- 1Design Interactive, Inc., Orlando, FL, United States
- 2Lockheed Martin Corporate, Washington, DC, United States
The aim of this study was to assess what drives gender-based differences in the experience of cybersickness within virtual environments. In general, those who have studied cybersickness (i.e., motion sickness associated with virtual reality [VR] exposure), oftentimes report that females are more susceptible than males. As there are many individual factors that could contribute to gender differences, understanding the biggest drivers could help point to solutions. Two experiments were conducted in which males and females were exposed for 20 min to a virtual rollercoaster. In the first experiment, individual factors that may contribute to cybersickness were assessed via self-report, body measurements, and surveys. Cybersickness was measured via the simulator sickness questionnaire and physiological sensor data. Interpupillary distance (IPD) non-fit was found to be the primary driver of gender differences in cybersickness, with motion sickness susceptibility identified as a secondary driver. Females whose IPD could not be properly fit to the VR headset and had a high motion sickness history suffered the most cybersickness and did not fully recover within 1 h post exposure. A follow-on experiment demonstrated that when females could properly fit their IPD to the VR headset, they experienced cybersickness in a manner similar to males, with high cybersickness immediately upon cessation of VR exposure but recovery within 1 h post exposure. Taken together, the results suggest that gender differences in cybersickness may be largely contingent on whether or not the VR display can be fit to the IPD of the user; with a substantially greater proportion of females unable to achieve a good fit. VR displays may need to be redesigned to have a wider IPD adjustable range in order to reduce cybersickness rates, especially among females.
Introduction
In general, those who have studied cybersickness (i.e., the motion sickness associated with VR exposure) and other forms of motion sickness oftentimes report that females are more susceptible than males. Cooper et al. (at sea; 1997), Kaplan (on trains; 1964), Lawther and Griffin (at sea; 1986; sea, 1988), Lederer and Kidera (on planes; 1954), Lentz and Collins (general susceptibility; 1977), Munafo et al. (in VR; 2017); Park and Hu (in a rotating drum; 1999), Stanney et al. (in VR; 2003); Turner and Griffin (in automobiles; 1999), and Turner et al. (on planes; 2000) all found females more susceptible to motion sickness as compared to males across diverse motion platforms. Yet when Lawson (2014) reviewed 46 studies examining gender differences in motion sickness, he reported that only 26/46 (56.5%) found higher levels of susceptibility in females as compared to males. Further, in immersive environments, there are many individual factors that could contribute to gender differences, including previous experience with virtual motion, field of view (FOV), IPD, field dependence, postural stability, female hormonal cycle, state/trait anxiety, migraine susceptibility, ethnicity, aerobic fitness, body mass index, among others (see Tables 1, 2). Researchers have yet to identify which of these factors are the primary drivers of susceptibility differences between the genders. Of the studies that do exist, generally only a few variables were considered at one time rather than examining across a large number of potential drivers (c.f. Parkman et al., 1996; Stanney et al., 2003; Klosterhalfen et al., 2005). In addition, gender differences in susceptibility have been speculated to be attributed to differences in symptom awareness and willingness to report symptomatology. However, past studies have shown a 5:3 female to male risk ratio for vomiting, which is an objective measure of motion sickness (Lawther and Griffin, 1986). Examining differences from a physiological level can address any such reporting differences. Yet, even from a physiological level conflicting data exist. While females have been shown to have higher emetic response rates (Kennedy et al., 1995; Golding, 2006), as well as greater sensitivity in peripheral alpha- and beta- adrenergic receptors (Girdler et al., 1990; Kajantie and Phillips, 2006), which increases autonomic responses associated with motion sickness (Finley et al., 2004), Jokerst et al. (1999) found no significant differences between the genders in gastric tachyarrhythmia during exposure to an optokinetic drum, and Cheung and Hofer (2002) found no significant gender-based physiological differences during coriolis cross-coupling stimulation. Thus, while females are generally thought to have higher susceptibility to cybersickness than males, this relationship has not been well-characterized, especially for the latest generation of VR headsets.
Why do gender differences matter? VR technology is anticipated to fill many enterprise roles in the coming decades, from training to maintenance to operational support to design, and more. Currently >150 companies in multiple industries, including >50 Fortune 500 companies, are testing and/or deploying VR solutions (Kaiser and Schatsky, 2017; Morris, 2018). As VR-based real-time guidance systems driven by artificial intelligence advance, persons who cannot tolerate these delivery systems may be left out of job advancement. We cannot create a divide, with those who can handle VR exposure advancing due to better, more immersive training, more effective repair jobs aided by real-time augmented guidance, more creative designs that evolve from a mesh of digital and physical worlds, etc., while those who are susceptible to cybersickness are left on the sidelines watching this new era of VR empowered productivity pass them by. Further, if the design of VR headsets is discriminative to females, they, in particular, may experience challenges when trying to harness the bevy of performance enhancing potential of VR enterprise applications.
The main goal of this study was to determine what the primary drivers of gender-based differences in cybersickness susceptibility within VR environments are so that potential countermeasures to better accommodate females can be identified. To this end, two experiments were conducted. The first study examined potential drivers of cybersickness, which are summarized in Table 1, to identify those that may be contributing the most to gender differences. It was anticipated that a subset of these factors would be identified as particularly influential in driving higher levels of cybersickness among females.
Experiment 1
Materials and Methods
The purpose of Experiment 1 was to determine how well males and females are able to tolerate VR exposure and what factors might be driving any differences in the cybersickness they may experience. Based on the studies summarized in Table 1, it was anticipated that females would experience higher levels of cybersickness than males, with the goal of the experiment being to identify which factors drive any such differences.
Participants
Adults aged 18–30 years, balanced between genders participated in this study. Participants were recruited through a market research firm. A total of 46 participants participated in the study and were randomized to either an experimental group (VR headset; n = 30 [15 male/15 female]) or a control condition (flatscreen television; n = 16 [8 male/8 female]). This research complied with the American Psychological Association Code of Ethics and was approved by the Institutional Review Board at Copernicus Group. Informed consent was obtained from each participant and all participants were compensated for their time in the experiment.
Equipment and Display Content
The displays used in this study included the HTC Vive VR headset (which does not fit, on average, ~35% of females and ~16% of males based on the adjustable IPD range) and a flatscreen television. The HTC Vive has OLED display technology, a resolution of 2,160 × 1,200 (1,080 × 1,200 per eye), a refresh rate of 90 Hz, a field of view of 110 degrees, weight of 555 g (1.22 lbs), and an IPD range adjustable from 60.5 to 74.4 mm. The flatscreen television was a Samsung H6350 Smart LED TV with a screen size of 60.0′′ measured diagonally and a resolution of 1,920 × 1,080.
Steam platform was used to develop a virtual rollercoaster of 20 min duration (see Figure 1). In order to create provocative content that would instigate cybersickness, the following factors were incorporated into the virtual rollercoaster ride:
• Off-vertical axis rotation (visual OVAR; e.g., rollercoaster wraparounds, spinning track), as OVAR can be expected to lead to extreme levels of nauseogenicity (Golding et al., 2009);
• Variable velocity, forward acceleration, and vertical acceleration via humps in the track that provided visual oscillation, as these motions are known to be provocative (Alexander et al., 1947; Lawther and Griffin, 1986);
• High level of optic flow (implemented via movement through support structures, maintenance gangways, ground tunnels, and other visual details), which tends to drive visually induced motion sickness (Smart et al., 2014);
• Anchoring to the lead rollercoaster car with no car in front to focus on, as a fixed-horizon or stable vehicle dashboard reduces cybersickness (Prothero and Parker, 2003);
• Constant, rhythmic, and repetitive sound that simulated movement along the track so that participants were visually and aurally convinced they were moving when they were actually sitting still in a chair, as such sounds can drive nausea and disorientation (Dawson, 1982); and
• No control by the participant over virtual motion, as lack of viewpoint control has been demonstrated to be very nauseogenic (Stanney and Hash, 1998).
To maintain consistency in the visual stimulus across groups, the SteamVR format was exported to a video format to run in flatscreen television format.
Procedure
The experiment involved the following phases— pre-screening, screening, pre-testing, immersive exposure, and post-testing.
In the pre-screening phase, a participant recruiter called potential participants and reviewed inclusion requirements with them to identify candidate participants. Any participant reporting affirmative to any exclusion criteria (neurological impairments, musculoskeletal problems of the knee, ankle, shoulder, and/or elbow, loss in depth perception, <20/20 corrected visual acuity, inner-ear anomalies, history of seizures, pregnancy) was not asked to participate in the study. Participants who met pre-screening eligibility and inclusion requirements were scheduled for on-site screening. During the on-site screening: (1) upon arrival, participants were welcomed, and provided with informed consent documentation; (2) all participants were provided with a 3-digit number based on order of participation and experimental condition that was used for data collection; (3) a Simulator Sickness Questionnaire (SSQ; Kennedy et al., 1993) was administered electronically and participants that scored > 12 were thanked for their willingness to participate and excluded from the study; (4) a visual acuity test was administered and participants who did not have corrected 20/20 vision were thanked for their willingness to participate and excluded from the study; and (5) the Titmus Stereotest was administered to assess depth perception and participants that scored <6/9 were thanked for their willingness to participate and excluded from the study. Participants who met screening eligibility proceeded to pre-testing.
During the pre-testing phase, participants completed a demographics form via which they reported their previous VR and gaming experience, phase of the menstrual cycle (female only), and ethnicity, as well as other demographic data. Participants FOV (i.e., open observable area an individual can see through their eyes, which covers both central foveal and peripheral vision; Strasburger et al., 2011) was then measured via a vision protractor, their IPD (i.e., distance between the center of their pupils; Dodgson, 2004) was measured via a digital pupilometer (binocular pupillary range: 45–80 mm), their weight and height were measured to assess body mass index, their aerobic fitness (i.e., peak expiratory flow) was assessed via the Philips Respironics HS755 Personal Best Full Peak Flow Meter, and postural stability was assessed via the Sharpened Rhomberg Test (Johnson et al., 2005) using a Polhemus G4 wireless magnetic motion-tracking device with the sensor mounted via a naval strap. Participants then filled out surveys, including the State–Trait Anxiety Inventory (STAI; Spielberger et al., 1970), Motion History Questionnaire (MHQ; Kennedy et al., 1992), Cube Comparison Survey (Ekstrom et al., 1976), and Migraine Susceptibility Survey based on the International Headache Society [International Headache Society (IHS), 2017] Criteria for Diagnosing Migraine.
During the immersive exposure phase, participants were randomized to a control (i.e., flatscreen television) or experimental group (i.e., VR headset) and fitted with physiological sensors of electrocardiogram (ECG; to assess alterations to cardiovascular activity, i.e., heart rate), electrogastrography (EGG; to assess abnormal gastric rhythms, including tachygastria and bradygastria), and electrodermal activity (EDA; to assess skin conductance level [SCL]). Following a 5 min baselining of the physiological measures, participants were exposed to immersive content (virtual rollercoaster) for 20 min. The IPD of participants in the VR group was entered into the headset software and adjusted on the headset prior to viewing the rollercoaster stimuli to the best match available based on the IPD range of the HTC Vive. Those participants with an IPD smaller or larger than the HTC VIVE range were, respectively, given the value at the lowest or highest value available (60.5 or 74.4 mm). Participants were monitored via the physiological measures throughout VR exposure.
During the post-testing phase, the SSQ Total Score was assessed immediately following the immersive exposure (AE [aftereffects] 1), and in 15 min increments for a total of 60 min (AE2–AE5) post exposure. Participants were then debriefed, thanked, and paid for participation.
Experimental Design
The experiment was a mixed design, with 2 (gender) × 2 (display type) between factors and a 5 (post exposure measurement time) within factor. The display types were VR headset and flatscreen television and gender types were male and female. The post exposure measurement times were 0, 15, 30, 45, and 60 min.
Predictor Variables
The cybersickness predictor variables (see Table 3) included self-report measures (previous VR and gaming experience, female hormonal cycle, ethnicity—all gathered via demographics form), survey assessed measures (field dependence, state/trait anxiety, migraine susceptibility, past motion sickness history), and body measures (FOV, IPD, postural stability, aerobic fitness, body mass index, ECG, EGG, EDA).
Dependent Measure
The dependent measure was cybersickness as measured by the SSQ Total Score (TS; Kennedy et al., 1993) at 0, 15, 30, 45, and 60 min post exposure. The time component after VR exposure is critical to understanding the sustained negative effects of exposure on an individual (Stanney and Hash, 1998). Thus, for the purposes of regression analysis, cybersickness was operationalized as a “recovery” SSQ Total Score (TS), which was defined by the average SSQ TS 45 min post exposure and SSQ TS 1 h post exposure normalized by the Baseline (BL). Given a 20 min VR exposure duration and 1 h post exposure measurement period (i.e., 3x exposure duration), participants would be expected to have “recovered” to BL SSQ TS levels at the conclusion of the experiment.
Data Analysis
A mixed-model analysis of variance (ANOVA) was used to identify main and interaction effects among Gender, Display Type, and Post Exposure Measurement Time on cybersickness. A regression analysis was then used to characterize what might be driving any differences. Several steps were taken to determine which of the pool of candidate predictive variables (i.e., previous VR and gaming experience, FOV, IPD, field dependence, postural stability, female hormonal cycle, state/trait anxiety, migraine susceptibility, ethnicity, aerobic fitness, body mass index, physiological mechanisms) should be included in the regression analysis. First a univariate ANOVA was performed to evaluate significant gender differences among the potential predictive variables. The selection criterion chosen was whether or not each possible predictor variable was significantly different between the genders; those variables that were significantly different (set at p < 0.27 for univariate analysis; the more traditional 0.05 level can fail to identify important variables; Bursac et al., 2008) between the genders were included in the regression analysis. Next, a zero-order correlation analysis determined the strength of linear association among the predictor variables, as well as with the “recovery” SSQ TS metric. High correlation among predictor variables suggests redundant variable inclusion.
To further increase the predictability of the variables, especially given that an appropriate predictor to sample size ratio is 1:15, independent variables with the highest zero-correlation with the recovery SSQ TS metric were included first in the model. All categorical variables were dummy coded with males who fit the VR headset as the comparator. The IPD Fit metric classified males and females as out of and below the IPD range of the headset (<60.5 mm), within the range (60.5–74.4 mm), or out of and above the range (>74.4 mm). A binary classification of IPD Fit was then determined, which signified participants in IPD range for the HTC Vive or out of range (either below or above). The regression coefficient (β) of each predictor variable on recovery SSQ TS was calculated using SPSS version 24 multiple linear regression analysis. Models were evaluated for significant R2 change using an F-test and an a priori α level of 0.05, as well as multicollinearity using ≥0.20 as a cut off for tolerance and a variance inflation factor cutoff of ≥ 4.
Results
The results revealed that there were significant differences in the cybersickness experienced between the flatscreen TV and VR conditions. While there was a main effect of Gender [F(1, 42) = 4.13, p < 0.049], and a main effect of Display Type [F(1, 42) = 8.29, p < 0.006], there was also a significant interaction between Gender and Display Type [F(1, 42) = 4.85, p < 0.033]; with Gender differences found for the VR display but not flatscreen TV. As expected, for both genders low levels of cybersickness were experienced with exposure to flatscreen TV immediately after exposure (female AE1 SSQ TS mean = 7.95; S.D. = 18.15; male AE1 SSQ TS mean = 5.61; S.D. = 8.48; see Table 4) and these low levels continued throughout the post exposure measurement periods (female AE5 SSQ TS mean = 0.94; S.D. = 2.64; male AE5 SSQ TS mean = 3.74; S.D. = 4.90; see Table 4). On the other hand, VR exposure proved problematic to both genders, but with some clear differences (see Table 4 and Figure 2-Top). Specifically, immediately after VR exposure the adverse effects in females (AE1 SSQ TS 52.11; S.D. = 41.63) were, on average, more than 2x that of males (SSQ TS mean = 24.93; S.D. = 32.69); this difference was significant [F(1, 28) = 4.104, p = 0.05]. Further, these adverse effects persisted long after VR exposure for females (AE5 SSQ TS mean = 31.42; S.D. = 40.65), while males recovered much more quickly (AE5 SSQ TS mean = 3.24; S.D. = 6.76); this difference was significant [F(1, 28) = 7.27, p = 0.012]. Females in the VR condition, on average, never returned to BL levels (see Table 4 and Figure 2-Top, AE5), while males, on average, recovered to BL within 30 min post exposure (see Figure 2-Top, AE3). A regression analysis was conducted to characterize these gender differences and identify which predictor variables may be driving them.
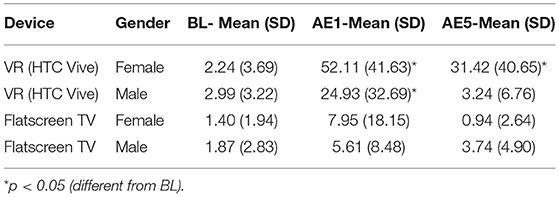
Table 4. Experiment 1 SSQ total score values at baseline (BL), immediately following exposure (aftereffect; AE1), and 1 h post exposure (aftereffect; AE5).
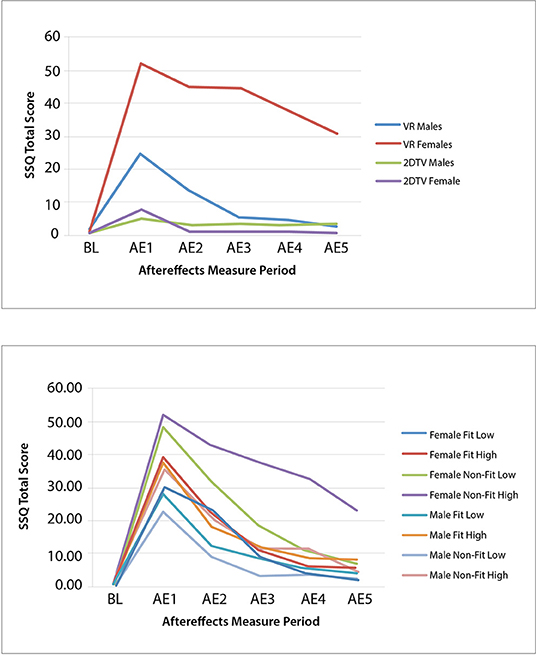
Figure 2. Experiment 1 (Top) and Experiment 2 (Bottom) group mean SSQ total score at baseline (BL) and each aftereffects (AE) measurement period for IPD fit and non–fit and motion sickness history low and high.
Table 3 summarizes the results from the ANOVA analysis for identifying variables significantly contributing to gender differences. Based on these results, the variables targeted for inclusion in the regression analysis were VR and gaming experience, FOV, IPD Fit, field dependence, female hormonal cycle, state anxiety, ethnicity, aerobic fitness, and past motion sickness history, as each of these variables demonstrated significant differences between genders (see Table 3). The variables excluded from the regression analysis were postural stability, trait anxiety, migraine susceptibility, body mass index, and physiological mechanisms, all of which were not significantly different between the genders (see Table 3).
As shown in Table 5, FOV was highly correlated with the IPD Fit measure, so FOV was removed from the model, as IPD Fit was correlated with Recovery SSQ TS but FOV was not. As well, Hormonal Cycle was highly correlated with Gender, so Hormonal Cycle was removed from the model, as Gender was correlated with Recovery SSQ TS but Hormonal Cycle was not. All other predictor variables targeted for inclusion were systematically added and removed from the model based on the F-statistic and multicollinearity until the model could no longer be significantly improved.
Table 6 shows the results from the multiple linear regression analysis. Of the 30 participants in the VR headset condition, 26 participants had complete data for the regression analysis. The results show that IPD Fit was a strong and significant (p = 0.009) predictor of cybersickness. Past motion sickness history also contributed to the explanatory power of the model. The resulting model, which had an R2 = 0.469, Adjusted R2 = 0.420, RMSE = 27.77, F(2, 23) = 9.70, p = 0.001, was as follows:
This model suggests that IPD non-fit and motion sickness history are positively correlated with cybersickness, with IPD non-fit being the most influential variable. This model accounted for 42.0% of the variability in cybersickness. Follow-up analyses indicated that the model passed the assumptions of multiple regression including normality and independence of residuals.
Experiment 1 Summary
The primary finding from Experiment 1 is that the most significant driver of gender differences in cybersickness was IPD non-fit, with motion sickness history also contributing. The IPD differences found in the sample population under evaluation in this study are summarized in Table 7. The table includes the number of individuals in each condition for which the HTC Vive IPD adjustable range could not be fit to the participant's IPD. The average male IPD (mean = 65.33; S.D. = 2.99) was 4.1% wider than females (mean = 62.63; S.D. = 3.52) and this difference was significant [F(1, 28) = 5.13, p = 0.031]. Within the female group, 5 of 15 or 33.3% (in line with expectations based on the US Army Anthropomorphic Survey [ANSUR] database; Gordon et al., 2014; see Table 2) of the females had an IPD that could not be properly fit to the VR headset, while all of the males fit. Of the five females whose IPD could not be fit, one had a low motion sickness history (MHQ ≤ 2). This individual had low sickness immediate post VR exposure (AE1 SSQ TS = 14.96) and recovered completely within 1 h post-VR exposure (AE5 SSQ TS = 0). The other four IPD non-fit females had a high motion sickness history (MHQ > 2) and these four females were profoundly sick immediate post VR exposure (AE1 SSQ TS mean = 74.8; S.D. = 48.76) and were not able to recover by AE5 (SSQ TS mean = 67.32; S.D. = 55.05). As all males could fit their IPD to the headset, no effects of IPD non-fit could be assessed for males. These results suggest that those for which a VR headset cannot be fit to their IPD and who have a high motion sickness history will be the most susceptible to cybersickness.
Why would IPD non-fit drive higher levels of cybersickness. There are plenty of online blogs and developer sites that claim that a little bit of a blurred image in a VR headset due to a mismatched IPD is no problem (c.f. SteamVR, 2016, 2018). Yet, even if the IPD non-fit results in a small loss of visual acuity, this can have a substantial negative impact (Skrbek and Petrov, 2013). IPD non-fit can lead to increased fusional difficulty (Rolland and Hua, 2005), binocular stress, increased near point convergence, an esophoric (inward) shift in distance heterophoria, and a drop in visual acuity, as well as asthenopia (i.e., fatigue, eye pain, blurred vision, double vision, headache, general malaise, nausea; Mon-Williams et al., 1993; Regan and Price, 1993; Best, 1996). These adverse effects occur because IPD non-fit leads to misalignment of the VR headset optics and/or inappropriate binocular overlap, resulting in perceptual issues. Regan and Price (1993) found that only those with an IPD less than the interocular distance (IOD), which refers to the distance between the optical centers of the lens systems installed in the VR headset, experienced such visual discomfort, with the greater the mismatch between the two measures (IPD and IOD) resulting in greater reported side-effects.
In this study, the IOD or distance between the HTC Vive lenses was set to coincide with the participant's IPD whenever possible. This alignment is anticipated to mitigate misalignment between optics of the eyes and that of the VR headset. However, as researchers note, when an alignment cannot be achieved this will result in viewing the VR headset lens system on an off-center axis, which will in turn lead to prismatic distortions that drive eyestrain and visual discomfort (Regan and Price, 1993; Costello, 1997; Peli, 1999; Lee et al., 2008). It is thus not surprising that in the current study, females experienced higher levels and longer lasting cybersickness than males, as one third of females had a smaller IPD than the VR headset. This mismatch between the IOD and IPD for woman is not correctible in software as it is a hardware issue and may lead to a higher likelihood over males of experiencing the taxing effects of a divergence demand such as visual fatigue (Costello, 1997).
Theoretical research suggests that the mismatch between inter-screen distance (ISD) and IOD is a driver of accommodation-convergence issues (Howarth, 1999). Choosing the correct eye-point for rendering computer generated graphics potentially diminishes these negative effects, especially depth errors, since choosing the correct eye-point will account for near- or far- field headset screen settings and aligns the center of the display with the optics and the correct eye-point of the end-user (Rolland et al., 2004). By adjusting the IPD of the end user and setting the IPD in the system software, the displays can be aligned to the eye-point of the participant if the headset IPD adjustable range allows. However, because the VR headset did not represent the full range of IPDs of the participants (i.e., while all males could properly fit their IPD to the headset, a third of the females could not be properly fit), there is a potential that the negative effects reported could be due to other types of interactions between the technology and rendered images.
If IPD non-fit is the main driver of gender differences in cybersickness, then females whose IPD fit the VR headset should experience cybersickness in a manner similar to males. Specifically, they should experience cybersickness at comparable levels upon immediate post VR exposure, and then they should recover at a rate similar to males. To test these assumptions, a second experiment was run.
Experiment 2
Materials and Methods
The purpose of Experiment 2 was to determine if females whose IPD could be fit to the VR headset experienced cybersickness in a manner similar to males. Based on the results of Experiment 1, it was anticipated that females would experience higher levels and longer lasting cybersickness than males only when their IPD could not be fit to the headset; and potentially only when their IPD was smaller than the IOD. It was also expected that both females and males with high motion sickness histories would experience cybersickness at higher levels as compared to those with low histories.
Participants
Adults aged 18–30 years, balanced between genders participated in this study. Participants were recruited through a market research firm. A total of 120 participants were recruited for the study based on their fit to one of eight experimental groups, which were defined according to gender (male vs. female), IPD (fit vs. non-fit), and motion sickness history (low vs. high). MHQ was defined as follows: Low Motion Sickness History = MHQ < = 2; High Motion Sickness History = MHQ > 2. This research complied with the American Psychological Association Code of Ethics and was approved by the Institutional Review Board at Copernicus Group. Informed consent was obtained from each participant and all participants were compensated for their time in the experiment. Data from the 30 VR participants from Experiment 1 were also included in the Experiment 2 data analysis, and the IPD Fit/Non-Fit and MHQ Low/High were identified for each Experiment 1 VR participant.
Experimental Design
The experiment was a mixed design, with 2 (gender) × 2 (VR headset IPD fit type) × 2 (motion sickness history type) between factors and a 5 (post exposure measurement time) within factor. Gender types were either male or female. VR headset IPD fit type was either IPD Fit or IPD Non-Fit. Motion sickness history type was either Low or High. The post exposure measurement times were 0, 15, 30, 45, and 60 min.
Beyond the randomization of participants to groups, the Equipment and Display Content, Procedure, Dependent Measures, and Data Analysis were the same as in Experiment 1. One additional Predictor Variable was added to Experiment 2, which was Exposure Duration. This was added to address any potential differences in drop-out rates.
Results
Complete datasets from 117 of the 120 participants in Experiment 2 were obtained and combined with the 30 VR participants from Experiment 1 to run the ANOVA, providing a total sample size of 147 participants. The combined data led to a total of: 40 female IPD Fit participants (19 were low MHQ; 21 were high MHQ), 45 male IPD Fit participants (25 were low MHQ; 20 were high MHQ), 34 female IPD Non-Fit participants (15 were low MHQ; 19 were high MHQ), and 28 male IPD Non-Fit participants (15 were low MHQ; 13 were high MHQ). Thus, when combining Experiment 1 and 2 data, there were a total of 85 IPD Fit VR participants and 62 IPD Non-Fit VR participants. The mixed-model ANOVA results revealed significant main effects for Gender [F(1, 139) = 7.36, p = 0.008] and MHQ, [F(1, 139) = 5.40, p = 0.022], as well as a significant interaction of Gender × MHQ × IPD Fit [F(1, 139) = 4.24, p = 0.008]. The results revealed that, as expected, females whose IPD fit the VR headset experienced cybersickness in a manner similar to males (see Table 8 and Figure 2-Bottom). Specifically, immediately after VR exposure, the average Recovery SSQ TS levels for those in the IPD Fit condition were high in both those with a high motion sickness history (females: AE1 SSQ TS mean = 40.25; S.D. = 35.99; males: AE1 SSQ TS mean = 32.91; S.D. = 36.78) and those with a low motion sickness history (females: SSQ TS mean = 28.94; S.D. = 30.25; males: AE1 SSQ TS mean = 23.49; S.D. = 28.98). There were no significant gender differences in the IPD Fit groups at AE1 (n = 85), regardless of motion sickness history [F(3, 81) = 1.029, p = 0.384]. All those who could fit their IPD to the VR headset recovered within 1 h post VR exposure, regardless of motion sickness history (high motion sickness history females: AE5 SSQ TS mean = 7.48; S.D. = 11.47; males: AE5 SSQ TS mean = 5.98; S.D. = 13.64; low motion sickness history females: AE5 SSQ TS mean = 4.53; S.D. = 19.13; males: AE5 SSQ TS mean = 0.45; S.D. = 5.10. There were no significant gender differences in the IPD Fit groups at AE5 (n = 85), regardless of motion sickness history [F(3, 81) = 1.29, p = 0.283]; further these groups had no significant differences from BL at AE5 [t(84) = −1.656, p = 0.104].
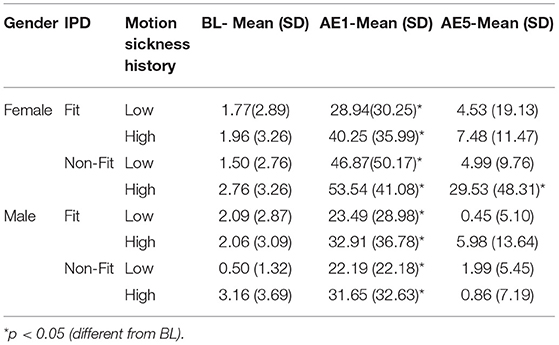
Table 8. Experiment 2 self-reported SSQ total score values at baseline (BL), immediately following exposure (aftereffect; AE1), and 1 h post exposure (aftereffect; AE5).
Immediately after VR exposure, the adverse effects in those that had an IPD non-fit were, on average, high in both those with a high motion sickness history (female AE1 SSQ TS mean = 53.54; S.D. = 41.08; male AE1 SSQ TS mean = 31.65; S.D. = 32.63) and those with a low motion sickness history (females AE1 SSQ TS mean = 46.87; S.D. = 50.17; males AE1 SSQ TS mean = 22.19; S.D. = 22.18). There were no significant gender effects in the IPD Non-Fit groups at AE1 (n = 62), regardless of motion sickness history [F(3, 58) = 2.25, p = 0.092]. There was a statistically significant difference in AE5 SSQ TS among the groups [F(3, 58) = 4.19, p = 0.009; n = 62]. A Tukey post-hoc analysis showed that these adverse aftereffects persisted long after VR exposure only for those females with an IPD non-fit and high motion sickness history (females AE5 SSQ TS mean = 29.53; S.D. = 48.31) as compared to females with low motion sickness history (AE5 SSQ TS mean = 4.99, S.D. = 9.76, p = 0.047), males with low motion sickness history (AE5 SSQ TS mean = 1.99, S.D. = 5.45, p = 0.033), or males with high motion sickness history (AE5 SSQ TS mean = 0.86, S.D. = 7.19, p = 0.034). Both females (female AE5 SSQ TS mean = 4.53; S.D. = 19.13) and males (male AE5 SSQ TS mean = 1.99; S.D. = 5.45) with an IPD non-fit and low motion sickness history recovered by the final measurement period. Females in the IPD Non-Fit, High Motion Sickness History condition also had, on average, less VR exposure duration (i.e., tended to drop-out; Mean Exposure Duration = 15.95 min; S.D. = 6.42) as compared to both males (Mean Exposure Duration = 20 min; S.D. = 0.00, i.e., no dropouts) and females (Mean Exposure Duration = 19.81 min; S.D. = 0.544) in the IPD Non-Fit, Low Motion Sickness History conditions; this difference was significant [F(7, 139) = 2.71, p = 0.012].
Table 9 shows the results from the multiple linear regression analysis from Experiment 2. Of the 120 participants in Experiment 2, 109 participants had complete data for the regression analysis. These data were combined with the 26 participants from Experiment 1 with complete data sets for the regression analysis, providing 135 complete data sets. The first step in the analysis was the same, the univariate analysis of each possible predictor variable, with the results mostly replicating the Experiment 1 findings (i.e., VR and gaming experience, FOV, IPD fit, female hormonal cycle, state anxiety, ethnicity, aerobic fitness, and past motion sickness history demonstrated significant differences between genders and were targeted for inclusion in the regression analysis), with the addition of EGG (bradygastria) and exposure duration also demonstrating significant gender differences (see Table 3) and thus these two additional variables were targeted for regression analysis inclusion. The variables excluded from the regression analysis were postural stability, trait anxiety, migraine susceptibility, and body mass index, which were the same as Experiment 1, with the addition of field dependence, all of which were not significantly different between the genders (see Table 3).
As in Experiment 1, in Experiment 2 Hormonal Cycle had a very high linear association with Gender, so Hormonal Cycle was removed from the model, as Gender was correlated with Recovery SSQ TS but Hormonal Cycle was not (see Table 9). All other predictor variables targeted for inclusion were systematically added and removed from the model based on the F-statistic and multicollinearity until the model could no longer be significantly improved.
Table 10 shows the results from the multiple linear regression analysis. The resulting model, which had an R2 = 0.322, Adjusted R2 = 0.301, RMSE = 20.06, F(4, 130) = 15.40, p = 0.001, was as follows:
This model suggests that IPD non-fit, motion sickness history, and bradygastria are positively correlated with cybersickness, while exposure duration (i.e., how long an individual was able to remain in VR) is negatively correlated to cybersickness. As in Experiment 1, IPD non-fit was found to be the most influential variable, followed by motion sickness history. This model accounted for 32.2% of the variability in cybersickness. Follow-up analyses indicated that the model passed the assumptions of multiple regression including normality and independence of residuals.
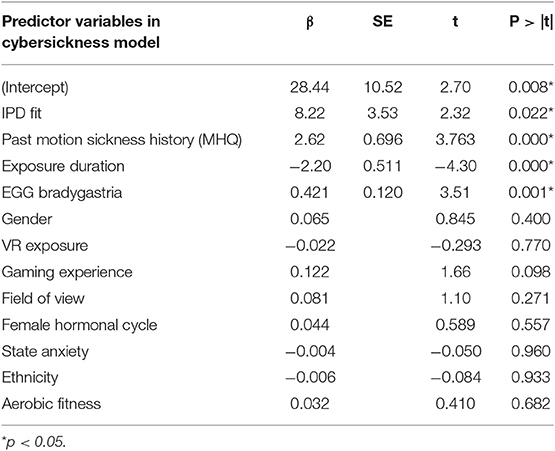
Table 10. Summary of multiple linear regression model of cybersickness for Experiment 1 and Experiment 2 combined data.
Experiment 2 Summary
Similar to Experiment 1, Experiment 2 found that the primary driver of cybersickness is IPD non-fit, followed by motion sickness history. Experiment 2 also found higher EGG (bradygastria) and higher dropout rates (i.e., lower exposure duration) associated with higher levels of cybersickness. In terms of EGG, previous research has indicated that bradygastria is a correlate of motion sickness (Lang et al., 1999) and changes to bradygastria immediately precede nausea (Kim et al., 2005; Dennison et al., 2016); this associated objective physiological response, in effect, validates the subjective SSQ TS results in the current study. In terms of exposure duration, increased cybersickness has been previously associated with higher drop-out rates (Stanney et al., 1999), and the negative correlation for exposure duration mirrors this finding. Further, the results from Experiment 2 demonstrated that females whose IPD could be fit to the VR headset experienced cybersickness in a manner similar to males, while those females who could not be fit experienced more severe and more persistent cybersickness. For females and males whose IPD could be fit to the VR headset, they experienced high levels of cybersickness immediately after VR exposure but fully recovered within 1 h post exposure, regardless of motion sickness history (all AE5 SSQ TS not significantly different than BL; see Table 8 and Figure 3-Top).
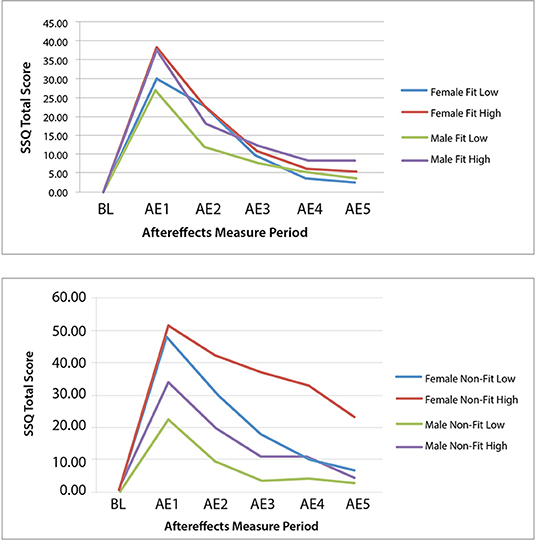
Figure 3. Experiment 2 group mean SSQ total score at baseline (BL) and each aftereffects (AE) measurement period for the IPD fit (Top) and non–fit (Bottom) and motion sickness history low and high experimental groups.
In the high motion sickness history conditions, IPD non-fit did not affect males to the same degree as females, as males were able to recover to baseline while females were not (see Table 8 and Figure 3-Bottom). This may be because males' IPD non-fit was not as severe as females' and a greater degree of mismatch has been associated with more severe adverse effects (Mon-Williams et al., 1993; Regan and Price, 1993; Best, 1996). In general, all but one female in the IPD Non-Fit condition had an IPD smaller than the adjustable IPD range, and the average IPD of this group was 57.52 mm (S.D. = 1.77). All but two males in the IPD Non-Fit condition had an IPD smaller than the adjustable IPD range, and the average IPD of this group was 58.87 mm (S.D. = 1.14). There was a significant difference [F(1, 60) = 7.48, p = 0.008], in the severity of the IPD non-fit between males and females (n = 62), with females having a more severe non-fit. This greater degree of IPD non-fit was associated with a significantly higher level of cybersickness for females vs. males immediately following VR exposure (see Tables 5, 8 and Figures 2, 3).
General Discussion
This study sought to identify the main drivers of gender differences associated with the adverse effects of VR exposure. Two experiments were conducted, the first to investigate the many variables that could contribute to gender differences and the second to validate and further explore the findings of the first. In both experiments, IPD non-fit was found to be the main driver of cybersickness, with motion sickness history a secondary driver.
Interpupillary Distance
Quite interestingly, it was not an inherent characteristic of females but rather a characteristic of the VR headset itself, IPD non-fit, that was found to be the primary driver of cybersickness in both experiments. To properly view objects in a virtual environment, most VR headsets have a variable IPD range that allows an individual to align the center of their pupils with the center of the VR lenses. Any deviation between IPD and IOD can cause a host of visual issues, as well as asthenopia (Mon-Williams et al., 1993; Regan and Price, 1993; Best, 1996; Rolland and Hua, 2005). To resolve this issue and allow both females and males to be able to properly center their pupils to the lenses, the IPD range needs to be adjustable from ~ 50 to 77 mm (Dodgson, 2004; Gordon et al., 2014). As can be seen in Table 2, the Sony PlayStation headset accommodates this range but many other VR headsets on the market today do not (e.g., Samsung Gear VR, Oculus Rift, Oculus Rift S, Oculus Quest, HTC Vive, HTC Vive Pro). Another option is to custom fit VR headsets to an individual, much like eyeglasses (Luckey, 2019). Software IPD adjustment helps with scale issues but does not address issues with hard to fuse imagery, blurry images, distortion, or VOR mismatches (Luckey, 2019), thus it may not be the panacea many believe it to be. Other possible alternatives to resolve this issue include gaze-contingent and adaptive focus displays (Padmanaban et al., 2017), yet these solutions still pose challenges to the human visual system (Rolland et al., 2000; Mercier et al., 2017). Until such modifications to VR headsets are made, females will be at a particular disadvantage with regard to cybersickness because in general the IPD range in current VR headsets accommodates substantially fewer females as compared to males (see Table 2) and their IPD mismatch will likely be more severe than males.
Beyond widening the IPD range, there are a number of design parameters that need to be considered to ensure the design of VR headsets better accommodates human physiology. Specifically, Robinett and Rolland (1992) noted that VR headsets are cross compared using engineering techniques that use a reduced eye model that sets average human constraints based on male specific measures, such as performing tests using a static IPD of 64 or 65 mm (note, Male mean IPD is 64.0 mm [S.D. = 3.4 mm]; Gordon et al., 2014). These model simplifications ignore performance limitations compared to the human eye (e.g., FOV, resolution). Specifically, the VR headset parameters of resolution, image focus, contrast, brightness, and frame rate are interdependent parameters of a VR headset that affect viewability of complex, dynamic VR imagery. At the same time, the human eye is an optical system that is functionally limited much like the VR headset in such parameters as display resolution and image quality. Clearly explicating these limitations and avoiding making display choices that do not match human visual capabilities (see potential mismatches in Table 11) will reduce cybersickness. By understanding these challenges, VR headset design can be much improved to better accommodate the human visual system.
The results of Experiment 2 suggest that if an individual's IPD can be properly fit to the VR headset, gender differences in cybersickness are not expected (see Table 8 and Figure 3-Top). Cybersickness is still expected, as was experienced in Experiment 2 (see AE1 in Table 8) due to visual-vestibular mismatches (Reason and Brand, 1975; Oman, 1998) and vergence-accommodation conflict (Szpak et al., 2019). Specifically, if designers create content with a great deal of vection (Webb and Griffin, 2003) associated with high levels of visual-vestibular mismatches and/or content with a large conflict between vergence and focal distances (Hoffman et al., 2008), these conflicts are expected to precipitate sickness (see Figure 4). Based on the results of Experiment 2, sickness levels upon immediate post VR exposure are expected to be higher in those with a high motion sickness history. However, regardless of motion sickness history, these adverse effects are expected to dissipate once adaptations (e.g., avoiding visual dominance, adopting postural control strategies such as through active viewpoint control, and cuing off a rest frame to minimize visual-vestibular mismatches) and habituation with repeat exposures kick-in (see Figure 4), as was experienced in Experiment 2 (see AE5 in Table 8), and in proportion to exposure duration for those individuals that can properly fit their IPD (Kennedy et al., 2000; Murata, 2004). Note that the VR content used in this study was designed to induce cybersickness by creating content that was intended to be provocative. Yet, even with potent VR content, the results from Experiment 2 demonstrated that when the IPD could be properly fit to the VR headset, both males and females recovered from the adverse effects of VR exposure within 1 h post VR exposure, regardless of motion sickness history (see Figure 3-Bottom). It is only when an individual has the provoking factor of an IPD that cannot be properly fit, specifically when the IPD of an individual is smaller than the IOD, and the individual has the predisposing factor of a high motion sickness history that the individual is expected to enter a perpetuating loop that does not allow cybersickness recovery and habituation (see Figure 4).
Motion Sickness History
Many individuals experience motion sensitivity during activities such as reading when being a passenger in an automobile (Turner and Griffin, 1999), riding on a boat (Lawther and Griffin, 1986; 1988; Cooper et al., 1997), riding on a train (Kaplan, 1964), and flying (Lederer and Kidera, 1954; Turner et al., 2000), with these activities leading to feelings of dizziness, general malaise, nausea, blurry vision, and other such adverse effects. Individuals with such a history of motion sensitivity may be more susceptible to cybersickness in virtual environment than those without such history. Motion sensitivity has been suggested to be caused by vestibular dysfunction (Akin and Davenport, 2003) and/or an over-reliance on the visual system with a residual deficit of the vestibular system (Akiduki et al., 2003). Daily, short (5 min) vestibular adaptation exercises in those with vestibular dysfunction have been shown to be effective in reducing symptomatology (Alyahya et al., 2016). In fact, habituation (i.e., desensitization with repeat exposures) has been suggested to be the most effective countermeasure to motion sickness, even more so than anti-motion sickness drugs (Cowings and Toscano, 2000). Specifically, over repeat exposures to VR environments, habituation may occur in which symptomatology decreases (Kennedy and Graybiel, 1965; Biocca, 1992; McCauley and Sharkey, 1992; Regan, 1995; Domeyer et al., 2013; Welch, 2014). Habituation is oftentimes highly effective (Golding, 2017), perhaps as high as 85% effective (Benson, 1999). Habituation protocols would involve designing VR applications with stepwise increments in stimulus intensity coupled with frequent exposures of slowly increasing duration, which may allow motion sensitive individuals to acclimate to the experience, enable initial faster recovery and more sessions to be tolerated. Based on Welch (2014), important elements of a habituation protocol would include: (1) active (not passive) interaction within the VR environment, coupled with the visual consequences associated with these actions (reafference), (2) immediate feedback to this interaction (any transport delays, response lags, etc. will hinder adaptation, however, if these lags are consistent, then adaptation may still be achieved), (3) incremental (rather than massed) exposure, with progressive VR stimulus strength (e.g., start with mild, slow movements, constant velocity, etc.), and (4) the use of distributed practice (e.g., 2–5 day intersession intervals). However, based on the results of these studies, should the VR headset pose an IPD non-fit, such habituation protocols may not prove effective.
If IPD fit is achieved, such habituation protocols hold great promise in addressing gender differences, as females are generally more disposed, as compared to males, to benefit from such conditioning countermeasures (Rohleder et al., 2006; Stockhorst et al., 2007). For example, Rohleder et al. (2006) demonstrated that physiological habituation to a repetitive rotation experience was demonstrated only in females via habituation of a rotation-induced cortisol response, whereas males continued to show cortisol sensitivity. Thus, even though females oftentimes report being more highly susceptible to motion sickness than males (Lentz and Collins, 1977; Park and Hu, 1999; Dobie et al., 2001; Graeber and Stanney, 2002; Stanney et al., 2003; Wilson and Kinsela, 2017), and this susceptibility may lead to higher levels of cybersickness in virtual environments, susceptibility difference for both females and males can be counteracted via appropriate habituation practices.
It is also interesting to note that when IPD was properly fit, even those with a high motion sickness history could recover within 1 h post exposure (see Figure 3-Top). Thus, the impact of motion sickness history is not as profound as that of IPD non-fit, which the regression model confirmed.
Limitations
Given the vast range of motion sensitivity in the general population, which varies by about 10-1 (Lackner, 2014), a larger sample would have been desirable. Further, while the SSQ (Kennedy et al., 1993) is a standard measure of motion sickness that has been used for decades (Bulk et al., 2013), future research should add objective measures of the adverse aftereffects of VR exposure to confirm subjective reports of cybersickness, e.g., measures of ataxia, VOR shift, kinesthetic position sense shift (Kennedy et al., 1998).
Conclusions
In summary, Experiment 1 identified that IPD non-fit is a primary driver of gender differences in cybersickness. Experiment 2 confirmed this finding and further demonstrated that when an individual's IPD could be properly fit to the VR headset, females experienced cybersickness in a manner similar to males, with high levels immediately post VR exposure and recovery within 1 h post exposure following a 20 min provocative VR exposure. As more females were unable to properly fit their IPD to currently available VR headsets, and any IPD non-fit experienced was more extreme in females than males, VR technology was indeed found to be sexist, but it does not have to be. If VR headset manufacturers implement an IPD adjustable range of ~ 50 to 77 mm to capture >99% of both females and males, it is anticipated that a far greater number of females will be able to harness the performance enhancing potential of VR technology. In addition, motion sickness susceptibility contributes to higher levels of cybersickness and this can be counteracted via habituation protocols.
Data Availability Statement
The datasets generated for this study are available on request to the corresponding author.
Ethics Statement
The studies involving human participants were reviewed and approved by Copernicus Group. The participants provided their written informed consent to participate in this study.
Author Contributions
KS conducted the literature review, designed the experiments, directed the study, and was the lead author of the paper. CF led the data analytics. LF reviewed and provided feedback on all aspects of this research.
Funding
The authors declare that this study received funding from Lockheed Martin Corporation. The funder was not involved in the study design, collection, analysis, interpretation of data, the writing of this article or the decision to submit it for publication. The funder did review the manuscript prior to publication.
Conflict of Interest
KS and CF are employed by Design Interactive, Inc. LF is employed by Lockheed Martin Corporate.
Acknowledgments
The authors would like to thank Erin Baker for helping to run the study, Charles Ortiz for developing the virtual rollercoaster, Scott Christian, Peyton Bailey, Caroline Bates, Haden Eckbert, and Jason English for their assistance in conducting the experiment, David Campbell for his support of the measurement tools, and Joanna Chiang for assistance with data analysis. Much gratitude is also extended to Robert S. Kennedy for his mentorship in the area of motion sickness and beyond throughout the past several decades.
References
Akiduki, H., Nishiike, S., Watanabe, H., Matsuoka, K., Kubo, T., and Takeda, N. (2003). Visual-vestibular conflict induced by virtual reality in humans. Neurosci. Lett. 340, 197–200. doi: 10.1016/S0304-3940(03)00098-3
Akin, F. W., and Davenport, M. J. (2003). Validity and reliability of the motion sensitivity test. J. Rehabil. Res. Dev. 40, 415–421. doi: 10.1682/JRRD.2003.09.0415
Alexander, S. J., Cotzin, M., Klee, J. B., and Wendt, G. R. (1947). Studies of motion sickness: XVI. The effects upon sickness rates of waves of various frequencies but identical acceleration. J. Exp. Psychol. 37, 440–448. doi: 10.1037/h0063240
Alyahya, D., Johnson, E. G., Daher, N. S., Gaikwad, S. B., Deshpande, S., Cordett, T. K., et al. (2016). Effect of vestibular adaptation exercises on chronic motion sensitivity: a randomized controlled trial. Phys. Ther. Rehabil. 3:1. doi: 10.7243/2055-2386-3-1
Amen, D. G., Trujillo, M., Keator, D., Taylor, D. V., Willeumier, K., Meysami, S., et al. (2017). Gender-based cerebral perfusion differences in 46,034 functional neuroimaging scans. J. Alzheimer's Dis. 60, 605–614. doi: 10.3233/JAD-170432
Ames, S. L., Wolffsohn, J. S., and Mcbrien, N. A. (2005). The development of a symptom questionnaire for assessing virtual reality viewing using a head-mounted display. Optom. Vision Sci. 82, 168–176. doi: 10.1097/01.OPX.0000156307.95086.6
Arslanian-Engoren, C., and Engoren, M. (2010). Physiological and anatomical bases for sex differences in pain and nausea as presenting symptoms of acute coronary syndromes. Heart Lung 39, 386–393. doi: 10.1016/j.hrtlng.2009.10.013
Banta, G. R., Ridley, W. C., McHugh, J., Grissett, J. D., and Guedry, F. E. (1987). Aerobic fitness and susceptibility to motion sickness. Aviat. Space Environ. Med. 58, 105–108.
Becker-Bense, S., Buchholz, H.-G., zu Eulenburg, P., Best, C., Bartenstein, P., Screckenberger, M., et al. (2012). Ventral and dorsal streams processing visual motion perception (FDG-PET study). BMC Neurosci. 13:81. doi: 10.1186/1471-2202-13-81
Benson, A. J. (1999). “Motion sickness,” in Aviation Medicine, eds J. Ernsting, A. N. Nicholson, and D. S. Rainford (Oxford, UK: Butterworth Ltd.), 455–471.
Best, S. (1996). “Perceptual and oculomotor implications of interpupillary distance settings on a head-mounted virtual display,” in Proceedings IEEE National Aerospace and Electronics Conference, Vol. 1 (Dayton, OH: IEEE), 429–434.
Biocca, F. (1992). Will simulator sickness slow down the diffusion of virtual environment technology? Presence 1, 334–343. doi: 10.1162/pres.1992.1.3.334
Bulk, S. A., Bertola, M. A., and Inman, V. W. (2013). “Simulator sickness questionnaire: twenty years later,” in Proceedings of the 7th International Driving Symposium on Human Factors in Driver Assessment, Training, and Vehicle Design (Bolton Landing, NY), 257–263.
Burg, A. (1966). Visual acuity as measured by dynamic and static tests: a comparative evaluation. J. Appl. Psychol. 50, 460–466. doi: 10.1037/h0023982
Bursac, Z., Gauss, C. H., Williams, D. K., and Hosmer, D. W. (2008). Purposeful selection of variables in logistic regression. Source Code Biol. Med. 3:17. doi: 10.1186/1751-0473-3-17
Cakmakci, O., and Rolland, J. (2006). Head-worn displays: a review. J Display Technol. 2, 199–216. doi: 10.1109/JDT.2006.879846
Carbotte, K. (2016). The Oculus Rift Review: What's Inside the Oculus Rift Box. Available online at: https://www.tomshardware.com/reviews/oculus-rift-virtual-reality-hmd,4506-2.html (accessed January 19, 2020).
Cha, Y. H., Chakrapani, S., Craig, A., and Baloh, R. W. (2012). Metabolic and functional connectivity changes in mal de debarquement syndrome. PLoS ONE 7:e49560. doi: 10.1371/journal.pone.0049560
Cheung, B., and Hofer, K. (2002). Lack of gender difference in motion sickness induced by vestibular coriolis cross-coupling. J. Vestib. Res. 12, 191–200.
Cho, A. A., Clark, J. B., and Rupert, A. H. (1995). Visually triggered migraine headaches affect spatial orientation and balance in a helicopter pilot. Aviat. Space Environ. Med. 66, 353–358.
Cooper, C., Dunbar, N., and Mira, M. (1997). Sex and seasickness on the Coral Sea. Lancet 350:892. doi: 10.1016/S0140-6736(05)62083-1
Costello, P. J. (1997). Health and Safety Issues Associated With Virtual Reality: a Review of Current Literature (Loughborough: Advisory Group on Computer Graphics), 1-23
Cowings, P. S., and Toscano, W. B. (2000). Autogenic-feedback training exercise is superior to promethazine for control of motion sickness symptoms. J. Clin. Pharmacol. 40, 1154–1165. doi: 10.1177/009127000004001010
Darlington, C. L., and Smith, P. F. (1998). Further evidence for gender differences in circular vection. J. Vestib. Res. 8, 151–153. doi: 10.3233/VES-1998-8203
Dawson, H. (1982). Practical aspects of the low frequency noise problem. J. Low Frequency Noise Vibration 1, 28–44. doi: 10.1177/026309238200100105
Dennison, M. S., Wisti, A. Z., and D'Zmura, M. (2016). Use of physiological signals to predict cybersickness. Displays 44, 42–52. doi: 10.1016/j.displa.2016.07.002
Diels, C., and Howarth, P. A. (2013). Frequency characteristics of visually induced motion sickness. Hum. Factors 55, 595–604. doi: 10.1177/0018720812469046
Dobie, T., McBride, D., Dobie, T. Jr., and May, J. (2001). The effects of age and sex on susceptibility to motion sickness. Aviat. Space Environ. Med. 72, 13–20.
Dodgson, N. A. (2004). “Variation and extrema of human interpupillary distance,” in Stereoscopic Displays and Virtual Reality Systems XI vol. 5291, eds A. J. Woods, J. O. Merritt, S. A. Benton, and M. T. Bolas (San Jose, CA: International Society for Optics and Photonics), 36–47.
Domeyer, J. E., Cassavaugh, N. D., and Backsa, R. W. (2013). The use of adaptation to reduce simulator sickness in driving assessment and research. Accid. Anal. Prev. 53, 127–132. doi: 10.1016/j.aap.2012.12.039
Donner, N. C., and Lowry, C. A. (2013). Sex differences in anxiety and emotional behavior. Pflügers Arch. 465, 601–626. doi: 10.1007/s00424-013-1271-7
Ekstrom, R. B., Dermen, D., and Harman, H. H. (1976). Manual for Kit of Factor-Referenced Cognitive Tests (Vol. 102). Princeton, NJ: Educational Testing Service.
Entertainment Software Association (ESA) (2016). Essential Facts About the Computer and Video Game Industry. Available online at: http://www.theesa.com/wp-content/uploads/2016/04/Essential-Facts-2016.pdf. (accessed October 16, 2017).
Finley, J. C. Jr., O'Leary, M., Wester, D., MacKenzie, S., Shepard, N., Farrow, S., and Lockette, W. (2004). A genetic polymorphism of the alpha2-adrenergic receptor increases autonomic responses to stress. J. Appl. Physiol. 96, 2231–2239. doi: 10.1152/japplphysiol.00527.2003
Flanagan, M. B., May, J. G., and Dobie, T. G. (2005). Sex differences in tolerance to visually-induced motion sickness. Aviat. Space Environ. Med. 76, 642–646.
Fledelius, H. C., and Stubgaard, M. (1986). Changes in eye position during growth and adult life as based on exophthalmometry, interpupillary distance and orbital distance measurements. Acta Ophthalmol. 64, 481–486. doi: 10.1111/j.1755-3768.1986.tb06958.x
Fulvio, J. M., Ji, M., and Rokers, B. (2018). Variability in sensory sensitivity predicts motion sickness in virtual reality. bioRxiv [pre print]. doi: 10.1101/488817
Girdler, S. S., Turner, J. R., Sherwood, A., and Light, K. C. (1990). Gender differences in blood pressure control during a variety of behavioral stressors. Psychosom. Med. 52, 571–591. doi: 10.1097/00006842-199009000-00009
Golding, J. F. (1998). Motion sickness susceptibility questionnaire revised and its relationship to other forms of sickness. Brain Res. Bull. 47, 507–516. doi: 10.1016/S0361-9230(98)00091-4
Golding, J. F. (2006). Motion sickness susceptibility. Auton. Neurosci. 129, 67–76. doi: 10.1016/j.autneu.2006.07.019
Golding, J. F. (2017). “Motion sickness susceptibility and management at sea,” in Maritime Psychology: Research in Organizational & Health Behavior at Sea, ed M. MacLachlan (Cham: Springer), 151–183
Golding, J. F., Arun, S., Wortley, E., Wotton-Hamrioui, K., Cousins, S., and Gresty, M. A. (2009). Off-vertical axis rotation of the visual field and nauseogenicity. Aviat. Space Environl Med. 80, 516–521. doi: 10.3357/ASEM.2433.2009
Golding, J. F., Kadzere, P., and Gresty, M. A. (2005). Motion sickness susceptibility fluctuates through the menstrual cycle. Aviat. Space Environ. Med. 76, 970–973.
Gordon, C. C., Blackwell, C. L., Bradtmiller, B., Parham, J. L., Barrientos, P., Paquette, S. P., et al. (2014). 2012 Anthropometric Survey of U.S. Army Personnel: Methods and Summary Statistics. Technical Report NATICK/15-007. U.S. Army Natick Soldier Research, Development and Engineering Center, Natick MA.
Graeber, D., and Stanney, K. M. (2002). “Gender differences in visually induced motion sickness,” in 46th Annual Human Factors and Ergonomics Society Meeting (Baltimore, MD), 2109–2113.
Granziera, C., DaSilva, A. F. M., Snyder, J., Tuch, D. S., and Hadjikhani, N. (2006). Anatomical alterations of the visual motion processing network in migraine with and without aura. PLoS Med. 3:e402. doi: 10.1371/journal.pmed.0030402
Harris, L. J. (1978). “Sex differences in spatial ability: possible environmental, genetic, and neurological factors,” in Asymmetrical Function of the Brain, ed M. Kinsbourne (Cambridge, UK: Cambridge University Press), 405–522.
Heaney, D. (2019). Data Suggests Oculus Rift S IPD Range ‘best' for Just Half of Adults. Available online at: https://uploadvr.com/data-suggests-oculus-rift-s-ipd-range-best-for-around-half-of-adults/ (accessed September 4, 2019).
Hellstroèm, L., Wahrenberg, H., Hruska, K., Reynisdottir, S., and Arner, P. (2000). Mechanisms behind gender differences in circulating leptin levels. J. Intern. Med. 247, 457–462. doi: 10.1046/j.1365-2796.2000.00678.x
Hoffman, D. M., Girshick, A. R., Akeley, K., and Banks, M. S. (2008). Vergence-accommodation conflicts hinder visual performance and cause visual fatigue. J. Vision 8, 1–30. doi: 10.1167/8.3.33
Howarth, P. A. (1999). Oculomotor changes within virtual environments. Appl. Ergon. 30, 59–67. doi: 10.1016/S0003-6870(98)00043-X
International Headache Society (IHS) (2017). Diagnosing Migraine (I.H.S Criteria). Available online at: https://migraine.ie/hcp/migraine-diagnosis/#diagnosingmigraine (accessed November, 2017)
Jennings, R. T., Davis, J. R., and Santy, M. (1988). Comparison of aerobic fitness and space motion sickness during the shuttle program. Aviat. Space Environ. Med. 59, 448–451.
Johnson, B. G., Wright, A. D., Beazley, M. F., Harvey, T. C., Hillenbrand, P., and Imray, C. H. E. (2005). The Sharpened Romberg Test for assessing ataxia in mild acute mountain sickness. Wilderness Environ. Med. 16, 62–66. doi: 10.1580/PR02-04.1
Jokerst, M. D., Gatto, M., Fazio, R., Gianaros, P. J., Stern, R. M., and Kock, K. L. (1999). Effects of gender of subjects and experimenter on susceptibility to motion sickness. Aviat. Space Environ. Med. 70, 962–965.
Kaiser, R., and Schatsky, D. (2017). For More Companies, New Ways of Seeing: Momentum Is Building for Augmented and Virtual Reality in the Enterprise. Deloitte Insights. Available online at: https://dupress.deloitte.com/dup-us-en/focus/signals-for-strategists/augmented-and-virtual-reality-enterprise-applications.html#endnote-sup-1. (accessed October 18, 2017).
Kajantie, E., and Phillips, D. I. W. (2006). The effects of sex and hormonal status on the physiological response to acute psychosocial stress. Psychoneuroendocrinology 31, 151–178. doi: 10.1016/j.psyneuen.2005.07.002
Kennedy, R. S., Fowlkes, J. E., Berbaum, K. S., and Lilienthal, M. G. (1992). se of a motion sickness history questionnaire for prediction of simulator sickness. Aviat. Space Environ. Med. 63, 588–593. doi: 10.1037/t04669-000
Kennedy, R. S., and Graybiel, A. (1965). The Dial test: A Standardized Procedure for the Experimental Production of Canal Sickness Symptomatology in a Rotating Environment (Report No. 113, NSAM 930). Naval School of Aerospace Medicine, Pensacola, FL. doi: 10.21236/AD0625863
Kennedy, R. S., Lane, N. E., Berbaum, K. S., and Lilienthal, M. G. (1993). Simulator sickness questionnaire: an enhanced method for quantifying simulator sickness. Int. J. Aviat. Psychol. 3, 203–220. doi: 10.1207/s15327108ijap0303_3
Kennedy, R. S., Lanham, D. S., Massey, C. J., Drexler, J. M., and Lilienthal, M. G. (1995). Gender differences in simulator sickness incidence: implications for military virtual reality systems. SAFE J. 25, 69–76.
Kennedy, R. S., Stanney, K. M., and Dunlap, W. P. (2000). Duration and exposure to virtual environments: sickness curves during and across sessions. Presence 9, 463–472. doi: 10.1162/105474600566952
Kennedy, R. S., Stanney, K. M., and Lawson, B. (1998). “Independence of different cybersickness aftereffects: basis for a theory,” in 69th Annual Scientific Meeting of the Aerospace Medical Association (Seattle, WA), 17–21.
Kim, Y. Y., Kim, H. J., Kim, E. N., Ko, H. D., and Kim, H. T. (2005). Characteristic changes in the physiological components of cybersickness. Psychophysiology 42, 616–625. doi: 10.1111/j.1469-8986.2005.00349.x
Klosterhalfen, S., Kellermann, S., Pan, F., Stockhorst, U., Hall, G., and Enck, P. (2005). Effects of ethnicity and gender on motion sickness susceptibility. Aviat. Space Environ. Med. 76, 1051–1057.
Klosterhalfen, S., Pan, F., Kellermann, S., and Enck, P. (2006). Gender and race as determinants of nausea induced by circular vection. Gend. Med. 3, 236–242. doi: 10.1016/S1550-8579(06)80211-1
Kohl, R. L. (1990). “Endocrinology of space/motion sickness,” in Motion and Space Sickness, ed G. H. Crampton (Boca Raton, FL: CRC), 65–86.
Koslucher, F., Haaland, E., and Stoffregen, T. A. (2016). Sex differences in visual performance and postural sway precede sex differences in visually induced motion sickness. Exp. Brain Res. 234, 313–322. doi: 10.1007/s00221-015-4462-y
Lackner, J. R. (2014). Motion sickness: more than nausea and vomiting. Exp. Brain Res. 232, 2493–2510. doi: 10.1007/s00221-014-4008-8
Lang, I. M., Sarna, S. K., and Shaker, R. (1999). Gastrointestinal motor and myoelectric correlates of motion sickness. Neuroregul. Motil. 277, G642–G652. doi: 10.1152/ajpgi.1999.277.3.G642
Lawson, B. D. (2014). “Motion sickness scaling,” in Handbook of Virtual Environments: Design, Implementation, and Applications, 2nd Edn, eds K. S. Hale and K. M. Stanney (Boca Raton, FL: CRC Press), 601–626.
Lawther, A., and Griffin, M. J. (1986). Prediction of the incidence of motion sickness from the magnitude, frequency, and duration of vertical oscillation. J. Acoust. Soc. Am. 79, S86–S86. doi: 10.1121/1.2023435
Lederer, L. G., and Kidera, G. J. (1954). Passenger comfort in commercial air travel with reference to motion sickness. Int. Rec. Med. Gen. Pract. Clin. 167, 661–668.
Lee, Y. Y., Chen, T., and Alvarez, T. L. (2008). Quantitative assessment of divergence eye movements. J. Vis. 8, 5.1–13. doi: 10.1167/8.12.5
Leibach, H. (2015). Meet the Consumers that will Make or Break Virtual Reality Next Year. SingularityHub. Available online at: https://singularityhub.com/2015/12/17/vr-consumer-insights-for-2016-provided-by-greenlight-vr/ (accessed October 16, 2017).
Lentz, J. M., and Collins, W. E. (1977). Motion sickness susceptibility and related behavioral characteristics in men and women. Aviat. Space Environ. Med. 48, 316–322.
Ling, Y., Brinkman, W.-P., Nefs, H. T., Qu, C., and Heynderickx, I. (2011). “Cybersickness and anxiety in virtual environments,” in Joint Virtual Reality Conference eds K. Helin and M. D'Cruz (Nottingham: VTT Symposium), 80–82.
Liu, L., Yuan, L., and Wang, H. B. (2002). The human alpha(2A) – AR gene and the genotype of site−1296 and the susceptibility to motion sickness. Chin. J. Biochem. Biophys. 34, 291–297.
Luckey, P. (2019, March 25). I Can't Use Rift S, and Neither Can You. The Blog of Palmer Luckey. Available online at: http://palmerluckey.com/i-cant-use-rift-s-and-neither-can-you/ (accessed April 3, 2019).
Marcus, D. A., Furman, J. M., and Balaban, C. D. (2005). Motion sickness in migraine sufferers. Expert Opin. Pharmacother. 6, 2691–2697. doi: 10.1517/14656566.6.15.2691
McCauley, M. E., and Sharkey, T. J. (1992). Cybersickness: perception of self-motion in virtual environments. Presence 1, 311–318. doi: 10.1162/pres.1992.1.3.311
Meissner, K., Enck, P., Muth, E. R., Kellermann, S., and Klosterhalfen, S. (2009). Cortisol levels predict motion sickness tolerance in women but not in men. Physiol. Behav. 97, 102–106. doi: 10.1016/j.physbeh.2009.02.007
Mercier, O., Sulai, Y., Mackenzie, K., Zanolli, M., Hillis, J., Nowrouzezahrai, D., et al. (2017). Fast gaze-contingent optimal decompositions for multifocal displays. ACM Trans. Graphics 36:237. doi: 10.1145/3130800.3130846
Mon-Williams, M., Wann, J. P., and Rushton, S. (1993). Binocular vision in a virtual world: visual deficits following the wearing of a head-mounted display. Ophthalmic Physiol. Optics 13, 387–391. doi: 10.1111/j.1475-1313.1993.tb00496.x
Morris, C. (2018). Why Walmart and Other F500 Companies Are Using Virtual Reality to Train the Next Generation of American Workers. CNBC@Work. Available on at: https://www.cnbc.com/2018/10/29/why-f500-companies-use-virtual-reality-to-train-workers-of-the-future.html (accessed March 15, 2019).
Munafo, J., Diedrick, M., and Stoffregen, T. A. (2017). The virtual reality head-mounted display Oculus Rift induces motion sickness and is sexist in its effects. Exp. Brain Res. 235, 889–901. doi: 10.1007/s00221-016-4846-7
Murata, A. (2004). Effects of duration of immersion in a virtual reality environment on postural stability. Int. J. Hum. Comput. Interact. 17, 463–477. doi: 10.1207/s15327590ijhc1704_2
Napadow, V., Sheehan, J. D., Kim, J., Lacount, L. T., Park, K., Kaptchuk, T. J., et al. (2013). The brain circuitry underlying the temporal evolution of nausea in humans. Cereb. Cortex 23, 806–813. doi: 10.1093/cercor/bhs073
NPD Group (2014). 37 Percent of U.S. Population Age 9 and Older Currently Plays PC Games. NPD.com. Available online at: https://www.npd.com/wps/portal/npd/us/news/press-releases/37-percent-of-us-population-age-9-and-older-currently-plays-pc-games/ (accessed October 16, 2017).
Oman, C. M. (1998). Sensory conflict theory and space sickness: our changing perspective. J. Vestib. Res. 8, 51–56. doi: 10.3233/VES-1998-8107
Padmanaban, N., Konrad, R., Stramer, T., Cooper, E. A., and Wetzstein, G. (2017). Optimizing virtual reality for all users through gaze-contingent and adaptive focus displays. Proc. Natl. Acad. Sci. U.S.A. 114, 2183–2188. doi: 10.1073/pnas.1617251114
Paillard, A. C., Quarck, G., Paolino, F., Denise, P., Paolino, M., Golding, J. F., et al. (2013). Motion sickness susceptibility in healthy subjects and vestibular patients: effects of gender, age and trait-anxiety. J. Vestib. Res. 23, 203–210. doi: 10.3233/VES-130501
Park, A. H., and Hu, S. (1999). Gender differences in motion sickness history and susceptibility to optokinetic rotation-induced motion sickness. Aviat. Space Environ. Med. 70, 1077–1080.
Parker, D. E., and Harm, D. L. (1992). Mental rotation: a key to mitigation of motion sickness in the virtual environment? Presence 1, 329–333. doi: 10.1162/pres.1992.1.3.329
Parkman, H. P., Harris, A., Miller, M. A., and Fisher, R. S. (1996). Influence of age, gender, and menstrual cycle on the normal electrogastrogram. Am. J. Gastroenterol. 91, 127–133.
Parsons, T. D., Larson, P., Kratz, K., Thiebaux, M., Bluestein, B. J, Buckwalter, J. G., et al. (2004). Sex differences in mental rotation and spatial rotation in a virtual environment. Neuropsychologia 42, 555–562. doi: 10.1016/j.neuropsychologia.2003.08.014
Peli, E. (1999). “Optometric and perceptual issues with head-mounted displays,” in Optical Design for Visual Instrumentation, ed P. Mouroulis (New York, NY: McGraw-Hill), 205–276.
Priot, A.-E., Hourlier, S., Giraudet, G., Leger, A., and Roumes, C. (2006). “Hyperstereopsis in night vision devices: basic mechanisms and impact for training requirements,” in Defense and Security Symposium (Orlando, FL: International Society for Optics and Photonics), 62240N. doi: 10.1117/12.669332
Prothero, J. D., and Parker, D. E. (2003). “A unified approach to presence and motion sickness,” in Virtual and Adaptive Environments, eds L. J. Hettinger and M. W. Haas (Boca Raton, FL: Lawrence Erlbaum Publishers), 47–66. doi: 10.1201/9781410608888.ch3
Rasmussen, B. K., Jensen, R., Schroll, M., and Olesen, J. (1991). Epidemiology of headache in a general population - a prevalence study. J. Clin. Epidemiol. 44, 1147–1157. doi: 10.1016/0895-4356(91)90147-2
Rawat, N., Connor, C. W., Jones, J. A., Kozlovskaya, I. B., and Sullivan, P. (2002). The correlation between aerobic fitness and motion sickness susceptibility. Aviat. Space Environ. Med. 73, 216–218.
Reavley, C. M., Golding, J. F., Cherkas, L. F., Spector, T. D., and MacGregor, A. J. (2006). Genetic influences on motion sickness susceptibility in adult women: a classical twin study. Aviat. Space Environ. Med. 77, 1148–1152.
Regan, C. (1995). An investigation into nausea and other side-effects of head-coupled immersive virtual reality. Virtual Real. 1, 17–32. doi: 10.1007/BF02009710
Regan, E., and Price, K. (1993). Some Side-Effects of Immersion Virtual Reality: An Investigation Into the Relationship Between Inter-Pupillary Distance and Ocular Related Problems. Army Personnel Research Establishment Report 93R023. Army Operational Research Group.
Riccio, G. E., and Stoffregen, T. A. (1991). An ecological theory of motion sickness and postural instability. Ecol. Psychol. 3, 195–240. doi: 10.1207/s15326969eco0303_2
Robin, O., Vinard, H., Vernet-Maury, E., and Saumet, J. L. (1987). Influence of sex and anxiety on pain threshold and tolerance. Funct. Neurol. 2, 173–179.
Robinett, W., and Rolland, J. P. (1992). A computational model for the stereoscopic optics of a head-mounted display. Presence 1, 45–62. doi: 10.1162/pres.1992.1.1.45
Rohleder, N., Otto, B., Wolf, J. M., Klose, J., Kirschbaum, C., Enck, P., et al. (2006). Sex-specific adaptation of endocrine and inflammatory responses to repeated nauseogenic body rotation. Psychoneuroendocrinology 31, 226–236. doi: 10.1016/j.psyneuen.2005.07.004
Rolland, J., Ha, Y., and Fidopiastis, C. (2004). Albertian errors in head-mounted displays: I. Choice of eye-point location for a near-or far-field task visualization. J. Optic. Soc. Am. A 21, 901–912. doi: 10.1364/JOSAA.21.000901
Rolland, J. P., and Hua, H. (2005). “Head-mounted display systems,” in Encyclopedia of Optical Engineering, eds R. B. Johnson and R. G. Driggers (New York, NY: Taylor & Francis), 1–13.
Rolland, J. P., Krueger, M. W., and Goon, A. (2000). Multifocus planes head-mounted displays. Appl. Opt. 39, 3209–3215. doi: 10.1364/AO.39.003209
Samsung (2016). Samsung Gear VR Specifications. Available online at: https://www.samsung.com/global/galaxy/gear-vr/specs/ (accessed January 19, 2020).
Sandbakk, Ø., Ettema, G., Leirdal, S., and Holmberg, H.-C. (2012). Gender differences in the physiological responses and kinematic behaviour of elite sprint cross-country skiers. Eur. J. Appl. Physiol. 112, 1087–1094. doi: 10.1007/s00421-011-2063-4
Sandi, C., Cordero, M. I., Ugolini, A., Varea, E., Caberlotto, L., and Large, C. H. (2008). Chronic stress-induced alterations in amygdala responsiveness and behavior—modulation by trait anxiety and corticotropin-releasing factor systems. Eur. J. Neurosci. 28, 1836–1848. doi: 10.1111/j.1460-9568.2008.06451.x
Singh, P., and Shepherd, A. J. (2016). Enhanced motion after-effects in migraine are related to contrast sensitivity: implications for models of differences in precortical/cortical function. Invest. Ophthalmol. Vis. Sci. 57, 1228–1234. doi: 10.1167/iovs.15-17692
Skrbek, M., and Petrov, S. (2013). Small refractive errors – their correction and practical importance. Coll. Antropol. 37(Suppl. 1), 209–216. Available online at: https://pdfs.semanticscholar.org/2691/5145306628826caf94ddeb48a288f9e00011.pdf
Smart, L. J. Jr., Otten, E. W., Strang, A. J., Littman, E. M., and Cook, I. V. H. E. (2014). Influence of complexity and coupling of optic flow on visually induced motion sickness. Ecol. Psychol. 26, 301–324. doi: 10.1080/10407413.2014.958029
Spielberger, C. D., Gorsuch, R. L., and Lushene, R. E. (1970). The State-Trait Anxiety Inventory (Test Manual). Palo Alto, CA: Consulting Psychologists Press.
Stanney, K. M., and Hash, P. (1998). Locus of user-initiated control in virtual environments: influences on cybersickness. Presence Teleop. Virt. Environ. 7, 447–459. doi: 10.1162/105474698565848
Stanney, K. M., Kingdon, K., Nahmens, I., and Kennedy, R. S. (2003). What to expect from immersive virtual environment exposure: Influences of gender, body mass index, and past experience. Hum. Factors 45, 504–522. doi: 10.1518/hfes.45.3.504.27254
Stanney, K. M., Lanham, S., Kennedy, R. S., and Breaux, R. B. (1999). “Virtual environment exposure drop-out thresholds,” in 43rd Annual Human Factors and Ergonomics Society Meeting (Houston, TX), 1223–1227.
SteamVR (2016). What is The Deal With the Pupillary Distance Range? Available online at: https://steamcommunity.com/app/358040/discussions/0/357285562497028310/ (accessed January 19, 2020).
SteamVR (2018). SteamVR FAQ. Available online at: https://support.steampowered.com/kb_article.php?ref=7770-WRUP-5951&l=english (accessed January 19, 2020).
Stern, R. M., Hu, S., LeBlanc, R., and Koch, K. L. (1993). Chinese hyper-susceptibility to vection-induced motion sickness. Aviat. Space Environ. Med. 64, 827–830.
Stockhorst, U., Enck, P., and Klosterhalfen, S. (2007). Role of classical conditioning in learning gastrointestinal symptoms. World J. Gastroenterol. 13, 3430–3437. doi: 10.3748/wjg.v13.i25.3430
Strasburger, H., Rentschler, I., and Jüttner, M. (2011). Peripheral vision and pattern recognition: a review. J. Vis. 11, 13–13. doi: 10.1167/11.5.13
Szpak, A., Michalski, S. C., Saredakis, D., Chen, C. S., and Loetscher, T. (2019). Beyond feeling sick: the visual and cognitive aftereffects of virtual reality. PsyArXiv. 7, 130883–130892. doi: 10.31234/osf.io/xerwz
Tucker, G. J., and Reinhardt, R. F. (1967). Airsickness and anxiety. Aerosp. Med. 38, 855–858. doi: 10.1037/e445072004-001
Turner, M., and Griffin, M. J. (1999). Motion sickness in public road transport: passenger behaviour and susceptibility. Ergonomics 42, 444–461. doi: 10.1080/001401399185586
Turner, M., Griffin, M. J., and Holland, I. (2000). Airsickness and aircraft motion during short-haul flights. Aviat. Space Environ. Med. 71, 1181–1189.
VR Heads (2017). How to Manually Set Your IPD in PlayStation VR. Available online at: https://www.vrheads.com/how-manually-set-your-ipd-playstation-vr (accessed January 19, 2020).
Wang, J., Korczykowski, M., Rao, H., Fan, Y., Pluta, J., Gur, R. C., et al. (2007). Gender difference in neural response to psychological stress. Soc. Cogn. Affect. Neurosci. 2, 227–239. doi: 10.1093/scan/nsm018
Webb, N. A., and Griffin, M. J. (2003). Eye movement, vection, and motion sickness with foveal and peripheral vision. Aviat. Space Environ. Med. 74, 622–625.
Welch, R. B. (2014). “Adapting to virtual environments,” in Handbook of Virtual Environments: Design, Implementation, and Applications, 2nd Edn, eds K. S. Hale and K. M. Stanney (Boca Raton, FL: CRC Press), 627–646.
Williams, J. M., and Thirer, J. (1975). Vertical and horizontal peripheral vision in male and female athletes and nonathletes. J. Res. Q. 46, 200–205. doi: 10.1080/10671315.1975.10615324
Wilson, M. L., and Kinsela, A. J. (2017). “Absence of gender differences in actual induced HMD motion sickness vs. pretrial susceptibility ratings,” in 61st Annual Human Factors and Ergonomics Society Meeting (Austin, TX), 1313–1316.
Witkin, H. A., and Goodenough, D. R. (1977). Field dependence and interpersonal behavior. Psychol. Bull. 84, 661–689. doi: 10.1037/0033-2909.84.4.661
Keywords: virtual reality, cybersickness, gender differences, interpupillary distance, head mounted displays, motion sickness
Citation: Stanney K, Fidopiastis C and Foster L (2020) Virtual Reality Is Sexist: But It Does Not Have to Be. Front. Robot. AI 7:4. doi: 10.3389/frobt.2020.00004
Received: 03 June 2019; Accepted: 09 January 2020;
Published: 31 January 2020.
Edited by:
David Swapp, University College London, United KingdomReviewed by:
Xueni Pan, Goldsmiths University of London, United KingdomAnne-Emmanuelle Priot, Institut de Recherche Biomédicale des Armées (IRBA), France
Copyright © 2020 Stanney, Fidopiastis and Foster. This is an open-access article distributed under the terms of the Creative Commons Attribution License (CC BY). The use, distribution or reproduction in other forums is permitted, provided the original author(s) and the copyright owner(s) are credited and that the original publication in this journal is cited, in accordance with accepted academic practice. No use, distribution or reproduction is permitted which does not comply with these terms.
*Correspondence: Kay Stanney, a2F5JiN4MDAwNDA7ZGVzaWduaW50ZXJhY3RpdmUubmV0