- 1Social Determinants of Obesity and Cardiovascular Risk Laboratory, Cardiovascular Branch, Division of Intramural Research, National Heart, Lung, and Blood Institute, National Institutes of Health, Bethesda, MD, United States
- 2National Institutes of Health, Clinical Center, Bethesda, MD, United States
- 3Intramural Research Program, National Institute on Minority Health and Health Disparities, Bethesda, MD, United States
It is evident that health disparities exist during the COVID-19 pandemic, a pandemic caused by the novel coronavirus SARS-CoV-2. Underlying reasons for COVID-19 health disparities are multi-factorial. However, social determinants, including those regarding socioeconomic status, social inequalities, health behaviors, and stress, may have implications on these disparities. Exposure to one or more of these social determinants is associated with heightened inflammatory responses, particularly increases in the cytokine interleukin-6 (IL-6), as well as immune system dysfunction. Thus, an amplified effect during COVID-19 could occur, potentially resulting in vulnerable patients experiencing an intensified cytokine storm due to a hyperactive and dysfunctional immune response. Further understanding how social determinants play a mechanistic role in COVID-19 disparities could potentially help reduce health disparities overall and in future pandemics.
Introduction
As of August 26th 2020, more than 24 million people have positively tested for SARS-CoV-2, the virus responsible for the COVID-19 pandemic and more than 820,000 globally have died in this current COVID-19 pandemic, which is affecting people with underlying conditions more severely than others (1). In the United States, racial/ethnic disparities in COVID-19-related severity, mortality and outcomes exist. Although not the majority of cases, there is a disproportionate number of COVID-19-related deaths among Americans who are of Hispanic or African descent (2–5). Additionally, COVID-19 disproportionally affects ethnic minority communities in countries outside of the U.S. (6, 7). COVID-19 disparities have also been reported in the UK (8, 9), while the majority of countries have yet to publish data on race/ethnic-related disparities. Unfortunately, prior epidemics have also highlighted existing health disparities and prior discussions have emphasized the importance of incorporating anticipated health disparity outcomes into pandemic planning (10–13).
The underlying reasons for racial/ethnic health disparities are almost certainly multi-factorial and include disproportionate chronic disease prevalence, socioeconomic factors, as well as cultural and political influences. However, well-documented underlying factors are socioeconomic and discriminatory inequities at the individual, familial, and community levels (14–19).
Over the last 30 years, growing evidence in the literature has demonstrated that social determinants can induce a state of low-grade inflammation accompanied by a dysfunctional immune system in individuals experiencing adversities (20–22), but mechanistically, there is less work linking adverse psychosocial or environmental conditions (e.g., neighborhood deprivation, built and social environment) on a cellular and signaling pathway level. Additionally, very little is known about potential common dysregulated signaling pathways regarding immune cell dysfunction and inflammation between adverse psychosocial or neighborhood environmental conditions and other comorbidities known to affect COVID-19 severity. An understanding of the pathophysiology of COVID-19 requires an understanding of the interplay of SARS-CoV-2 virus with immune cells and inflammation which is slowly emerging in the literature (23). This understanding may guide therapeutics, translational studies, and multidisciplinary interventions that may mitigate some effects of COVID-19, while also elucidating mechanisms by which health disparities influence outcomes during pandemics and beyond for future targeted treatment. In this perspective, we present evidence of potential connections between the observed health disparities and COVID-19 progression/severity based on immune regulation and response, particularly from natural killer (NK) cells, monocyte/macrophages, and the cytokine, interleukin-6 (IL-6) (Figure 1). While we recognize that there are existing disparities in terms of SARS-CoV-2 susceptibility and detection, our intent with this perspective is to state that racial/ethnic health disparities in COVID-19 severity and mortality that are present in this pandemic may at least partially represent the biological consequences of social determinants of health, including socioeconomic and environmental inequities. Beyond pharmacologic therapies, behavioral interventions focused on reducing psychosocial stressors and accounting for existing social determinants in at-risk populations may help in mitigating the harmful immune and inflammatory responses.
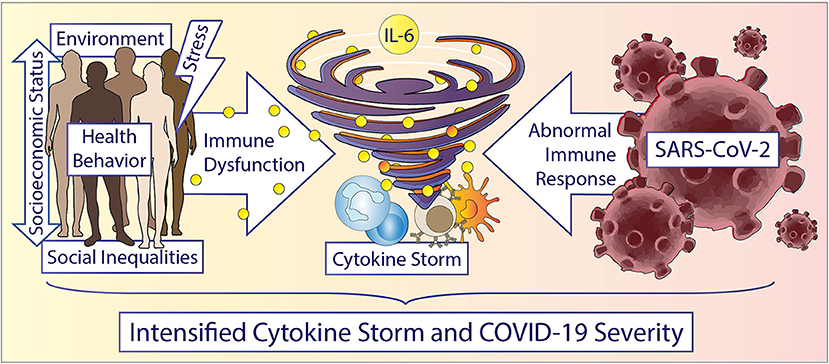
Figure 1. Accelerating the storm in vulnerable populations during COVID-19. Race/ethnicity, gender, and social determinants are important factors driving inflammation and immune cell dysfunction. Social determinants include but are not limited to: structural inequities, socioeconomic status, education, neighborhood environment, experiences of abuse, racism or other adversities, chronic stress, income, and education etc. Humans experiencing any of these social determinants can display increased inflammation, especially increased IL-6 levels and dysfunctional immune cells. Vulnerable patients potentially experience an accelerated cytokine storm during a COVID-19 infection due to a hyperactive and dysfunctional cytokine and immune response. Understanding the underlying cellular and immune related mechanisms in health disparities could potentially help in reducing disparate outcomes overall and in future pandemics.
COVID-19 Related Cytokine Storm: A System out of Balance
The body's immune system is a well-controlled and balanced interplay of various players, involving cellular components (immune cells) and circulating proteins with pro- and anti-inflammatory function (cytokines and chemokines) (24). The immune system detects and neutralizes foreign pathogens, including viruses like SARS-CoV-2. Two major subsystems of the immune system exist in many species: the adaptive vs. innate immune system. The adaptive immune system is acquired and able to adjust with time, recognizing pathogens more specifically by developing a memory after the first encounter with a pathogen. In contrast, the innate immune system serves as the active defense against a foreign pathogen. Upon encountering a pathogen, immune cells are recruited to the site of infection due to chemokines released by the infected tissue resident cells. Immune cells are further activated to provide an adequate response by various pro-and anti-inflammatory cytokines also released by the infected tissue, regulating immune cell function as well as immune cell interplay, coordinating healing and clearance of the pathogen (25–27).
Immune cells include, but are not limited to, neutrophils, T cells, B cells, NK cells, and monocytes (25, 27, 28). Neutrophils are the most abundant immune cell in the human body and important in the early stages of inflammation. T cells, B cells, and NK cells are also called lymphocytes. T cells are divided into many different subclasses and are major cytokine producers, which is important for coordination of the overall immune response; T cells also exhibit killing abilities of cancerous or virus-infected cells. As a part of the adaptive immune system, B cells are antibody producing and secreting cells. NK cells exhibit different mechanisms of detecting virus-infected or cancerous cells as compared to T cells, subsequently killing cells detected as “abnormal,” and are generally divided into two subclasses: (a) the proliferative NK cells activating additional parts of the immune system by cytokine secretion, or (b) the cytotoxic NK cell which release cytotoxic granules that mediate NK cell killing. Monocytes are phagocytosing immune cells and are responsible for “cleaning” debris derived from NK cell- or T cell-mediated killing of infected cells. Monocytes can then present parts of the engulfed pathogens to T cells, further activating an immune response for clearing the infection. Monocytes can also exit the circulation and transmigrate into surrounding tissues, clearing debris and supporting healing of the infected and inflamed tissue. Furthermore, monocytes differentiate into macrophages mainly responsible for cleaning up debris by phagocytosis mechanisms. Additionally, monocytes are present in three different subtypes: classical, non-classical and intermediate, with each of them having distinct functions.
Cytokines are a small group of proteins secreted by cells to communicate with each other, regulating proliferation, differentiation, inflammatory responses, immune cell activation and recruitment, as well as angiogenesis (29, 30). Among cytokines within the human body, interleukin (IL)-6 is probably one of the more complex as it exhibits pro- and anti-inflammatory properties and plays an important role in vascular disease, pathologic lipid metabolism, insulin resistance, and several chronic lung diseases (31, 32), all known risk factors for COVID-19 morbidity and mortality (1). Regarding COVID-19, Herold et al. (33) reported in a recent publication that the risk for respiratory failure in confirmed COVID-19 patients is higher in patients with IL-6 levels above 80 pg/ml when compared to patients with lower IL-6 levels. Herold et al. suggested that IL-6 levels could be used as a biomarker for disease progression. An earlier study reported that the detectable serum viral load of COVID-19 was closely associated with drastically increased IL-6 levels in the critically ill (34). Activation of a coordinated cytokine response, especially in the case of an acute infection, is crucial in activating immune cells and, therefore, fighting the infection. During SARS-CoV-2 infections, a “cytokine storm,” or an overactive inflammatory response of the immune system (35), can occur (30, 35, 36). But important questions come to mind: How and why are some patients experiencing this cytokine storm and others don't? What is known from epidemiologic research that puts COVID-19 patients at risk for experiencing a cytokine storm and could this help understand existing COVID-19 severity and mortality disparities?
While genetics are certainly postulated to play an important role for the development of a cytokine storm, we would like to highlight in this perspective that social position, racial discrimination, adverse neighborhood environment conditions, or experiences of individual-level adversity could potentially increase the risk of COVID-19 progression and mortality by altering immune cell function and existing inflammation levels, therefore altering the crucial balance needed for a coordinated immune system response. Due to prior work of our laboratory, this perspective will focus on the potential role of monocytes and NK cells at the intersection of social determinants and COVID-19 outcomes. Additionally, we chose to focus on the role of IL-6 over other cytokines as (a) IL-6 is consistently shown in the literature to be altered by psychosocial stress or environmental factors, and (b) its importance in COVID-19 severity is highly suggested based on available treatments. Certainly, while this perspective focuses on monocytes, NK cells, and IL-6, it is important to note that other immune cells and cytokines or chemokines are also key players in the body's coordinated response to infection, are affected by adverse psychosocial or environmental conditions, and hence warrant acknowledgment and future investigation.
Markers of Social Position and the Immune System
It is by now evident that racial/ethnic disparities in COVID-19 exist (4, 5, 14, 37) and there are indications that COVID-19 severity might differ depending on one's neighborhood deprivation and socioeconomic status (38–40). These studies highlight the importance to further understand the impact of social position on immune system function.
Amongst all immune cells, T cells, monocytes, and NK cells are taking a center stage in COVID-19 severity (41–44), recovery (45), and mortality (46), indicating that disturbed baseline T cell, monocyte or NK cell function might hinder adequate response to SARS-CoV-2 infection. In the past, our group and others have demonstrated racial/ethnic differences in immune cell distribution and cytokine levels (47–52). For instance, we and others showed shifts in monocyte subsets in non-Hispanic Blacks toward intermediate (CD14−/CD16+) and non-classical (CD14+/CD16+) monocytes accompanied by a decrease in classical monocytes (CD14+/CD16−) as compared to Caucasians. We hypothesize that this subset shift could relate to more severe COVID-19 outcomes in non-Hispanic Blacks, especially as the pro-inflammatory, non-classical (CD14+/CD16+) monocyte subset can play an important role in the inflammatory storm observed in severe COVID-19 (42) and has been postulated as potentially crucial in separating mild from severe COVID-19 cases (53). Additionally, we have recently demonstrated in a small hypothesis-generating study that the NK cell profile of non-Hispanic Black blood donors differs significantly from that of Caucasian blood donors (47). Overall NK cell proportions were increased in non-Hispanic Black blood donors. While increasing NK cell numbers might sound like an enhancement of immune cell function, the opposite is true when additional NK cell subset changes or functional changes are present. We found decreased levels of “cytotoxic” NK cells (CD56hi/CD16dim) and increased levels of “proliferative” NK cells (CD56dim/CD16hi) within the overall NK cell populations among non-Hispanic Black as compared to Caucasian blood donors (47). This shift in NK cell subsets is indicative of a loss of NK cell “killing” ability and could potentially be linked to a delayed or decreased response to an acute viral infection, like SARS-CoV-2.
A study published in Science in 2016 elegantly displayed how unequal social status with subsequent harassment during social interactions altered T-helper cells and NK cells (54); although this study was conducted in rhesus macaques and translation to humans has to be determined, these findings could potentially be of crucial importance for COVID-19 where T cells are particularly affected during the immune response in severe COVID-19 cases (42, 44, 55). Interestingly, socioeconomic differences in early life seem to drive epigenetic aging and DNA methylation of monocytes and therefore, alter monocyte functionality, potentially contributing to future health disparities (56, 57). In children with asthma, a risk factor for more severe COVID-19 disease, lower socioeconomic status (SES) was accompanied with worse quality of life and a shift of immune cell function toward more inflammatory monocytes and altered T-helper cell responses when compared to children of higher SES with asthma (58). Socioeconomic position across the life course also appears to be of crucial importance in shaping the immune cell landscape, with lower socioeconomic position across the life course being associated with greater baseline inflammation in adulthood (59). During COVID-19 progression, exaggerated baseline inflammation and a dysfunctional immune system could lead to an inadequate and, therefore, less effective immune response. This could partially explain increased COVID-19 severity and mortality in individuals experiencing disadvantages across the life course.
Leukocyte telomere length has been linked to immune cell dysfunction, various chronic diseases (60), and psychosocial stress [as summarized in (61)]; telomere length has been suggested as a new tool for immunoepigenetics (62). A recent study from our lab using data from the National Health and Nutrition Examination Survey demonstrated that living in socioeconomically disadvantaged neighborhoods associated with shorter leukocyte telomere length among U.S. adults, indicating the potential impact of adverse neighborhood conditions on immune cells (63). It is important to note that leukocyte telomere length has also been reported to have inconclusive results for some diseases (64) and certainly warrants future research as racial/ethnic differences have been shown when comparing telomere length and/or telomere shortening rate of Blacks to those of Whites (65–67). Overall, urgent studies are needed to investigate cellular signaling mechanisms by which markers of social position, including socioeconomic position and the neighborhood environment of individuals (68), shape the transcriptional identity of immune cells (69) to further understand the disparities in COVID-19 outcomes and potential targets for therapeutic intervention.
Racial/ethnic differences in IL-6 levels have been reported. IL-6 levels are higher in non-Hispanic Blacks when compared to Hispanics or non-Hispanic Whites after age adjustment (70). While this study did not adjust for gender, an impact of gender in IL-6 response to stressors has been reported (71), but evidence in larger studies is sparse. In another study, Blacks displayed higher IL-6 even after adjustment for socioeconomic factors (48). IL-6 is also the major cytokine associated with chronic psychosocial and environmental stress in epidemiologic studies. For example, the list of factors reported to increase circulating IL-6 levels include lower SES (72–74), higher levels of neighborhood deprivation, and lower levels of neighborhood safety (75). Additionally, elevated IL-6 levels are present in older adults living in communities with greater poverty and racial segregation (76). In another study, no baseline differences in circulating IL-6 levels in individual of lower or higher SES were found, but a prolonged and accelerated plasma IL-6 increase was observed when individuals of lower SES were subjected to an acute stressor (77). When Azad et al. analyzed the IL-6 release of stimulated PBMCs of children of various SES levels, they reported that PBMCs from those with lower SES displayed an increase of IL-6 release twice as high as PBMCs from children with higher SES, indicating excessive IL-6 production may be dependent on SES (78). These relationships between lower SES and increased inflammation in adulthood have been reported in other studies as well (59). Early childhood stressors can include exposure to poverty, war, and systemic racism, or lower education while an adulthood stressor could certainly include the COVID-19 pandemic. This indicates that COVID-19 patients who have previously experienced poverty, and/or institutional racism, especially at a young age (79), might react to COVID-19 with an overproduction of cytokines, including IL-6, accelerating the cytokine storm and worsening disease prognosis.
Individual-Level Adversities and the Immune System
Individual-level markers of adversity include, amongst others, experiences of abuse, bereavement, relationship stress, limited social cohesion, loneliness, or sleep deprivation and have partially been linked to markers of social position and vice versa (80–84).
Epidemiologic data suggest relationships between the function of NK cell, monocyte/macrophage, and T cell populations and chronic psychosocial stress. For example, multiple psychosocial stressors like bereavement, relationship stress, depression, and generalized chronic mental stress are associated with a loss in NK cell function (85). A study focusing on loneliness found that NK cell numbers as determined by CD56/CD16 positive NK cells in flow cytometry decrease with increasing levels of loneliness (86). A potential source of confusion when examining the literature might be that markers of lower social status or adversity may correlate with higher numbers of overall immune cells. For example, the number of circulating NK cells overall has been demonstrated to increase in situations of acute mental stress or depression (87). In future studies, it will be important to expand the phenotypic characterization of immune cell subtypes and to measure actual immune cell function, as a simple increase of a particular immune cell can still be accompanied by a decrease in important subsets of cells with a required specific function (i.e., attacking a virally infected cell).
When PBMCs were isolated from middle-aged women with various levels of physical assault history, in vitro production of IL-6 upon stimulation was significantly associated with the level of assault experienced (88). Similar results were observed for individuals who have experienced stalking (88), indicating a dysfunctional immune system in women experiencing assault. Sleep deprivation as well as loneliness (89) have also been demonstrated to alter immune cell IL-6 production, further strengthening the evidence that individual-level adversity alters immune cell function and hence could predispose an individual to greater risk for increasing COVID-19 severity (90).
Social cohesion is another factor of importance particularly in times of social distancing. Social cohesion has been shown to be accompanied by decreasing IL-6 levels, especially amongst African American women (91). Additionally, increasing social support in parents of children with cancer has been demonstrated to attenuate glucocorticoid resistance and subsequent IL-6 production, while no impact of social support could be found regarding TNFα or IL-1β (92). Furthermore, hopelessness in adolescent girls in the setting of bullying was also shown to be associated with increased IL-6 levels (93). During a pandemic, it is likely that anxiety levels, hopelessness, and psychosocial distress in individuals are increasing. Anxiety is a psychosocial factor shown to associate with increased IL-6 levels (94) independent of depressive symptoms (95), potentially further accelerating COVID-19 severity.
Interestingly, it has also been shown that increased IL-6 levels can be found in night shift workers when compared to day shift workers, while CRP, a general marker of inflammation often measured in the clinical setting did not reveal significant differences (96). Higher IL-6 levels were also detected with sleep deprivation and fatigue (97), bullying (93), or sexual abuse (98). In non-Hispanic Black women, higher IL-6 levels were found in those anticipating racism threats (99), with perceived lifetime discrimination (100), or experiencing ongoing racial discrimination (101). Intriguingly, there is growing evidence that stress in childhood accelerates IL-6 production when exposed to an acute stressor in adulthood, indicating that childhood stress may manifest in adults as accelerated IL-6 responses to acute stressors (102).
These findings highlight the importance of analyzing a wide variety of cytokines to determine the impact of “stressors” on health and in health disparities, and to potentially identify a few cytokines which could be useful as biomarkers in clinical practice estimating an individual's risk for adverse health outcomes.
Behavioral Interventions Impacting IL-6 Levels and the Immune System
Several interventions have been shown to decrease IL-6 levels; in particular, behavioral interventions around physical activity, diet, and behavioral approaches to stress could be of benefit by potentially dampening the IL-6 production and other cytokine changes in COVID-19 progression. Dietary behaviors and physical activity, in particular, may serve to provide a two-way approach to combat health disparities during the COVID-19 pandemic, principally through their ability to prevent underlying chronic diseases but also through potential protective changes in the immune and inflammatory responses. In older adults, increasing exercise levels long term has been beneficial in decreasing IL-6 levels (103), a finding supported by other studies (104), which highlights the importance of long term regular exercise, even in times of a pandemic. In a study focusing on acute psychosocial stress, increased self-compassion was also accompanied by a decrease in the IL-6 response (105). Another study explored monocyte subsets and inflammatory markers in connection with healthy diet habits. The authors found that adherence to nutritional recommendations resulted in lower levels of inflammatory markers and non-classical monocytes. Moreover, a study by Mayr et al. demonstrated that altering diet by reducing the dietary inflammatory index correlated with a significant reduction in serum IL-6 levels after 6 months of dietary change (106). Several interventions have also been shown to alter NK cells; for instance, daily nature walks have been shown to increase NK cell numbers and functionality (107) and support for psychosocial well-being increases NK cell cytotoxicity (108). As mentioned above, social cohesion is a critical component affecting the immune system, indicating the potential importance of online or mobile health support groups even in times of necessary social distancing.
Exploring the role of behavioral interventions may also provide insight into the connection between the immune system, inflammation and the contextual environmental and extrinsic factors that underlie health disparities. For example, the presence of low-grade inflammation in young adults experiencing racism was shown to be less extensive if a positive racial identity was present (79).
Diabetes itself is linked to higher circulating IL-6 levels, and in turn, diet has been linked to IL-6 as well. The connection between the two seems obvious and could potentially be a part of the reason why certain diseases in vulnerable populations lead to worse COVID-19 outcomes. Healthy food choices are also influenced by multiple parameters like socioeconomic status or one's neighborhood environment (109), with most predominantly Black urban neighborhoods having limited fresh food access. As an example of the potential confluence of stress, food access, and racism affecting health behavior that influences chronic disease, non-Hispanic Black women with diabetes reported that experienced racism led to maladaptive coping strategies which included unhealthy food choices and that these choices affected diabetes self-management (91, 110). Lastly, interventions should certainly incorporate the element of hope, as hope, optimism, and happiness are essential components to human health and have been shown to be accompanied by decreasing IL-6 levels (111, 112). This might seem like a list of unbreakable, vicious cycles pre-dispositioning vulnerable populations to COVID-19. However, this is not the intention of this perspective article. Especially in times of social distancing, small behavioral interventions to promote healthy diet, physical activity, or reduce stress might make a difference in COVID-19 outcomes.
Conclusion
In conclusion, we must consider how we can address social determinants of health that promote psychosocial stress and disparate outcomes regarding disease severity and mortality in COVID-19. There is clear evidence that social disadvantages and stressors experienced in early life will shape the immune cell function and cytokine levels across the life course. The COVID-19 pandemic highlights several important questions to consider. Could anti-inflammatory treatments, especially clinical trials targeting IL-6, the IL-6 receptor, or the IL-6 tyrosine kinase signaling pathway, be of potential benefit especially for the most vulnerable in our society? How can we guarantee clinical trial access to minority-serving institutions and clinical providers around the country to ensure that study participants of all races/ethnicities and socioeconomic backgrounds are enrolled in these critical COVID-19 related clinical trials? Can we phenotype immune cells and/or determine cytokine levels in bio-banked samples from existing clinical trials or health care system cohorts and combine these data with electronic health record data or publicly accessible data to evaluate the impact of social determinants on underlying signaling pathways potentially accelerating poor COVID-19 outcomes? Can we leverage existing epidemiological cohort studies or behavioral interventions to identify participants that have been affected by COVID-19 and investigate factors contributing to COVID-19 health disparities by analyzing participants' disease progression and outcomes in relation to psychosocial stressors, neighborhood environment, health behaviors, chronic diseases or inflammatory markers? Some of this work has already begun with epidemiologic cohorts like the Multiethnic Study of Atherosclerosis and the Nurses' Health Study.
Looking ahead to the months and years to come, we need to consider more interdisciplinary approaches to evaluate cellular signaling pathways involved in disparate outcomes in COVID-19 that may serve as intervention targets. This work may also elucidate important targets for reducing racial/ethnic health disparities in chronic health conditions. Future clinical trials need to incorporate rigorous qualitative methodologies and validated quantitative questionnaires regarding study participants' background like socio-demographics, address data to link with data on neighborhood traits, and questions about adverse early childhood and life experiences, including psychosocial stressors. Behavioral interventions should be designed to gather phenotypic data and understand cellular pathways that may be most impacted by changes in health behavior. Evaluation of these data could add tremendously to understanding the contribution of social determinants of health to clinical outcomes and how their contribution might be attenuated by intervention. Certainly, it would also be desirable if epidemiological studies and behavioral interventions could incorporate a more detailed translational research component by detecting cytokine levels, immune cell phenotypes, immune cell function, and the underlying cellular signaling pathways, to potentially identify common regulators between diseases allowing for better development of treatment strategies or interventional therapies. While several epidemiologic studies have been conducted over the last 30 years that include translational research, it will be important to incorporate deeper immune cell phenotyping, characterization of immune cell functionality, and larger cytokine profiling across epidemiologic studies for data harmonization that allow for inter-study comparison. For basic research, it will be particularly important to address basic research questions regarding the impact of stress and stressors on cellular function especially in the most vulnerable patients affected by health disparities. A more intense focus should involve psychoneuroimmunology (113–115) to further identify underlying mechanisms and signaling pathways. As none of us can be an expert in every field, interdisciplinary collaborations are of crucial importance to make this kind of in-depth, overarching research feasible and to potentially better understand the underlying mechanisms for health disparities.
In this perspective we have highlighted inflammation and immune cell changes as potential mediators of the relationships between exposure to adverse social conditions and COVID-19 severity and outcomes. It is known that existing inequities, may lead to the disproportionate burden of chronic diseases, such as asthma (116–118), Type 2 diabetes (119, 120), obesity (121–126), and cardiovascular disease (127–129). In turn, these chronic diseases could mediate associations between social factors and worsening COVID-19 severity and outcomes (1, 130). However, while we believe that comorbidities are of great importance in understanding COVID-19 progression, formal mediation analyses in epidemiologic studies can begin to determine whether biomarkers of immune system alterations and inflammation may mediate associations between social conditions and COVID-19 severity and outcomes, independent of comorbid conditions. This epidemiologic work could provide direction to translational research to further understand the underlying mechanisms by which immune cell changes and inflammation might be altered by social determinants.
In the future, it will be important for countries to make data regarding racial/ethnic disparities available and accessible to the scientific community to allow further understanding of the impact of social determinants of health on COVID-19 progression, severity, and mortality. Furthermore, this type of data could be utilized to determine the impact of existing health care systems, access to healthcare, testing and treatment, as well as population structure on health disparities, ultimately allowing for improved future strategic planning in pandemics.
The need to work together as a world team of science is now more crucial than ever to understand COVID-19 progression in the most vulnerable, giving hope for the future of patient care and treatment development. Hope is essential to make it through this pandemic and its tragic consequences and for us to build a world where future pandemics are not only less likely but less disparate in their impact.
Data Availability Statement
The original contributions presented in the study are included in the article/supplementary material, further inquiries can be directed to the corresponding author/s.
Author Contributions
YB and TP-W conceptualized and wrote the article. NF and GW critically reviewed and revised the article. TP reviewed the article and developed the graphical abstract. All authors read the article and agreed to its submission.
Funding
The Social Determinants of Obesity and Cardiovascular Risk Laboratory was funded by Division of Intramural Research of the National Heart, Lung, and Blood Institute and Intramural Research Program of the National Institute on Minority Health and Health Disparities with the grant numbers ZIA HL006168, ZIA HL006225, and ZIA HL006252. The statements and contents expressed in this perspective of those of the authors and do not reflect the official position of the NIH, DHHS, and/or the US Government.
Conflict of Interest
The authors declare that the research was conducted in the absence of any commercial or financial relationships that could be construed as a potential conflict of interest.
Acknowledgments
We'd like to thank all the health care workers, first responders, and their support personnel fighting COVID-19 at the forefront of our hospitals. Additionally, we'd like to thank everyone in the essential businesses keeping our society moving in these times of crisis.
References
1. Team CCR. Preliminary estimates of the prevalence of selected underlying health conditions among patients with coronavirus disease 2019. MMWR Morb Mortal Wkly Rep. (2020) 69:382–6. doi: 10.15585/mmwr.mm6913e2
2. Johnson A, Buford T. Early data shows african americans have contracted and died of coronavirus at an alarming rate. Propublica. (2020).
3. Kendi I. What the racial data show. The pandemic seems to be hitting people of color the hardest. The Atlantic. (2020).
4. Milam AJ, Furr-Holden D, Edwards-Johnson J, Webb B, Patton JW, Ezekwemba NC, et al. Are clinicians contributing to excess African American COVID-19 deaths? Unbeknownst to them, they may be. Health Equity. (2020) 4:139–41. doi: 10.1089/heq.2020.0015
6. Kirby T. Evidence mounts on the disproportionate effect of COVID-19 on ethnic minorities. Lancet Respir Med. (2020) 8:547–8. doi: 10.1016/S2213-2600(20)30228-9
7. Pan D, Sze S, Minhas JS, Bangash MN, Pareek N, Divall P, et al. The impact of ethnicity on clinical outcomes in COVID-19: a systematic review. EClinicalMedicine. (2020) 23:100404. doi: 10.1016/j.eclinm.2020.100404
8. White C, Nafilyan V. Coronavirus (COVID-19) Related Deaths by Ethnic Group, England and Wales. United Kingdom: Office for National Statistics (2020).
9. Lassale C, Gaye B, Hamer M, Gale CR, Batty GD. Ethnic disparities in hospitalisation for COVID-19 in England: the role of socioeconomic factors, mental health, and inflammatory and pro-inflammatory factors in a community-based cohort study. Brain Behav Immun. (2020) 88:44–49. doi: 10.1016/j.bbi.2020.05.074
10. Quinn SC, Kumar S. Health inequalities and infectious disease epidemics: a challenge for global health security. Biosecur Bioterror. (2014) 12:263–73. doi: 10.1089/bsp.2014.0032
11. Blumenshine P, Reingold A, Egerter S, Mockenhaupt R, Braveman P, Marks, et al. Pandemic influenza planning in the United States from a health disparities perspective. Emerg Infect Dis. (2008) 14:709–15. doi: 10.3201/eid1405.071301
12. Oramasionwu CU, Brown CM, Ryan L, Lawson KA, Hunter JM, Frei, et al. HIV/AIDS disparities: the mounting epidemic plaguing US blacks. J Natl Med Assoc. (2009) 101:1196–204. doi: 10.1016/S0027-9684(15)31130-5
13. Quinn SC, Kumar S, Freimuth VS, Musa D, Casteneda-Angarita N, Kidwell, et al. Racial disparities in exposure, susceptibility, and access to health care in the US H1N1 influenza pandemic. Am J Public Health. (2011) 101:285–93. doi: 10.2105/AJPH.2009.188029
14. Webb Hooper M, Napoles AM, Perez-Stable EJ. COVID-19 and racial/ethnic disparities. JAMA. (2020) 323:2466–7. doi: 10.1001/jama.2020.8598
15. Perez-Escamilla R. Health disparities. In: Caballero B, editor. Encyclopedia of Human Nutrition. 3rd Edn. (New Haven, CT: Elsevier) (2013). p. 417–23. doi: 10.1016/B978-0-12-375083-9.00142-2
16. National Academies of Sciences, Engineering, and Medicine Health and Medicine Division, Board on Population Health and Public Health Practice, Committee on Community-Based Solutions to Promote Health Equity in the United States. Communities in Action: Pathways to Health Equity. Baciu A, Negussie Y, Geller A, Weinstein JN, editors. Washington, DC: National Academies Press (US) (2017).
17. Abercrombie DD. Chapter 43 - Health Disparities. In Physician Assistant. Ballweg R, Sullivan EM, Brown D, Vetrosky D, editors. Philadelphia, PA: W.B. Saunders (2008). p. 739–48.
18. Egede LE, Walker RJ. Structural racism, social risk factors, and Covid-19 — a dangerous convergence for black Americans. N Engl J Med. (2020). doi: 10.1056/NEJMp2023616. [Epub ahead of print].
19. Singu S, Acharya A, Challagundla K, Byrareddy SN. Impact of social determinants of health on the emerging COVID-19 pandemic in the United States. Front Public Health. (2020) 8:406. doi: 10.3389/fpubh.2020.00406
20. Egger G, Dixon J. Beyond obesity and lifestyle: a review of 21st century chronic disease determinants. Biomed Res Int. (2014) 2014:731685. doi: 10.1155/2014/731685
21. Aiello AE, Kaplan GA. Socioeconomic position and inflammatory and immune biomarkers of cardiovascular disease: applications to the panel study of income dynamics. Biodemography Soc Biol. (2009) 55:178–205. doi: 10.1080/19485560903382304
22. Sterling P, Eyer J. Biological basis of stress-related mortality. Soc Sci Med E. (1981) 15:3–42. doi: 10.1016/0271-5384(81)90061-2
23. St. John AL, Rathore APS. Early insights into immune responses during COVID-19. J Immunol. (2020) 205:555–64. doi: 10.4049/jimmunol.2000526
25. Goldman A, Prabhakar B. Immunology overview. In: editor, Baron S. Medical Microbiology, 4 ed. Galveston, TX: University of Texas Medical Branch at Galveston (1996). p. 1–33.
26. Justiz Vaillant AA, Jan A. Physiology, Immune Response. [Updated 2020 Aug 16]. In: StatPearls [Internet]. Treasure Island, FL: StatPearls Publishing (2020).
28. Abbas A, Lichtman A, Pilai S. Cellular and Molecular Immunology. Philadelphia, PA: Elsevier (2017).
29. Akdis M, Aab A, Altunbulakli C, Azkur K, Costa RA, Crameri R, et al. A. Interleukins (from IL-1 to IL-38), interferons, transforming growth factor beta, and TNF-alpha: Receptors, functions, and roles in diseases. J Allergy Clin Immunol. (2016) 138:984–1010. doi: 10.1016/j.jaci.2016.06.033
30. Tisoncik JR, Korth MJ, Simmons CP, Farrar J, Martin TR, Katze, et al. Into the eye of the cytokine storm. Microbiol Mol Biol Rev. (2012) 76:16–32. doi: 10.1128/MMBR.05015-11
31. Rubini A. Interleukin-6 and lung inflammation: evidence for a causative role in inducing respiratory system resistance increments. Inflamm Allergy Drug Targets. (2013) 12:315–21. doi: 10.2174/1871528111312050003
32. Hunter CA, Jones SA. IL-6 as a keystone cytokine in health and disease. Nat Immunol. (2015) 16:448–57. doi: 10.1038/ni.3153
33. Herold T, Jurinovic V, Arnreich C, Lipworth BJ, Hellmuth JC, Von Bergwelt-Baildon M, et al. Elevated levels of IL-6 and CRP predict the need for mechanical ventilation in COVID-19. J Allergy Clin Immunol. (2020) 146:128–36.e24. doi: 10.1016/j.jaci.2020.05.008
34. Chen X, Zhao B, Qu Y, Chen Y, Xiong J, Feng Y, et al. Detectable serum SARS-CoV-2 viral load (RNAaemia) is closely correlated with drastically elevated interleukin 6 (IL-6) level in critically ill COVID-19 patients. Clin Infect Dis. (2020). doi: 10.1101/2020.02.29.20029520. [Epub ahead of print].
35. Zhang C, Wu Z, Li JW, Zhao H, Wang GQ. The cytokine release syndrome (CRS) of severe COVID-19 and Interleukin-6 receptor (IL-6R) antagonist Tocilizumab may be the key to reduce the mortality. Int J Antimicrob Agents. (2020) 55:105954. doi: 10.1016/j.ijantimicag.2020.105954
36. Hu B, Huang S, Yin L. The cytokine storm and COVID-19. J Med Virol. (2020). doi: 10.1002/jmv.26232. [Epub ahead of print].
37. Krouse HJ. COVID-19 and the widening gap in health inequity. Otolaryngol Head Neck Surg. (2020) 163:65–6. doi: 10.1177/0194599820926463
38. Prats-Uribe A, Paredes R, Prieto-Alhambra D. Ethnicity, comorbidity, socioeconomic status, and their associations with COVID-19 infection in England: a cohort analysis of UK Biobank data. medRxiv. (2020). doi: 10.1101/2020.05.06.20092676
39. Emeruwa UN, Ona S, Shaman JL, Turitz A, Wright JD, Gyamfi-Bannerman C, et al. Associations between built environment, neighborhood socioeconomic status, and SARS-CoV-2 infection among pregnant women in New York City. JAMA. (2020) 324:390–2. doi: 10.1001/jama.2020.11370
40. Williamson EJ, Walker AJ, Bhaskaran K, Bacon S, Bates C, Morton CE, et al. Factors associated with COVID-19-related death using OpenSAFELY. Nature. (2020) 584:430–6. doi: 10.1038/s41586-020-2521-4
41. Wang F, Nie J, Wang H, Zhao Q, Xiong Y, Deng L, et al. Characteristics of peripheral lymphocyte subset alteration in COVID-19 pneumonia. J Infect Dis. (2020) 221:1762–9. doi: 10.1093/infdis/jiaa150
42. Wei H, Xu X, Tian Z, Sun R, Qi Y, Zhao C, et al. Pathogenic T-cells and inflammatory monocytes incite inflammatory storms in severe COVID-19 patients. Natl Sci Rev. (2020) 7:998–1002. doi: 10.1093/nsr/nwaa041
43. Maucourant C, Filipovic I, Ponzetta A, Aleman S, Cornillet M, Hertwig L, et al. Natural killer cell immunotypes related to COVID-19 disease severity. Sci Immunol. (2020) 5:eabd6832. doi: 10.1126/sciimmunol.abd6832
44. Diao B, Wang C, Tan Y, Chen X, Liu Y, Ning L, et al. Reduction and functional exhaustion of t cells in patients with coronavirus disease 2019 (COVID-19). Front Immunol. (2020) 11:827. doi: 10.3389/fimmu.2020.00827
45. Wen W, Su W, Tang H, Le W, Zhang X, Zheng Y, et al. Immune cell profiling of COVID-19 patients in the recovery stage by single-cell sequencing. Cell Discov. (2020) 6:31. doi: 10.1038/s41421-020-00187-5
46. Wang F, Hou H, Yao Y, Wu S, Huang M, Ran X, et al. Systemically comparing host immunity between survived and deceased COVID-19 patients. Cell Mol Immunol. (2020) 17:857–77. doi: 10.1038/s41423-020-0483-y
47. Baumer Y, Gutierrez-Huerta CA, Saxena A, Dagur PK, Langerman SD, Tamura K, et al. Immune cell phenotyping in low blood volumes for assessment of cardiovascular disease risk, development, and progression: a pilot study. J Transl Med. (2020) 18:29. doi: 10.1186/s12967-020-02207-0
48. Paalani M, Lee JW, Haddad E, Tonstad S. Determinants of inflammatory markers in a bi-ethnic population. Ethn Dis. (2011) 21:142–9.
49. Hsieh MM, Everhart JE, Byrd-Holt DD, Tisdale JF, Rodgers GP. Prevalence of neutropenia in the US population: age, sex, smoking status, and ethnic differences. Ann Intern Med. (2007) 146:486–92. doi: 10.7326/0003-4819-146-7-200704030-00004
50. Bain BJ. Ethnic and sex differences in the total and differential white cell count and platelet count. J Clin Pathol. (1996) 49:664–6. doi: 10.1136/jcp.49.8.664
51. Kurupati R, Kossenkov A, Haut L, Kannan S, Xiang Z, Li Y, et al. Race-related differences in antibody responses to the inactivated influenza vaccine are linked to distinct pre-vaccination gene expression profiles in blood. Oncotarget. (2016) 7:62898–911. doi: 10.18632/oncotarget.11704
52. Appleby LJ, Nausch N, Midzi N, Mduluza T, Allen JE, Mutapi, et al. Sources of heterogeneity in human monocyte subsets. Immunol Lett. (2013) 152:32–41. doi: 10.1016/j.imlet.2013.03.004
53. Silvin A, Chapuis N, Dunsmore G, Goubet AG, Dubuisson A, Derosa L, et al. Elevated calprotectin and abnormal myeloid cell subsets discriminate severe from Mild COVID-19. Cell. (2020) 182:1401–18. doi: 10.1016/j.cell.2020.08.002
54. Snyder-Mackler N, Sanz J, Kohn JN, Brinkworth JF, Morrow S, Shaver AO, et al. Social status alters immune regulation and response to infection in macaques. Science. (2016) 354:1041–5. doi: 10.1126/science.aah3580
55. Chen G, Wu D, Guo W, Cao Y, Huang D, Wang H, et al. Clinical and immunological features of severe and moderate coronavirus disease 2019. J Clin Invest. (2020) 130:2620–9. doi: 10.1172/JCI137244
56. Austin MK, Chen E, Ross KM, Mcewen LM, Maclsaac JL, Kobor MS, et al. Early-life socioeconomic disadvantage, not current, predicts accelerated epigenetic aging of monocytes. Psychoneuroendocrinology. (2018) 97:131–4. doi: 10.1016/j.psyneuen.2018.07.007
57. Needham BL, Smith JA, Zhao W, Wang X, Mukherjee B, Kardia SL, et al. Life course socioeconomic status and DNA methylation in genes related to stress reactivity and inflammation: The multi-ethnic study of atherosclerosis. Epigenetics. (2015) 10:958–69. doi: 10.1080/15592294.2015.1085139
58. Miller GE, Chen E, Shalowitz MU, Story RE, Leigh AKK, Ham P, et al. Divergent transcriptional profiles in pediatric asthma patients of low and high socioeconomic status. Pediatr Pulmonol. (2018) 53:710–9. doi: 10.1002/ppul.23983
59. Berger E, Castagne R, Chadeau-Hyam M, Bochud M, D'errico A, Gandini M, et al. Multi-cohort study identifies social determinants of systemic inflammation over the life course. Nat Commun. (2019) 10:773. doi: 10.1038/s41467-019-08732-x
60. Weng NP. Telomeres and immune competency. Curr Opin Immunol. (2012) 24:470–5. doi: 10.1016/j.coi.2012.05.001
61. Rentscher KE, Carroll JE, Mitchell C. Psychosocial stressors and telomere length: a current review of the science. Ann Rev Public Health. (2020) 41:223–45. doi: 10.1146/annurev-publhealth-040119-094239
62. Melicher D, Buzas EI, Falus A. Genetic and epigenetic trends in telomere research: a novel way in immunoepigenetics. Cell Mol Life Sci. (2015) 72:4095–109. doi: 10.1007/s00018-015-1991-2
63. Powell-Wiley TM, Gebreab SY, Claudel SE, Ayers C, Andrews MR, Adu-Brimpong J, et al. The relationship between neighborhood socioeconomic deprivation and telomere length: The 1999-2002 national health and nutrition examination survey. SSM Popul Health. (2020) 10:100517. doi: 10.1016/j.ssmph.2019.100517
64. Smith L, Luchini C, Demurtas J, Soysal P, Stubbs B, Hamer M, et al. Telomere length and health outcomes: an umbrella review of systematic reviews and meta-analyses of observational studies. Ageing Res Rev. (2019) 51:1–10. doi: 10.1016/j.arr.2019.02.003
65. Rewak M, Buka S, Prescott J, De Vivo I, Loucks EB, Kawachi I, et al. Race-related health disparities and biological aging: does rate of telomere shortening differ across blacks and whites? Biol Psychol. (2014) 99:92–9. doi: 10.1016/j.biopsycho.2014.03.007
66. Savage SA. Beginning at the ends: telomeres and human disease. F1000Res. (2018) 7:F1000. doi: 10.12688/f1000research.14068.1
67. Hunt SC, Chen W, Gardner JP, Kimura M, Srinivasan SR, Eckfeldt JH, et al. Leukocyte telomeres are longer in African Americans than in whites: the national heart, lung, and blood institute family heart study and the bogalusa heart study. Aging Cell. (2008) 7:451–8. doi: 10.1111/j.1474-9726.2008.00397.x
68. Carr EJ, Dooley J, Garcia-Perez JE, Lagou V, Lee JC, Wouters C, et al. The cellular composition of the human immune system is shaped by age and cohabitation. Nat Immunol. (2016) 17:461–8. doi: 10.1038/ni.3371
69. Noren Hooten N, Evans MK. Age and poverty status alter the coding and noncoding transcriptome. Aging. (2019) 11:1189–203. doi: 10.18632/aging.101823
70. Stowe RP, Peek MK, Cutchin MP, Goodwin JS. Plasma cytokine levels in a population-based study: relation to age and ethnicity. J Gerontol A Biol Sci Med Sci. (2010) 65:429–33. doi: 10.1093/gerona/glp198
71. Edwards KM, Burns VE, Ring C, Carroll D. Sex differences in the interleukin-6 response to acute psychological stress. Biol Psychol. (2006) 71:236–9. doi: 10.1016/j.biopsycho.2005.06.006
72. Friedman EM, Herd P. Income, education, and inflammation: differential associations in a national probability sample (The MIDUS study). Psychosom Med. (2010) 72:290–300. doi: 10.1097/PSY.0b013e3181cfe4c2
73. Ranjit N, Diez-Roux AV, Shea S, Cushman M, Ni H, Seeman, et al. Socioeconomic position, race/ethnicity, and inflammation in the multi-ethnic study of atherosclerosis. Circulation. (2007) 116:2383–90. doi: 10.1161/CIRCULATIONAHA.107.706226
74. Steptoe A, Hiltl TJ, Dowd JB, Hamer M. Socioeconomic status and central adiposity as determinants of stress-related biological responses relevant to cardiovascular disease risk. Brain Behav Immun. (2019) 77:16–24. doi: 10.1016/j.bbi.2018.11.019
75. Nazmi A, Diez Roux A, Ranjit N, Seeman TE, Jenny NS. Cross-sectional and longitudinal associations of neighborhood characteristics with inflammatory markers: findings from the multi-ethnic study of atherosclerosis. Health Place. (2010) 16:1104–12. doi: 10.1016/j.healthplace.2010.07.001
76. Purser JL, Kuchibhatla MN, Miranda ML, Blazer DG, Cohen HJ, Fillenbaum, et al. Geographical segregation and IL-6: a marker of chronic inflammation in older adults. Biomark Med. (2008) 2:335–48. doi: 10.2217/17520363.2.4.335
77. Brydon L, Edwards S, Mohamed-Ali V, Steptoe A. Socioeconomic status and stress-induced increases in interleukin-6. Brain Behav Immun. (2004) 18:281–90. doi: 10.1016/j.bbi.2003.09.011
78. Azad MB, Lissitsyn Y, Miller GE, Becker AB, Hayglass KT, Kozyrskyj, et al. Influence of socioeconomic status trajectories on innate immune responsiveness in children. PLoS ONE. (2012) 7:e38669. doi: 10.1371/journal.pone.0038669
79. Brody GH, Yu T, Miller GE, Chen E. Discrimination, racial identity, and cytokine levels among African-American adolescents. J Adolesc Health. (2015) 56:496–501. doi: 10.1016/j.jadohealth.2015.01.017
80. Shovestul B, Han J, Germine L, Dodell-Feder D. Risk factors for loneliness: the high relative importance of age versus other factors. PLoS ONE. (2020) 15:e0229087. doi: 10.1371/journal.pone.0229087
81. Matthews T, Odgers CL, Danese A, Fisher HL, Newbury JB, Caspi A, et al. Loneliness and neighborhood characteristics: a multi-informant, nationally representative study of young adults. Psychol Sci. (2019) 30:765–75. doi: 10.1177/0956797619836102
82. Troxel WM, Shih RA, Ewing B, Tucker JS, Nugroho A, D'amico, et al. Examination of neighborhood disadvantage and sleep in a multi-ethnic cohort of adolescents. Health Place. (2017) 45:39–45. doi: 10.1016/j.healthplace.2017.03.002
83. Grimes M, Camerota M, Propper CB. Neighborhood deprivation predicts infant sleep quality. Sleep Health. (2019) 5:148–51. doi: 10.1016/j.sleh.2018.11.001
84. Kawachi I, Kennedy BP. Health and social cohesion: why care about income inequality? BMJ. (1997) 314:1037–40. doi: 10.1136/bmj.314.7086.1037
85. Duggal NA, Upton J, Phillips AC, Hampson P, Lord JM. NK cell immunesenescence is increased by psychological but not physical stress in older adults associated with raised cortisol and reduced perforin expression. Age. (2015) 37:9748. doi: 10.1007/s11357-015-9748-2
86. Steptoe A, Owen N, Kunz-Ebrecht SR, Brydon L. Loneliness and neuroendocrine, cardiovascular, and inflammatory stress responses in middle-aged men and women. Psychoneuroendocrinology. (2004) 29:593–611. doi: 10.1016/S0306-4530(03)00086-6
87. Zorrilla EP, Luborsky L, Mckay JR, Rosenthal R, Houldin A, Tax A, et al. The relationship of depression and stressors to immunological assays: a meta-analytic review. Brain Behav Immun. (2001) 15:199–226. doi: 10.1006/brbi.2000.0597
88. Newton TL, Fernandez-Botran R, Miller JJ, Lorenz DJ, Burns VE, Fleming, et al. Markers of inflammation in midlife women with intimate partner violence histories. J Womens Health. (2011) 20:1871–80. doi: 10.1089/jwh.2011.2788
89. Jaremka LM, Fagundes CP, Peng J, Bennett JM, Glaser R, Malarkey WB, et al. Loneliness promotes inflammation during acute stress. Psychol Sci. (2013) 24:1089–97. doi: 10.1177/0956797612464059
90. Irwin MR, Witarama T, Caudill M, Olmstead R, Breen EC. Sleep loss activates cellular inflammation and signal transducer and activator of transcription (STAT) family proteins in humans. Brain Behav Immun. (2015) 47:86–92. doi: 10.1016/j.bbi.2014.09.017
91. Neergheen VL, Topel M, Van Dyke ME, Sullivan S, Pemu PE, Gibbons GH, et al. Neighborhood social cohesion is associated with lower levels of interleukin-6 in African American women. Brain Behav Immun. (2019) 76:28–36. doi: 10.1016/j.bbi.2018.10.008
92. Miller GE, Cohen S, Ritchey AK. Chronic psychological stress and the regulation of pro-inflammatory cytokines: a glucocorticoid-resistance model. Health Psychol. (2002) 21:531–41. doi: 10.1037/0278-6133.21.6.531
93. Giletta M, Slavich GM, Rudolph KD, Hastings PD, Nock MK, Prinstein, et al. Peer victimization predicts heightened inflammatory reactivity to social stress in cognitively vulnerable adolescents. J Child Psychol Psychiatr. (2018) 59:129–39. doi: 10.1111/jcpp.12804
94. Martinez P, Lien L, Zemore S, Bramness JG, Neupane SP. Circulating cytokine levels are associated with symptoms of depression and anxiety among people with alcohol and drug use disorders. J Neuroimmunol. (2018) 318:80–6. doi: 10.1016/j.jneuroim.2018.02.011
95. O'donovan A, Hughes BM, Slavich GM, Lynch L, Cronin MT, O'farrelly C, Malone, et al. Clinical anxiety, cortisol and interleukin-6: evidence for specificity in emotion-biology relationships. Brain Behav Immun. (2010) 24:1074–7. doi: 10.1016/j.bbi.2010.03.003
96. Amano H, Fukuda Y, Yokoo T, Yamaoka K. Interleukin-6 level among shift and night workers in Japan: cross-sectional analysis of the J-HOPE study. J Atheroscler Thromb. (2018) 25:1206–14. doi: 10.5551/jat.42036
97. Rohleder N, Aringer M, Boentert M. Role of interleukin-6 in stress, sleep, and fatigue. Ann N Y Acad Sci. (2012) 1261:88–96. doi: 10.1111/j.1749-6632.2012.06634.x
98. Bertone-Johnson ER, Whitcomb BW, Missmer SA, Karlson EW, Rich-Edwards JW. Inflammation and early-life abuse in women. Am J Prev Med. (2012) 43:611–20. doi: 10.1016/j.amepre.2012.08.014
99. Nuru-Jeter A, Chae DH, Price M, Telesford J, Mendoza-Denton R, Woods-Giscombe C. Abstract 9550: anticipatory racism threat and superwoman schema: elucidating the relationship between racial discrimination and chronic inflammation. Circulation. (2013) 128:A9550.
100. Stepanikova I, Bateman LB, Oates GR. Systemic inflammation in midlife: race, socioeconomic status, and perceived discrimination. Am J Prev Med. (2017) 52:S63–76. doi: 10.1016/j.amepre.2016.09.026
101. Giurgescu C, Engeland CG, Templin TN, Zenk SN, Koenig MD, Garfield, et al. Racial discrimination predicts greater systemic inflammation in pregnant African American women. Appl Nurs Res. (2016) 32:98–103. doi: 10.1016/j.apnr.2016.06.008
102. Carpenter LL, Gawuga CE, Tyrka AR, Lee JK, Anderson GM, Price, et al. Association between plasma IL-6 response to acute stress and early-life adversity in healthy adults. Neuropsychopharmacology. (2010) 35:2617–23. doi: 10.1038/npp.2010.159
103. Starkweather AR. The effects of exercise on perceived stress and IL-6 levels among older adults. Biol Res Nurs. (2007) 8:186–94. doi: 10.1177/1099800406295990
104. Kasapis C, Thompson PD. The effects of physical activity on serum C-reactive protein and inflammatory markers: a systematic review. J Am Coll Cardiol. (2005) 45:1563–9. doi: 10.1016/j.jacc.2004.12.077
105. Breines JG, Thoma MV, Gianferante D, Hanlin L, Chen X, Rohleder, et al. Self-compassion as a predictor of interleukin-6 response to acute psychosocial stress. Brain Behav Immun. (2014) 37:109–14. doi: 10.1016/j.bbi.2013.11.006
106. Mayr HL, Itsiopoulos C, Tierney AC, Ruiz-Canela M, Hebert JR, Shivappa N, et al. Improvement in dietary inflammatory index score after 6-month dietary intervention is associated with reduction in interleukin-6 in patients with coronary heart disease: The AUSMED heart trial. Nutr Res. (2018) 55:108–21. doi: 10.1016/j.nutres.2018.04.007
107. Tsao TM, Tsai MJ, Hwang JS, Cheng WF, Wu CF, Chou CK, et al. Health effects of a forest environment on natural killer cells in humans: an observational pilot study. Oncotarget. (2018) 9:16501–11. doi: 10.18632/oncotarget.24741
108. Fang CY, Reibel DK, Longacre ML, Rosenzweig S, Campbell DE, Douglas, et al. Enhanced psychosocial well-being following participation in a mindfulness-based stress reduction program is associated with increased natural killer cell activity. J Altern Complement Med. (2010) 16:531–8. doi: 10.1089/acm.2009.0018
109. Pechey R, Monsivais P. Socioeconomic inequalities in the healthiness of food choices: exploring the contributions of food expenditures. Prev Med. (2016) 88:203–9. doi: 10.1016/j.ypmed.2016.04.012
110. Wagner JA, Osborn CY, Mendenhall EA, Budris LM, Belay S, Tennen, et al. Beliefs about racism and health among African American women with diabetes: a qualitative study. J Natl Med Assoc. (2011) 103:224–32. doi: 10.1016/S0027-9684(15)30298-4
111. Panagi L, Poole L, Hackett RA, Steptoe A. Happiness and inflammatory responses to acute stress in people with type 2 diabetes. Ann Behav Med. (2019) 53:309–20. doi: 10.1093/abm/kay039
112. Hernandez R, Kershaw KN, Siddique J, Boehm JK, Kubzansky LD, Diez-Roux A, et al. Optimism and cardiovascular health: Multi-Ethnic Study of Atherosclerosis (MESA). Health Behav Policy Rev. (2015) 2:62–73. doi: 10.14485/HBPR.2.1.6
113. Danese A, Lewis SJ. Psychoneuroimmunology of early-life stress: the hidden wounds of childhood trauma? Neuropsychopharmacology. (2017) 42:99–114. doi: 10.1038/npp.2016.198
114. Schneiderman N, Ironson G, Siegel SD. Stress and health: psychological, behavioral, and biological determinants. Annu Rev Clin Psychol. (2005) 1:607–28. doi: 10.1146/annurev.clinpsy.1.102803.144141
115. Straub RH, Cutolo M. Psychoneuroimmunology-developments in stress research. Wien Med Wochenschr. (2018) 168:76–84. doi: 10.1007/s10354-017-0574-2
116. Rosas-Salazar C, Celedón JC. Eliminating health disparities in asthma: are we at the end of the beginning? Ann Allergy Asthma Immunol. (2019) 123:3–5. doi: 10.1016/j.anai.2019.02.001
117. Fitzpatrick AM, Gillespie SE, Mauger DT, Phillips BR, Bleecker ER, Israel E, et al. Racial disparities in asthma-related health care use in the national heart, lung, and blood institute's severe asthma research program. J Allergy Clin Immunol. (2019) 143:2052–61. doi: 10.1016/j.jaci.2018.11.022
118. Cohen RT, Celedón JC. Community violence and health disparities in asthma. J Pediatr. (2016) 173:13–5. doi: 10.1016/j.jpeds.2016.03.043
119. Senteio CR, Akincigil A. Illuminating racial inequity in diabetes control: differences based on gender and geography. J Racial Ethn Health Disparities. (2020). doi: 10.1007/s40615-020-00830-7. [Epub ahead of print].
120. Walker AF, Hu H, Cuttriss N, Anez-Zabala C, Yabut K, Haller MJ, et al. The neighborhood deprivation index and provider geocoding identify critical catchment areas for diabetes outreach. J Clin Endocrinol Metab. (2020) 105:dgaa462. doi: 10.1210/clinem/dgaa462
121. Powell-Wiley TM, Ayers CR, De Lemos JA, Lakoski SG, Vega GL, Grundy S, et al. Relationship between perceptions about neighborhood environment and prevalent obesity: data from the Dallas Heart Study. Obesity. (2013) 21:E14–21. doi: 10.1002/oby.20012
122. Powell-Wiley TM, Cooper-Mccann R, Ayers C, Berrigan D, Lian M, Mcclurkin M, et al. Change in neighborhood socioeconomic status and weight gain: dallas heart study. Am J Prev Med. (2015) 49:72–9. doi: 10.1016/j.amepre.2015.01.013
123. Powell-Wiley TM, Moore K, Allen N, Block R, Evenson KR, Mujahid M, et al. Associations of neighborhood crime and safety and with changes in body mass index and waist circumference: the multi-ethnic study of atherosclerosis. Am J Epidemiol. (2017) 186:280–8. doi: 10.1093/aje/kwx082
124. Powell-Wiley TM, Wong MS, Adu-Brimpong J, Brown ST, Hertenstein DL, Zenkov E, et al. Simulating the impact of crime on African American women's physical activity and obesity. Obesity (Silver Spring). (2017) 25:2149–55. doi: 10.1002/oby.22040
125. Vazquez CE, Cubbin C. Socioeconomic status and childhood obesity: a review of literature from the past decade to inform intervention research. Curr Obes Rep. (2020). doi: 10.1007/s13679-020-00400-2. [Epub ahead of print].
126. Anekwe CV, Jarrell AR, Townsend MJ, Gaudier GI, Hiserodt JM, Stanford, et al. Socioeconomics of obesity. Curr Obes Rep. (2020) 9:272–9. doi: 10.1007/s13679-020-00398-7
127. Tamura K, Langerman SD, Ceasar JN, Andrews MR, Agrawal M, Powell-Wiley, et al. Neighborhood social environment and cardiovascular disease risk. Curr Cardiovasc Risk Rep. (2019) 13:7. doi: 10.1007/s12170-019-0601-5
128. Brothers RM, Fadel PJ, Keller DM. Racial disparities in cardiovascular disease risk: mechanisms of vascular dysfunction. Am J Physiol Heart Circ Physiol. (2019) 317:H777–89. doi: 10.1152/ajpheart.00126.2019
129. Schultz WM, Kelli HM, Lisko JC, Varghese T, Shen J, Sandesara P, et al. Socioeconomic status and cardiovascular outcomes: challenges and interventions. Circulation. (2018) 137:2166–78. doi: 10.1161/CIRCULATIONAHA.117.029652
Keywords: health disparities, COVID-19, IL-6, immune system, psychoneuroimmunology, psychosocial stress
Citation: Baumer Y, Farmer N, Premeaux TA, Wallen GR and Powell-Wiley TM (2020) Health Disparities in COVID-19: Addressing the Role of Social Determinants of Health in Immune System Dysfunction to Turn the Tide. Front. Public Health 8:559312. doi: 10.3389/fpubh.2020.559312
Received: 07 May 2020; Accepted: 31 August 2020;
Published: 08 October 2020.
Edited by:
Stéphane Cullati, University of Fribourg, SwitzerlandReviewed by:
Fulvio Ricceri, University of Turin, ItalyMel Bartley, University College London, United Kingdom
Copyright © 2020 Baumer, Farmer, Premeaux, Wallen and Powell-Wiley. This is an open-access article distributed under the terms of the Creative Commons Attribution License (CC BY). The use, distribution or reproduction in other forums is permitted, provided the original author(s) and the copyright owner(s) are credited and that the original publication in this journal is cited, in accordance with accepted academic practice. No use, distribution or reproduction is permitted which does not comply with these terms.
*Correspondence: Tiffany M. Powell-Wiley, dGlmZmFueS5wb3dlbGwmI3gwMDA0MDtuaWguZ292