) 25:888–94. doi: 10.1007/s00586-015-4241-2
131. Radcliff KE, Sidhu GD, Kepler CK, Gruskay J, Anderson DG, Hilibrand A, et al. Complications of flat bed rest after incidental durotomy. Clin Spine Surg. (2016) 29:281–4. doi: 10.1097/BSD.0b013e31827d7ad8
132. Ganau M, Prisco L, Cebula H, Todeschi J, Abid H, Ligarotti G, et al. Risk of deep vein thrombosis in neurosurgery: state of the art on prophylaxis protocols and best clinical practices. J Clin Neurosci. (2017) 45:60–6. doi: 10.1016/j.jocn.2017.08.008
133. Fineberg SJ, Oglesby M, Patel AA, Pelton MA, Singh K. The incidence and mortality of thromboembolic events in lumbar spine surgery. Spine. (2013) 38:1154–9. doi: 10.1097/BRS.0b013e318286b7c0
Keywords: spine surgery, prone position, fluid therapy, fluid management, perioperative care
Citation: Ongaigui C, Fiorda-Diaz J, Dada O, Mavarez-Martinez A, Echeverria-Villalobos M and Bergese SD (2020) Intraoperative Fluid Management in Patients Undergoing Spine Surgery: A Narrative Review. Front. Surg. 7:45. doi: 10.3389/fsurg.2020.00045
Received: 07 November 2019; Accepted: 17 June 2020;
Published: 29 July 2020.
Edited by:
Philipp Taussky, The University of Utah, United StatesReviewed by:
Mario Ganau, University of Toronto, CanadaGirija Prasad Rath, All India Institute of Medical Sciences, India
Copyright © 2020 Ongaigui, Fiorda-Diaz, Dada, Mavarez-Martinez, Echeverria-Villalobos and Bergese. This is an open-access article distributed under the terms of the Creative Commons Attribution License (CC BY). The use, distribution or reproduction in other forums is permitted, provided the original author(s) and the copyright owner(s) are credited and that the original publication in this journal is cited, in accordance with accepted academic practice. No use, distribution or reproduction is permitted which does not comply with these terms.
*Correspondence: Juan Fiorda-Diaz, anVhbi5maW9yZGFAb3N1bWMuZWR1