- 1National Institute for Research in Environmental Health, Indian Council of Medical Research, Bhopal, India
- 2Department of Stem Cell Research Sanjay Gandhi Post Graduate Institute of Medical Sciences, Lucknow, India
The exponential growth of pollutant discharges into the environment due to increasing industrial and agricultural activities is a rising threat for human health and a biggest concern for environmental health globally. Several synthetic chemicals, categorized as potential environmental endocrine-disrupting chemicals (EDCs), are evident to affect the health of not only livestock and wildlife but also humankind. In recent years, human exposure to environmental EDCs has received increased awareness due to their association with altered human health as documented by several epidemiological and experimental studies. EDCs are associated with deleterious effects on male and female reproductive health; causes diabetes, obesity, metabolic disorders, thyroid homeostasis and increase the risk of hormone-sensitive cancers. Sewage effluents are a major source of several EDCs, which eventually reach large water bodies and potentially contaminate the drinking water supply. Similarly, water storage material such as different types of plastics also leaches out EDCs in drinking Water. Domestic wastewater containing pharmaceutical ingredients, metals, pesticides and personal care product additives also influences endocrine activity. These EDCs act via various receptors through a variety of known and unknown mechanisms including epigenetic modification. They differ from classic toxins in several ways such as low-dose effect, non-monotonic dose and trans-generational effects. This review aims to highlight the hidden burden of EDCs on human health and discusses the non-classical toxic properties of EDCs in an attempt to understand the magnitude of the exposome on human health. Present data on the environmental EDCs advocate that there may be associations between human exposure to EDCs and several undesirable health outcomes that warrants further human bio-monitoring of EDCs.
Introduction
Effects of environmental pollution on human health are increasingly gaining more attention globally. Environmental pollutants are chemicals that result from human activities, which end up in the environment and subsequently pose risks to human and animal health. Several of these chemicals are collectively known as endocrine-disrupting chemicals (EDCs), which are nowadays gaining more importance in terms of public health due to their widespread effects on human health and potential cause of morbidity.
The Endocrine Society defines EDCs as follows: “an exogenous (non-natural) chemical, or a mixture of chemicals, that interferes with any aspect of hormone action.” These chemicals alter the hormonal balance of the body through several different mechanisms; they can mimic hormones, disrupt hormone synthesis or breakdown, alter the development of hormone receptors, act as hormone antagonists or alter hormone binding. Environmental EDCs are mostly released during manufacture and use of human-made materials such as pesticides, plastics/plasticisers, electronic wastes, flame-retardants, metals, food additives, and personal care products. These EDCs can disturb hormonal balance and consequently result in several health disorders, including developmental and reproductive abnormalities, increased prevalence of hormone-sensitive cancers, neurodevelopmental delays and abnormal growth patterns in children, and alterations in immune function.
EDCs are a group of highly heterogeneous synthetic chemicals used in a variety of settings. Some of the common chemicals included in this group are chemicals used in industries and their by-products (polychlorinated biphenyls [PCBs], polybrominated biphenyls [PBBs] and dioxins), plastics (bisphenol A [BPA]), plasticisers (phthalates) and pesticides (methoxychlor [MXC], chlorpyrifos and dichlorodiphenyltrichloroethane [DDT]). Some EDCs were designed to have long half-lives for industrial purposes and are known as “persistent organic pollutants” (POPs) exemplified by PCBs, dichlorodiphenyldichloroethylene (DDE), dioxin, organochlorine pesticides and hexachlorobenzene (HCB) (Figure 1). The POPs are highly lipophilic and tend to accumulate in the adipose tissue. Due to this property, they also accumulate in the food chain and undergo biomagnification. Many of these substances either do not decay or decay slowly, while others may be metabolized to compounds that are more toxic than the parent chemicals (1, 2). Other EDCs such as BPA and phthalates may not be persistent but are used so extensively that environmental exposure is widespread. EDCs are present ubiquitously in the environment and human exposure occurs through intake of water and food, via inhalation, and through the skin.
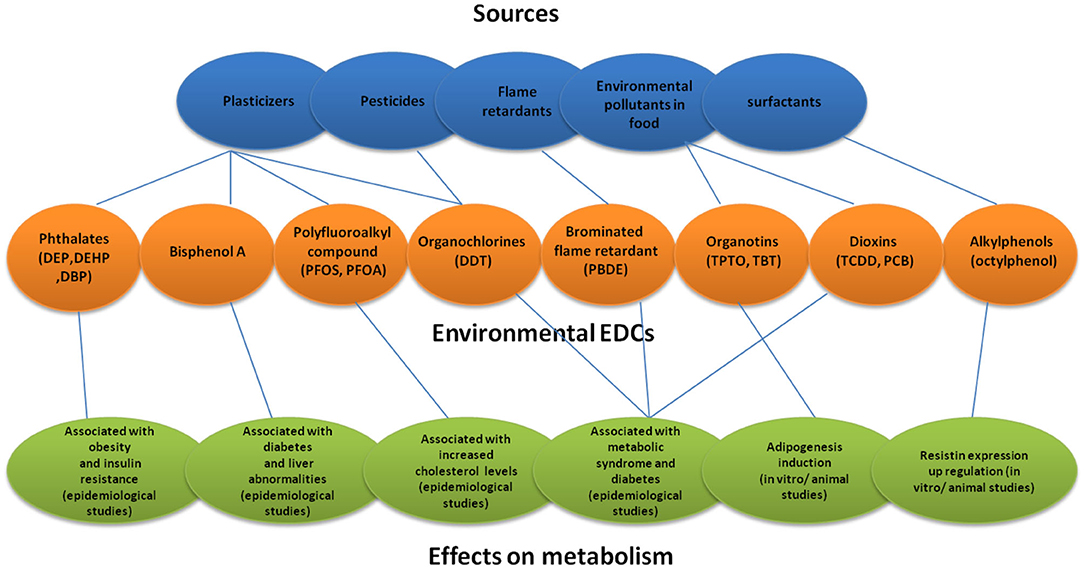
Figure 1. Sources of environmental EDCs and their effects on metabolism. DEP, Diethyl phthalate; DEHP, Diethylhexyl phthalate; DBP, Dibutyl phthalate; PFOS, Perfluorooctanote; DDT, Dichlorodiphenyltrichloro ethane; PBDE, Polybrominated diphenyl ether; TPTO, bis (triphenyltin) oxide; TBT, Tributyltin.
Exposure of plastics to high temperature can lead to leaching or migration of toxic components in environment in the form of microplastics or nanoplastic. In drug delivery system micro- and nanoplastic are used via intravenous, oral, and transcutaneous routes (3), and also there is a migration of nanopolymers from various packaging materials into food (4, 5). Microplastics or nanoplastic accumulates in food chains making them bioavailable again for direct or indirect human exposure via ingestion, skin contact and inhalation (6). Biomonitoring studies have shown that Human consumption of animals that exposed to microplastics and additives persist in human population and can be harmful for human health (7, 8). At present health impact assessments that exclusively focus on the plastic compositions of products while overlook the thousands of plastic additives and their effects.
Drinking water particularly is thought to be a source of significant exposure through leaching, industrial waste discharge and inadequate treatment of water supply for chemicals. EDCs can also be transferred from mother to child through trans-placental route as well as through breast milk. Developing fetuses and children are highly susceptible to environmental exposures, and their effects may not become apparent until a later age. Research shows that such exposures may increase the susceptibility of the child to several non-communicable diseases in their adult life (Table 1). EDCs can potentially target various hormone systems; however, it has been observed that the effect of these chemicals primarily involves the reproductive system, puberty, embryonic development and sex differentiation in fetal life. Thus, it is likely that the primary mechanism of their action is through interference with sex steroid hormones. Furthermore, there is also a growing apprehension that metabolic disorders of adult hood may be linked with EDCs. Several in vitro, in vivo and epidemiological studies link human-environmental EDC exposures with obesity, metabolic syndrome, type 2 diabetes and possibly with some hormone-responsive cancers (Figure 2).

Table 1. Studies documenting associations between different EDC exposures and risk to different diseases.
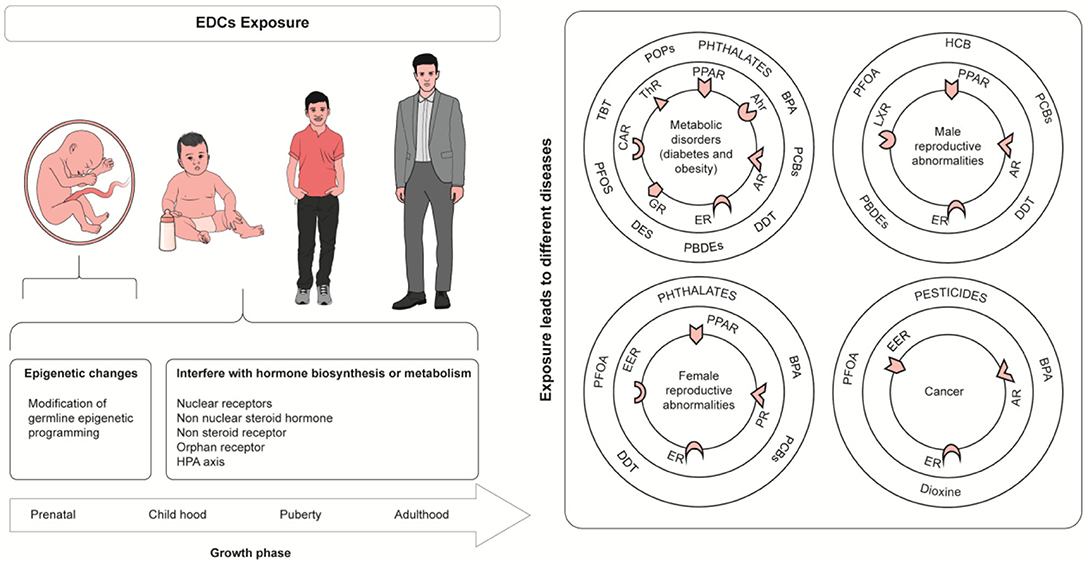
Figure 2. Health Effect of Endocrine disrupting chemicals. Tributyltin (TBT), PerfluorooctaneSulfonate (PFOS), Perfluorinated compounds (PFCs), Perfluorooctanoic acid (PFOA), Bisphenol A (BPA), Diethylstilbestrol (DES), hexachlorobenzene (HCB), dichlorodiphenyltri-chloroethane (DDT), Polybrominated diphenyls ethers (PBDEs), constitutive androstane receptor (CAR), Thyroid Hormone Receptor (ThR), glucocorticoid receptor (GR), Aryl hydrocarbon receptor (Ahr), Androgen receptor (AR), Peroxisome proliferator-activated receptor (PPAR), Estrogen receptor (ER), Liver X Receptor (LXR).
The breadth of this review identifying negative human health impacts of various EDC's strongly suggests that there are significant risks to human health and a precautionary approach and human bio-monitoring of EDC's is warranted.
Effects of EDCs on Glucose Metabolism and Obesity
Over the past several decades, obesity and type two diabetes rates have increased markedly in all age groups worldwide and particularly in developed countries. Obesity is frequently associated with other morbidities such as metabolic disorders (including metabolic syndrome, type 2 diabetes, cardiovascular diseases, pulmonary complications, and liver disease), psychological/social problems, reproductive abnormalities and some forms of cancers. An amalgamation of lifestyle, genetic and environmental factors probably accounts for the quick and considerable increase in obesity rates. An increase in the occurrence of metabolic diseases has been noted in last several decades, which coincide, with the significant changes in the chemical environment around us, which came in practice during the same period. Velmurugan et al. (9) has demonstrated that the common risk factors for diabetes may not be the sole contributor, while other factors such as production of EDCs (plastics, plasticizers, pesticides, e-waste, food additives) has been found to be positively associated with the growing trend of the global diabetes incidence in last few decades (Figures 3, 4).
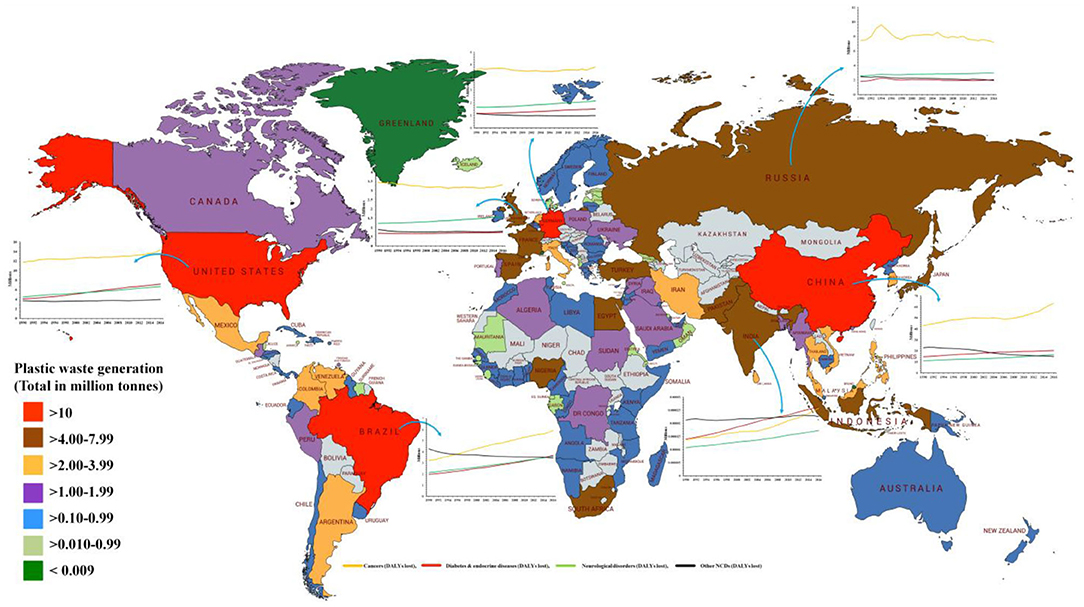
Figure 3. Total plastic waste generation by country, measured in million tons per year for the year 2010 and disease burden from non-communicable diseases (from 1990 to 2016). Total disease burden from non-communicable diseases (NCDs), measured in DALYs (Disability-Adjusted Life Years) per year. DALYs are used to measure total burden of disease—both from years of life lost and years lived with a disability. One DALY equals 1 lost year of healthy life. Base map courtesy of mapchart.net (http://www.mapchart.net) [Source: (10, 11)].
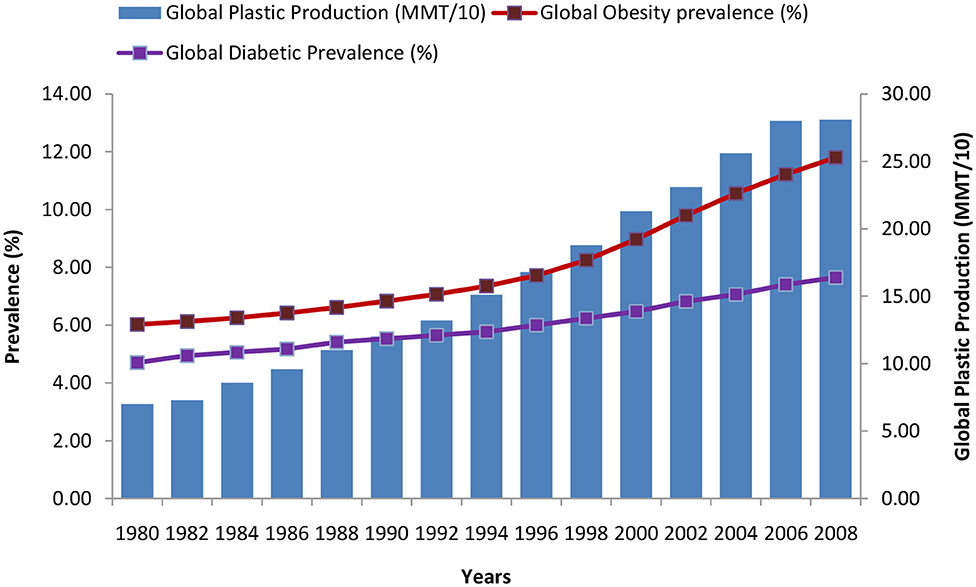
Figure 4. Trends of global diabetes, obesity, and plastic production during the period of 1980–2008 [Data source: https://ourworldindata.org/plastic-pollution and (9, 12)].
This change in our chemical environment seems to be a contributing factor in this “obesity pandemic” apart from genetic factors and modern lifestyle that embraces high energy intake, reduced physical activity, sleep deprivation and near stable home temperatures. This has led to the hypothesis that some of these environmental chemicals disrupt the endocrine process and lead to obesity by interfering with various endocrine aspects of metabolism (13, 14). This hypothesis garners support from animal as well as epidemiological studies, which have revealed that a diverse array of environmental chemicals can influence adipogenesis and obesity; however, its extent, exact mechanism and epidemiology are yet to be elucidated. The EDCs linked with obesity and adiposity include several POPs (15–18), BPA (19, 20), and phthalates (21–23). It has been suggested that in utero or childhood exposure to certain EDCs predisposes some individuals to obesity, highlighting the importance of timing of exposure (17, 21, 22, 24, 25). Such EDCs have been referred to as “environmental obesogens” (26). The term “metabolic disruptors” has also been suggested as many of these chemicals be associated with other metabolic diseases such as metabolic syndrome, insulin resistance and type 2 diabetes as well (17, 27). Several studies have found association of type 2 diabetes and/or its risk factors with PBCs, DDE, organochlorine pesticides, HCB, dioxins, BPA and phthalates (28–37). Some prospective studies have interestingly concluded that no single POP could significantly predict T2D, but some combination of POPs could predict the future risk of T2D (28, 31–33, 38). This also lends support to the concern that exposure to EDCs as mixtures that occur in the environment may have greater significance than that to single chemicals tested in laboratories. In addition, several studies have shown association of gestational diabetes to phthalates, BPA and PCB (39–42). Increased risk of non-alcoholic fatty liver disease has been linked to BPA (43) as well as cardiovascular risk factors are found to be linked to both organochlorine pesticides (44–46) and BPA (47, 48).
EDCs alter metabolic balance through multiple mechanisms including alterations in peroxisome proliferator-modulated pathways (49), adipogenesis (50), pancreatic β-cell function (51–53) and hypothalamic neuropeptides (54, 55). In addition, decreased physical activity in relation to whole-body energy balance has been reported in mice that were exposed to BPA either during a perinatal period or in adult life (56, 57). However, most of the evidence regarding the mechanism of action of EDCs comes from experimental studies. In a recent study, it was shown that prenatal exposure to BPA and certain phthalates might affect the levels of adiponectin and leptin in human fetus (58). A novel hypothesis advanced is the role of gut microbiota in EDC-induced type 2 diabetes. It is thought that EDCs not only cause dysbiosis of gut microbiota but also undergo metabolism by gut microbes, and both these processes alter the gut neuroendocrine regulation of metabolism leading to insulin resistance and type 2 diabetes (9).
Effect of EDC on Blood Pressure
To elucidate associations of urinary EDC's like phthalate with blood pressure (BP), a cross-sectional analysis of 6–19 years children in the 2003–2008 National Health and Nutrition Examination Survey (NHANES) was carried out which reveals an increase in blood pressure associated with 3-fold increase in urine phthalate (DEHP) (59). A study conducted in people living near a dioxin-contaminated area showed that increasing serum dioxin levels are correlated with elevated diastolic blood pressure. In addition, the prevalence of hypertension was also found to be correlated with serum PCDD and PCDF levels in Taiwanese and Florida adults with dioxin exposure (60, 61). Similarly, high serum PCB Level was also found to be associated with elevated blood pressure in NHANES 1999–2002 and NHANES 1999–2004 participants (62). Studies derive conclusions about the effects of EDCs on hypertension but further studies with larger diverse cohorts are now needed to verify an association between EDC's exposure and risk of hypertension.
EDCs: Female and Male Reproductive Health
Female Reproductive Disorders: Role of Environmental EDCs
Several EDCs are known to exert effects on female reproductive hormones and their receptors through oestrogenic, anti-estrogenic, androgenic and anti-androgenic mechanisms. Some of these EDCs include BPA and phthalate, which are classified as xeno-oestrogens. They act by both receptor-dependent and receptor-independent mechanisms, either directly by binding to estrogen receptors, increasing aromatase activity and increasing estrogen sensitivity, or indirectly by their effect on gonadotropin-releasing hormone, leading to an increase in endogenous estrogen production (63–65). EDCs are thought to affect the menstrual cycle, alter fertility and oogenesis and are implicated in diseases such as polycystic ovary syndrome (PCOS) and endometriosis. Hauser et al. (66) documented the first-ever adverse effect of urinary phthalate metabolites by using in vitro fertilization (IVF) where they found that concentrations of urinary metabolites of di(2-ethylhexyl) phthalate (DEHP) and di-isodecyl phthalate (DiDP) was inversely related with oocyte yield and number of metaphase II oocytes at retrieval, whereas only metabolites of di-isononyl phthalate (DiNP) and di-isodecyl phthalate (DiDP) was linked with reduced fertilization rate. However, the exact mechanism of action of these chemicals is not yet clearly understood, but it is likely that multiple pathways are involved including hormone receptors.
Female Puberty
Puberty is a phase of transition between adolescence and adulthood during which the reproductive system reaches full maturity (67). The onset of puberty involves a complex neuroendocrine mechanism and influenced by several factors including ethnicity, genetics, nutrition and environmental factors (68, 69). Recently early onset of puberty in girls has been noted across several countries and a role of environmental pollutants has been implicated, notably that of BPA, phthalates, and organohalogens (70). Puberty is brought about by activation of the hypothalamic-pituitary gonadotropic cells-gonadal axis. The activation of this axis is thought to be regulated by a novel neuropeptide and its receptor, which are in turn modulated through sex steroid receptors in hypothalamic neurons (71). It is possible that oestrogenic EDCs or xeno-oestrogens act through the sex steroid receptors to bring about early puberty. During childhood, the levels of sex hormones in blood are very low. However, it has been found that estrogen and androgen receptors are expressed since a very early age (72). Therefore, even a small change in these levels through an exogenous source may bring about several pubertal changes (73). In several studies, early onset of pubertal changes in girls has been reported to be associated with phthalates (74–77), BPA (78, 79), and organohalogens (80–82), whereas in other studies, no such association (30, 83, 84) or even inverse association has been reported (85, 86). Another study found a non-linear association of BPA and phthalate metabolites with pubertal development (87). However, it is possible that these differences result from variation in environmental exposure, timing of exposure and non-linear dose-response patterns of EDC effects. Exposure to EDCs during prenatal and perinatal period has also been thought to be linked with early onset of puberty in girls as well (88).
Polycystic Ovary Syndrome (PCOS)
Most of the information on direct effects of EDCs on fertility is available from in vitro and animal studies. A few human studies have been carried out in women attending fertility clinics and have provided variable results. Some of these studies have shown an association of EDCs directly with infertility and its parameters, whereas others have correlated them with PCOS, which is a complex endocrinopathy leading to infertility and insulin resistance. A negative association between urinary concentrations of phthalate metabolites and serum inhibin B levels was found, suggesting a detrimental effect of phthalates on growing antral follicles (89). In the Longitudinal Investigation of Fertility and the Environment Study, concentrations of phthalates, but not BPA in women were associated with longer time to pregnancy (90). In other studies, BPA was found to be associated with reduced ovarian reserve (91), lower antral follicle count (92) and PCOS (93, 94) in infertile women. Higher quartiles of urinary BPA concentration were also shown to be associated with increased odds of implantation failure (95). One of the studies showed that high PCB and pesticide concentrations in the follicular fluid correlated with poor embryological intracytoplasmic sperm injection outcomes (96). Another study documented higher serum concentrations of perfluorinated compounds and phthalate metabolites was present in women with PCOS (97). DDT was found to be associated with altered hormonal levels in Chinese women with PCOS suggesting a role in its pathogenesis (98). In a large American cross-sectional study involving more than 30,000 women, researchers found an association between EDCs and earlier age at menopause, and they also identified 15 EDCs (including 9 PCBs, 3 pesticides, 1 furan and 2 phthalates) that needed further evaluation for potential adverse effects on ovarian function (99).
Endometriosis
Endometriosis is the presence of ectopic endometrial tissue, which is a cause of pain and infertility. Relatively few studies have explored the role of EDCs in endometriosis. However, its association has been reported with higher blood or urinary levels of BPA (94, 100), various phthalates (101–103) and organochlorine pesticides (104, 105). On the other hand, no association or inverse association with phthalates was observed in studies on infertile Japanese women and American women, respectively (106, 107). A recent meta-analysis of past epidemiological studies has recorded a significant constant increase in the risk of endometriosis to polychlorinated bisphenyls (PCBs) group (OR = 1.58; 95% CI: 1.18–2.12), organochlorine pesticides (OCPs) group (OR = 1.40; 95% CI: 1.02–1.92) and phthalate esters (PAEs) group (OR = 1.27; 95% CI: 1.00–1.60), while BPA showed no significant association with endometriosis (108).
Obstetric Outcomes
In utero exposure to EDCs through trans-placental route has become a concern as it is thought that small endocrine imbalance during fetal development can lead to significant and lasting changes. Several researchers investigated this in the last decade. In utero BPA exposure was found to be associated with decreased birth weight (109–112) and increase in gestational length (112, 113). However, Shoaff et al. (114), did not find any association between prenatal phthalate exposure and birth size or duration of gestation. In another study, both maternal and paternal BPA and phthalate concentration were found to be associated with smaller birth size and increased gestational age (115). Though both maternal and paternal exposure to these chemicals will be similar as they are exposed to similar environment, paternal EDC levels likely reflect maternal exposure and do not directly affect the fetal exposure. Maternal urinary levels of paraben were found to be associated with increased length of male neonates in a Chinese cohort (116). An American study on several classes of EDCs showed that gestational exposure to perfluoroalkyl substances and organophosphate pesticides was associated with reduced birth weight while phthalates, BPA, PCBs, PBDEs (Polybrominated diphenyl ethers) or OCPs (organochlorine pesticides) had either small or no association with differences in birth weight (117).
Male Reproductive Disorders: Role of Environmental EDCs
EDCs are thought to affect the male reproductive system as well. Human studies indicate that these chemicals affect pubertal growth and semen quality and cause defects such as hypospadias and cryptorchidism. Well over two decades back, Sharpe and Skakkebaek (1993) proposed that several male reproductive disorders such as low sperm counts, cryptorchidism and hypospadias may share a common fetal origin and that environmental exposure to oestrogenic chemicals might play a key role (118). Though this estrogen hypothesis was later refuted, except diethylstilbestrol, it propelled scientific research on effects of EDCs on male reproductive system. It is now thought that EDCs act on male reproductive system by disrupting fetal endocrine balance through their action on steroid hormone receptors (SHRs) or interference with the synthesis, kinetics or metabolism natural hormones (World Health Organization [WHO] and United Nations Environment Program 2012). Sperm function affected by reactive oxygen species generated during metabolism of these chemicals is another possible effect of EDCs leading to infertility (119).
Male Puberty
The effect of EDCs on male puberty has received relatively less attention compared with that on female puberty, and the results of these studies show wide discrepancies. These studies unlike those on semen quality mostly show correlations with rapidly metabolizing chemicals. Association of early pubarche in boys was noted with Polybrominated diphenyl ether (PBDE) (86) and some phthalates (120), while inconsistent or no association of phthalates and BPA with puberty in boys was found in other studies (87, 121). In another study, monomethyl phthalate exposure was found to be inversely associated with pubarche among boys while there was no association with other phthalate esters (122). Wang et al. (123) documented that peripubertal BPA exposure in boys was associated with earlier pubertal onset, but delayed pubertal progression.
Semen Quality
There are numerous causes of infertility in men. Even though sperm count does not associate specifically with fertility, it is documented that men with very low sperm counts frequently have fertility problems. In 1996, in their review article, Toppari et al. expressed concern regarding decreasing semen quality in the last few decades, and they advanced the hypothesis that exposure to environmental chemicals may be responsible for adverse trends in male reproductive health (124). Several EDCs have been found to affect semen parameters adversely. These include organophosphate pesticides (125–127), BPA (128), perfluorinated compounds (129), phthalates (130), and organochlorines (131). It was observed that adult life exposure to PBC affects mainly sperm motility while perfluorinated compounds alter sperm morphology primarily. However, the overall impact of EDCs on male fertility is not clear so far. Though sperm count and morphology appear to be altered to some extent, the effect on fertility seems to be minor at best.
Cryptorchidism
Cryptorchidism is the condition in which the testis does not descend completely to the bottom of the scrotum. Its incidence has been documented to increase from 1–3% in the late 1950s to 7–9% in the 2000s (132). This condition shows familial clustering suggesting a role of genetic and environmental factors. Known risk factors for cryptorchidism include maternal gestational diabetes and maternal use of alcohol, acetaminophen or nicotine-containing substances. In recent research, cryptorchidism has been found to be associated with prenatal or early childhood exposure of several chemicals including PBDE (133, 134), BPA (135), parabens (135), and PBCs (134). Other researchers have found no association of cryptorchidism with PBBs (136), organochlorine pesticides (137), perfluorinated compounds (138, 139), or PCBs (140). Because several environmental factors are also associated with cryptorchidism, the effects of individual factors are difficult to establish and the possibility of confounders cannot always be eliminated in these studies.
Hypospadias
Hypospadias is a congenital malformation in which the urethral folds do not fuse properly. A meta-analysis suggested an association between pesticide exposure in men and risk of hypospadias in their offspring (141). Another study suggests that exposure to HCB and p,p′-DDE during fetal life may be a risk factor for hypospadias (142). However, no significant association of exposure to PCBs (140, 143), organochlorine pesticides (137), PBBs (136), PBDE (143), or perfluorinated compounds (144) with hypospadias was noted in other studies. Hypospadias is a relatively uncommon condition; therefore, most of these studies face the problem of small sample size and are not statistically robust.
Overall, the effect of individual EDCs on reproductive system appears uncertain; however, it is possible that some additive or synergistic effects of mixtures do exist. Conflicting results and differences in study variables make it difficult to establish the role and extent of effects of EDCs either individually or in combinations. Large-scale studies taking into account the exposure of all known EDCs in a uniform manner will be needed to explore such complex association.
Role of EDCs in Neurodevelopmental Disorders
Prenatal exposure to EDCs is thought to affect brain development in the fetus, and some neurodevelopmental abnormalities have been attributed to them. Prenatal BPA exposure has been linked to poor social behavior (145, 146), higher anxiety and depressive behaviors (147). Some studies have suggested a sex-specific effect of BPA on behavior. A study showed that poor social behavior was found to be more pronounced in girls (146), while a study showed that significant positive associations were found between prenatal BPA and emotionally reactive and aggressive behavior in boys (148). In another study, prenatal BPA exposure was noted to be associated with increased anxiety and depression in boys but not in girls (149). The gender difference is likely due to the close relation of brain development with gonadal hormones as proven by animal studies. The brain, particularly hypothalamus, has been shown to be affected by oestrogenic EDCs in a sex-, time- and exposure-dependent manner (150). In utero exposure to phthalates is shown to be associated with adverse cognitive and behavioral outcomes such as lower IQ, attention deficit, hyperactivity and poorer social communication in children (151–153). Other EDCs such as organophosphates (152, 154), PBDEs (155, 156), perfluorinated compounds (157), and PCBs (153, 158) have also been linked to adverse neurodevelopmental performance, behavioral problems and lower IQ to varying degrees. There is also some evidence that some PBDEs and PCBs affect neuropsychological status in adults (159, 160). Animal studies have provided ample evidence that EDCs act on developing brain through multiple mechanisms: (a) steroid hormones and receptors, (b) neuroendocrine system, and (c) epigenetic changes (161). One of the main biological mechanisms proposed for adverse neurodevelopmental effects of prenatal EDC exposure is disturbance of thyroid hormone homeostasis, which is critical for fetal brain development (162).
Thyroid Homeostasis and EDCs
Thyroid hormones are controlled through the hypothalamic–pituitary–thyroid axis, and they are vital for the regulation of several biological systems and can be disrupted by EDC exposure. Specifically, the phenols and phthalates are thought to act directly on the thyroid hormone receptor, and studies have shown that these compounds are thyroid receptor antagonists. Epidemiological studies have shown that cognitive functions of the offspring may be adversely affected by even a marginal lowering in thyroxin levels in a pregnant woman (163–165). Thus, exposure to thyroid-disrupting chemicals may have significant consequences for public health even if they bring about a small reduction in thyroid hormone levels. Several studies have evaluated the EDC exposure effect on thyroid hormones in humans. Most of these have explored the effect on pregnant women and new-borns. A study reported that urinary concentrations of phthalate metabolites were seen to be associated with lower T4 and T3 or higher thyroid-stimulating hormone (TSH) levels in adults (166). In another study, prenatal exposure to phthalates was found to be inversely associated with both total serum thyroxine in pregnant women and newborns as well as TSH in newborns (167). The association with BPA is more variable in several studies. Two studies found no association between thyroid hormone levels and maternal BPA exposure (168) and cord blood BPA levels (58), respectively. Another study reported no association between maternal urinary BPA concentrations and thyroid hormones measured in cord serum when analyzed for all newborns, but they found that in female newborns, lower cord TSH was associated with the 10-fold increased BPA in maternal urine (169). In the analysis of data from the U.S. National Health and Examination Survey, urinary BPA showed a negative relationship with serum TSH levels in adults (166), while another European study concluded that a significant positive association was observed between urinary BPA and serum TSH in adult women (170). A study found that there was a negative correlation between PCB levels and maternal free T3 but not with cord blood (171). However, a systematic analysis did not find any correlation between PCBs and thyroid hormones in either pregnant women or newborns (172). In a study including three European cohorts, the researchers concluded that PCB was associated with lower TSH levels in newborns (173). In another study, in the case of PBDEs, negative correlation was found with maternal and cord blood thyroid hormones (171), while positive correlation was seen with maternal blood thyroid hormone levels in two other studies (174, 175). Organochlorine pesticides were noted to be associated with reduction in thyroid hormones (176).
Renal Function and EDCs
Chronic kidney disease (CKD) is a worldwide health menace of growing concern, and its prevalence all over world is estimated at 13.4% (177). Kidneys serve as the filtration unit and thus are exposed to all compounds in a greater degree compared to other organs. Humans are not exposed to a single compound but to mixture of compounds and they are likely to affect the kidneys as well. Without a doubt, many non-persistent EDCs, e.g., phthalates, bisphenols, benzophenones, and parabens, share common exposure sources such as plasticware and personal care products in various things.
There are growing evidences suggesting that non-persistent endocrine disrupting chemicals (EDCs), are linked with adverse kidney function (178). Studies also showed that exposure to di-(2-ethylhexyl) phthalate (DEHP) through the consumption of contaminated milk was associated with micro-albuminuria in children (179, 180). The US National Health and Nutrition Examination Survey (NHANES) delineated the association of urinary phthalate metabolites with CKD markers such as albumin-to-creatinine ratio (ACR), estimated glomerular filtration rate (eGFR), and urinary protein-to-creatinine ratio (UPCR) has also been revealed in a population of children (181, 182). Urinary BPA levels were also associated with ACR among the US children (183), as well as Chinese adults (184). Patients on dialysis have more exposure to BPA due to daily use of dialysis tubing. BPA levels are higher in patients undergoing hemodialysis and peritoneal dialysis, than in healthy controls (185). NHANES 2003–2006 Estimated Glomerular Filtration Rate (eGFR) among a general population of the US adult females shows positive association with urinary BPA levels but not in the adult males (186).
EDCs and Hormone-Dependent Cancers
A large fraction of EDCs acts through oestrogenic and androgenic receptors; therefore, researches have focused on their relation to hormone-dependent cancers. Such neoplasm's include breast, endometrial, ovarian, and cervical cancers in women and prostate cancer in men. The incidence of these cancers has risen at a higher rate over the last few decades (187–189). As other non-communicable disease, this increase is multifactorial, and change in lifestyle, industrialization and EDCs are thought to be responsible at least in part (161).
The development and progression of estrogen-dependent malignancies, particularly breast cancer, depend on prolonged or exaggerated estrogen exposure (190). Approximately 70% of breast cancers are sensitive to estrogen as they show ER-positivity (191). Unopposed estrogen or estrogen-mimicking EDCs can bind to ER, switching on the downstream signaling of estrogen-responsive genes involved in the cell cycle (64, 192, 193). Several oestrogenic EDCs, such as BPA and phthalates, have been shown to induce epigenetic modification or genotoxic effects (27, 194, 195), which can alter gene products and genetics, eventually predisposing to certain diseases. However, only a few human studies have explored this association so far. Although meta-analysis did not find any association between organochlorine pesticides and breast cancer (196), another study linked it to exposure before 14 years of age (197). A study in Mexico showed a positive correlation between MEP, a phthalate metabolite, and breast cancer while other phthalates (MBP, MEOHP, and mono [3-carboxypropyl]) had negative correlation with breast cancer risk. The evidence of any relation between BPA exposure and breast cancer is weak, and it is observed only in animal studies. This may be due to its short half-life so that measurement of exposure is difficult (161). Agricultural Health Study, USA, has found that several pesticides are linked with increased risk of prostate cancer (198, 199). The link between EDCs and other hormone-dependent cancers is tenuous as it is based on a handful of animal and laboratory studies. It has not yet been explored through human studies, and it constitutes a lacuna in our knowledge.
Endocrine Disrupters Accelerate Aging
Growth is the consequence of a multifaceted interaction of genetic, constitutional, nutritional, endocrine and socio-economic factors as well as psychosocial well-being (200, 201). The evidence is seen in the fact that over the last century, children have gradually become taller and are reaching puberty earlier. For example, the age of menarche in European girls in the middle of the nineteenth century was 16–17 years, in contrast to the current age of 13 years or less. Rampant use of sex steroids as growth promoters has occurred in animal farming, which continues in several countries of the world.
The timing and evolution of reproductive aging depend mainly on genetic and environmental factors. So far, most research has focused on genetic predispositions (202). Nevertheless, the environment probably plays a role, and there is an assumption that EDCs may accelerate reproductive aging, resulting in shortened reproductive lifespan. Recent studies show that exposures to EDC during critical developmental periods, particularly during fetal life and early childhood can cause molecular/cellular changes that modify the function of the affected tissues later in life, a concept referred to as the fetal/developmental basis of adult disease (203). These effects are thought to be brought about by epigenetic modifications. Animal studies show that reproductive aging is accelerated by EDC (MXC) (204), BPA (205), and dioxins (206). Recent epidemiological evidence also links EDC exposures during periods of growth to accelerated menopause [diethylstilbestrol (207) and perfluorocarbons (208)]. BPA has also been shown to promote oxidative stress and inflammation, and it has been suggested that it makes postmenopausal women suffer health effects related to aging (209).
Dose-Response Dynamics of EDCs
Several biological effects of EDCs are mediated through SHRs (210). Hormone receptors are high-affinity receptors, that is, a small concentration of hormones or hormone mimickers can produce a significant biological effect. In addition, the dose-response curves of these receptors are non-linear; they can be sigmoidal, or more complex, including being non-monotonic. The non-monotonic dose-response (NMDR) curves are often “U-shaped” (with maximal responses observed at low and high doses) or “inverted U-shaped” (with maximal responses observed at intermediate doses) (161). EDCs mimic hormones in their actions including low-dose effects and dose-response patterns, which depend on characteristics of their binding receptors and ligands (161). Due to this property, environmentally relevant concentrations of these chemicals need to be studied thoroughly for their effects on the general population. Several studies have provided evidence that low-dose environmental exposures to EDCs are associated with disorders in humans as well as wildlife (17, 70, 211, 212). Research shows that many SHR-mediated adverse effects of EDCs may be non-linear or even non-monotonic and such effects commonly occur in dose ranges exerting no overt cytotoxicity (213–218). One of the setbacks due to these non-classical responses is in extrapolating the effect from high doses to low doses or vice versa because there is no linear relationship (219). Defining safe limits for such chemicals also becomes troublesome.
Most of the evidence for NMDR comes from cell culture and animal studies. Several epidemiological studies have also focused their attention on NMDR in EDCs recently but they differ significantly in their conclusions (23, 219). However, these differences in conclusions can arise from several inherent drawbacks in human epidemiological studies, for example, cross-sectional vs. prospective design dichotomy and difference in study analysis methods. In addition, there is a lack of sufficient knowledge about kinetics of these chemicals, their interactions with adiposity and diet, and changes to these factors with age and gender. Furthermore, in epidemiological studies, populations are often exposed to a variable mixture of chemicals at various exposure levels for various durations, and predictably, these interactions are complex. These factors make human studies difficult to interpret.
Epigenetic and Transgenerational Effects of EDCs
More recently, scientists have discovered evidence showing that EDCs can modify gene expression without mutating DNA, which is also known as epigenetic change (220). The known possible mechanisms of epigenetic changes include methylation of cytosine residues in DNA, post-translational modification of histones and altered microRNA expression. These changes may lead to transgenerational effects on numerous organs and organ systems and are thought to be tissue-specific as well as dose-dependent (221–223). Epigenetic transgenerational inheritance is the phenomenon where environmental exposures of a woman during gestation lead to germline epimutations, which are then transmitted to subsequent generations with an observable phenotypic expression (224, 225). BPA is the most widely studied EDC in terms of epigenetic changes, and several animal studies support this theory (226–228). Other chemicals implicated in epigenetic changes leading to transgenerational effects are dioxins (229, 230), DDT (231, 232), and phthalates (233, 234). In addition, the epigenetic changes are also thought to cause latent effects in case of early life exposure, with manifestation later in life (235). However, most of these studies have evaluated only the effects on the reproductive system of experimental animals. The epigenetic changes add another dimension to understand the complexity and mechanism in the studies of environmental exposures and their effects.
Timing of EDC Exposures as a Critical Element
EDCs influence and modify the hormonal homeostasis and, therefore, it is plausible that their effects will be more pronounced in periods during which marked hormonal changes occur, such as perinatal period, puberty and pregnancy. Tissue differentiation and maturation of several organs occur during these “critical windows” which may be influenced by EDCs (236). Moreover, the effects of exposure may not be apparent immediately and may take several years to be observable. This is supported by several studies that show the association between several health and behavioral parameters and EDC exposure during these key periods. In utero exposure to several EDCs was found to influence fetal growth and birth weight (109–112, 117) and also predisposed some individuals to obesity and fat gain during later life (17, 21, 22). Exposure to EDCs during the prenatal and perinatal period has been thought to influence the timing of puberty as well (86, 88). Several studies evaluating the neurological development of children have shown association of prenatal EDC exposure with adverse cognitive and behavioral outcomes (145, 146, 148, 151–153). Prenatal exposure to phthalates was shown to alter thyroid homeostasis in pregnant women as well as the offspring (167).
For POPs, latency could be due to long-term continuous exposure. For non-persistent EDCs, exposure could be variable. Apart from the molecular mechanism, epigenetic changes may be important in delayed or latent effects, especially in perinatal exposures.
Risks From Exposure to EDCs Mixture: Cocktail Effect
EDCs do not behave in the manner of usual toxins. Unlike occupational exposure, environmental exposure to ubiquitously present contaminants occurs not only in low doses as discussed earlier, but also simultaneously to a variety of chemicals in varying combinations. Several of these EDCs act through the same receptors either in agonistic or antagonistic mechanisms. The possible combined effects may be additive, synergistic, antagonistic or nullifying (237, 238). In combination, it is even possible to affect different from individual chemicals. It has been demonstrated in animal studies that combinations of EDCs can produce effects in levels at which these chemicals do not induce any measurable effects individually (237, 239). This unique property of EDCs has been termed as “cocktail effect” by some researchers (240). The interactions of chemicals in the mixture appear to be dose-dependent, adding to the complexity further. One of the drawbacks of most epidemiological studies and animal studies is that they take into account each chemical one by one. Thus, the results in these studies may not precisely effect reflect the environmental effect. A study evaluating presence of mixtures of organochlorines in the sera of healthy women and women diagnosed with breast cancer found that only dichlorodiphenyldichloroethane (DDD) individually showed a moderate increase in the risk of developing breast cancer. They also found that a combination of aldrin, p,p′-DDE and DDD was more frequently present in breast cancer patients while it is absent in healthy women (241).
Gutmicrobiome: A Player in the Toxicity of Environmental Pollutants
Human beings are exposed to different chemicals through air, water and soil, but oral exposure to EDCs, largely via the food chain, is their key pathway into human metabolism. The gut microbiome comprises trillions of bacteria, viruses and fungi, and it is documented as a main participant in the metabolism of nutritional compounds, drugs, antibiotics and environmental toxins (259–264). Bacterial fermentation of undigested carbohydrates and xenobiotic compounds produces short-chain fatty acids, choline, bile metabolites and several volatile compounds including hydrogen sulfide. The gut microbiome also plays a major role in regulation of various hormones, neurotransmitters and ultimately host metabolism. Some metabolites produced during bacterial fermentation in gut behave as hormones that can affect diverse host metabolic functions, and hence the gut microbiome has been anticipated to be a new endocrine organ (265–267). Dysbiosis of gut microbiome is commonly observed in medical conditions. Concerning diabetes, a shift in the Bacteroidetes/Firmicutes ratio was found to have a key link with plasma glucose concentration (268). Microbial metabolism of chemicals, as well as EDCs, by gut microbiome can lead to microbial dysbiosis, stimulation of specific bacterial genes, and altered microbial transformation of molecules (260, 268).
Previous researchers had determined how diet and host health could influence the gut microbiome composition (269–273). On the other hand, several acquired factors encountered on an everyday basis can exert extreme effects on the gut microbiome. Environmental chemicals, such as EDCs, heavy metals and air pollutants are increasingly invasive in terrestrial and aquatic environments, and there is a signal that such chemicals will turn out to be even more profuse in near future (274–281). Such chemicals are also found in things that we use daily, such as plastic water bottles, storage containers, and antibacterial supplies. Introduction to such chemicals can result in extensive host effects and concurrently aim commensal microbes harbor within the gut and perhaps other organs. The chemical-induced dysbiosis of the gut microbiome leads to disruptions in various host systems, mainly the central nervous system, via the gut–microbiome–brain axis (282–284). An improved understanding of how environmental chemical-induced gut microbiome dysbiosis changes strengthen host disease is essential. Disorders associated with gut dysbiosis comprise metabolic, neurobehavioral, immunological, cardiovascular, gastrointestinal and several other disease states (273, 285–291). The gut microbiomes have a different class of enzymes such as Phase I and Phase II. They can metabolize environmental xenobiotic chemicals and environmental chemicals may cause dysbiosis of gut microbiome, with potential effects on human health. Based on previous studies we may conclude that gut microbiome plays a major role in the toxicity of environmental pollutants.
Evaluating Health Effects of EDCs in Humans: Is Exposomes Mapping the Key?
Breakthrough in the mapping of the human genome has laid the foundation of potent tool to classify genes and biological processes underlying any feature influenced by inheritance, including diseases. Now a similar approach can be used for mapping the “exposome”—the aggregate of environmental influences and biological responses throughout the life span (292, 293). The major challenge in EDC research is the difficulty in precisely measuring the exposure during critical sensitive windows as well as throughout the lifespan (292). Emerging technologies in genomics, epigenomics and mitochondriomics are novel opportunities to bridge the gap in exposome measurement and precision in EDC research. Every aspect of the continuously changing external and internal environment (294) constantly affects Epigenomics, proteomics and metabolomics. Extensive exposome approach will facilitate the assessment of deleterious health effects of EDCs in humans through targeted biomarkers that restructure past environmental exposure and forecast future hazard (295, 296).
Impact of EDCs in the Context of Climate Change
Climate change has been globally acclaimed as a major environmental risk, also when combined with chemical pollution and habitat loss it has the potential to have a severe impact on human health. The impact of escalating climate change has added exigency to the rising problem of EDC's pollutants. A study by Brown et al. (297), the first of its kind, showed that climate change could amplify the deleterious effects of pollution from EDCs on aquatic environments. Climate change is influencing the rate at which toxic chemicals are released from plastic materials, stockpiles and polluted sites. Higher temperatures increase the release of persistent organic pollutants (POPs) to air by changing their rate of partitioning between air and soil, and between air, water and sediment. POPs persist in the environment, bioaccumulate through the food chain, and pose a threat of causing detrimental effects to human health (298). The fate of environmental POPs in these ecosystems is controlled mainly by temperature and biogeochemical processes. Climate change may increase the re-emissions and redistribution of POPs in different environmental settings via soil–air exchange. Soil–air exchange is a main process of controlling distribution of POPs and terrestrial ecosystem carbon at local and universal scales.
Each POP has a moving potential in air and/or water and can bioaccumulate in biological system such as lipid-rich tissues and biomagnified through terrestrial and aquatic food chains (299). Hence, POPs have a potential adverse impact on higher trophic organisms in aquatic as well as environment (300–302) and on human health (303). Moreover, intensification of global warming, an increase in POPs levels has been reported in different settings of environment because of the release from reservoirs (299, 304). These higher emissions induced by climate change would increase the susceptibility of exposed animals and humans via food chain and lead to greater adverse effects on human health and terrestrial ecosystems (305).
While the researcher's grapples with the problem of ever-increasing environmental pollution, at the same time, the causes of climate change and its impacts on the fate and toxicity of chemicals must be addressed.
EDC Regulations in Various Countries
The issue of EDCs is puzzled due to lack of scientific data and many gaps in knowledge, it is still essential to frame regulatory policies to deal with the present scenario. Framing of policies and regulations must go parallel with ongoing research and any measure must be compliant to rapid advances in scientific knowledge. At present countries having regulations addressing the manufacture, use and disposal of various chemicals includes carcinogens, teratogens, mutagens and substances that interrupt reproduction. EDCs represent a relatively new classification, involving diverse chemical classes that are able to mimic endogenous hormones. Although there is no agreement on their regulation, EDCs are addressed in different cases in EU law, such as the Water Framework Directive, Registration, Evaluation and Authorization of Chemicals (REACH), Plant Protection Products Regulation (PPPR) and Cosmetic Regulation (Table 2). The government and regulatory agencies of developing countries with large population and huge plastic consumers may restrict the use of endocrine disrupting chemicals in the production of cosmetics, food-contact plastics, plastic water tanks and packaging materials and may provide guidelines on the use of EDC's/plastics, awareness on its harmful impacts and the use of risk-free alternatives must be provided, with an intention to ensures the safety of vulnerable group such as infants, children and pregnant women. The challenges that arise from the field of endocrine disruption are the immense diversity of chemicals produced, but not tested, the mixtures and the unknown interactions between them, together with their consequent effects. The lack of an effective and strong legislation and regulation poses a significant threat to humans, animals and plants, and contributes to the exposure to chemicals that may disrupt the endocrine system.
Conclusions
Most of the information on the detrimental effect of EDCs comes from animal studies. It is becoming more evident that EDCs may trigger disorders such as metabolic diseases, reproductive abnormalities, endocrine dysfunction and cancers. The sudden escalating rates of these diseases, especially metabolic disorders, correlate with global industrialization and the production and release of EDCs to the environment, drinking water and eventually to food chain. Countries should formulate acts and guidelines including EDCs for the safety of drinking water. To understand the exposure routes of EDCs better, a comprehensive assessment of drinking water supply is required. Exposure to EDC or mixtures, even at low doses, especially during critical window or early development can have an impact on endocrine system by altering transgenerational/epigenetic pathway. Hence, further studies are required to establish the threshold concentrations of EDCs in the environment as well as in human matrixes (bio monitoring) below which its detrimental effects do not occur. Large-scale systematic epidemiology studies need to be carried out taking into consideration the low dose effect and cocktail effect of EDCs.
Author Contributions
MKumar, MKumawat, SS, and DS: manuscript draft. RT and AP: overall review. VV: manuscript drafting and editing. All authors contributed to the article and approved the submitted version.
Funding
This study was supported by Indian Council of Medical Research grant no: NIREH//BPL/PJ-30/2018-19/127. The funders had no role in study design, data collection and analysis, decision to publish, or preparation of the manuscript.
Conflict of Interest
The authors declare that the research was conducted in the absence of any commercial or financial relationships that could be construed as a potential conflict of interest.
References
1. Calafat AM, Needham LL. Human exposures and body burdens of endocrine-disrupting chemicals. In: Endocrine-Disrupting Chemicals. Humana Press (2007) 253–268. doi: 10.1007/1-59745-107-x_11
2. Porte C, Janer G, Lorusso L, Ortiz-Zarragoitia M, Cajaraville M, Fossi M, et al. Endocrine disruptors in marine organisms, approaches and perspectives. Comp Biochem Physiol C Toxicol Pharmacol. (2006) 143:303–15. doi: 10.1016/j.cbpc.2006.03.004
3. Kim BY, Rutka JT, Chan WC. Nanomedicine. N Engl J Med. (2010) 363:2434–43. doi: 10.1056/NEJMra0912273
4. Hardy A, Benford D, Halldorsson T, Jeger MJ, Knutsen HK, More S, et al. Guidance on risk assessment of the application of nanoscience and nanotechnologies in the food and feed chain, Part. 1, human and animal health. EFSA J. (2018) 16:e05327. doi: 10.2903/j.efsa.2018.5327
5. Lagaron JM, Lopez-Rubio A. Nanotechnology for bioplastics, opportunities, challenges and strategies. Trends Food Sci Technol. (2011) 22:611–7. doi: 10.1016/j.tifs.2011.01.007
6. Carbery M, O'Connor W, Palanisami T. Trophic transfer of microplastics and mixed contaminants in the marine food web and implications for human health. Environ Int. (2018) 115:400–9. doi: 10.1016/j.envint.2018.03.007
7. Talsness CE, Andrade AJ, Kuriyama SN, Taylor JA, vom Saal SF. Components of plastic, experimental studies in animals and relevance for human health. Philos Trans R Soc Lond B Biol Sci. (2009) 364:2079–96. doi: 10.1098/rstb.2008.0281
8. Melzer D, Osborne NJ, Henley WE, Cipelli R, Young A, Money C, et al. Urinary bisphenol a concentration and risk of future coronary artery disease in apparently healthy men and women. Circulation. (2012) 125:1482–90. doi: 10.1161/CIRCULATIONAHA.111.069153
9. Velmurugan G, Ramprasath T, Gilles M, Swaminathan K, Ramasamy S. Gut microbiota, endocrine-disrupting chemicals, and the diabetes epidemic. Trends Endocrinol Metab. (2017) 28:612–25. doi: 10.1016/j.tem.2017.05.001
10. Jambeck JR, Geyer R, Wilcox C, Siegler TR, Perryman M, Andrady A, et al. Plastic waste inputs from land into the ocean. Science. (2015) 347:768–71. doi: 10.1126/science.1260352
11. GBD 2013 DALYs and HALE Collaborators, Murray CJ, Barber RM, Foreman KJ, Abbasoglu Ozgoren A, et al. Global, regional, and national disability-adjusted life years (DALYs) for 306 diseases and injuries and healthy life expectancy (HALE) for 188 countries, 1990-2013, quantifying the epidemiological transition. Lancet. (2015) 386:2145–91. doi: 10.1016/S0140-6736(15)61340-X
12. Stevens GA, Singh GM, Lu Y, Danaei G, Lin JK, Finucane MM, et al. National, regional, and global trends in adult overweight and obesity prevalences. Popul Health Metr. (2012) 10:22. doi: 10.1186/1478-7954-10-22
13. McAllister EJ, Dhurandhar NV, Keith SW, Aronne LJ, Barger J, Baskin M, et al. Ten putative contributors to the obesity epidemic. Crit Rev Food Sci Nutr. (2009) 49:868–913. doi: 10.1080/10408390903372599
14. Baillie-Hamilton PF. Chemical toxins, a hypothesis to explain the global obesity epidemic. J Altern Complement Med. (2002) 8:185–92. doi: 10.1089/107555302317371479
15. Elobeid MA, Padilla MA, Brock DW, Ruden DM, Allison DB. Endocrine disruptors and obesity, an examination of selected persistent organic pollutants in the NHANES 1999-2002 data. Int J Environ Res Public Health. (2010) 7:2988–3005. doi: 10.3390/ijerph7072988
16. Halldorsson TI, Rytter D, Haug LS, Bech BH, Danielsen I, Becher G, et al. Prenatal exposure to perfluorooctanoate and risk of overweight at 20 years of age, a prospective cohort study. Environ Health Perspect. (2012) 120:668–73. doi: 10.1289/ehp.1104034
17. Vafeiadi M, Georgiou V, Chalkiadaki G, Rantakokko P, Kiviranta H, Karachaliou M, et al. Association of prenatal exposure to persistent organic pollutants with obesity and cardiometabolic traits in early childhood, the rhea mother-child cohort (Crete, Greece). Environ Health Perspect. (2015) 123:1015–21. doi: 10.1289/ehp.1409062
18. Lauritzen HB, Larose TL, Øien T, Sandanger TM, Odland JØ, Van De Bor M, et al. Prenatal exposure to persistent organic pollutants and child overweight/obesity at 5-year follow-up, a prospective cohort study. Environ Health. (2018) 17:9. doi: 10.1186/s12940-017-0338-x
19. Liu B, Lehmler HJ, Sun Y, Xu G, Liu Y, Zong G, et al. Bisphenol a substitutes and obesity in US adults, analysis of a population-based, cross-sectional study. Lancet Planet Health. (2017) 1:e114–22. doi: 10.1016/S2542-5196(17)30049-9
20. Yang TC, Peterson KE, Meeker JD, Sánchez BN, Zhang Z, Cantoral A, et al. Bisphenol a and phthalates in utero and in childhood, association with child BMI z-score and adiposity. Environ Res. (2017) 156:326–33. doi: 10.1016/j.envres.2017.03.038
21. Valvi D, Casas M, Romaguera D, Monfort N, Ventura R, Martinez D, et al. Prenatal phthalate exposure and childhood growth and blood pressure, evidence from the Spanish INMA-Sabadell birth cohort study. Environ Health Perspect. (2015) 123:1022–9. doi: 10.1289/ehp.1408887
22. Buckley JP, Engel SM, Braun JM, Whyatt RM, Daniels JL, Mendez MA, et al. Prenatal phthalate exposures and body mass index among 4 to 7 year old children, a pooled analysis. Epidemiology. (2016) 27:449. doi: 10.1097/EDE.0000000000000436
23. Yang P, Sun H, Gong Y-J, Wang Y-X, Liu C, Chen Y-J, et al. Repeated measures of urinary polycyclic aromatic hydrocarbon metabolites in relation to altered reproductive hormones, A cross-sectional study in China. Int J Hyg Environ Health. (2017) 220:1340–6. doi: 10.1016/j.ijheh.2017.09.004
24. Janesick A, Blumberg B. Endocrine disrupting chemicals and the developmental programming of adipogenesis and obesity. Birth Defect Res C Embryo Today. (2011) 93:34–50. doi: 10.1002/bdrc.20197
25. Janesick A, Blumberg B. Obesogens, stem cells and the developmental programming of obesity. Int J Androl. (2012) 35:437–48. doi: 10.1111/j.1365-2605.2012.01247.x
26. Grün F, Blumberg B. Environmental obesogens, organotins and endocrine disruption via nuclear receptor signaling. Endocrinology. (2006) 147:s50–5. doi: 10.1210/en.2005-1129
27. Ben-Jonathan N, Steinmetz R. Xenoestrogens, the emerging story of bisphenol a. Trends Endocrinol Metab. (1998) 9:124–8. doi: 10.1016/S1043-2760(98)00029-0
28. Lee DH, Lind PM, Jacobs DR Jr., Salihovic S, van Bavel B, Lind L. Polychlorinated biphenyls and organochlorine pesticides in plasma predict development of type. 2 diabetes in the elderly, the prospective investigation of the vasculature in Uppsala Seniors (PIVUS) study. Diabetes Care. (2011) 34:1778–84. doi: 10.2337/dc10-2116
29. Rignell-Hydbom A, Lidfeldt J, Kiviranta H, Rantakokko P, Samsioe G, Agardh C-D, et al. Exposure to p, p′-DDE, a risk factor for type 2 diabetes. PLoS ONE. (2009) 4:e7503. doi: 10.1371/journal.pone.0007503
30. Lee DH, Porta M, Jacobs DR Jr., Vandenberg LN. Chlorinated persistent organic pollutants, obesity, and type 2 diabetes. Endocr Rev. (2014) 35:557–601. doi: 10.1210/er.2013-1084
31. Turyk M, Anderson H, Knobeloch L, Imm P, Persky V. Organochlorine exposure and incidence of diabetes in a cohort of Great Lakes sport fish consumers. Environ Health Perspect. (2009) 117:1076–82. doi: 10.1289/ehp.0800281
32. Wang SL, Tsai PC, Yang CY, Guo YL. Increased risk of diabetes and polychlorinated biphenyls and dioxins, a 24-year follow-up study of the Yucheng cohort. Diabetes Care. (2008) 31:1574–9. doi: 10.2337/dc07-2449
33. Wu H, Bertrand KA, Choi AL, Hu FB, Laden F, Grandjean P, et al. Persistent organic pollutants and type 2 diabetes, a prospective analysis in the nurses' health study and meta-analysis. Environ Health Perspect. (2013) 121:153–61. doi: 10.1289/ehp.1205248
34. Shankar A, Teppala S. Relationship between urinary bisphenol a levels and diabetes mellitus. J Clin Endocrinol Metab. (2011) 96:3822–26. doi: 10.1210/jc.2011-1682
35. Beydoun HA, Khanal S, Zonderman AB, Beydoun MA. Sex differences in the association of urinary bisphenol-A concentration with selected indices of glucose homeostasis among US. adults. Ann Epidemiol. (2014) 24:90–7. doi: 10.1016/j.annepidem.2013.07.014
36. Wang T, Li M, Chen B, Xu M, Xu Y, Huang Y, et al. Urinary bisphenol A (BPA) concentration associates with obesity and insulin resistance. J Clin Endocrinol Metab. (2012) 97:E223–7. doi: 10.1210/jc.2011-1989
37. Sun Q, Cornelis MC, Townsend MK, Tobias DK, Eliassen AH, Franke AA, et al. Association of urinary concentrations of bisphenol A and phthalate metabolites with risk of type 2 diabetes, a prospective investigation in the Nurses' Health Study (NHS) and NHSII cohorts. Environ Health Perspect. (2014) 122:616–23. doi: 10.1289/ehp.1307201
38. Lee D-H, Steffes MW, Sjödin A, Jones RS, Needham LL, Jacobs RD Jr. Low dose of some persistent organic pollutants predicts type 2 diabetes, a nested case–control study. Environ Health Perspect. (2010) 118:1235–42. doi: 10.1289/ehp.0901480
39. James-Todd TM, Meeker JD, Huang T, Hauser R, Ferguson KK, Rich-Edwards JW, et al. Pregnancy urinary phthalate metabolite concentrations and gestational diabetes risk factors. Environ Int. (2016) 96:118–26. doi: 10.1016/j.envint.2016.09.009
40. Fisher BG, Frederiksen H, Andersson A-M, Juul A, Thankamony A, Ong KK, et al. Serum phthalate and triclosan levels have opposing associations with risk factors for gestational diabetes mellitus. Front Endocrinol. (2018) 9:99. doi: 10.3389/fendo.2018.00099
41. Shapiro GD, Dodds L, Arbuckle TE, Ashley-Martin J, Fraser W, Fisher M, et al. Exposure to phthalates, bisphenol A and metals in pregnancy and the association with impaired glucose tolerance and gestational diabetes mellitus, the MIREC study. Environ Int. (2015) 83:63–71. doi: 10.1016/j.envint.2015.05.016
42. Vafeiadi M, Roumeliotaki T, Chalkiadaki G, Rantakokko P, Kiviranta H, Fthenou E, et al. Persistent organic pollutants in early pregnancy and risk of gestational diabetes mellitus. Environ Int. (2017) 98:89–95. doi: 10.1016/j.envint.2016.10.005
43. Verstraete SG, Wojcicki JM, Perito ER, Rosenthal P. Bisphenol a increases risk for presumed non-alcoholic fatty liver disease in hispanic adolescents in NHANES 2003-2010. Environ Health. (2018) 17:12. doi: 10.1186/s12940-018-0356-3
44. Min JY, Cho JS, Lee KJ, Park JB, Park SG, Kim JY, et al. Potential role for organochlorine pesticides in the prevalence of peripheral arterial diseases in obese persons, results from the National Health and Nutrition Examination Survey 1999-2004. Atherosclerosis. (2011) 218:200–6. doi: 10.1016/j.atherosclerosis.2011.04.044
45. La Merrill M, Cirillo PM, Terry MB, Krigbaum NY, Flom JD, Cohn BA. Prenatal exposure to the pesticide DDT and hypertension diagnosed in women before age 50, a longitudinal birth cohort study. Environ Health Perspect. (2013) 121:594–99. doi: 10.1289/ehp.1205921
46. Siddiqui MK, Nigam U, Srivastava S, Tejeshwar D, Chandrawati. Association of maternal blood pressure and hemoglobin level with organochlorines in human milk. Hum Exp Toxicol. (2002) 21:1–6. doi: 10.1191/0960327102ht198oa
47. Bae S, Hong YC. Exposure to bisphenol A from drinking canned beverages increases blood pressure, randomized crossover trial. Hypertension. (2015) 65:313–9. doi: 10.1161/HYPERTENSIONAHA.114.04261
48. Bae S, Kim JH, Lim YH, Park HY, Hong YC. Associations of bisphenol A exposure with heart rate variability and blood pressure. Hypertension. (2012) 60:786–93. doi: 10.1161/HYPERTENSIONAHA.112.197715
49. Desvergne B, Feige JN, Casals-Casas C. PPAR-mediated activity of phthalates, A link to the obesity epidemic? Mol Cell Endocrinol. (2009) 304:43–8. doi: 10.1016/j.mce.2009.02.017
50. Chamorro-García R, Kirchner S, Li X, Janesick A, Casey SC, Chow C, et al. Bisphenol A diglycidyl ether induces adipogenic differentiation of multipotent stromal stem cells through a peroxisome proliferator–activated receptor gamma-independent mechanism. Environ Health Perspect. (2012) 120:984–89. doi: 10.1289/ehp.1205063
51. Ropero AB, Alonso-Magdalena P, Garcia-Garcia E, Ripoll C, Fuentes E, Nadal A. Bisphenol-A disruption of the endocrine pancreas and blood glucose homeostasis. Int J Androl. (2008) 31:194–200. doi: 10.1111/j.1365-2605.2007.00832.x
52. Lin Y, Wei J, Li Y, Chen J, Zhou Z, Song L, et al. Developmental exposure to di(2-ethylhexyl) phthalate impairs endocrine pancreas and leads to long-term adverse effects on glucose homeostasis in the rat. Am J Physiol Endocrinol Metab. (2011) 301:E527–38. doi: 10.1152/ajpendo.00233.2011
53. Soriano S, Alonso-Magdalena P, Garcia-Arevalo M, Novials A, Muhammed SJ, Salehi A, et al. Rapid insulinotropic action of low doses of bisphenol-A on mouse and human islets of Langerhans, role of estrogen receptor beta. PLoS ONE. (2012) 7:e31109. doi: 10.1371/journal.pone.0031109
54. Asakawa A, Toyoshima M, Fujimiya M, Harada K, Ataka K, Inoue K, et al. Perfluorooctane sulfonate influences feeding behavior and gut motility via the hypothalamus. Int J Mol Med. (2007) 19:733–9. doi: 10.3892/ijmm.19.5.733
55. Walker DM, Goetz BM, Gore AC. Dynamic postnatal developmental and sex-specific neuroendocrine effects of prenatal polychlorinated biphenyls in rats. Mol Endocrinol. (2014) 28:99–115. doi: 10.1210/me.2013-1270
56. van Esterik JC, Dolle ME, Lamoree MH, van Leeuwen SP, Hamers T, Legler J, et al. Programming of metabolic effects in C57BL/6JxFVB mice by exposure to bisphenol A during gestation and lactation. Toxicology. (2014) 321:40–52. doi: 10.1016/j.tox.2014.04.001
57. Johnson S, Painter M, Javurek A, Ellersieck M, Wiedmeyer C, Thyfault J, et al. Sex-dependent effects of developmental exposure to bisphenol A and ethinyl estradiol on metabolic parameters and voluntary physical activity. J Dev Origins Health Dis. (2015) 6:539–52. doi: 10.1017/S2040174415001488
58. Minatoya M, Sasaki S, Araki A, Miyashita C, Itoh S, Yamamoto J, et al. Cord blood bisphenol a levels and reproductive and thyroid hormone levels of neonates, the hokkaido study on environment and children's health. Epidemiology. (2017) 28(Suppl.1):S3–9. doi: 10.1097/EDE.0000000000000716
59. Trasande L, Sathyanarayana S, Spanier AJ, Trachtman H, Attina TM, Urbina EM. Urinary phthalates are associated with higher blood pressure in childhood. J Pediatr. (2013) 163:747–53.e1. doi: 10.1016/j.jpeds.2013.03.072
60. Chang J-W, Ou H-Y, Chen H-L, Guo H-R, Liao P-C, Lee C-C. Interrelationship between exposure to PCDD/Fs and hypertension in metabolic syndrome in Taiwanese living near a highly contaminated area. Chemosphere. (2010) 81:1027–32. doi: 10.1016/j.chemosphere.2010.08.050
61. Karouna-Renier NK, Rao KR, Lanza JJ, Davis DA, Wilson PA. Serum profiles of PCDDs and PCDFs, in individuals near the Escambia wood treating company superfund site in pensacola, FL. Chemosphere. (2007) 69:1312–9. doi: 10.1016/j.chemosphere.2007.05.028
62. Yorita Christensen KL, White P. A methodological approach to assessing the health impact of environmental chemical mixtures, PCBs and hypertension in the National Health and Nutrition Examination Survey. Int J Environ Res Public Health. (2011) 8:4220–37. doi: 10.3390/ijerph8114220
63. De Coster S, Van Larebeke N. Endocrine-disrupting chemicals, associated disorders and mechanisms of action. J Environ Public Health. (2012) 2012:713696. doi: 10.1155/2012/713696
64. Morgan M, Deoraj A, Felty Q, Yoo C, Roy D. Association between exposure to estrogenic endocrine disruptors-polychlorinated biphenyls, phthalates, and bisphenol A and gynecologic cancers-cervical, ovarian, uterine cancers. J. Carcinogen. Mutagen. (2016) 7:1–14. doi: 10.4172/2157-2518.1000275
65. Schoeters G, Den Hond E, Dhooge W, van Larebeke N, Leijs M. Endocrine disruptors and abnormalities of pubertal development. Basic Clin Pharmacol Toxicol. (2008) 102:168–75. doi: 10.1111/j.1742-7843.2007.00180.x
66. Hauser R, Gaskins AJ, Souter I, Smith KW, Dodge LE, Ehrlich S, et al. Urinary phthalate metabolite concentrations and reproductive outcomes among women undergoing in vitro fertilization, results from the EARTH study. Environ Health Perspect. (2016) 124:831–9. doi: 10.1289/ehp.1509760
67. Kane L, Ismail N. Puberty as a vulnerable period to the effects of immune challenges, focus on sex differences. Behav Brain Res. (2017) 320:374–82. doi: 10.1016/j.bbr.2016.11.006
68. Parent AS, Teilmann G, Juul A, Skakkebaek NE, Toppari J, Bourguignon JP. The timing of normal puberty and the age limits of sexual precocity, variations around the world, secular trends, and changes after migration. Endocr Rev. (2003) 24:668–93. doi: 10.1210/er.2002-0019
69. Ozen S, Darcan S. Effects of environmental endocrine disruptors on pubertal development. J Clin Res Pediatr Endocrinol. (2011) 3:1–6. doi: 10.4274/jcrpe.v3i1.01
70. Poursafa P, Ataei E, Kelishadi R. A systematic review on the effects of environmental exposure to some organohalogens and phthalates on early puberty. J Res Med Sci. (2015) 20:613. doi: 10.4103/1735-1995.165971
71. Millar RP, Babwah AV. KISS1R: hallmarks of an effective regulator of the neuroendocrine axis. Neuroendocrinology. (2015) 101:193–210. doi: 10.1159/000381457
72. Paris F, Servant N, Térouanne B, Balaguer P, Nicolas JC, Sultan C. A new recombinant cell bioassay for ultrasensitive determination of serum estrogenic bioactivity in children. J Clin Endocrinol Metab. (2002) 87:791–7. doi: 10.1210/jcem.87.2.8269
73. Pigneur B, Trivin C, Brauner R. Idiopathic central precocious puberty in 28 boys. Med Sci Monit. (2008) 14:CR10–4.
74. Srilanchakon K, Thadsri T, Jantarat C, Thengyai S, Nosoognoen W, Supornsilchai V. Higher phthalate concentrations are associated with precocious puberty in normal weight Thai girls. J Pediatr Endocrinol Metab. (2017) 30:1293–8. doi: 10.1515/jpem-2017-0281
75. Wolff MS, Pajak A, Pinney SM, Windham GC, Galvez M, Rybak M, et al. Associations of urinary phthalate and phenol biomarkers with menarche in a multiethnic cohort of young girls. Reprod Toxicol. (2017) 67:56–64. doi: 10.1016/j.reprotox.2016.11.009
76. Bulus AD, Asci A, Erkekoglu P, Balci A, Andiran N, Kocer-Gumusel B. The evaluation of possible role of endocrine disruptors in central and peripheral precocious puberty. Toxicol Mech Methods. (2016) 26:493–500. doi: 10.3109/15376516.2016.1158894
77. Shi H, Cao Y, Shen Q, Zhao Y, Zhang Z, Zhang Y. Association between urinary phthalates and pubertal timing in Chinese adolescents. J Epidemiol. (2015) 25:574–82. doi: 10.2188/jea.JE20140205
78. Miao M, Wang Z, Liu X, Liang H, Zhou Z, Tan H, et al. Urinary bisphenol A and pubertal development in Chinese school-aged girls, a cross-sectional study. Environ Health. (2017) 16:80. doi: 10.1186/s12940-017-0290-9
79. Supornsilchai V, Jantarat C, Nosoognoen W, Pornkunwilai S, Wacharasindhu S, Soder O. Increased levels of bisphenol A (BPA) in Thai girls with precocious puberty. J Pediatr Endocrinol Metab. (2016) 29:1233–9. doi: 10.1515/jpem-2015-0326
80. Ozen S, Darcan S, Bayindir P, Karasulu E, Simsek DG, Gurler T. Effects of pesticides used in agriculture on the development of precocious puberty. Environ Monitor Assess. (2012) 184:4223–32. doi: 10.1007/s10661-011-2257-6
81. Deng F, Tao FB, Liu DY, Xu YY, Hao JH, Sun Y, et al. Effects of growth environments and two environmental endocrine disruptors on children with idiopathic precocious puberty. Eur J Endocrinol. (2012) 166:803–9. doi: 10.1530/EJE-11-0876
82. Vasiliu O, Muttineni J, Karmaus W. In utero exposure to organochlorines and age at menarche. Hum Reprod. (2004) 19:1506–12. doi: 10.1093/humrep/deh292
83. Axmon A. Menarche in women with high exposure to persistent organochlorine pollutants in utero and during childhood. Environ Res. (2006) 102:77–82. doi: 10.1016/j.envres.2005.12.014
84. Lomenick JP, Calafat AM, Melguizo Castro MS, Mier R, Stenger P, Foster MB, et al. Phthalate exposure and precocious puberty in females. J Pediatr. (2010) 156:221–5. doi: 10.1016/j.jpeds.2009.09.047
85. McGuinn LA, Ghazarian AA, Su LJ, Ellison GL. Urinary bisphenol A and age at menarche among adolescent girls, evidence from NHANES 2003–2010. Environ Res. (2015) 136:381–6. doi: 10.1016/j.envres.2014.10.037
86. Harley KG, Rauch SA, Chevrier J, Kogut K, Parra KL, Trujillo C, et al. Bradman, et al. Association of prenatal and childhood PBDE exposure with timing of puberty in boys and girls. Environ Int. (2017) 100:132–8. doi: 10.1016/j.envint.2017.01.003
87. Kasper-Sonnenberg M, Wittsiepe J, Wald K, Koch HM, Wilhelm M. Pre-pubertal exposure with phthalates and bisphenol A and pubertal development. PLoS ONE. (2017) 12:e0187922. doi: 10.1371/journal.pone.0187922
88. Watkins DJ, Sanchez BN, Tellez-Rojo MM, Lee JM, Mercado-Garcia A, Blank-Goldenberg C, et al. Phthalate and bisphenol A exposure during in utero windows of susceptibility in relation to reproductive hormones and pubertal development in girls. Environ Res. (2017) 159:143–51. doi: 10.1016/j.envres.2017.07.051
89. Du Y-Y, Fang Y-L, Wang Y-X, Zeng Q, Guo N, Zhao H, et al. Follicular fluid and urinary concentrations of phthalate metabolites among infertile women and associations with in vitro fertilization parameters. Reprod Toxicol. (2016) 61:142–50. doi: 10.1016/j.reprotox.2016.04.005
90. Buck Louis GM, Sundaram R, Sweeney AM, Schisterman EF, Maisog J, Kannan K. Urinary bisphenol A, phthalates, couple fecundity. The Longitudinal Investigation of Fertility and the Environment (LIFE) study. Fertil Steril. (2014) 101:1359–66. doi: 10.1016/j.fertnstert.2014.01.022
91. Zhou W, Fang F, Zhu W, Chen Z-J, Du Y, Zhang J. Bisphenol A and ovarian reserve among infertile women with polycystic ovarian syndrome. Int J Environ Res Public Health. (2017) 14:18. doi: 10.3390/ijerph14010018
92. Souter I, Smith KW, Dimitriadis I, Ehrlich S, Williams PL, Calafat AM, et al. The association of bisphenol-A urinary concentrations with antral follicle counts and other measures of ovarian reserve in women undergoing infertility treatments. Reprod Toxicol. (2013) 42:224–31. doi: 10.1016/j.reprotox.2013.09.008
93. Kandaraki E, Chatzigeorgiou A, Livadas S, Palioura E, Economou F, Koutsilieris M, et al. Endocrine disruptors and polycystic ovary syndrome (PCOS), elevated serum levels of bisphenol A in women with PCOS. J Clin Endocrinol Metab. (2011) 96:E480–4. doi: 10.1210/jc.2010-1658
94. Rashidi BH, Amanlou M, Lak TB, Ghazizadeh M, Haghollahi F, Bagheri M, et al. The association between bisphenol a and polycystic ovarian syndrome, a case-control study. Acta Med Iranica. (2017) 55:759–64.
95. Ehrlich S, Williams PL, Missmer SA, Flaws JA, Berry KF, Calafat AM, et al. Urinary bisphenol A concentrations and implantation failure among women undergoing in vitro fertilization. Environ Health Perspect. (2012) 120:978–83. doi: 10.1289/ehp.1104307
96. AL-Hussaini TK, Abdelaleem AA, Elnashar I, Shabaan OM, Mostafa R, El-Baz MA, et al. The effect of follicullar fluid pesticides and polychlorinated biphenyls concentrations on intracytoplasmic sperm injection (ICSI) embryological and clinical outcome. Eur J Obstetr Gynecol Reprod Biol. (2018) 220:39–43. doi: 10.1016/j.ejogrb.2017.11.003
97. Vagi SJ, Azziz-Baumgartner E, Sjödin A, Calafat AM, Dumesic D, Gonzalez L, et al. Exploring the potential association between brominated diphenyl ethers, polychlorinated biphenyls, organochlorine pesticides, perfluorinated compounds, phthalates, and bisphenol a in polycystic ovary syndrome, a case–control study. BMC Endocr Disord. (2014) 14:86. doi: 10.1186/1472-6823-14-86
98. Guo Z, Qiu H, Wang L, Wang L, Wang C, Chen M, et al. Association of serum organochlorine pesticides concentrations with reproductive hormone levels and polycystic ovary syndrome in a Chinese population. Chemosphere. (2017) 171:595–600. doi: 10.1016/j.chemosphere.2016.12.127
99. Grindler NM, Allsworth JE, Macones GA, Kannan K, Roehl KA, Cooper AR. Persistent organic pollutants and early menopause in US women. PLoS ONE. (2015) 10:e0116057. doi: 10.1371/journal.pone.0116057
100. Cobellis L, Colacurci N, Trabucco E, Carpentiero C, Grumetto L. Measurement of bisphenol A and bisphenol B levels in human blood sera from healthy and endometriotic women. Biomed Chromatogr. (2009) 23:1186–90. doi: 10.1002/bmc.1241
101. Kim SH, Chun S, Jang JY, Chae HD, Kim CH, Kang BM. Increased plasma levels of phthalate esters in women with advanced-stage endometriosis, a prospective case-control study. Fertil Steril. (2011) 95:357–9. doi: 10.1016/j.fertnstert.2010.07.1059
102. Huang P-C, Tsai E-M, Li W-F, Liao P-C, Chung M-C, Wang Y-H, et al. Association between phthalate exposure and glutathione S-transferase M1 polymorphism in adenomyosis, leiomyoma and endometriosis. Hum Reprod. (2010) 25:986–94. doi: 10.1093/humrep/deq015
103. Buck Louis GM, Peterson CM, Chen Z, Croughan M, Sundaram R, Stanford J, Varner MW, et al. Bisphenol A and phthalates and endometriosis, the endometriosis, natural history, diagnosis and outcomes study. Fertil Steril. (2013) 100:162–9.e1–2. doi: 10.1016/j.fertnstert.2013.03.026
104. Ploteau S, Cano-Sancho G, Volteau C, Legrand A, Vénisseau A, Vacher V, et al. Associations between internal exposure levels of persistent organic pollutants in adipose tissue and deep infiltrating endometriosis with or without concurrent ovarian endometrioma. Environ Int. (2017) 108:195–203. doi: 10.1016/j.envint.2017.08.019
105. Upson K, De Roos AJ, Thompson ML, Sathyanarayana S, Scholes D, Barr DB, et al. Organochlorine pesticides and risk of endometriosis, findings from a population-based case-control study. Environ Health Perspect. (2013) 121:1319–24. doi: 10.1289/ehp.1306648
106. Itoh H, Iwasaki M, Hanaoka T, Sasaki H, Tanaka T, Tsugane S. Urinary phthalate monoesters and endometriosis in infertile Japanese women. Sci Total Environ. (2009) 408:37–42. doi: 10.1016/j.scitotenv.2009.09.012
107. Upson K, Sathyanarayana S, De Roos AJ, Thompson ML, Scholes D, Dills R, et al. Phthalates and risk of endometriosis. Environ Res. (2013) 126:91–7. doi: 10.1016/j.envres.2013.07.003
108. Wen X, Xiong Y, Qu X, Jin L, Zhou C, Zhang M, et al. The risk of endometriosis after exposure to endocrine-disrupting chemicals, a meta-analysis of 30 epidemiology studies. Gynecol Endocrinol. (2019) 35:645–50. doi: 10.1080/09513590.2019.1590546
109. Miao M, Yuan W, Zhu G, He X, Li DK. In utero exposure to bisphenol-A and its effect on birth weight of offspring. Reprod Toxicol. (2011) 32:64–8. doi: 10.1016/j.reprotox.2011.03.002
110. Chou WC, Chen JL, Lin CF, Chen YC, Shih FC, Chuang CY. Biomonitoring of bisphenol A concentrations in maternal and umbilical cord blood in regard to birth outcomes and adipokine expression, a birth cohort study in Taiwan. Environ Health. (2011) 10:94. doi: 10.1186/1476-069X-10-94
111. Huo W, Xia W, Wan Y, Zhang B, Zhou A, Zhang Y, et al. Maternal urinary bisphenol A levels and infant low birth weight, a nested case–control study of the health baby cohort in China. Environ Int. (2015) 85:96–103. doi: 10.1016/j.envint.2015.09.005
112. Veiga-Lopez A, Kannan K, Liao C, Ye W, Domino SE, Padmanabhan V. Gender-specific effects on gestational length and birth weight by early pregnancy BPA exposure. J Clin Endocrinol Metab. (2015) 100:E1394–403. doi: 10.1210/jc.2015-1724
113. Ding G, Wang C, Vinturache A, Zhao S, Pan R, Han W, et al. Prenatal low-level phenol exposures and birth outcomes in China. Sci Total Environ. (2017) 607–8:1400–7. doi: 10.1016/j.scitotenv.2017.07.084
114. Shoaff JR, Romano ME, Yolton K, Lanphear BP, Calafat AM, Braun JM. Prenatal phthalate exposure and infant size at birth and gestational duration. Environ Res. (2016) 150:52–8. doi: 10.1016/j.envres.2016.05.033
115. Smarr MM, Grantz KL, Sundaram R, Maisog JM, Kannan K, Louis GM. Parental urinary biomarkers of preconception exposure to bisphenol A and phthalates in relation to birth outcomes. Environ Health. (2015) 14:73. doi: 10.1186/s12940-015-0060-5
116. Wu C, Huo W, Li Y, Zhang B, Wan Y, Zheng T, et al. Maternal urinary paraben levels and offspring size at birth from a Chinese birth cohort. Chemosphere. (2017) 172:29–36. doi: 10.1016/j.chemosphere.2016.12.131
117. Woods MM, Lanphear BP, Braun JM, McCandless LC. Gestational exposure to endocrine disrupting chemicals in relation to infant birth weight, a Bayesian analysis of the HOME study. Environ Health. (2017) 16:115. doi: 10.1186/s12940-017-0332-3
118. Sharpe RM, Skakkebaek NE. Are oestrogens involved in falling sperm counts and disorders of the male reproductive tract? Lancet. (1993) 341:1392–5. doi: 10.1016/0140-6736(93)90953-E
119. Sidorkiewicz I, Zareba K, Wolczynski S, Czerniecki J. Endocrine-disrupting chemicals-Mechanisms of action on male reproductive system. Toxicol Ind Health. (2017) 33:601–9. doi: 10.1177/0748233717695160
120. Xie C, Zhao Y, Gao L, Chen J, Cai D, Zhang Y. Elevated phthalates' exposure in children with constitutional delay of growth and puberty. Mol Cell Endocrinol. (2015) 407:67–73. doi: 10.1016/j.mce.2015.03.006
121. Ferguson KK, Peterson KE, Lee JM, Mercado-Garcia A, Blank-Goldenberg C, Tellez-Rojo MM, et al. Prenatal and peripubertal phthalates and bisphenol A in relation to sex hormones and puberty in boys. Reprod Toxicol. (2014) 47:70–6. doi: 10.1016/j.reprotox.2014.06.002
122. Hou JW, Lin CL, Tsai YA, Chang CH, Liao KW, Yu CJ, et al. The effects of phthalate and nonylphenol exposure on body size and secondary sexual characteristics during puberty. Int J Hyg Environ Health. (2015) 218:603–15. doi: 10.1016/j.ijheh.2015.06.004
123. Wang Z, Li D, Miao M, Liang H, Chen J, Zhou Z, et al. Urine bisphenol A and pubertal development in boys. Int J Hyg Environ Health. (2017) 220:43–50. doi: 10.1016/j.ijheh.2016.10.004
124. Toppari J, Larsen JC, Christiansen P, Giwercman A, Grandjean P, Guillette LJ, et al. Male reproductive health and environmental xenoestrogens. Environ Health Perspect. (1996) 104(Suppl. 4):741–803. doi: 10.1289/ehp.96104s4741
125. Melgarejo M, Mendiola J, Koch HM, Moñino-García M, Noguera-Velasco JA, Torres-Cantero AM. Associations between urinary organophosphate pesticide metabolite levels and reproductive parameters in men from an infertility clinic. Environ Res. (2015) 137:292–8. doi: 10.1016/j.envres.2015.01.004
126. Aneck-Hahn NH, Schulenburg GW, Bornman MS, Farias P, de Jager C. Impaired semen quality associated with environmental DDT exposure in young men living in a malaria area in the Limpopo Province, South Africa. J Androl. (2007) 28:423–34. doi: 10.2164/jandrol.106.001701
127. Bonde JP, Toft G, Rylander L, Rignell-Hydbom A, Giwercman A, Spano M, et al. Fertility and markers of male reproductive function in Inuit and European populations spanning large contrasts in blood levels of persistent organochlorines. Environ Health Perspect. (2008) 116:269–77. doi: 10.1289/ehp.10700
128. Meeker JD, Ehrlich S, Toth TL, Wright DL, Calafat AM, Trisini AT, et al. Semen quality and sperm DNA damage in relation to urinary bisphenol A among men from an infertility clinic. Reprod Toxicol. (2010) 30:532–9. doi: 10.1016/j.reprotox.2010.07.005
129. Vested A, Ramlau-Hansen CH, Olsen SF, Bonde JP, Kristensen SL, Halldorsson TI, et al. Associations of in utero exposure to perfluorinated alkyl acids with human semen quality and reproductive hormones in adult men. Environ Health Perspect. (2013) 121:453–8. doi: 10.1289/ehp.1205118
130. Bloom M, Whitcomb B, Chen Z, Ye A, Kannan K G. Buck Louis, Associations between urinary phthalate concentrations and semen quality parameters in a general population. Hum Reprod. (2015) 30:2645–57. doi: 10.1093/humrep/dev219
131. Minguez-Alarcon L, Sergeyev O, Burns JS, Williams PL, Lee MM, Korrick SA, et al. A longitudinal study of peripubertal serum organochlorine concentrations and semen parameters in young men, the Russian Children's study. Environ Health Perspect. (2017) 125:460–6. doi: 10.1289/EHP25
132. Toppari J, Virtanen HE, Main KM, Skakkebaek NE. Cryptorchidism and hypospadias as a sign of testicular dysgenesis syndrome (TDS), environmental connection. Birth Defect Res A Clin Mol Teratol. (2010) 88:910–9. doi: 10.1002/bdra.20707
133. Goodyer CG, Poon S, Aleksa K, Hou L, Atehortua V, Carnevale A, et al. A case–control study of maternal polybrominated diphenyl ether (PBDE) exposure and cryptorchidism in Canadian populations. Environ Health Perspect. (2017) 125:057004. doi: 10.1289/EHP522
134. Koskenniemi JJ, Virtanen HE, Kiviranta H, Damgaard IN, Matomäki J, Thorup JM, et al. Association between levels of persistent organic pollutants in adipose tissue and cryptorchidism in early childhood, a case–control study. Environ Health. (2015) 14:78. doi: 10.1186/s12940-015-0065-0
135. Fernández MF, Arrebola JP, Jiménez-Díaz I, Sáenz JM, Molina-Molina JM, Ballesteros O, et al. Bisphenol A and other phenols in human placenta from children with cryptorchidism or hypospadias. Reprod Toxicol. (2016) 59:89–95. doi: 10.1016/j.reprotox.2015.11.002
136. Small CM, DeCaro JJ, Terrell ML, Dominguez C, Cameron LL, Wirth J, et al. Maternal exposure to a brominated flame retardant and genitourinary conditions in male offspring. Environ Health Perspect. (2009) 117:1175–79. doi: 10.1289/ehp.0800058
137. Bhatia R, Shiau R, Petreas M, Weintraub JM, Farhang L, Eskenazi B. Organochlorine pesticides and male genital anomalies in the child health and development studies. Environ Health Perspect. (2005) 113:220–4. doi: 10.1289/ehp.7382
138. Jensen DV, Christensen J, Virtanen HE, Skakkebæk NE, Main KM, Toppari J, et al. No association between exposure to perfluorinated compounds and congenital cryptorchidism, a nested case-control study among 215 boys from Denmark and Finland. Reproduction. (2013) 147:411–7. doi: 10.1530/REP-13-0444
139. Toft G, Jönsson B, Lindh C, Giwercman A, Spano M, Heederik D, et al. Exposure to perfluorinated compounds and human semen quality in Arctic and European populations. Hum Reprod. (2012) 27:2532–40. doi: 10.1093/humrep/des185
140. McGlynn KA, Quraishi SM, Graubard BI, Weber JP, Rubertone MV, Erickson RL. Polychlorinated biphenyls and risk of testicular germ cell tumors. Cancer Res. (2009) 69:1901–9. doi: 10.1158/0008-5472.CAN-08-3935
141. Rocheleau CM, Romitti PA, Dennis LK. Pesticides and hypospadias, a meta-analysis. J Pediatr Urol. (2009) 5:17–24. doi: 10.1016/j.jpurol.2008.08.006
142. Rignell-Hydbom A, Lindh CH, Dillner J, Jönsson BA, Rylander L. A nested case-control study of intrauterine exposure to persistent organochlorine pollutants and the risk of hypospadias. PLoS ONE. (2012) 7:e44767. doi: 10.1371/journal.pone.0044767
143. Carmichael SL, Herring AH, Sjodin A, Jones R, Needham L, Ma C, et al. Hypospadias and halogenated organic pollutant levels in maternal mid-pregnancy serum samples. Chemosphere. (2010) 80:641–6. doi: 10.1016/j.chemosphere.2010.04.055
144. Toft G, Jonsson BA, Bonde JP, Norgaard-Pedersen B, Hougaard DM, Cohen A, et al. Perfluorooctane sulfonate concentrations in amniotic fluid, biomarkers of fetal leydig cell function, and cryptorchidism and hypospadias in Danish Boys (1980-1996). Environ Health Perspect. (2016) 124:151–6. doi: 10.1289/ehp.1409288
145. Braun JM, Muckle G, Arbuckle T, Bouchard MF, Fraser WD, Ouellet E, et al. Associations of prenatal urinary bisphenol a concentrations with child behaviors and cognitive abilities. Environ Health Perspect. (2017) 125:067008. doi: 10.1289/EHP984
146. Lim YH, Bae S, Kim BN, Shin CH, Lee YA, Kim JI, et al. Prenatal and postnatal bisphenol A exposure and social impairment in 4-year-old children. Environ Health. (2017) 16:79. doi: 10.1186/s12940-017-0289-2
147. Braun JM, Kalkbrenner AE, Calafat AM, Yolton K, Ye X, Dietrich KN, et al. Impact of early-life bisphenol A exposure on behavior and executive function in children. Pediatrics. (2011) 128:873–82. doi: 10.1542/peds.2011-1335
148. Perera F, Vishnevetsky J, Herbstman JB, Calafat AM, Xiong W, Rauh V, et al. Prenatal bisphenol a exposure and child behavior in an inner-city cohort. Environ Health Perspect. (2012) 120:1190–4. doi: 10.1289/ehp.1104492
149. Perera F, Nolte ELR, Wang Y, Margolis AE, Calafat AM, Wang S, et al. Bisphenol A exposure and symptoms of anxiety and depression among inner city children at 10–12 years of age. Environ Res. (2016) 151:195–202. doi: 10.1016/j.envres.2016.07.028
150. Rebuli ME, Patisaul HB. Assessment of sex specific endocrine disrupting effects in the prenatal and pre-pubertal rodent brain. J Steroid Biochem Mol Biol. (2016) 160:148–59. doi: 10.1016/j.jsbmb.2015.08.021
151. Ejaredar M, Nyanza EC, Ten Eycke K, Dewey D. Phthalate exposure and childrens neurodevelopment, a systematic review. Environ Res. (2015) 142:51–60. doi: 10.1016/j.envres.2015.06.014
152. Engel SM, Miodovnik A, Canfield RL, Zhu C, Silva MJ, Calafat AM, et al. Prenatal phthalate exposure is associated with childhood behavior and executive functioning. Environ Health Perspect. (2010) 118:565–71. doi: 10.1289/ehp.0901470
153. Kim S, Eom S, Kim H-J, Lee JJ, Choi G, Choi S, et al. Association between maternal exposure to major phthalates, heavy metals, and persistent organic pollutants, and the neurodevelopmental performances of their children at 1 to 2 years of age-CHECK cohort study. Sci Total Environ. (2018) 624:377–84. doi: 10.1016/j.scitotenv.2017.12.058
154. Bouchard MF, Chevrier J, Harley KG, Kogut K, Vedar M, Calderon N, et al. Prenatal exposure to organophosphate pesticides and IQ in 7-year-old children. Environ Health Perspect. (2011) 119:1189–95. doi: 10.1289/ehp.1003185
155. Chen AM, Yolton K, Rauch SA, Webster GM, Hornung R, Sjodin A, et al. Prenatal polybrominated diphenyl ether exposures and neurodevelopment in US Children through. 5 years of age, the HOME study. Environ Health Perspect. (2014) 122:856–62. doi: 10.1289/ehp.1307562
156. Herbstman JB, Sjodin A, Kurzon M, Lederman SA, Jones RS, Rauh V, et al. Prenatal exposure to PBDEs and neurodevelopment. Environ Health Perspect. (2010) 118:712–9. doi: 10.1289/ehp.0901340
157. Jeddy Z, Hartman TJ, Taylor EV, Poteete C, Kordas K. Prenatal concentrations of perfluoroalkyl substances and early communication development in British girls. Early Hum Dev. (2017) 109:15–20. doi: 10.1016/j.earlhumdev.2017.04.004
158. Boucher O, Muckle G, Jacobson JL, Carter RC, Kaplan-Estrin M, Ayotte P, et al. Domain-specific effects of prenatal exposure to PCBs, mercury, and lead on infant cognition, results from the environmental contaminants and child development study in Nunavik. Environ Health Perspect. (2014) 122:310–6. doi: 10.1289/ehp.1206323
159. Fitzgerald EF, Belanger EE, Gomez MI, Cayo M, McCaffrey RJ, Seegal RF, et al. Polychlorinated biphenyl exposure and neuropsychological status among older residents of upper Hudson River communities. Environ Health Perspect. (2007) 116:209–15. doi: 10.1289/ehp.10432
160. Fitzgerald EF, Shrestha S, Gomez MI, McCaffrey RJ, Zimmerman EA, Kannan K, et al. Polybrominated diphenyl ethers (PBDEs), polychlorinated biphenyls (PCBs) and neuropsychological status among older adults in New York. Neurotoxicology. (2012) 33:8–15. doi: 10.1016/j.neuro.2011.10.011
161. Gore AC, Chappell V, Fenton S, Flaws JA, Nadal A, Prins GS, et al. EDC-2, the Endocrine Society's second scientific statement on endocrine-disrupting chemicals. Endocr Rev. (2015) 36:E1–50. doi: 10.1210/er.2015-1010
162. Mughal BB, Fini J-B, Demeneix BA. Thyroid-disrupting chemicals and brain development, an update. Endocr Connect. (2018) 7:R160–86. doi: 10.1530/EC-18-0029
163. Berbel P, Mestre JL, Santamaria A, Palazon I, Franco A, Graells M, et al. Delayed neurobehavioral development in children born to pregnant women with mild hypothyroxinemia during the first month of gestation, the importance of early iodine supplementation. Thyroid. (2009) 19:511–9. doi: 10.1089/thy.2008.0341
164. Haddow JE, Palomaki GE, Allan WC, Williams JR, Knight GJ, Gagnon J, et al. Maternal thyroid deficiency during pregnancy and subsequent neuropsychological development of the child. N Engl J Med. (1999) 341:549–55. doi: 10.1056/NEJM199908193410801
165. Pop VJ, Brouwers EP, Vader HL, Vulsma T, van Baar AL, de Vijlder JJ. Maternal hypothyroxinaemia during early pregnancy and subsequent child development, a 3-year follow-up study. Clin Endocrinol. (2003) 59:282–8. doi: 10.1046/j.1365-2265.2003.01822.x
166. Meeker JD, Ferguson KK. Relationship between urinary phthalate and bisphenol A concentrations and serum thyroid measures in US adults and adolescents from the National Health and Nutrition Examination Survey (NHANES) 2007–2008. Environ Health Perspect. (2011) 119:1396–402. doi: 10.1289/ehp.1103582
167. Romano ME, Eliot MN, Zoeller RT, Hoofnagle AN, Calafat AM, Karagas MR, et al. Maternal urinary phthalate metabolites during pregnancy and thyroid hormone concentrations in maternal and cord sera, the HOME study. Int J Hyg Environ Health. (2018) 221:623–31. doi: 10.1016/j.ijheh.2018.03.010
168. Sanlidag B, Dalkan C, Yetkin O, Bahceciler NN. Evaluation of dose dependent maternal exposure to bisphenol a on thyroid functions in newborns. J Clin Med. (2018) 7:119. doi: 10.3390/jcm7060119
169. Romano ME, Webster GM, Vuong AM, Thomas Zoeller R, Chen A, Hoofnagle AN, et al. Gestational urinary bisphenol A and maternal and newborn thyroid hormone concentrations, the HOME Study. Environ Res. (2015) 138:453–60. doi: 10.1016/j.envres.2015.03.003
170. Andrianou XD, Gangler S, Piciu A, Charisiadis P, Zira C, Aristidou K, et al. Human exposures to bisphenol A, bisphenol F and chlorinated bisphenol a derivatives and thyroid function. PLoS ONE. (2016) 11:e0155237. doi: 10.1371/journal.pone.0155237
171. Abdelouahab N, Langlois MF, Lavoie L, Corbin F, Pasquier JC, Takser L. Maternal and cord-blood thyroid hormone levels and exposure to polybrominated diphenyl ethers and polychlorinated biphenyls during early pregnancy. Am J Epidemiol. (2013) 178:701–13. doi: 10.1093/aje/kwt141
172. El Majidi Bouchard NM, Carrier G. Systematic analysis of the relationship between standardized biological levels of polychlorinated biphenyls and thyroid function in pregnant women and newborns. Chemosphere. (2014) 98:1–17. doi: 10.1016/j.chemosphere.2013.10.006
173. de Cock M, de Boer MR, Govarts E, Iszatt N, Palkovicova L, Lamoree MH, et al. Thyroid-stimulating hormone levels in newborns and early life exposure to endocrine-disrupting chemicals, analysis of three European mother-child cohorts. Pediatr Res. (2017) 82:429–37. doi: 10.1038/pr.2017.50
174. Vuong AM, Webster GM, Romano ME, Braun JM, Zoeller RT, Hoofnagle AN, et al. Maternal Polybrominated Diphenyl Ether (PBDE) exposure and thyroid hormones in maternal and cord sera, the HOME study, Cincinnati USA. Environ Health Perspect. (2015) 123:1079–85. doi: 10.1289/ehp.1408996
175. Stapleton HM, Eagle S, Anthopolos R, Wolkin A, Miranda ML. Associations between polybrominated diphenyl ether (PBDE) flame retardants, phenolic metabolites, and thyroid hormones during pregnancy. Environ Health Perspect. (2011) 119:1454–59. doi: 10.1289/ehp.1003235
176. Kim S, Park J, Kim H-J, Lee JJ, Choi G, Choi S, et al. Association between several persistent organic pollutants and thyroid hormone levels in serum among the pregnant women of Korea. Environ Int. (2013) 59:442–8. doi: 10.1016/j.envint.2013.07.009
177. Hill NR, Fatoba ST, Oke JL, Hirst JA, O'Callaghan CA, Lasserson DS, et al. Global prevalence of chronic kidney disease–a systematic review and meta-analysis. PloS ONE. (2016) 11:e0158765. doi: 10.1371/journal.pone.0158765
178. Kataria A, Trasande L, Trachtman H. The effects of environmental chemicals on renal function. Nat Rev Nephrol. (2015) 11:610–25. doi: 10.1038/nrneph.2015.94
179. Tsai HJ, Chen BH, Wu CF, Wang SL, Huang PC, Tsai YC, et al. Intake of phthalate-tainted foods and microalbuminuria in children, the 2011 Taiwan food scandal. Environ Int. (2016) 89–90:129–37. doi: 10.1016/j.envint.2016.01.015
180. Wu CF, Hsiung CA, Tsai HJ, Tsai YC, Hsieh HM, Chen BH, et al. Interaction of melamine and di-(2-ethylhexyl) phthalate exposure on markers of early renal damage in children, The 2011 Taiwan food scandal. Environ Pollut. (2018) 235:453–61. doi: 10.1016/j.envpol.2017.12.107
181. Malits J, Attina TM, Karthikraj R, Kannan K, Naidu M, Furth S, et al. Renal function and exposure to bisphenol A and phthalates in children with chronic kidney disease. Environ Res. (2018) 167:575–82. doi: 10.1016/j.envres.2018.08.006
182. Trasande L, Sathyanarayana S, Trachtman H. Dietary phthalates and low-grade albuminuria in US children and adolescents. Clin J Am Soc Nephrol. (2014) 9:100–9. doi: 10.2215/CJN.04570413
183. Trasande L, Attina TM, Trachtman H. Bisphenol A exposure is associated with low-grade urinary albumin excretion in children of the United States. Kidney Int. (2013) 83:741–48. doi: 10.1038/ki.2012.422
184. Li M, Bi Y, Qi L, Wang T, Xu M, Huang Y, et al. Exposure to bisphenol A is associated with low-grade albuminuria in Chinese adults. Kidney Int. (2012) 81:1131–9. doi: 10.1038/ki.2012.6
185. Kanno Y, Okada H, Kobayashi T, Takenaka T, Suzuki H. Effects of endocrine disrupting substance on estrogen receptor gene transcription in dialysis patients. Ther Apher Dial. (2007) 11:262–5. doi: 10.1111/j.1744-9987.2007.00472.x
186. You L, Zhu X, Shrubsole MJ, Fan H, Chen J, Dong J, et al. Renal function, bisphenol A, and alkylphenols, results from the National Health and Nutrition Examination Survey (NHANES 2003-2006). Environ Health Perspect. (2011) 119:527–33. doi: 10.1289/ehp.1002572
187. American Cancer Society. Cancer Treatment and Survivorship Facts and Figures 2014–2015. Atlanta, GA: American Cancer Society (2014).
188. Jobling S, Bjerregaard P, Blumberg B, Brandt I, Brian JV, Casey SC, et al. Evidence for endocrine disruption in humans and wildlife. In: State of the Science of Endocrine Disrupting Chemicals, United Nations Environment Programme and the World Health Organization. (2012). p. 23–188.
189. Bergman Å, Heindel J, Jobling S, Kidd K, Zoeller R. WHO (World Health Organization)/UNEP (United Nations Environment Programme) Global assessment of the state-of-the-science of endocrine disruptors (2014). doi: 10.1289/ehp.1306695
190. Key TJ. Hormones and cancer in humans. Mutat Res. (1995) 333:59–67. doi: 10.1016/0027-5107(95)00132-8
191. Lumachi F, Brunello A, Maruzzo M, Basso U, Basso SM. Treatment of estrogen receptor-positive breast cancer. Curr Med Chem. (2013) 20:596–604. doi: 10.2174/092986713804999303
192. Morgan M, Deoraj A, Felty Q, Roy D. Environmental estrogen-like endocrine disrupting chemicals and breast cancer. Mol Cell Endocrinol. (2017) 457:89–102. doi: 10.1016/j.mce.2016.10.003
193. Yager JD, Davidson NE. Estrogen carcinogenesis in breast cancer. N Engl J Med. (2006) 354:270–82. doi: 10.1056/NEJMra050776
194. Erkekoglu P, Kocer-Gumusel B. Genotoxicity of phthalates. Toxicol Mech Methods. (2014) 24:616–26. doi: 10.3109/15376516.2014.960987
195. Roy D, Palangat M, Chen C-W, Thomas RD, Colerangle J, Atkinson A, et al. Biochemical and molecular changes at the cellular level in response to exposure to environmental estrogen-like chemicals. J Toxicol Environ Health A. (1997) 50:1–30. doi: 10.1080/009841097160573
196. Ingber SZ, Buser MC, Pohl HR, Abadin HG, Murray HE, Scinicariello F. DDT/DDE and breast cancer, a meta-analysis. Regul Toxicol Pharmacol. (2013) 67:421–33. doi: 10.1016/j.yrtph.2013.08.021
197. Cohn BA, Wolff MS, Cirillo PM, Sholtz RI. DDT and breast cancer in young women, new data on the significance of age at exposure. Environ Health Perspect. (2007) 115:1406–14. doi: 10.1289/ehp.10260
198. Koutros S. 389 Risk of total and aggressive prostate cancer and pesticide use in the agricultural health study. Occup Environ Med. (2013) 70(Suppl. 1):A133. doi: 10.1136/oemed-2013-101717.389
199. Christensen CH, Platz EA, Andreotti G, Blair A, Hoppin JA, Koutros S, et al. Coumaphos exposure and incident cancer among male participants in the Agricultural Health Study (AHS). Environ Health Perspect. (2009) 118:92–6. doi: 10.1289/ehp.0800446
201. Mascie-Taylor CN. Nutritional status, its measurement and relation to health. Appl Biol Anthropol Hum Affairs. (1991) 55–83. doi: 10.1017/CBO9780511629129.003
202. Kok HS, van Asselt KM, van der Schouw YT, Peeters PH, Wijmenga C. Genetic studies to identify genes underlying menopausal age. Hum Reprod Update. (2005) 11:483–93. doi: 10.1093/humupd/dmi024
203. Barker DJ The developmental origins of adult disease. Eur J Epidemiol. (2003) 18:733–6. doi: 10.1023/A:1025388901248
204. Armenti AE, Zama AM, Passantino L, Uzumcu M. Developmental methoxychlor exposure affects multiple reproductive parameters and ovarian folliculogenesis and gene expression in adult rats. Toxicol Appl Pharmacol. (2008) 233:286–96. doi: 10.1016/j.taap.2008.09.010
205. Adewale HB, Jefferson WN, Newbold RR, Patisaul HB. Neonatal bisphenol-a exposure alters rat reproductive development and ovarian morphology without impairing activation of gonadotropin-releasing hormone neurons. Biol Reprod. (2009) 81:690–9. doi: 10.1095/biolreprod.109.078261
206. Shi Z, Valdez KE, Ting AY, Franczak A, Gum SL, Petroff BK. Ovarian endocrine disruption underlies premature reproductive senescence following environmentally relevant chronic exposure to the aryl hydrocarbon receptor agonist 2,3,7,8-tetrachlorodibenzo-p-dioxin. Biol Reprod. (2007) 76:198–202. doi: 10.1095/biolreprod.106.053991
207. Hatch EE, Troisi R, Wise LA, Hyer M, Palmer JR, Titus-Ernstoff L, et al. Age at natural menopause in women exposed to diethylstilbestrol in utero. Am J Epidemiol. (2006) 164:682–8. doi: 10.1093/aje/kwj257
208. Knox SS, Jackson T, Javins B, Frisbee SJ, Shankar A, Ducatman AM. Implications of early menopause in women exposed to perfluorocarbons. J Clin Endocrinol Metab. (2011) 96:1747–53. doi: 10.1210/jc.2010-2401
209. Yang YJ, Hong Y-C, Oh S-Y, Park M-S, Kim H, Leem J-H, et al. Bisphenol A exposure is associated with oxidative stress and inflammation in postmenopausal women. Environ Res. (2009) 109:797–801. doi: 10.1016/j.envres.2009.04.014
210. Li Y, Luh CJ, Burns KA, Arao Y, Jiang Z, Teng CT, et al. Endocrine-disrupting chemicals (EDCs), in vitro mechanism of estrogenic activation and differential effects on ER target genes. Environ Health Perspect. (2013) 121:459–66. doi: 10.1289/ehp.1205951
211. Street ME, Angelini S, Bernasconi S, Burgio E, Cassio A, Catellani C, et al. Current knowledge on Endocrine Disrupting Chemicals (EDCs) from animal biology to humans, from pregnancy to adulthood, highlights from a national Italian meeting. Int J Mol Sci. (2018) 19:1647. doi: 10.3390/ijms19061647
212. Lauritzen HB, Larose TL, Oien T, Sandanger TM, Odland JO, van de Bor M, et al. Maternal serum levels of perfluoroalkyl substances and organochlorines and indices of fetal growth, a scandinavian case-cohort study. Pediatr Res. (2017) 81:33–42. doi: 10.1038/pr.2016.187
213. Kohlerova E, Skarda J. Mouse bioassay to assess oestrogenic and anti-oestrogenic compounds, hydroxytamoxifen, diethylstilbestrol and genistein. J Vet Med A Physiol Pathol Clin Med. (2004) 51:209–17. doi: 10.1111/j.1439-0442.2004.00634.x
214. Alonso-Magdalena P, Vieira E, Soriano S, Menes L, Burks D, Quesada I, et al. Bisphenol A exposure during pregnancy disrupts glucose homeostasis in mothers and adult male offspring. Environ Health Perspect. (2010) 118:1243–50. doi: 10.1289/ehp.1001993
215. Wei J, Lin Y, Li Y, Ying C, Chen J, Song L, et al. Perinatal exposure to bisphenol A at reference dose predisposes offspring to metabolic syndrome in adult rats on a high-fat diet. Endocrinology. (2011) 152:3049–61. doi: 10.1210/en.2011-0045
216. Angle BM, Do RP, Ponzi D, Stahlhut RW, Drury BE, Nagel SC, et al. Metabolic disruption in male mice due to fetal exposure to low but not high doses of bisphenol A (BPA), evidence for effects on body weight, food intake, adipocytes, leptin, adiponectin, insulin and glucose regulation. Reprod Toxicol. (2013) 42:256–68. doi: 10.1016/j.reprotox.2013.07.017
217. Hines EP, White SS, Stanko JP, Gibbs-Flournoy EA, Lau C, Fenton SE. Phenotypic dichotomy following developmental exposure to perfluorooctanoic acid (PFOA) in female CD-1 mice, Low doses induce elevated serum leptin and insulin, and overweight in mid-life. Mol Cell Endocrinol. (2009) 304:97–105. doi: 10.1016/j.mce.2009.02.021
218. Hao C, Cheng X, Xia H, Ma X. The endocrine disruptor mono-(2-ethylhexyl) phthalate promotes adipocyte differentiation and induces obesity in mice. Biosci Rep. (2012) 32:619–29. doi: 10.1042/BSR20120042
219. Vandenberg LN, Colborn T, Hayes TB, Heindel JJ, Jacobs DR Jr., Lee DH, et al. Hormones and endocrine-disrupting chemicals, low-dose effects and nonmonotonic dose responses. Endocr Rev. (2012) 33:378–455. doi: 10.1210/er.2011-1050
220. Cruz G, Foster W, Paredes A, Yi KD, Uzumcu M. Long-term effects of early-life exposure to environmental oestrogens on ovarian function, role of epigenetics. J Neuroendocrinol. (2014) 26:613–24. doi: 10.1111/jne.12181
221. Christensen BC, Marsit CJ. Epigenomics in environmental health. Front Genet. (2011) 2:84. doi: 10.3389/fgene.2011.00084
222. Rissman EF, Adli M. Minireview, transgenerational epigenetic inheritance, focus on endocrine disrupting compounds. Endocrinology. (2014) 155:2770–80. doi: 10.1210/en.2014-1123
223. Jirtle RL, Skinner MK. Environmental epigenomics and disease susceptibility. Nat Rev Genet. (2007) 8:253–62. doi: 10.1038/nrg2045
224. Skinner MK, Manikkam M, Guerrero-Bosagna C. Epigenetic transgenerational actions of environmental factors in disease etiology. Trends Endocrinol Metab. (2010) 21:214–22. doi: 10.1016/j.tem.2009.12.007
225. Anway MD, Cupp AS, Uzumcu M, Skinner MK. Epigenetic transgenerational actions of endocrine disruptors and male fertility. Science. (2005) 308:1466–9. doi: 10.1126/science.1108190
226. Santangeli S, Maradonna F, Olivotto I, Piccinetti CC, Gioacchini G, Carnevali O. Effects of BPA on female reproductive function, the involvement of epigenetic mechanism. Gen Comp Endocrinol. (2017) 245:122–26. doi: 10.1016/j.ygcen.2016.08.010
227. Eichenlaub-Ritter U, Pacchierotti F. Bisphenol A effects on mammalian oogenesis and epigenetic integrity of oocytes, a case study exploring risks of endocrine disrupting chemicals. Biomed Res Int. (2015) 2015:698795. doi: 10.1155/2015/698795
228. Salian S, Doshi T, Vanage G. Perinatal exposure of rats to Bisphenol A affects fertility of male offspring–an overview. Reprod Toxicol. (2011) 31:359–62. doi: 10.1016/j.reprotox.2010.10.008
229. Manikkam M, Tracey R, Guerrero-Bosagna C, Skinner MK. Dioxin (TCDD) induces epigenetic transgenerational inheritance of adult onset disease and sperm epimutations. PLoS ONE. (2012) 7:e46249. doi: 10.1371/journal.pone.0046249
230. Baker TR, King-Heiden TC, Peterson RE, Heideman W. Dioxin induction of transgenerational inheritance of disease in zebrafish. Mol Cell Endocrinol. (2014) 398:36–41. doi: 10.1016/j.mce.2014.08.011
231. Messerschmidt DM, Knowles BB, Solter D. DNA methylation dynamics during epigenetic reprogramming in the germline and preimplantation embryos. Genes Dev. (2014) 28:812–28. doi: 10.1101/gad.234294.113
232. Skinner MK, Manikkam M, Tracey R, Guerrero-Bosagna C, Haque M, Nilsson EE. Ancestral dichlorodiphenyltrichloroethane (DDT) exposure promotes epigenetic transgenerational inheritance of obesity. BMC Med. (2013) 11:228. doi: 10.1186/1741-7015-11-228
233. Doyle TJ, Bowman JL, Windell VL, McLean DJ, Kim KH. Transgenerational effects of di-(2-ethylhexyl) phthalate on testicular germ cell associations and spermatogonial stem cells in mice. Biol Reprod. (2013) 88:112. doi: 10.1095/biolreprod.112.106104
234. Chen J, Wu S, Wen S, Shen L, Peng J, Yan C, et al. Correction, the mechanism of Environmental Endocrine Disruptors (DEHP) induces epigenetic transgenerational inheritance of cryptorchidism. PLoS ONE. (2015) 10:e0132749. doi: 10.1371/journal.pone.0132749
235. Stel J, Legler J. The role of epigenetics in the latent effects of early life exposure to obesogenic endocrine disrupting chemicals. Endocrinology. (2015) 156:3466–72. doi: 10.1210/en.2015-1434
236. Palanza P, Nagel SC, Parmigiani S, Vom Saal SF. Perinatal exposure to endocrine disruptors, sex, timing and behavioral endpoints. Curr Opin Behav Sci. (2016) 7:69–75. doi: 10.1016/j.cobeha.2015.11.017
237. Kortenkamp A. Ten years of mixing cocktails, a review of combination effects of endocrine-disrupting chemicals. Environ Health Perspect. (2007) 115(Suppl. 1):98–105. doi: 10.1289/ehp.9357
238. Marty MS, Carney EW, Rowlands JC. Endocrine disruption, historical perspectives and its impact on the future of toxicology testing. Toxicol Sci. (2011) 120(Suppl. 1):S93–108. doi: 10.1093/toxsci/kfq329
239. Kortenkamp A. Low dose mixture effects of endocrine disrupters and their implications for regulatory thresholds in chemical risk assessment. Curr Opin Pharmacol. (2014) 19:105–11. doi: 10.1016/j.coph.2014.08.006
240. Bonde JP, Flachs EM, Rimborg S, Glazer CH, Giwercman A, Ramlau-Hansen CH, et al. The epidemiologic evidence linking prenatal and postnatal exposure to endocrine disrupting chemicals with male reproductive disorders, a systematic review and meta-analysis. Hum Reprod Update. (2016) 23:104–25. doi: 10.1093/humupd/dmw036
241. Boada LD, Zumbado M, Henríquez-Hernández LA, Almeida-González M, Álvarez-León EE, Serra-Majem L, et al. Complex organochlorine pesticide mixtures as determinant factor for breast cancer risk, a population-based case–control study in the Canary Islands (Spain). Environmental Health. (2012) 11:28. doi: 10.1186/1476-069X-11-28
242. Wolf K, Bongaerts BWC, Schneider A, Huth C, Meisinger C, Peters A, et al. Persistent organic pollutants and the incidence of type 2 diabetes in the CARLA and KORA cohort studies. Environ Int. (2019) 129:221–8. doi: 10.1016/j.envint.2019.05.030
243. Soundararajan A, Prabu P, Mohan V, Gibert Y, Balasubramanyam M. Novel insights of elevated systemic levels of bisphenol-A (BPA) linked to poor glycemic control, accelerated cellular senescence and insulin resistance in patients with type 2 diabetes. Mol Cell Biochem. (2019) 458:171–83. doi: 10.1007/s11010-019-03540-9
244. Nakamoto M, Arisawa K, Uemura H, Katsuura S, Takami H, Sawachika F, et al. Association between blood levels of PCDDs/PCDFs/dioxin-like PCBs and history of allergic and other diseases in the Japanese population. Int Arch Occup Environ Health. (2013) 86:849–59. doi: 10.1007/s00420-012-0819-8
245. Uemura H, Arisawa K, Hiyoshi M, Kitayama A, Takami H, Sawachika F, et al. Prevalence of metabolic syndrome associated with body burden levels of dioxin and related compounds among Japan's general population. Environ Health Perspect. (2009) 117:568–73. doi: 10.1289/ehp.0800012
246. Ha M, Lee D, Son H, Park S, Jacobs D. Association between serum concentrations of persistent organic pollutants and prevalence of newly diagnosed hypertension, results from the National Health and Nutrition Examination Survey 1999–2002. J Hum Hypert. (2009) 23:274–86. doi: 10.1038/jhh.2008.124
247. Lee D-H, Lee I-K, Porta M, Steffes M, Jacobs D. Relationship between serum concentrations of persistent organic pollutants and the prevalence of metabolic syndrome among non-diabetic adults, results from the National Health and Nutrition Examination Survey 1999–2002. Diabetologia. (2007) 50:1841–51. doi: 10.1007/s00125-007-0755-4
248. Valera B, Ayotte P, Poirier P, Dewailly É. Associations between plasma persistent organic pollutant levels and blood pressure in Inuit adults from Nunavik. Environ Int. (2013) 59:282–9. doi: 10.1016/j.envint.2013.06.019
249. Lind PM, Penell J., Salihovic S, van Bavel B, Lind L. Circulating levels of p,p'-DDE are related to prevalent hypertension in the elderly. Environ Res. (2014) 129:27–31. doi: 10.1016/j.envres.2013.12.003
250. Ahmadkhaniha R, Mansouri M, Yunesian M, Omidfar K, Jeddi MZ, Larijani B, et al. Association of urinary bisphenol a concentration with type-2 diabetes mellitus. J Environ Health Sci Eng. (2014) 12:64. doi: 10.1186/2052-336X-12-64
251. Chang JW, Ou HY, Chen HL, Su HJ, Lee CC. Hyperuricemia after exposure to polychlorinated dibenzo-p-dioxins and dibenzofurans near a highly contaminated area. Epidemiology. (2013) 24:582–9. doi: 10.1097/EDE.0b013e318294ef68
252. Lee Y-M, Bae S-G, Lee S-H, Jacobs DR Jr., Lee D-H. Persistent organic pollutants and hyperuricemia in the US general population. Atherosclerosis. (2013) 230:1–5. doi: 10.1016/j.atherosclerosis.2013.06.012
253. Geiger SD, Xiao J, Shankar A. Positive association between perfluoroalkyl chemicals and hyperuricemia in children. Am J Epidemiol. (2013) 177:1255–62. doi: 10.1093/aje/kws392
254. Imamura T, Matsumoto S, Akahane M, Kanagawa Y, Koike S, Tajima B, et al. Cutaneous symptoms such as acneform eruption and pigmentation are closely associated with blood levels of 2, 3, 4, 7, 8-penta-chlorodibenzofurans in Yusho patients, using data mining analysis. BMC Res Notes. (2009) 2:27. doi: 10.1186/1756-0500-2-27
255. Martinez-Zamora MA, Mattioli L, Parera J, Abad E, Coloma JL, van Babel B, et al. Increased levels of dioxin-like substances in adipose tissue in patients with deep infiltrating endometriosis. Hum Reprod. (2015) 30:1059–68. doi: 10.1093/humrep/dev026
256. Li D, Zhou Z, Qing D, He Y, Wu T, Miao M, et al. Occupational exposure to bisphenol-A (BPA) and the risk of self-reported male sexual dysfunction. Hum Reprod. (2010) 25:519–27. doi: 10.1093/humrep/dep381
257. Lopez-Carrillo L, Hernandez-Ramirez RU, Calafat AM, Torres-Sanchez L, Galvan-Portillo M, Needham LL, et al. Exposure to phthalates and breast cancer risk in northern Mexico. Environ Health Perspect. (2010) 118:539–44. doi: 10.1289/ehp.0901091
258. Chuang SC, Chen HC, Sun CW, Chen YA, Wang YH, Chiang CJ, et al. Phthalate exposure and prostate cancer in a population-based nested case-control study. Environ Res. (2020) 181:108902. doi: 10.1016/j.envres.2019.108902
259. Spanogiannopoulos P, Bess EN, Carmody RN, Turnbaugh PJ. The microbial pharmacists within us, a metagenomic view of xenobiotic metabolism. Nat Rev Microbiol. (2016) 14:273–87. doi: 10.1038/nrmicro.2016.17
260. Snedeker SM, Hay AG. Do interactions between gut ecology and environmental chemicals contribute to obesity and diabetes? Environ Health Perspect. (2011) 120:332–9. doi: 10.1289/ehp.1104204
261. Patterson AD, Turnbaugh PJ. Microbial determinants of biochemical individuality and their impact on toxicology and pharmacology. Cell Metab. (2014) 20:761–8. doi: 10.1016/j.cmet.2014.07.002
262. Carmody RN, Turnbaugh PJ. Host-microbial interactions in the metabolism of therapeutic and diet-derived xenobiotics. J Clin Invest. (2014) 124:4173–81. doi: 10.1172/JCI72335
263. Claus SP, Guillou H, Ellero-Simatos S. The gut microbiota, a major player in the toxicity of environmental pollutants? NPJ Biofilms Microb. (2016) 2:16003. doi: 10.1038/npjbiofilms.2016.3
264. Donovan SM. Introduction to the special focus issue on the impact of diet on gut microbiota composition and function and future opportunities for nutritional modulation of the gut microbiome to improve human health. Gut Microbes. (2017) 8:75–81. doi: 10.1080/19490976.2017.1299309
265. Clarke G, Stilling RM, Kennedy PJ, Stanton C, Cryan JF, Dinan TG. Minireview gut microbiota, the neglected endocrine organ. Mol Endocrinol. (2014) 28:1221–38. doi: 10.1210/me.2014-1108
266. De Vadder F, Kovatcheva-Datchary P, Goncalves D, Vinera J, Zitoun C, Duchampt A, et al. Microbiota-generated metabolites promote metabolic benefits via gut-brain neural circuits. Cell. (2014) 156:84–96. doi: 10.1016/j.cell.2013.12.016
267. Egshatyan L, Kashtanova D, Popenko A, Tkacheva O, Tyakht A, Alexeev D, et al. Gut microbiota and diet in patients with different glucose tolerance. Endocr Connect. (2016) 5:1–9. doi: 10.1530/EC-15-0094
268. Qin J, Li Y, Cai Z, Li S, Zhu J, Zhang F, et al. A metagenome-wide association study of gut microbiota in type 2 diabetes. Nature. (2012) 490:55–60. doi: 10.1038/nature11450
269. Munyaka PM, Khafipour E, Ghia JE. External influence of early childhood establishment of gut microbiota and subsequent health implications. Front Pediatr. (2014) 2:109. doi: 10.3389/fped.2014.00109
270. Murphy EA, Velazquez KT, Herbert KM. Influence of high-fat diet on gut microbiota, a driving force for chronic disease risk. Curr Opin Clin Nutr Metab Care. (2015) 18:515–20. doi: 10.1097/MCO.0000000000000209
271. Xu Z, Knight R. Dietary effects on human gut microbiome diversity. Br J Nutr. (2015) 113:S1–5. doi: 10.1017/S0007114514004127
272. Zhang M, Yang XJ. Effects of a high fat diet on intestinal microbiota and gastrointestinal diseases. World J Gastroenterol. (2016) 22:8905–09. doi: 10.3748/wjg.v22.i40.8905
273. Singh RK, Chang HW, Yan D, Lee KM, Ucmak D, Wong K, et al. Influence of diet on the gut microbiome and implications for human health. J Transl Med. (2017) 15:73. doi: 10.1186/s12967-017-1175-y
274. Yuswir NS, Praveena SM, Aris AZ, Hashim Z. Bioavailability of heavy metals using in vitro digestion model, a state of present knowledge. Rev Environ Health. (2013) 28:181–7. doi: 10.1515/reveh-2013-0012
275. Jurewicz J, Polanska K, Hanke W. Chemical exposure early in life and the neurodevelopment of children–an overview of current epidemiological evidence. Ann Agric Environ Med. (2013) 20:456–86.
276. Grand View Research. Global Bisphenol A (BPA) Market by Application (Appliances, Automotive, Consumer, Construction, Electrical and Electronics) Expected to Reach USD 20.03 Billion by 2020 (2014).
277. Caravanos J, Dowling R, Tellez-Rojo MM, Cantoral A, Kobrosly R, Estrada D, et al. Blood lead levels in Mexico and pediatric burden of disease implications. Ann Glob Health. (2014) 80:269–77. doi: 10.1016/j.aogh.2014.08.002
278. Hadrup N, Lam HR. Oral toxicity of silver ions, silver nanoparticles and colloidal silver–a review. Regul Toxicol Pharmacol. (2014) 68:1–7. doi: 10.1016/j.yrtph.2013.11.002
279. Theodorou IG, Ryan MP, Tetley TD, Porter AE. Inhalation of silver nanomaterials–seeing the risks. Int J Mol Sci. (2014) 15:23936–74. doi: 10.3390/ijms151223936
280. Zeng X, Xu X, Boezen HM, Huo X. Children with health impairments by heavy metals in an e-waste recycling area. Chemosphere. (2016) 148:408–15. doi: 10.1016/j.chemosphere.2015.10.078
281. Chowdhury S, Mazumder MAJ, Al-Attas O, Husain T. Heavy metals in drinking water, occurrences, implications, and future needs in developing countries. Sci Total Environ. (2016) 569-570:476–88. doi: 10.1016/j.scitotenv.2016.06.166
282. Rhee SH, Pothoulakis C, Mayer EA. Principles and clinical implications of the brain–gut–enteric microbiota axis. Nat Rev Gastroenterol Hepatol. (2009) 6:306. doi: 10.1038/nrgastro.2009.35
283. Collins SM, Bercik P. The relationship between intestinal microbiota and the central nervous system in normal gastrointestinal function and disease. Gastroenterology. (2009) 136:2003–14. doi: 10.1053/j.gastro.2009.01.075
284. Cryan JF, Dinan TG. Mind-altering microorganisms, the impact of the gut microbiota on brain and behaviour. Nat Rev Neurosci. (2012) 13:701. doi: 10.1038/nrn3346
285. Cenit MC, Nuevo IC, Codoner-Franch P, Dinan TG, Sanz Y. Gut microbiota and attention deficit hyperactivity disorder, new perspectives for a challenging condition. Eur Child Adolesc Psychiatry. (2017) 26:1081–1092. doi: 10.1007/s00787-017-0969-z
286. Quigley EM. Gut microbiome as a clinical tool in gastrointestinal disease management, are we there yet? Nat Rev Gastroenterol Hepatol. (2017) 14:315. doi: 10.1038/nrgastro.2017.29
287. Rieder R, Wisniewski PJ, Alderman BL, Campbell SC. Microbes and mental health, a review. Brain Behav Immun. (2017) 66:9–17. doi: 10.1016/j.bbi.2017.01.016
288. Shukla SD, Budden KF, Neal R, Hansbro PM. Microbiome effects on immunity, health and disease in the lung. Clin Transl Immunol. (2017) 6:e133. doi: 10.1038/cti.2017.6
289. Smolinska S, Groeger D, O'Mahony L. Biology of the microbiome 1, interactions with the host immune response. Gastroenterol Clin North Am. (2017) 46:19–35. doi: 10.1016/j.gtc.2016.09.004
290. Tang WH, Kitai T, Hazen SL. Gut microbiota in cardiovascular health and disease. Circ Res. (2017) 120:1183–96. doi: 10.1161/CIRCRESAHA.117.309715
291. van de Wouw M, Schellekens H, Dinan TG, Cryan JF. Microbiota-gut-brain axis, modulator of host metabolism and appetite. J Nutr. (2017) 147:727–45. doi: 10.3945/jn.116.240481
293. Miller GW, Jones DP. The nature of nurture, refining the definition of the exposome. Toxicol Sci. (2014) 137:1–2. doi: 10.1093/toxsci/kft251
294. Feil R, Fraga MF. Epigenetics and the environment, emerging patterns and implications. Nat Rev Genet. (2012) 13:97–109. doi: 10.1038/nrg3142
295. Messerlian C, Martinez RM, Hauser R, Baccarelli AA. 'Omics' and endocrine-disrupting chemicals—new paths forward. Nat Rev Endocrinol. (2017) 13:740. doi: 10.1038/nrendo.2017.81
296. Vrijheid M, Slama R, Robinson O, Chatzi L, Coen M, van den Hazel P, et al. The human early-life exposome (HELIX), project rationale and design. Environ Health Perspect. (2014) 122:535–44. doi: 10.1289/ehp.1307204
297. Brown AR, Owen SF, Peters J, Zhang Y, Soffker M, Paull GC, et al. Climate change and pollution speed declines in zebrafish populations. Proc Natl Acad Sci USA. (2015) 112:E1237–46. doi: 10.1073/pnas.1416269112
298. Yoshida H, Takahashi K, Takeda N, Sakai S. Japan's waste management policies for dioxins and polychlorinated biphenyls. J Mater Cycles Waste Manag. (2009) 11:229–43. doi: 10.1007/s10163-008-0235-z
299. Ma J, Hung H, Blanchard P. How do climate fluctuations affect persistent organic pollutant distribution in North America? Evidence from a decade of air monitoring. Environ Sci Technol. (2004) 38:2538–43. doi: 10.1021/es0349610
300. Olsson A, Ceder K, Bergman Å, Helander B. Nestling blood of the white-tailed sea eagle (Haliaeetus albicilla) as an indicator of territorial exposure to organohalogen compounds– an evaluation. Environ Sci Technol. (2000) 34:2733–40. doi: 10.1021/es991426k
301. Christensen JR, MacDuffee M, Macdonald RW, Whiticar M, Ross PS. Persistent organic pollutants in British Columbia grizzly bears, consequence of divergent diets. Environ Sci Technol. (2005) 39:6952–60. doi: 10.1021/es050749f
302. Kucklick J, Schwacke L, Wells R, Hohn A, Guichard A, Yordy J, et al. Bottlenose dolphins as indicators of persistent organic pollutants in the western North Atlantic Ocean and Northern Gulf of Mexico. Environ Sci Technol. (2011) 45:4270–77. doi: 10.1021/es1042244
303. Arnot JA, Armitage JM, McCarty LS, Wania F, Cousins IT, Toose-Reid L. Toward a consistent evaluative framework for POP risk characterization. Environ Sci Technol. (2011) 45:97–103. doi: 10.1021/es102551d
304. Nizzetto L, Macleod M, Borga K, Cabrerizo A, Dachs J, Di Guardo A, et al. Past, present, and future controls on levels of persistent organic pollutants in the global environment. Environ Sci Technol. (2010) 44:6526–31. doi: 10.1021/es100178f
305. Cousins I, Kong D, Vestergren B. Climate Change and POPs, Predicting the Impacts. Impact of Climate Change on Exposure to POPs for Wildlife and Humans. Report of the UNEP/AMAP Expert Group (2010). p. 21–8.
308. Andersson N, Arena M, Auteri D, Barmaz S, Grignard E, Kienzler A, et al. Guidance for the identification of endocrine disruptors in the context of Regulations (EU) No. 528/2012 and (EC) No 1107/2009. EFSA J. (2018) 16:e05311. doi: 10.2903/j.efsa.2018.5311
309. Rocha BA, Azevedo LF, Gallimberti M, Campiglia AD, Barbosa F Jr. High levels of bisphenol A and bisphenol s in brazilian thermal paper receipts and estimation of daily exposure. J Toxicol Environ Health A. (2015) 78:1181–8. doi: 10.1080/15287394.2015.1083519
311. The European Parliament and of the Council. Registration, Evaluation, Authorisation and Restriction of Chemicals (2006).
312. The European Parliament and of the Council. Placing of Plant Protection Products on the Market and Repealing Council Directives. The European Parliament and of the Council (2009).
313. The European Parliament and of the Council. Making Available on the Market and Use of Biocidal Products (2012).
314. The European Parliament and of the Council. European Parliament and of the Council of 23 October 2000 Establishing a Framework for Community Action in the Field of Water Policy (2000).
316. USEP Agency. A Summary of EPA's Bisphenol A (BPA) Action Plan. United States Environmental Protection Agency (2010).
317. USEP Agency. How EPA Regulates Drinking Water Contaminants. United States Environmental Protection Agency (2016).
320. Government of Canada. Approach for Identification of Chemicals and Polymers as Risk Assessment Priorities Under Part 5 of the Canadian Environmental Protection Act, 1999 (2017).
323. Presidency of the Republic Sub-Sofia. DECREE No. 4,074. Presidency of the Republic Sub-Sofia (2002).
324. Pelaez V, da Silva LR, Araújo EB. Regulation of pesticides, a comparative analysis. Sci Public Policy. (2013) 40:644–56. doi: 10.1093/scipol/sct020
325. The State Council. Circular of the State Council on Printing and Distributing the “Thirteenth Five-Year” Ecological Environmental Protection Plan. Chinese Government Website (2016).
326. Ministry of the Environment, Japan (MOE). Further Actions to Endocrine Disrupting Effects of Chemical Substances (2010).
Keywords: endocrine-disrupting chemicals, exposome, hormone receptor, trans-generational effects, pollutant
Citation: Kumar M, Sarma DK, Shubham S, Kumawat M, Verma V, Prakash A and Tiwari R (2020) Environmental Endocrine-Disrupting Chemical Exposure: Role in Non-Communicable Diseases. Front. Public Health 8:553850. doi: 10.3389/fpubh.2020.553850
Received: 20 April 2020; Accepted: 18 August 2020;
Published: 24 September 2020.
Edited by:
Mohiuddin Md. Taimur Khan, Washington State University Tri-Cities, United StatesReviewed by:
Tanvir Ahmed, Bangladesh University of Engineering and Technology, BangladeshYue-Wern Huang, Missouri University of Science and Technology, United States
Martine Marie Bellanger, École des Hautes Etudes en Santé Publique, France
Copyright © 2020 Kumar, Sarma, Shubham, Kumawat, Verma, Prakash and Tiwari. This is an open-access article distributed under the terms of the Creative Commons Attribution License (CC BY). The use, distribution or reproduction in other forums is permitted, provided the original author(s) and the copyright owner(s) are credited and that the original publication in this journal is cited, in accordance with accepted academic practice. No use, distribution or reproduction is permitted which does not comply with these terms.
*Correspondence: Manoj Kumar, bWFub2oxNW5kcmkmI3gwMDA0MDtnbWFpbC5jb20=