Corrigendum: Keeping Track of Greenhouse Gas Emission Reduction Progress and Targets in 167 Cities Worldwide
- 1School of Environmental Science and Engineering, Sun Yat-sen University, Guangzhou, China
- 2Guangdong Provincial Key Laboratory of Environmental Pollution Control and Remediation Technology, Guangzhou, China
Actions in cities shape the outcome of greenhouse gas (GHG) emission mitigation and our climate change response. Accurate and consistent carbon inventories are essential for identifying the main sources of emissions and global comparison of carbon reduction progress and would help inform targeted policies for low-carbon transition. To identify the effectiveness of historical carbon reduction policies, our study conducted energy-related GHG emission inventories for 167 globally distributed cities with information from different sectors, and assessed the city-scale near-term, mid-term, and long-term goals carbon mitigation targets from 2020 to 2050. On this basis, we propose mitigation strategies to achieve local and global climate targets. We found that, although Asian cities are the biggest carbon emitters in totals, the per capita GHG emissions of cities in developed countries are still generally higher than that in developing countries. In terms of sectors, the GHG emissions from the stationary energy uses (such as residential, commercial, and industrial buildings) and transportation sector contributed the most. However, cities in more developed nations have been inclined to set absolute carbon reduction targets before 2050, while intensity reduction target has been largely set for cities at the stage of rapid economic growth and accelerated industrialization. More ambitious and easily-tracked climate targets should be proposed by cities and more effective measures of reducing GHG emissions are required to stay consistent with the global ambition of climate change mitigation.
Introduction
The Paris Agreement was adopted by more than 170 countries in 2015. The aim of this agreement is to constrain global warming to levels well below 2°C or even 1.5°C compared with pre-industrial levels (UNFCCC, 2015; Rogelj et al., 2019). Under this agreement, many global regions have proposed specific targets for greenhouse gas (GHG) mitigation and stricter environmental polices (Liobikiene and Butkus, 2017; Sobrinho et al., 2020; Zhou et al., 2021). However, there is still a significant gap in achieving these climate targets. According to Emission Gap Report 2020, the world is still heading for a temperature rise in excess of 3°C by the end of the twenty-first century. Although there is an ~66% possibility of achieving the goal of limiting global temperature increase to below 2°C when green recovery is prioritized by international communities, the relevant efforts are insufficient to achieve the 1.5°C targets (UNEP, 2020). Greater and sustainable efforts are still needed worldwide to address climate change.
The climate effects of urbanization and urbanized economy have received increasing concerns in recent decades (Georgeson et al., 2016; Sun et al., 2016). Cities are reported to be responsible for more than 70% of GHG emissions (IEA, 2012; IIASA, 2012), and they share a big responsibility of decarbonization of the global economy. Numerous previous studies have investigated the GHG emission inventories for global cities that were developed based on various reporting methods (Dodman, 2009; Kennedy et al., 2009, 2010; Sovacool and Brown, 2010; Chen and Chen, 2012; Ibrahim et al., 2012). Although a recommended international standard for the accounting of community-scale GHG emissions has been published (GPC, 2014), current inventory methods used by cities significantly vary, making it hard to assess and compare the progress of emission mitigation over time and space. For example, CO2 emissions have been estimated using the methods of the Intergovernmental Panel on Climate Change (Shan et al., 2018) or the multi-regional input–output model (Kanemoto et al., 2020) for cities.
City-level GHG emission inventories have been widely developed in many countries. For instance, the characteristics of CO2 emissions in 12 East Asian megacities (in China, South Korea, and Japan) were identified by incorporating emission inventories into spatial mapping models and a driving forces analysis was conducted based on their carbon reduction targets (Sun et al., 2021). The inventories of GHG emission of 18 cities in China from 2000 to 2014 were established to analyze the effects of socioeconomic development and industrial structure (Shan et al., 2017; Xu et al., 2018). In addition, carbon inventories of 294 Chinese cities were built by applying China high-resolution emission database, which suggests that reducing emission intensity through improving the efficiency of production and energy use is one of most important strategies for decarbonization (Shan et al., 2021).
In addition, studies have been looking into the variations of carbon reduction targets among cities. Many cities in the European Union (EU), have committed to climate targets that lead to a sharp carbon reduction or even carbon neutrality (Reckien et al., 2018; Salvia et al., 2021). Hsu et al. (2020) suggested that the European cities are achieving their carbon redution targets guadually and 60% of EU Covenant of Mayors' cities are able to meet their 2020 carbon reduction targets. Salvia et al. (2021) assessed local-level plans of 327 EU cities and indicated some should double their ambitions to meet the aims set by Paris Agreement. Some Asian cities also obtained achievements in reducing GHG emission. However, the potentials of carbon reduction in these areas are still large. Sun et al. (2021) suggested if to achieve the 1.5° climate goal, the CO2 emission per capita in Seoul, Tokyo Metropolis, and Beijing would have to decrease by 36, 26, and 35% in 2030 compared to 2015 levels, repectively. Overall, current urban decarbonization targets are still not sufficient to achieve the global climate targets by the end of this centrury. Although researches have evaluated the effectiveness of GHG emission reduction progress or climate targets in some individual cities, a global inventory for cities at different development stages at sectoral scale is lacking and comparisons between different years is often in consistent. Also, it is also essential to compare carbon reduction targets among cities, which is important for assessing the gaps between current mitigation progress and future mitigation expectation.
To address these issues, our study assessed the progress of historical GHG emission reduction and the climate targets of global cities in a comparable manner. First, we conducted the sector-level GHG emission inventories for 167 major cities across the globe at different developmental stages and compare their differences in main emission contributors. Then, the carbon reduction progresses of cities were analyzed and compared based on the inventories of emissions recorded in different years. Finally, the city-scale near-term, mid-term, and long-term goals carbon mitigation targets from 2020 to 2050 were assessed and discussed for their climate relevance.
Methods and Data
Our urban sample consists of 167 global urban regions (cities or metropolitan areas) from 53 countries worldwide, which was selected based on the global coverage and representativeness in urban sizes and regional distribution. These representative urban samples are usually core cities, larger urban zones, and metropolitan areas in their countries. For the major carbon emitters in the world (such as China, the US, India and the EU), more cities would be selected to increase the relevance to the climate discourse. Finally, the carbon data at city level has a lower availability than that in country level. The selection of samples is also constrained by the GHG emission and mitigation target data that are most available in the study period, with the goal of ensuring consistency and continuity of inter-city emissions comparison. We also distinguished between the degree of development of these cities, which were based on whether they belong to developed and developing countries in the UN classification criteria.
We conducted detailed sector-level inventories for the GHG emissions in cities, which are represented by CO2 equivalent (CO2-eq). Two major data sources used in our study are: the public databases of C40 Cities (https://www.c40.org/) and CDP (Carbon Disclosure Project) platform (https://data.cdp.net/). C40 Cities is a network that connected 97 of world's greatest cities to address climate change, including information about carbon emission and reduction plans. Carbon Disclosure Project is a global disclosure system that have the most comprehensive collection of self-reported environmental data in the world, which contains data on GHG emissions, climate mitigation, adaption plans, and climate targets. These databases have high coverage of global cities and relatively good data quality, and are available to the public, which have been widely used by previous urban carbon studies (Nangini et al., 2019; Wiedmann et al., 2020; Salvia et al., 2021). Population data was also collected from the two major databases above, together with local city statistics, which were used for further analysis (i.e., the calculation of per capita GHG emissions and indicate the urban sizes). For the analysis of carbon reduction targets contributed by cities, the primary data source was the CDP platform. Climate action plans of cities or city-authored mitigation policy documents were also collected and used as complements when necessary. Three major steps of study are:
GHG Emission Inventories
Our inventories cataloged GHG emissions by eight urban sectors: residential and institutional buildings; commercial buildings; industrial buildings (energy use); industrial process and fugitive emissions; on-road transportation (e.g., cars, buses); railways, aviation, and waterway; waste disposal (wastewater treatment, landfills); and other (agriculture, mining). Both territorial emissions within urban geographical boundaries and those related to imported electricity were included in these inventories. When emission data of some cities are available at different data sources, the inventory data from C40 Cities were selected to maximize data consistency and avoid mismatches of statistical calibers. Because of the scarcity of emission data at sector scale, some cities may have missing data for certain sectors (for example, waste-related emission data was not available for Amsterdam and other emission (agriculture, mining) data was not available for Los Angeles).
Tracking of Historical Emission Change
We selected the 42 out of the 167 cities that had GHG emission inventories for at least 2 years to analyze the progresses of GHG emission reduction in cities. The basic information and study periods of these cities are shown in Table A1. If cities had inventories for more than 2 years, we prioritized the use of data within the same source. Additionally, we tend to maximize the timespan by using the first year and last year data that were available to facilitate a more meaningful comparison. If an urban region only had 2 years of inventories with different data sources, we first determine whether their emission boundaries are consistent by comparing their urban land areas in respective data sources. If they are consistent, we confirm the GHG emission data of the city is comparable and could be selected.
Carbon Reduction Target Analysis
Our survey showed that 113 out of the 167 cities have set clear and traceable carbon reduction targets. The target information was extracted on the basis of the following aspects: general city information (name, location, and boundaries), target details (reduction target types and magnitude, baseline year, and the latest update date), and supporting data (i.e., population). The near-term (2020s), mid-term (2030s), and long-term (2040s−2050) climate targets were identified for each city. They are further classified by absolute emission reduction targets, intensity targets, and baseline scenario targets to discuss their climate relevance and appropriateness from a global perspective.
Results
GHG Emissions From Cities and Their Sectors
The total and per capita GHG emissions of the 167 cities are mapped in Figure 1. As shown in Figure 1a, the total GHG emissions ranged between 3.5 kt CO2-eq and 199.7 Mt CO2-eq. The top 25 (15%) of the 167 cities accounted for 52% of the total GHG emissions, which are mainly from Asian and European countries [such as China (Handan, Shanghai, and Suzhou) and Japan (Tokyo), Russia (Moscow) and Turkey (Istanbul)]. As suggested by Moran et al. (2018), concerted actions implemented by a small number of local governments could have remarkable impacts on global emissions reduction. In general, both developed and developing countries have cities with high total GHG emissions, and some cities in developed countries still generated a great deal of emissions (such as cities in Japan, the USA, Korea, Germany, and Singapore) in the study period. This could raise equity concerns, since major cities have generated the biggest share of GHG emissions, but the consequences of climate change (such as extreme weathers, wide fires, and biodiversity loss) are borne by the whole world, and the poorer regions are possibly more vulnerable to these consequences (Morgan and Waskow, 2014).
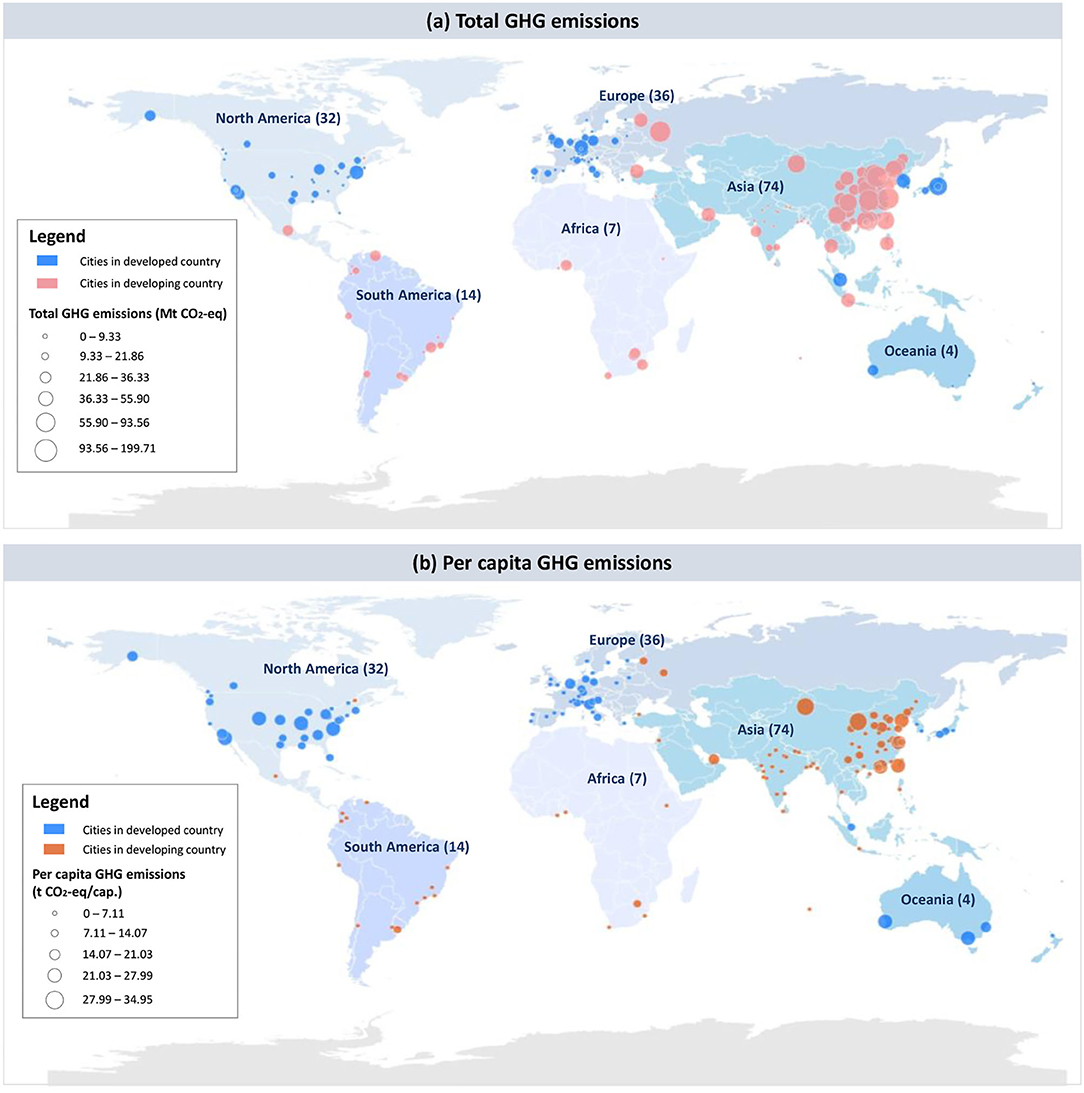
Figure 1. The (a) total GHG emissions and (b) per capita GHG emissions of 167 cities. Most recent data of cities are shown, which could be in different years (2005–2016), depending on the data availability.
The divide of per capita GHG emissions among cities were also huge (ranging from 0.15–34.95 t CO2-eq/capita) (Figure 1b). Cities in the USA, Europe, and Australia had a notably higher per capita GHG emissions than those in most developing areas. For the former cities, efforts for decreasing per capita GHG emissions should be further enhanced. Though China is still a developing country, several Chinese cities (such as Yinchuan, Urumqi, and Dalian) had the per capita GHG emissions (34.95, 30.99, and 24.30 t CO2-eq/capita, respectively) approaching those of developed countries, which is partially attributed to their rapid urbanization, industrialization, and relatively high reliance on coal energy. Another major reason is that many high-carbon production chains were outsourced from developed nations to Chinese cities, thus increasing the export-related emission of the latter.
The sector-level emissions of cities around 2012 (108 cities in total) were shown in Figure 2. Stationary energy is one of the largest contributors to GHG emissions of cities, which includes emissions from the fuel combustion and electricity use in residential and institutional buildings, commercial buildings, and industrial buildings in the study. Nearly half of the 108 cities had stationary energy emissions that represented more than 70% of their total GHG emissions, and more than 80% of the cities had stationary energy emissions that represented over 50% of total emissions; therefore, most of these cities can achieve significant GHG reduction progress only if this portion of energy emissions can be well-controlled. Stationary energy emissions are typically prominent in Chinese cities (especially from industrial buildings). Among them, Shanghai, Suzhou, Dalian, Handan, and Tianjin had the largest stationary energy emissions, largely because of their big manufacturing or service sectors. In comparison, some South American and Asian cities had a relatively small proportion of stationary energy emissions. For example, Belo Horizonte in Brazil had 25% of GHG emissions from stationary energy, while the proportions for Amman and Manila of Asia were 24 and 22%, respectively, which was due to the relatively more developed agricultural and farming industries in these regions. Emission of stationary energy in European and North American cities contributes 60–80% (e.g., 74% in New York City, Chicago, Torino, and 60% in Stockholm). Consumers in richer cities tend to buy more and carbon-intensive products manufactured in Chinese cities or other upstream regions, which would greatly increase the energy demand and GHG emissions within those areas. Therefore, plateauing or reducing the consumption for consumers worldwide should be considered as an important supplementary strategy for mitigating GHG emissions that related to international trade.
Transportation also plays an important role in the total GHG emissions in most cities. In about one-third of the cities, more than 30% of total GHG emissions were from on-road transportation. In comparison, the emissions from railways, aviation, and waterways (<15% of total GHG emissions) were much lower. The variation of emissions in the transportation sector may due to a range of factors such as economic development, urban forms, traffic structures, and types of vehicle fuel (Chester and Cano, 2016; Li et al., 2019a). The average GHG emissions of on-road transportation in developed regions was about two times of that in the developing regions. In cities of developed nations, the higher level of urbanization arouses more traffic activities and vehicle fleets that result in larger transport emissions; and transportation sector has a higher proportion in total economy-wide emission. As for the cities in developing areas, although the emission from transportation could also be considerable given the reliance on fossil fuels, it generally has a lower proportion in total emission.
Waste disposal and industrial process and fugitive emissions were smaller sources of GHG emissions by comparison. Although many studies recognized that waste is a minor contributor to the emissions in most of cities (Kennedy et al., 2009, 2014), it should be noted that technology advance in waste treatment and emission capture and reuse need further development toward carbon-neutral waste disposal and recycling systems (Dong et al., 2018).
Historical Emission Variations
Figure 3 reveals the annual change of total GHG emissions, and Figure 4 shows the annual change of per capita GHG emissions over the study period in 42 cities that had reliable emission data for at least 2 years (detailed information is shown in Table A1). Figure 3 shows that 30 cities reduced their annual GHG emissions, many of which are in America and Europe. Seattle (North America), Oslo (Europe), Bogotá (South America), and Houston (North America) are the top four cities with the largest per capita emission reduction (Bogotá is also the second largest city in terms of total reduction). European cities were heading for achieving their climate mitigation targets from a territorial perspective. From 2008 to 2016, the EU Emissions Trading System regulated ~50% of EU GHG emissions and reduced more than one billion tons of CO2 from 2008 to 2016 (Bayer and Aklin, 2020). Furthermore, the total GHG emissions and emissions from the power sector have been largely reduced in the USA in recent decades due to the changes from the use of coal to natural gas (Feng et al., 2015) and the transformation toward a cleaner and renewable energy system (Aslani and Wong, 2014; Ahn et al., 2021), where the climate actions have already significantly promoted GHG emission reduction. It should be noted that cities in the developed countries (e.g., American and European cities) had moved a certain amount of high-carbon industries to developing areas (mostly in Asian and Latin American cities) in the past few decades. The transfer of industrial activities across cities would possibly lead to an ineffective GHG emission reduction. The decrease of the local emissions in the developed cities may end up transferring the GHG emission to cities in the developing regions. Therefore, it has been acknowledged appropriate city boundaries and accounting methods for carbon inventory should be developed and applied to identify the climate responsibility for cities (Chen et al., 2020a,b).
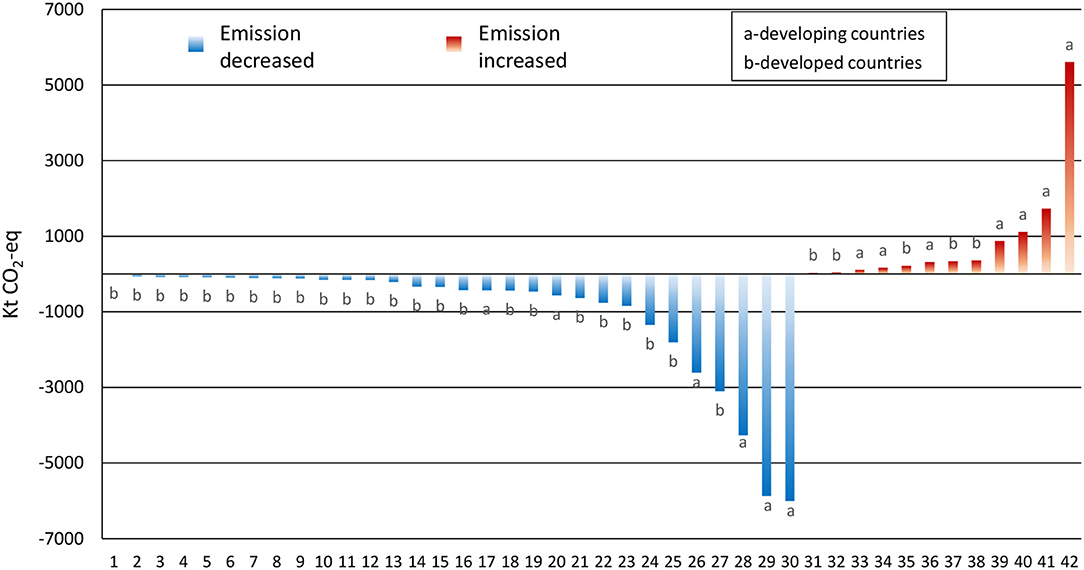
Figure 3. Annual change of GHG emission in cities over 2005–2016. 1, Yokohama; 2, Vancouver; 3, Stockholm; 4, Paris; 5, Sydney; 6, San Francisco; 7, Milan; 8, Barcelona; 9, Boston; 10, New Orleans; 11, Austin; 12, Washington, DC; 13, Copenhagen; 14, Athens; 15, Los Angeles; 16, Durban; 17, Toronto; 18, Chicago; 19, Chennai; 20, Philadelphia; 21, Oslo; 22, New York City; 23, Seoul; 24, Seattle; 25, Houston; 26, Amman; 27, London; 28, Istanbul; 29, Bogotá; 30, Bangkok; 31, Auckland; 32, Melbourne; 33, Cape Town; 34, Buenos Aires; 35, Montréal; 36, Ciudad de México; 37, Venice; 38, Madrid; 39, Lima; 40, Curitiba; 41, Johannesburg; 42, Rio de Janeiro.
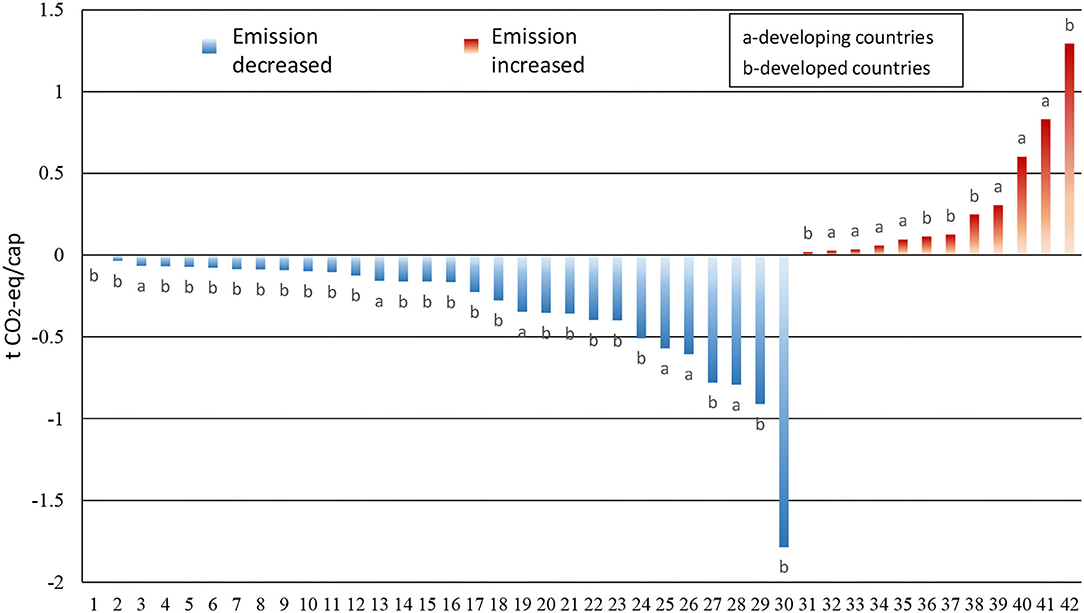
Figure 4. Annual change of per capita GHG emission in cities over 2005–2016. 1, Yokohama; 2, Paris; 3, Chennai; 4, Barcelona; 5, Milan; 6, Stockholm; 7, Los Angeles; 8, Seoul; 9, New York City; 10, Vancouver; 11, San Francisco; 12, Durban; 13, Toronto; 14, Chicago; 15, Austin; 16, Boston; 17, Washington, DC; 18, Istanbul; 19, London; 20, Copenhagen; 21, Philadelphia; 22, Sydney; 23, New Orleans; 24, Athens; 25, Bangkok; 26, Amman; 27, Houston; 28, Bogotá; 29, Oslo; 30, Seattle; 31, Auckland; 32, Cape Town; 33, Ciudad de México; 34, Buenos Aires; 35, Lima; 36, Madrid; 37, Montréal; 38, Melbourne; 39, Johannesburg; 40, Curitiba; 41, Rio de Janeiro; 42, Venice.
The GHG emissions continued to increase in several cities over the research period. Venice, Rio de Janeiro, Curitiba, and Johannesburg were the top four cities with the largest annual increases in per capita GHG emission, while Rio de Janeiro and Curitiba were also among cities with the largest annual total GHG emissions. Most of them are the cities in developing countries. Compared with the cities in developed areas, their industrial development mainly relies on industries with low technology and high energy consumption. For example, as the second largest industrial base of Brazil, Rio de Janeiro is under a fast development of chemical industry and mining industry. Some of these high-carbon industries are transferred from cities in developed countries (Cai et al., 2018). These cities have a great potential of emission reduction by making more aggressive climate mitigation plans that are anchored upon local sustainable development goals (Octaviano et al., 2016; Busch et al., 2019; Roe et al., 2019).
Varying Reduction Targets
Of the 167 analyzed cities, 113 already set traceable targets for GHG emission reduction, which included absolute emission reduction targets for 68 cities, intensity targets for 40 cities, and baseline scenario targets for 8 cities. For some cities, more than one kind of target exists. Most of these cities set targets on GHG emissions (CO2-eq) and some of them only referred to CO2 emissions. The cities showed great variance from 1990 to 2015, the emission targets ranged from 15 to 100% reduction. Here, we classified these targets into near-term (2020s), mid-term (2030s), and long-term (2040s−2050) goals of emission reduction (Figure 5).
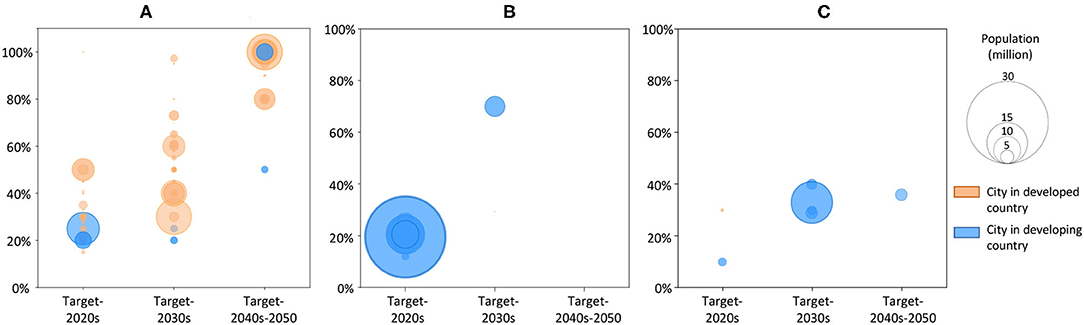
Figure 5. The (A) absolute emission reduction target, (B) intensity target, and (C) baseline scenario target proposed for GHGs emission mitigation in cities.
Absolute emission reduction targets (Figure 5A) were most widely reported in cities of developed nations. In general, these absolute targets are easier to trace over time and one can readily compare the near-term, mid-term, and long-term goals of the same city or between different cities. The commitments were concentrated in developed areas like Europe and North America. For instance, Toronto had announced to cut its GHG emissions by 80% of 1990 levels by 2050, with the near-term target of 30% reduction by 2020 and mid-term target of 65% target by 2030. European cities like Milan, Bologna, Warsaw had committed to achieve 20% of GHG emission reduction compared to 2005 levels by 2020, in alignment with the overall targets of the EU. There are cities that strive for even more ambitious goals (e.g., Porto adopted the goal of 45% reduction in community-wide GHG emissions compared to 2004 level by 2020 and Manchester has committed to be net-zero carbon by 2038). In comparison, cities tend to propose their climate targets through reduction of intensity (Figure 5B) or a simulated business-as-usual scenario (Figure 5C) if they are at the stage of rapid economic growth and accelerated industrialization (C40 Cities ARUP, 2014). For example, many Chinese cities have proposed clear intensity targets, following the national targets. The city-level goals were to reduce per unit GDP CO2 emissions by 12–25% by 2020 compared with 2015 according to the “13th Five-Year-Plan,” although the latest 14th Five-Year-Plan for 2020–2025 set a national target of 18% reduction in carbon intensity. The targets for the cities with more developed economy and higher urbanization rate are more ambitious (e.g., 25% of carbon intensity declined for Hangzhou and 23% for Guangzhou and Shenzhen), while the goals are generally more conservative for cities that are still at a fast-developing stage (e.g., the intensity targets for Urumqi and Haikou were 12 and 14%, respectively). Absolute targets are developed for Chinese cities in the “14th Five-Year-Plan,” and some cities (e.g., Guangzhou, Shenzhen, Suzhou, Jinan, and Qingdao) have pledged to achieve carbon peak before 2025. These targets are consistent with China's commitments to mid-term goals (60–65% reduction in CO2 emissions per unit of GDP by 2030 compared with 2005). The Chinese government had promised that the carbon intensity would be reduced by 40–45% during the periods of 2005–2020. In fact, by the end of 2019, China had reduced its carbon intensity by ~48.1% compared with 2005, which already exceeded their commitment.
More than 30 countries have announced their commitments of carbon neutrality and almost 100 countries that have net-zero-emission targets under discussion (Net Zero Tracker, 2021), which may inspire other regions to strengthen their own climate goals. An increasing number of cities have also proposed carbon neutrality targets. In total, 40 (24%) of the 167 cities in this study have set carbon neutrality (or net-zero-carbon, climate neutrality) goal, which may account for over 80% of their current GHG reduction. Most European cities strive to achieve carbon neutrality by 2050 (36 cities; i.e., 90%) to synchronize with the EU's goal as a whole; and four European cities even set these goals by 2020s−2030s. Similarly, most American cities have set targets to reduce GHG emissions by 80% by 2050. Copenhagen (Denmark) aims to be the first carbon-neutral capital in 2025. However, there is still a gap between the current climate policies in cities and the global climate goal. Studies have shown that 60% of EU Covenant of Mayors' cities are able to meet their 2020 carbon reduction targets (Hsu et al., 2020), which is 20% of CO2 reduction between 1990 and 2020 (Kona et al., 2018); however, overall, they must double their efforts to comply with the Paris Agreement of net-zero emissions around 2050 (Salvia et al., 2021). To address this gap, megacities in China have proposed carbon peak and carbon neutrality targets ahead of the nation. As the most populated urban agglomerations and the frontier of low-carbon economic transition in mainland China, Guangdong-Hong Kong-Macao Greater Bay Area (consists of nine cities in Guangdong Province, Hong Kong. and Macao) is expected to peak their emissions by 2025 and become carbon neutrality by 2050, which is 5 years and a decade ahead of the national goals, respectively.
Discussion
To limit the global warming to 1.5°C in this century, cities need to transform from a resource-dependent industrialization path to an innovation-driven sustainable development path. Moreover, policies should be formulated to facilitate this transformation and upgrade of traditional industries, the development of cleaner supply chains, and the formulation of low-carbon lifestyles. On the basis of these results, we proposed several policy recommendations to further advance future climate actions in cities.
More Effective Mitigation Strategies Target Key Sectors
Stationary energy and on-road transportation are the most significant contributors to GHG emissions of global cities. Among all the stationary energy users, residential, and institutional buildings play important roles in emission of global cities. Measures such as promoting energy audits on building energy use (Kontokosta et al., 2020), adjusting building structures (Li et al., 2019b), and controlling and decreasing unnecessary construction activities (Zhang et al., 2019) should be considered when striving for the net-zero emission of buildings.
Cities with large size of populations, fast-developing transportation infrastructures, and a high level of travel demand tend to have larger shares of transportation-related emissions. For example, on-road transportation accounted for 44 and 42% of total GHG emissions in Bangkok and Seoul, respectively. These results are consistent with previous studies, which suggested that the GHG emission of transportation is closely relevant to the size of population and economy (Lakshmanan and Han, 1997; Timilsina and Shrestha, 2009; Ehrlich, 2017; Liang et al., 2017). Population growth during urbanization increases travel activities and vehicle fleets, although the growing population density could end up with lower transport emission per capita. To effectively control the transportation emissions, policy makers can use various policy tools. For example, in South Korea's cities, the governors proposed that the public sectors should buy green cars and there should be rewards and subsidies for using public buses and taxis (CSTNET, 2021). Moreover, fossil fuels for transport should be replaced by cleaner energy (e.g., hydrogen, and biofuels) (Axsen et al., 2020), and a higher leverl of electrification in both public and private transportation should promoted (Safarzyńska and van den Bergh, 2018; Xylia et al., 2019). In addition, the on-road emission is significantly higher than those from other types of traffic (e.g., railway, aviation, and shipping) from a global perspective. The on-road emission standards should be improved and updated to minimize the impact of existing gasoline-based and diesel-based vehicles (Jacobsen and Benthem, 2013; Thiel et al., 2016). Besides, a green traffic mode should be also formed in communities. People travel with bicycle and public transportation should be encouraged through incentive economic measures (e.g., a carbon currency system that rewards low-carbon transport choices). The rail transit should be also invested to appropriately relieve the travel pressure of ground transportation in cities of high population density.
Additionally, waste management and recycling for disposal should not be ignored. As shown in our results, for some developed cities such as Lima and Geneva, the contribution of waste disposal to total emission were relatively high. Their total GHG emissions were lower than most cities but their waste disposal proportions reached 44.5 and 18.5%, respectively. Strengthening the reuse of waste as new products in a low-carbon way is highly important (such as the reuse of biomass for energy generation) (Tripathi et al., 2019). Properly managing the solid waste can help realize the maximization of resource utilization and the “zero emission” of waste, which are significant for building a circular economy.
Timely and Consistent Evaluation of Mitigation Progress
To trace the effectiveness of carbon reduction policies in urban areas, it is necessary to compile accurate and time-series emission inventories through a consistent methodology. Currently, despite many efforts exist in establishing carbon accounts of individual cities, high-quality dynamic GHG emission database of cities is still lacking at a global scale. Most cities do not have comparable time-series GHG emission inventories at a detailed sector level. Some American and EU cities developed relatively comprehensive GHG inventories, while other cities only had emission data for a few discrete years or did not report emission from some sectors. Several GHG emission databases have been developed in developing nations such as China. Carbon Emission Accounts and Datasets (CEAD) (https://www.ceads.net.cn/) reports GHG emission accounts and dataset for Chinese provincial regions and cities in different years. Moreover, the China High Resolution Emission Gridded Database (CHRED) (http://www.cityghg.com/) has established a GHG database for China, including a high-resolution CO2 emissions dataset for Chinese cities (Cai et al., 2017). The global society and world organizations should help the cities in developing cities set up traceable GHG emission data and foster better collaboration of database development among major cities. It is necessary to build transparent and available carbon data disclosure systems so that the carbon mitigation processes can be consistently tracked. Also, the consumption-based and infrastructure-based carbon accounts have been increasingly adopted in determining climate impact and mitigation responsibilities of cities (Chen and Chen, 2017; Ramaswami et al., 2021). Development of global databases that support the consumption-based and infrastructure-based accounting should be also in the agenda. Improving the reporting scheme and data quality of GHG emission would be better for the evaluation of carbon mitigation processes. This places higher demands on basic data collection, both in terms of data volume and the level of accuracy.
More Ambitious and Easily-Traceable Mitigation Goals
Although many countries have announced ambitious climate commitments, climate targets seem less clear and available for many cities. In our study, only around 60% of cities have traceable climate targets (e.g., absolute emission reduction targets), which is insufficient. Cities in most developed countries have set clear long-term climate goals such as carbon neutrality by 2050. In comparison, a large number of cities in developing regions prefer intensity targets and baseline scenario targets that are usually short-term or mid-term based, which is not entirely compatible with the global climate goals. At certain stage, carbon intensity is a useful indicator showing the decarbonization of economy and provides better flexibilities for cities of fast economic growth and increase in emission. But in the long run, switching from intensity targets to absolute targets in cities is essential to achieve the global carbon neutrality by 2050. In fact, some Chinese cities have already presented their timetables for carbon peak and carbon neutrality in their 14th Five-Year Plans. For example, Guangzhou and Shenzhen aim to peak their GHG emission first in 2020, while other cities (such as Zhuhai and Huizhou) aim for around 2025, and finally achieve overall carbon neutrality by 2050. Beijing, Shanghai, cities in Jiangsu Province have also committed to achieve carbon peak no later than the national goal.
In addition, specific targets that are decomposed into various sectors (e.g., specific targets for energy consumption, transportation, and waste) are needed to mitigate the emission gaps. For example, Copenhagen plans to achieve 20% reduction in heat consumption in 2025 compared with 2010 and net-zero carbon emissions in public transport by 2025 (CPH, 2012). A wide cooperation among governments, business communities, research institutions, and social organizations are required to provide synergistic technological solutions toward achieving emission mitigation targets for sectors. The climate targets for different sectors and industries should be designed with a timely and easily-traceable way, and facilitate a transparent sector-level comparison among cities (Levin and Fransen, 2019).
Limitations
Some limitations and uncertainties should be noted. First, the definitions of “unban unit” are diverse and sometimes inconsistent from cities to cities, which makes it difficult to assess cities within the same geographical scale (inclusion of urban areas) and organization status (forms of local administration), given the current data. This may introduce uncertainties to the carbon accounts and budgets. For example, some of the climate mitigation measures are expected to take place in suburban areas or higher level of metropolis (such as the carbon reduction of intercity transportation and the waste disposal outside the city center). Second, the population data are collected to match with the carbon data, and could be subject to some extents of inconsistency. However, by cross-checking the scales of carbon data and population data of cities, the main findings on per capita carbon results are generally reliable and comparable within a broad view. Future steps are suggested to improve the consistency of GHG emission inventories of cities at a global scale and track the dynamics of urban emission changes over a longer term based on a unified accounting standard.
Data Availability Statement
The original contributions presented in the study are included in the article/supplementary material, further inquiries can be directed to the corresponding author.
Author Contributions
TW and JW: visualization, methodology, writing-original draft, and editing. SC: conceptualization, review, and editing. All authors contributed to the article and approved the submitted version.
Funding
This study was supported by Natural Science Funds for Distinguished Young Scholar of Guangdong Province, China (2018B030306032), National Natural Science Foundation of China (72074232, 71704015), and the Fundamental Research Funds for the Central Universities (19lgzd26).
Conflict of Interest
The authors declare that the research was conducted in the absence of any commercial or financial relationships that could be construed as a potential conflict of interest.
References
Ahn, K., Chu, Z., and Lee, D. (2021). Effects of renewable energy use in the energy mix on social welfare. Energy Econ. 96, 105174. doi: 10.1016/j.eneco.2021.105174
Aslani, A., and Wong, K. V. (2014). Analysis of renewable energy development to power generation in the United States. Renew. Energy 63, 153–161. doi: 10.1016/j.renene.2013.08.047
Axsen, J., Plötz, P., and Wolinetz, M. (2020). Crafting strong, integrated policy mixes for deep CO2 mitigation in road transport. Nat. Clim. Chang. 10, 809–818. doi: 10.1038/s41558-020-0877-y
Bayer, P., and Aklin, M. (2020). The European Union Emissions Trading System reduced CO2 emissions despite low prices. Proc. Natl. Acad. Sci. U.S.A. 117, 8804–8812. doi: 10.1073/pnas.1918128117
Busch, J., Engelmann, J., Cook-Patton, S. C., Griscom, B. W., Kroeger, T., Possingham, H., et al. (2019). Potential for low-cost carbon dioxide removal through tropical reforestation. Nat. Clim. Chang. 9, 463–466. doi: 10.1038/s41558-019-0485-x
C40 Cities ARUP (2014). Global Aggregation of City Climate Commitments: Methodological Review (Version 2.0 Final White Paper). Available online at: https://www.c40.org/researches/global-aggregation-of-city-climate-commitments-methodology (accessed April 20, 2021).
Cai, B., Liang, S., Zhou, J., Wang, J., Cao, L., Qu, S., et al. (2017). China high resolution emission database (CHRED) with point emission sources, gridded emission data, and supplementary socioeconomic data. Resour. Conserv. Recycl.. 129, 232–239. doi: 10.1016/j.resconrec.2017.10.036
Cai, X., Che, X., Zhu, B., Zhao, J., and Xie, R. (2018). Will developing countries become pollution havens for developed countries? An empirical investigation in the Belt and Road. J. Clean. Prod. 198, 624–632. doi: 10.1016/j.jclepro.2018.06.291
Chen, S. Q., and Chen, B. (2012). Network environ perspective for urban metabolism and carbon emissions: a case study of Vienna, Austria. Environ. Sci. Technol. 46, 4498–4506. doi: 10.1021/es204662k
Chen, S. Q., and Chen, B. (2017). Changing urban carbon metabolism over time: historical trajectory and future pathway. Environ. Sci. Technol. 51, 7560–7571. doi: 10.1021/acs.est.7b01694
Chen, S. Q., Chen, B., Feng, K., Liu, Z., Former, N., Tan, X., et al. (2020a). Physical and virtual carbon metabolism of global cities. Nat. Commun. 11:182. doi: 10.1038/s41467-019-13757-3
Chen, S. Q., Long, H., Chen, B., Feng, K., and Hubacek, K. (2020b). Urban carbon footprints across scale: important considerations for choosing system boundaries. Appl. Energy 259:114201. doi: 10.1016/j.apenergy.2019.114201
Chester, M. V., and Cano, A. (2016). Time-based life-cycle assessment for environmental policymaking: greenhouse gas reduction goals and public transit. Transp. Res. D Transp. Environ. 43, 49–58. doi: 10.1016/j.trd.2015.12.003
CPH (2012). CPH 2025 Climate Plan: A Green, Smart and Carbon Neutral City. Copenhagen Carbon Neutral by 2025. Available online at: https://kk.sites.itera.dk/apps/kk_pub2/pdf/983_jkP0ekKMyD.pdf (accessed June 26, 2021).
CSTNET (2021). South Korea Plans to Promote 7.85 Million Environmentally Friendly Vehicles by 2030. China Science and Technology Network. Available online at: http://www.stdaily.com/kjrb/kjrbbm/2021-03/03/content_1085172.shtml (accessed June 12, 2021).
Dodman, D. (2009). Blaming cities for climate change? An analysis of urban greenhouse gas emissions inventories. Environ. Urban. 21, 185–201. doi: 10.1177/0956247809103016
Dong, H., Geng, Y., Yu, X., and Li, J. (2018). Uncovering energy saving and carbon reduction potential from recycling wastes: a case of Shanghai in China. J. Clean. Prod. 205, 27–35. doi: 10.1016/j.jclepro.2018.08.343
Ehrlich, J. (2017). Infrastructure requirements for the self-driving vehicle. Routes Roads 43–46. https://trid.trb.org/view/1487822 (accessed June 25, 2021).
Feng, K., Davis, S. J., Sun, L., and Hubacek, K. (2015). Drivers of the US CO2 emissions 1997–2013. Nat. Commun. 6:7714. doi: 10.1038/ncomms8714
Georgeson, L., Maslin, M., Poessinouw, M., and Howard, S. (2016). Adaptation responses to climate change differ between global megacities. Nat. Clim. Chang. 6, 584–588. doi: 10.1038/nclimate2944
GPC (2014). Global Protocol for Community-Scale Greenhouse Gas Emission Inventories: An Accounting and Reporting Standard for Cities. Greenhouse Gas Protocol.
Hsu, A., Tan, J., Ng, Y. M., Toh, W., Vanda, R., and Goyal, N. (2020). Performance determinants show European cities are delivering on climate mitigation. Nat. Clim. Chang. 10, 1015–1022. doi: 10.1038/s41558-020-0879-9
Ibrahim, N., Sugar, L., Hoornweg, D., and Kennedy, C. (2012). Greenhouse gas emissions from cities: comparison of international inventory frameworks. Local Environ. 17, 223–241. doi: 10.1080/13549839.2012.660909
IEA (2012). World Energy Outlook 2012. Paris: International Energy Agency. Available online at: https://www.iea.org/reports/world-energy-outlook-2012 (accessed April 10, 2021).
IIASA (2012). GEA 2012: Global Energy Assessment – Toward a Sustainable Future. Cambridge University Press. Available online at: http://assets.cambridge.org/97811070/05198/frontmatter/9781107005198_frontmatter.pdf (accessed June 25, 2021).
Jacobsen, M., and Benthem, A. (2013). Vehicle scrappage and gasoline policy. Am. Econ. Rev. 105, 1312–1338. doi: 10.1257/aer.20130935
Kanemoto, K., Shigetomi, Y., Hoang, N. T., Okuoka, K., and Moran, D. (2020). Spatial variation in household consumption-based carbon emission inventories for 1,200 Japanese cities. Environ. Res. Lett. 15:114053. doi: 10.1088/1748-9326/abc045
Kennedy, C., Ibrahim, N., and Hoornweg, D. (2014). Low-carbon infrastructure strategies for cities. Nat. Clim. Chang. 4, 343–346. doi: 10.1038/nclimate2160
Kennedy, C., Steinberger, J., Gasson, B., Hansen, Y., Hillman, T., Havránek, M., et al. (2009). Greenhouse gas emissions from global cities. Environ. Sci. Technol. 43, 7297–7302. doi: 10.1021/es900213p
Kennedy, C., Steinberger, J., Gasson, B., Hansen, Y., Hillman, T., Havránek, M., et al. (2010). Methodology for inventorying greenhouse gas emissions from global cities. Energy Policy 37, 4828–4827. doi: 10.1016/j.enpol.2009.08.050
Kona, A., Bertoldi, P., Monforti-Ferrario, F., Rivas, S., and Dallemand, J. F. (2018). Covenant of mayors signatories leading the way towards 1.5 degree global warming pathway. Sust. Cities Soc. 41, 568–575. doi: 10.1016/j.scs.2018.05.017
Kontokosta, C. E., Spiegel-Feld, D., and Papadopoulos, S. (2020). The impact of mandatory energy audits on building energy use. Nat. Energy 5, 309–316. doi: 10.1038/s41560-020-0589-6
Lakshmanan, T. R., and Han, X. (1997). Factors underlying transportation CO2 emissions in the U.S.A.: A decomposition analysis. Transp. Res. Part D: Transp. Environ. 2, 1–15. doi: 10.1016/S1361-9209(96)00011-9
Levin, K., and Fransen, T. (2019). Commentary: Climate Action for Today and Tomorrow: The Relationship between NDCs and LTSs. World Resources Institute. Available online at: https://www.wri.org/news/climate-action-today-and-tomorrow-relationship-between-ndcs-and-ltss (accessed April 13, 2021).
Li, F., Cai, B., Ye, Z., Wang, Z., Zhang, W., Zhou, P., et al. (2019a). Changing patterns and determinants of transportation carbon emissions in Chinese cities. Energy 174, 562–575. doi: 10.1016/j.energy.2019.02.179
Li, X., Yao, R., Yu, W., Meng, X., Liu, M., Short, A., et al. (2019b). Low carbon heating and cooling of residential buildings in cities in the hot summer and cold winter zone - a bottom-up engineering stock modeling approach. J. Clean. Prod. 220, 271–288. doi: 10.1016/j.jclepro.2019.02.023
Liang, Y., Niu, D., Wang, H., and Li, Y. (2017). Factors affecting transportation sector CO2 emissions growth in China: An LMDI decomposition analysis. Sustainability 9, 1730. doi: 10.3390/su9101730
Liobikiene, G., and Butkus, M. (2017). The European Union possibilities to achieve targets of Europe 2020 and Paris agreement climate policy. Renew. Energy 106, 298–309. doi: 10.1016/j.renene.2017.01.036
Moran, D., Kanemoto, K., Jiborn, M., Wood, R., Többen, J., and Seto, K. C. (2018). Carbon footprints of 13000 cities. Environ. Res. Lett. 13:064041. doi: 10.1088/1748-9326/aac72a
Morgan, J., and Waskow, D. (2014). A new look at climate equity in the UNFCCC. Clim. Pol. 14, 17–22. doi: 10.1080/14693062.2014.848096
Nangini, C., Peregon, A., Ciais, P., Weddige, U., Vogel, F., Wang, J., et al. (2019). A global dataset of CO2 emissions and ancillary data related to emissions for 343 cities. Sci. Data 6:180280. doi: 10.1038/sdata.2018.280
Net Zero Tracker. (2021). Available at: https://eciu.net/netzerotracker (accessed June 13, 2021).
Octaviano, C., Paltsev, S., and Gurgel, A. C. (2016). Climate change policy in Brazil and Mexico: results from the MIT EPPA model. Energy Econ. 56, 600–614. doi: 10.1016/j.eneco.2015.04.007
Ramaswami, A., Tong, K., Canadell, J. G., Jackson, R. B., Stokes, E., Dhakal, S., et al. (2021). Carbon analytics for net-zero emissions sustainable cities. Nat. Sustain. 4, 460–463. doi: 10.1038/s41893-021-00715-5
Reckien, D., Salvia, M., Heidrich, O., Church, J. M., Pietrapertosa, F., et al. (2018). How are cities planning to respond to climate change? Assessment of local climate plans from 885 cities in the EU-28. J. Clean. Prod. 191, 207–219. doi: 10.1016/j.jclepro.2018.03.220
Roe, S., Streck, C., Obersteiner, M., Frank, S., Griscom, B., Drouet, L., et al. (2019). Contribution of the land sector to a 1.5 °C world. Nat. Clim. Chang. 9, 817–828. doi: 10.1038/s41558-019-0591-9
Rogelj, J., Huppmann, D., Krey, V., Riahi, K., Clarke, L., Gidden, M., et al. (2019). A new scenario logic for the Paris Agreement long-term temperature goal. Nature 573, 357–363. doi: 10.1038/s41586-019-1541-4
Safarzyńska, K., and van den Bergh, J. (2018). A higher rebound effect under bounded rationality: interactions between car mobility and electricity generation. Energy Econ. 74, 179–196. doi: 10.1016/j.eneco.2018.06.006
Salvia, M., Reckien, D., Pietrapertosa, F., Eckersley, P., Spyridaki, N., Krook-Riekkola, A., et al. (2021). Will climate mitigation ambitions lead to carbon neutrality? An analysis of the local-level plans of 327 cities in the EU. Renew. Sustain. Energy Rev. 135:110253. doi: 10.1016/j.rser.2020.110253
Shan, Y., Fang, S., Cai, B., Zhou, Y., Li, D., Feng, K., et al. (2021). Chinese cities exhibit varying degrees of decoupling of economic growth and CO2 emissions between 2005 and 2015. One Earth 4, 124–134. doi: 10.1016/j.oneear.2020.12.004
Shan, Y., Guan, D., Hubacek, K., Zheng, B., Davis, S. J., Jia, L., et al. (2018). City-level climate change mitigation in China. Sci. Adv. 4:eaaq0390. doi: 10.1126/sciadv.aaq0390
Shan, Y., Guan, D., Liu, J., Mi, Z., Liu, Z., Liu, J., et al. (2017). Methodology and applications of city level CO2 emission accounts in China. J. Clean. Prod. 161, 1215–1225. doi: 10.1016/j.jclepro.2017.06.075
Sobrinho, V., Lagutov, V., and Baran, S. (2020). Green with savvy? Brazil's climate pledge to the Paris Agreement and its transition to the green economy. Energy Clim. Chang. 1:100015. doi: 10.1016/j.egycc.2020.100015
Sovacool, B. K., and Brown, M. A. (2010). Twelve metropolitan carbon footprints: a preliminary comparative global assessment. Energy Pol. 38, 4856–4869. doi: 10.1016/j.enpol.2009.10.001
Sun, L., Liu, W., Li, Z., Cai, B., Fujii, M., Luo, X., et al. (2021). Spatial and structural characteristics of CO2 emissions in East Asian megacities and its indication for low-carbon city development. Appl. Energy 284:116400. doi: 10.1016/j.apenergy.2020.116400
Sun, Y., Zhang, X., Ren, G., Zwiers, F. W., and Hu, T. (2016). Contribution of urbanization to warming in China. Nat. Clim. Chang. 6, 706–709. doi: 10.1038/nclimate2956
Thiel, C., Nijs, W., Simoes, S., Schmidt, J., van Zyl, A., and Schmid, E. (2016). The impact of the EU car CO2 regulation on the energy system and the role of electro-mobility to achieve transport decarbonisation. Energy Policy 96, 153–166. doi: 10.1016/j.enpol.2016.05.043
Timilsina, G. R., and Shrestha, A. (2009). Transport sector CO2 emissions growth in Asia: Underlying factors and policy options. Energy Policy 37, 4523–4539. doi: 10.1016/j.enpol.2009.06.009
Tripathi, N., Hills, C. D., Singh, R. S., and Atkinson, C. J. (2019). Biomass waste utilisation in low-carbon products: harnessing a major potential resource. NPJ Clim. Atmos. Sci 2:35. doi: 10.1038/s41612-019-0093-5
UNFCCC (2015). Paris Agreement 2015. United Nations Conference on Climate Change. Available online at: https://unfccc.int/process-and-meetings/the-paris-agreement/the-paris-agreement (accessed April 10, 2021).
Wiedmann, T., Chen, G., Owen, A., Lenzen, M., Doust, M., Barrett, J., et al. (2020). Three-scope carbon emission inventories of global cities. J. Indus. Ecol. 25, 735–750. doi: 10.1111/jiec.13063
Xu, X., Huo, H., Liu, J., Shan, Y., Li, Y., Zheng, H., et al. (2018). Patterns of CO2 emissions in 18 central Chinese cities from 2000 to 2014. J. Clean. Prod. 172, 529–540. doi: 10.1016/j.jclepro.2017.10.136
Xylia, M., Leduc, S., Laurent, A.-B., Patrizio, P., van der Meer, Y., Kraxner, F., et al. (2019). Impact of bus electrification on carbon emissions: the case of Stockholm. J. Clean. Prod. 209, 74–87. doi: 10.1016/j.jclepro.2018.10.085
Zhang, Y., Yan, D., Hu, S., and Guo, S. (2019). Modelling of energy consumption and carbon emission from the building construction sector in China, a process-based LCA approach. Energy Pol. 134:110949. doi: 10.1016/j.enpol.2019.110949
Zhou, S., Tong, Q., Pan, X., Cao, M., Wang, H., Gao, J., et al. (2021). Research on low-carbon energy transformation of China necessary to achieve the Paris agreement goals: a global perspective. Energy Econ. 95:105137. doi: 10.1016/j.eneco.2021.105137
Appendix A
Keywords: climate change, GHG emission reduction, energy consumption, climate targets, low-carbon cities
Citation: Wei T, Wu J and Chen S (2021) Keeping Track of Greenhouse Gas Emission Reduction Progress and Targets in 167 Cities Worldwide. Front. Sustain. Cities 3:696381. doi: 10.3389/frsc.2021.696381
Received: 16 April 2021; Accepted: 15 June 2021;
Published: 12 July 2021.
Edited by:
Edgar Liu, University of New South Wales, AustraliaReviewed by:
Neil Simcock, Liverpool John Moores University, United KingdomXochitl Cruz-Núñez, National Autonomous University of Mexico, Mexico
Copyright © 2021 Wei, Wu and Chen. This is an open-access article distributed under the terms of the Creative Commons Attribution License (CC BY). The use, distribution or reproduction in other forums is permitted, provided the original author(s) and the copyright owner(s) are credited and that the original publication in this journal is cited, in accordance with accepted academic practice. No use, distribution or reproduction is permitted which does not comply with these terms.
*Correspondence: Shaoqing Chen, chenshaoqing@mail.sysu.edu.cn
†These authors have contributed equally to this work