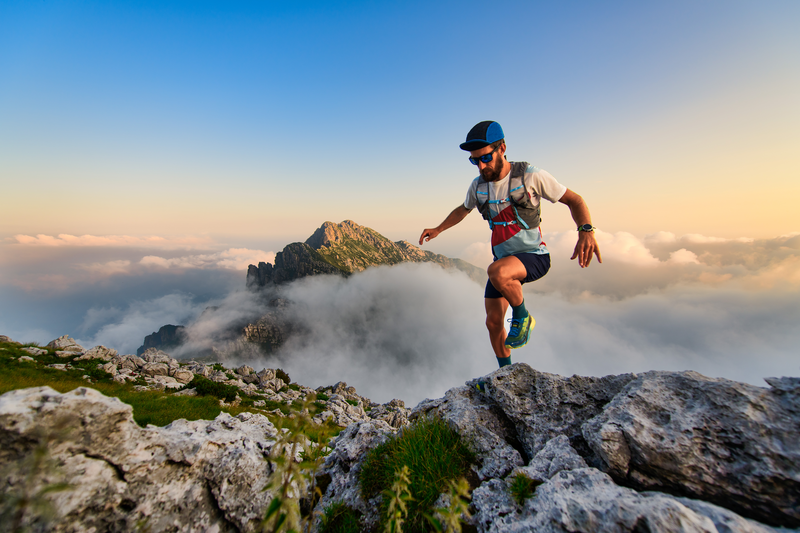
94% of researchers rate our articles as excellent or good
Learn more about the work of our research integrity team to safeguard the quality of each article we publish.
Find out more
REVIEW article
Front. Public Health , 08 October 2020
Sec. Environmental Health and Exposome
Volume 8 - 2020 | https://doi.org/10.3389/fpubh.2020.00464
This article is part of the Research Topic Biomarkers of Exposure, Effect and Susceptibility to Environmental and Occupational Chemicals View all 18 articles
Arsenic contamination in drinking water and associated adverse outcomes are one of the major health issues in more than 50 countries worldwide. The scenario is getting even more detrimental with increasing number of affected people and newer sites reported from all over the world. Apart from drinking water, the presence of arsenic has been found in various other dietary sources. Chronic arsenic toxicity affects multiple physiological systems and may cause malignancies leading to death. Exposed individuals, residing in the same area, developed differential dermatological lesion phenotypes and varied susceptibility toward various other arsenic-induced disease risk, even after consuming equivalent amount of arsenic from the similar source, over the same duration of time. Researches so far indicate that differential susceptibility plays an important role in arsenic-induced disease manifestation. In this comprehensive review, we have identified major population-based studies of the last 20 years, indicating possible causes of differential susceptibility emphasizing arsenic methylation capacity, variation in host genome (single nucleotide polymorphism), and individual epigenetic pattern (DNA methylation, histone modification, and miRNA expression). Holistic multidisciplinary strategies need to be implemented with few sustainable yet cost-effective solutions like alternative water source, treatment of arsenic-contaminated water, new adaptations in irrigation system, simple modifications in cooking strategy, and dietary supplementations to combat this menace. Our review focuses on the present perspectives of arsenic research with special emphasis on the probable causes of differential susceptibility toward chronic arsenic toxicity and sustainable remediation strategies.
Arsenic exposure is one of the major threats to public health in more than 50 nations including China, Australia, India, Bangladesh, Argentina, Brazil, Thailand, Vietnam, Pakistan, Chile, Bulgaria, Canada, Czech Republic, Egypt, Iran, parts of USA, etc. (1). The worldwide scenario of arsenic contamination has been changing with the discovery of newer sites and increasing number of affected people. The latest global count of arsenic-affected individuals, exposed above the WHO safety standard for drinking water of 10 μg/L (1), is ~140 million, which has increased substantially over the decade (2). Since World War II, the initial reports of arsenic toxicity came up-front (3, 4) and have been a prime focus of environmental health research spanning various fields of research including geologists, chemists, pharmacologists, and more so biologists. Arsenic is a metalloid, its inorganic form (e.g., arsenic trioxide, sodium arsenite, and arsenic trichloride are trivalent forms, and lead arsenate and calcium arsenate are pentavalent forms) being found within the natural elements, while organic form circulates within the ecosystem. Common forms of organic arsenic compounds are methylarsonic acid, dimethylarsinic acid, arsanilic acid, etc., formed during metabolism inside living organisms in most of the cases (5). Inorganic arsenic is predominantly found in drinking water and dietary sources like dairy products, meats, cereals, etc. On the other hand, organic form like arsenobetaine is mostly present in seafood, fruits, and vegetables. Arsenic contamination in the groundwater of Indo-Gangetic region occurs from rapid weathering of arsenic-bearing rock in the upper Himalayan catchments, and various river systems get buried in young, low-lying alluvial floodplains of various riverine deltas. The elevated concentration depends on biogeochemical and hydrogeochemical process along with higher sedimentation rate. The slow aquifer-flushing rate is the primary reason for the higher sedimentation in these regions. A potential source of arsenic in the ecosystem is attributed to anthropogenic activities like mining, smelting, and industrial processes, use of arsenic-laden pesticides, etc. (2). Arsenic has three ionized states: arsines As(III−), arsenite As(III+), and arsenate As(V+), the latter two being most mobile in both organic and inorganic forms (2, 5). Both acute and chronic arsenic toxicities generate various deleterious effects in multiple organs and tissues, like hyperkeratosis and change in skin pigmentation, cardiovascular diseases, pulmonary disease, peripheral neuropathy, and developmental and cognitive impairments. Moreover, long-term arsenic exposure even at very low-level causes development of carcinogenic changes in the skin, liver, lung, bladder, and prostate (2). According to recent reports, chronic arsenic exposure around the WHO recommended level (10 μg/L) is also associated with increased risk of urinary tract cancer (6, 7). These evidence indicate that the current guideline for maximum permissible limit of arsenic in drinking water may still present a hazard to the population that are chronically exposed for a long time (8). Earlier researches on arsenic toxicity were primarily focused on population-based epidemiological outcomes, analysis of particular disease risk, chemical, and physiological aspects of arsenic metabolism, study of related gene expression profile, cancer, and DNA damage; all were associated with the mechanism of toxicity and subsequent outcome or disease manifestation. At present, the research perspective shifts toward the study of epigenetic alterations (DNA methylation, histone modification, and miRNA) to justify differential susceptibility toward arsenic exposure, detailed “omics” analysis (whole genome microarray, proteomic, and metabolomic profiling, etc.) of arsenic-induced cancer cases, bioremediation, and development of new therapeutic strategies, which are necessary for combating the outcome of arsenic toxicity as the affected population is increasing around the world. Since the year 2000, with advancement in high throughput techniques, arsenic research has evolved, and newer insights have been discovered (source: PubMed1, Figure 1). Research trends from the year 2010 to 2019 indicate that “arsenic metabolism” remained the key focused area for arsenic research (Figure 1). It is indeed important to know the arsenic methylation status, which has been discussed in the following section to understand its toxic effects on biological system, related disease manifestation, and individual susceptibility. The next important focused area was found to be DNA damage and cancer. Understanding epigenetics was increasingly emphasized for the last 5–6 years, where the researchers attempted to explore the role of DNA methylation, histone modification, and miRNA alteration in arsenic toxicity as well as in arsenic-induced carcinogenesis. In this review, we try to string together the recent perspectives of arsenic research with special focus on understanding differential susceptibility in exposed population and how the innovative thinking may soon formulate better remedial strategies against this menace (Figure 2).
Figure 1. Graphical representation showing trends in research publications on arsenic toxicity (from year 2000 to 2018).
Arsenic is the 20th most abundant element found in the earth's crust, with an average concentration of 1–2 mg/kg in the continental crust (9). However, there are some geographical hotspots where the content is very high. It includes parts of South America and South and Southeast Asia, the latter two being most populated among all the regions and harboring nearly 75% of the total affected humans mentioned earlier. Arsenic is mobilized into the environment by naturally occurring processes like rainwater leaching, weathering, and seismic and volcanic activities. Another potent source of arsenic is through the emissions of arsenic-laden fumes and wastes that are carried by natural vectors like wind and water, expanding the topological periphery. To date exposure to arsenic is mostly due to groundwater contaminations where inorganic arsenic (iAs) normally exists in the form of arsenite/As(III+) or arsenate/As(V+). Depending on the oxidation potential of the microenvironment, the two states are interconvertible. The pH of the microenvironment regulates the ligand exchange process between the metallic oxides and hydroxides of iAs and the organic intermediates (microbiota) to release arsenic species (10). Higher concentration of arsenic tends to occur in association with metal oxides of iron as well as minerals with high sulfur content. In the Indian subcontinent, the Ganga–Brahmaputra–Meghna basin of the Indo-Bangladesh delta has high deposition of alluvial soil rich in sulfide drained down from the Chota Nagpur region. Increased weathering and rhythmic leaching of arsenic into the water table reflects that the increase in human intervention in abuse of chemical fertilizers laden with arsenic to aid agricultural yield have been associated with incidence of arsenic toxicity (11). Earlier, it was proposed that the organometallic component of arsenic in the groundwater might form complex and subsequently associate strongly with the dissolved arsenic anions, decreasing the release of arsenic, but research has shown that organic decomposition by certain bacteria generates anaerobic conditions whereby they release the arsenic species from these solid phases (12, 13). Apart from drinking water, relevant amount of arsenic exposure has been accounted for diet, especially through rice (Figure 3, Supplementary Table 1). The exposure becomes more pronounced in the region of Bangladesh and India, as the arsenic-affected zone have population whose staple is rice, grown and consumed locally (14). This shows that the dynamic spectrum of arsenic transports into the human physiological domain, the effects of which are being discussed in the following section.
Figure 3. Graphical representation showing recent country-wise reports about arsenic concentration found in (A) drinking water, (B) rice, (C) fish, and (D) egg (detailed report is in Supplementary Table 1 with all references).
Majority of the affected population are exposed to arsenic primarily through drinking water and food. Several studies have described the mechanism of arsenic metabolism inside human biological system (15–17). The inorganic form of oxy-anions, including pentavalent arsenite (H2) and trivalent arsenate (H3AsO3) is present abundantly in natural water. Organic form of arsenic is rarely found in the environment (18). The major metabolic pathways of iAs in humans includes several biochemical reactions like oxidation, reduction, methylation, thiolation, and glutathiolation (15, 19), of which methylation is critically important for the toxic pathology, tissue distribution, and cellular retention of arsenic. Arsenic in the form of arsenite/As(III+) or arsenate/As(V+) is absorbed in the gastrointestinal tract (GIT) of human, where arsenite is absorbed more rapidly than arsenate. As(III+) enters into the cell through aquaglyceroporins [AQP3, AQP7, AQP9, and AQP10], and As(V+) uses phosphate transporters, respectively, whereas cellular efflux of arsenic primarily occurs through ATP-binding cassette transporters (MRP1, MRP2, and MRP5). Apart from these, certain glucose transporters (GLUT1 and GLUT5) and organic anion transporting polypeptides were also reported to be responsible for cellular arsenic uptake under different circumstances (15). The initial reduction in arsenate to arsenite is done by liver enzyme arsenate reductase. It is then methylated by arsenic(III) methyl transferase (AS3MT) in the presence of S-adenosyl-methionine (SAM) as methyl donor to monomethylarsonic acid (MMAv), which is reduced to monomethylarsonous acid (MMAIII). MMAIII is again methylated to dimethylarsinic acid (DMAv), which is subsequently reduced to dimethylarsinous acid (DMAIII). The main metabolites monomethylarsonic acid (MMAv) and dimethylarsinic acid (DMAv), where arsenic is in the pentavalent state, are less toxic compared to inorganic arsenic (iAs) and readily excreted in urine. In humans, during metabolism, some arsenic is accumulated (about 40–60%) in skin, hair, nails, muscle, bones, and teeth as iAs and MMA (MMAIII and MMAv), which may impart toxic effects in multiple tissues and organs in their later life (16, 18, 19). Another pathway of arsenic metabolism shows sequential addition of methyl groups to trivalent arsenicals and its conjugation with glutathione generating intermediate derivatives like arsenic triglutathione (ATG), monomethyl arsenic diglutathione (MADG), and dimethyl arsenic glutathione (DMAG). A third pathway explained about the formation of iAs/protein conjugate and subsequent generation of methylated metabolites (17). Various studies have also shown effect of folate intake and folate metabolism on arsenic metabolism and related disease risks. The amount of folate intake and genetic variants of folate metabolizing enzymes might be responsible for interindividual variation in arsenic metabolism and differential disease susceptibility (19). Relative distribution of arsenic metabolites in urine is commonly used as a biomarker of current exposure and indicates the individualistic metabolism efficiency, which is one of the major causes of differential susceptibility (16, 20). The percentage of urinary metabolite varies between individuals; most of the literature suggests 10–30% iAs, 10–20% MMA, and 60–80% DMA (19, 20). Conventionally, concentration ratios of MMA/iA and DMA/MMA in the urine indicate the methylation capacity of the affected individual. In addition, the activity of AS3MT might have an association with the tissue-specific retention of various arsenic metabolites in the body and subsequently with individual susceptibility (21, 22). Very few reports are available on thio-DMA as urinary metabolite. Raml et al. (23) identified thio-DMA in the urine samples of Bangladeshi women, and also reported from in vitro studies that it was about 10-fold more cytotoxic than dimethylarsinate; however, the specific health consequences of such metabolites are not yet known (22). In another work by Taylor et al. (24), the urine samples from arsenic-contaminated seaweed consumers had been analyzed, and thio-DMA was detected as urinary metabolite. Future studies are required to identify and evaluate the possible outcome of such unique metabolites for better understanding of the toxic nature of this metalloid.
The major hallmark of arsenic toxicity is the occurrence of dermatological lesions of various types like raindrop hypopigmentation, pigmentation, keratosis (palmer and plantar), and even skin cancers like basal cell carcinoma (BCC), squamous cell carcinoma (SCC), and Bowen's disease. Interestingly, only 15–20% of the population show such manifestations (25, 26). Based on the toxic outcome on human health, arsenic is considered as Group I human carcinogen. In recent years, in vitro work with human cancer cell lines have helped to unravel various toxic mechanisms related to dermatological health outcomes and cancerous outcomes of the liver, lungs, bladder, and neuronal disorders, but most of the common health outcomes in human to date are dermatological lesions, peripheral neuropathy, liver damage, respiratory disorders, ocular inflammation and irritations, etc. (27–31). In the last decade, arsenic research at the cellular level revealed that arsenic alters the gene expression pattern within the cell and alters telomere length, epigenomic profile, cell cycle, etc. (32–36). One of the most explored aspects of arsenic toxicity in last decade has been on DNA damage and repair mechanism. Studies both in humans as well as cell lines have yielded results, which have made the detection of genetic damage as a reliable biomarker for arsenic-induced toxic outcomes (31, 37–39). Both chromosomal aberrations as well as micronucleus have been associated strongly with arsenic exposure and have shown prominent correlation to arsenic toxicity when compared with unexposed human subjects (40–42).
Arsenic-induced characteristic skin lesions have been considered for years as the hallmark of chronic toxicity. We have found that exposed individuals, residing in the same area, show varied dermatological lesion phenotypes even after consuming equivalent amount of arsenic over the same duration of time. In fact, our observation suggests that only a smaller percentage of the exposed individuals show arsenic-induced characteristic skin lesions (43). On the other hand, the precancerous lesions like plantar and palmer hyperkeratosis often lead toward detrimental malignancies in some of the affected individuals, whereas others retain only the precancerous forms lifelong. Different researches indicate several factors like individual arsenic methylation capacity, genetic susceptibility, epigenetic profile, etc. as major role players behind the differential susceptibility (16, 44).
The interindividual variation in arsenic methylation potential could be an important predictor of individual's susceptibility. Majority of the researchers found that people with skin lesions and high arsenic exposure are likely to have reduced arsenic methylation capacity with high trivalent species MMA in urine (45–48). The methylation capacity might reduce with increasing dose of arsenic, smoking and alcohol consumption, age, and nutritional folate deficiency (46, 49, 50). On the contrary, one study from China reported about higher methylation capacity among people above 40 years of age compared to below 40 years, but they also found positive correlation between %MMA and risk of skin lesion. On the other hand, women, especially at pregnancy, have increased methylation capacity than men and non-pregnant women, respectively, which may be due to the effect of estrogen, and children also have better methylation capacity than adults (51–53). A case–control study from China reported similar total arsenic concentration in skin lesion and no skin lesion group, but they found increased concentration of MMA among the skin lesion individuals (54), which indicates that efficiency of arsenic methylation is indeed important for differential susceptibility. A detailed account of recent studies regarding arsenic methylation efficiency and risk of skin lesion is summarized in Table 1. Arsenite methyltransferase (As3MT; EC: 2.1.1.137) catalyzes both monomethyl arsenate (MMA) as well as dimethyl arsenate (DMA) using SAM and arsenite and MMA as substrates, respectively. It oxidizes SAM to S-adenosyl L-homocysteine (SAH) in both cases. Recently, using X-ray crystallography, the molecular structure of As3MT in conjugation with AsIII and SAH have been resolved (69). Several single nucleotide polymorphic (SNP) forms of As3MT have been identified in relation to arsenic. In the next section, we try to evaluate the structural aspects of As3MT polymorphisms along with SNPs in other relevant genes in relation to arsenic-induced toxic outcomes.
Table 1. Summary of studies on individual arsenic methylation capacity and risk of skin lesions in chronic arsenic exposed population.
Previous reports indicate that arsenic-induced health effects might be more deleterious among the exposed population carrying susceptible variants of genes primarily related to arsenic metabolism, oxidative stress, DNA damage repair pathways, etc. Variations in the gene for AS3MT have been shown to be the most influential parameter in urinary arsenic metabolites and different disease manifestations including carcinogenic outcome. Several population-based association studies were conducted with a number of SNP sites, among which G>A change in the C10orf32 region (rs 9527) was found to be associated with increased skin lesion risk in Indian (70) and Bangladesh population (71). AS3MT, Met287Thr polymorphisms (rs11191439) were reported to be having different arsenic methylation efficiency compared with the wild type and associated with risk of development of skin lesions, bladder cancer, and increased micronucleus frequency (72, 73). Apart from AS3MT, other important genes related to arsenic metabolism are Purine nucleoside phosphorylase (PNP), methylenetetrahydrofolate reductase (MTHFR), methyltetrahydrofolate-homocysteine methyltransferase (MTR), cystathionine-beta-synthase (CBS), glutathione S-transferase omega 1 (GSTO1), and glutathione S-transferase omega 2 (GSTO2). MTHFR catalyzes the biochemical conversion of 5,10-methylenetetrahydrofolate (5,10-methyl-THF) to 5-methyltetrahydrofolate (5-methyl-THF) during the formation of SAM, which acts as a methyl donor of arsenic methylation. MTHFR, C677T, and C1298A polymorphism was reported to be associated with increased urinary MMA%, decreased DMA%, and risk of arsenic-induced skin lesion in arsenic exposed population from Bangladesh, Argentina, and Taiwan (57, 74, 75). Chen et al. (76) observed that individuals with the MTHFR 677TT/1298AA and 677CT/1298AA genotypes were 1.66 and 1.77 times more susceptible to develop skin lesions, compared with those having 677CC/1298CC genotype. Catalyzing the reduction in AsV to AsIII is one of the functions of PNP during arsenic metabolism. A case–control study on 428 subjects from arsenic-exposed region of West Bengal, India found that polymorphisms of PNP, His20His, Gly51Ser, and Pro57Pro were significantly associated with arsenic-induced skin lesions risk (77). Similar type of studies depicting association between PNP SNPs and arsenic-induced health effects were discussed in Supplementary Table 2. CBS catalyzes the conversion of homocysteine to cystathionine, which has an influence on arsenic methylation. Two studies on arsenic-exposed population from Argentina found C234709T and G4920037A variants of CBS to be associated with urinary MMA% (19, 78). However, to date, there are no reports from any other population regarding the CBS polymorphism, and thus, more studies are needed to confirm its association irrespective of ethnicity. GSTOs are another group of genes participating in arsenic metabolism. Several studies found significant association of GSTO1 polymorphic variants with the risk of skin lesion and cancer. Previous studies reported that Ala140Asp was associated with urinary MMA% and skin cancer risk in population chronically exposed to arsenic from drinking water from Bangladesh, Taiwan, and China (54, 57, 74). However, studies on arsenic exposed population from India, Mexico, Hungary, Romania, Slovakia, and USA did not find any significant association of GSTO1 polymorphism and arsenic-induced disease etiology (72, 77, 79, 80). Luo et al. (81) reported that, for GSTO2, AG genotype for rs156697 and the AG genotype or at least one G allele for rs2297235 had an increased risk of arsenic-induced skin lesions, and for GSTO1, individuals carrying at least one C allele for the rs11191979 polymorphism or at least one A allele or the AA genotype for rs2164624 or at least one A allele for rs4925 showed a significant risk of arsenic-induced skin lesions. Glutathione S-transferases (GSTs, including GSTM1, GSTT1, and GSTP1) are important protectors for arsenic-related oxidative stress. GSTP1, Ile105Val polymorphism was reported to be associated with skin lesion and urinary arsenic profile among arsenic-exposed population from Bangladesh (82), China (83), and Vietnam (22), whereas no association was reported from the population of India (43) and Turkey (84). Ghosh et al. (43) did not find any association of GSTT1 null genotype with arsenic-induced skin lesion in a study on 422 Indian subjects but reported that GSTM1-positive genotypes are associated with a high risk of skin lesions. On the other hand, McCarty et al. (58) reported that wild-type GSTT1 is associated with a higher risk of skin lesions than null genotype, but no association was found in case of GSTM1. In a recent study on 241 people from Italy, no association was found between arsenic exposure and urinary arsenic profile (85). Genetic variants in BER-pathway-associated genes such as 8-oxoguanine DNA glycosylase (OGG), X-ray and repair and cross-complementing groups 1 and 3 (XRCC1, XRCC3), and apurinic/apyrimidinic endonuclease (APE1) may alter the genotoxicity of arsenic. Multiple case–control studies reported about the association of polymorphic variants of these genes with arsenic-induced disease risk. A detailed description of most recent (considering last 10 years) population-based polymorphism studies stating population size, source of arsenic exposure, and polymorphic variant associated with the particular disease have been summarized in Supplementary Table 2. To date, several population-based studies revealed significant association between the genotypic variants and arsenic-induced disease manifestation; however, the exact mechanistic aspect behind the role of single nucleotide polymorphism of a specific gene in understanding differential susceptibility still remains questionable.
Recent researches identified epigenetic regulations, which include primarily DNA methylation, histone modification, and miRNA interaction as one of the critical regulators of arsenic-induced disease manifestations. Dynamic reversibility of epigenetic marks is a truly significant property, and it may pave the pathway of epitherapeutics to overcome the road blocks in developing potential drug targets for curing diseases due to arsenic toxicity. Smeester et al. (86) did a comprehensive examination of DNA methylation levels within CpG islands for over 14,000 genes among arsenic exposed with skin lesion (arsenicosis cases) and without skin lesion individuals. They found 182 hypermethylated genes in arsenicosis cases, the majority of which is involved in cancer-associated pathways. A whole genome microarray-based study was conducted on Bangladesh population, where 10 subjects with newly developed skin lesion and 10 no skin lesion were selected from a previous cross-sectional study of 957 individuals to evaluate the possible epigenetic changes. Results indicated DNA methylation changes over time in people having arsenic-induced skin lesions compared to control. They found top 20 differentially methylated CpG sites of which 13 CpGs (TCEB3B, CYC1, CDH4, RHBDF1, CCDC154, JAKMIP3, AGAP2, PL-5283, CHPF, PPAP2C, PCNT, SLC6A3, and MAP3K1) were increased in % methylation, and 7 CpGs (MYO3B, KIAA1683, LOC642597, C2orf81, ESRRG, PRDM9, and TNXB) were decreased in % methylation between baseline and follow-up (87). Majumder et al. (88) observed a correlation pattern between different stages of arsenic-induced skin lesion and whole genome DNA methylation. A study on Bangladesh population showed that genomic hypomethylation of peripheral blood lymphocyte DNA is associated with 1.8-fold increase risk for skin lesions (89). Another genome-wide DNA methylation study on 120 individuals from China found changes in global DNA methylation among patients afflicted with arsenical skin lesions. They also depicted about detectable DNA methylation changes due to arsenic exposure over the generations even though exposure occurred decades ago. Chanda et al. (90) observed GMDS gene fragment hypermethylation in the peripheral blood leukocyte DNA of skin cancer persons exposed to arsenic and suggested as a biomarker for arsenic-induced cancer. AS3MT gene plays an important role in arsenic metabolism and its toxicological response. Gribble et al. (91) found marked promoter hypomethylation AS3MT gene in arsenic-exposed population from Arizona, but no reports are found regarding the relationship between skin lesion status and AS3MT promoter methylation to date. It will be an interesting and important finding for future researchers, which will help in mechanistic understanding of how epigenetic modification of AS3MT contributes in differential susceptibility. In another study by Janasik et al. (92), significant promoter hypermethylation of NRF2 and KEAP1 was observed among occupationally arsenic-exposed copper mill workers from Poland. Our group had reported about mitochondrial DNA hypomethylation among arsenic-exposed individuals from highly arsenic affected areas of West Bengal, India, but no significant difference between with and without skin lesion group was observed. However, mitochondrial DNA copy number was found to be significantly elevated among skin lesion individuals with increased expression of electron transport chain complex I, subunit ND6 and ND4 genes (93). Recently, we had reported about significant promoter hypomethylation with increased expression of mitochondrial biogenesis regulatory genes, Tfam and PGC1α, among arsenic-induced skin lesion individuals compared to no skin lesion group, chronically exposed to arsenic through drinking water (94). Few studies also reported about altered promoter methylation of various important genes and subsequent change in gene expression among individuals with skin lesion compared to those without skin lesion explaining the vital role of epigenetic changes behind differential susceptibility (Table 2). High-throughput whole genome omics studies are useful tools to analyze and identify specific gene expression alterations in response to arsenic toxicity. In a study of proteomic profiling of arsenic-induced keratosis samples, three key proteins were identified, which were consistently differentially expressed in lesional skin compared to unaffected skin. The cadherin-like transmembrane glycoprotein, desmoglein 1 (DSG1), was suppressed, whereas the expression of keratin 6c (KRT6C) and fatty acid binding protein 5 (FABP5) were significantly increased (100). Argos et al. (101) analyzed the effect of arsenic toxicity on the development of arsenical skin lesion status by genome-wide gene expression patterns, where the expression of about 22,000 transcripts was evaluated between with skin lesion and without skin lesion group. They found 468 differentially expressed genes between the two groups. The presence of genomic deletion(s) in a number of genes (OR5J2, GOLGA6L7P, APBA2, GALNTL5, VN1R31P, PHKG1P2, SGCZ, ZNF658) and long intergenic non-coding RNA (lincRNA) genes (RP11-76I14.1, CTC-535 M15.2, RP11-73B2.2) were associated with higher risk for development of skin lesions independent of gender, age, and arsenic exposure (102). In one study, HaCaT cell line was treated with low dose of arsenic (100 nM sodium arsenite) for 6 months, and then, SILAC-based quantitative proteomics approach resulted in the identification of 2,111 proteins, among which 42 proteins were found to be overexpressed and 54 downregulated upon chronic arsenic exposure (103). Altogether, these studies provide insight into molecular alteration behind differential susceptibility.
Table 2. Studies on arsenic induced alterations in promoter DNA methylation related to skin lesion status.
Another important regulator of epigenetic machinery is the different miRNAs, which may play vital roles behind arsenic-induced individual susceptibility. In a recent study, total miRNA expression analysis was done on premalignant and malignant skin lesion tissues (basal cell carcinoma and squamous cell carcinoma) from an Indian population chronically exposed to arsenic. A total of 35 miRNAs were reported to be differentially expressed among the three lesion types analyzed. Two miRNAs (miR-425-5p and miR-433) were increased in both BCC and SCC relative to hyperkeratosis, indicating their association with malignancy. Two other miRNAs (miR-184 and miR-576-3p) were activated in SCC relative to both BCC and hyperkeratosis, suggesting selective induction in tumors capable of metastasis. Six miRNAs (miR-29c, miR-381, miR-452, miR-487b, miR-494, and miR-590-5p) were selectively suppressed in BCC relative to both SCC and hyperkeratosis (104). A previous study of Banerjee et al. (105) reported about 4.5-fold upregulation of miR21 in skin lesion individuals compared to the no skin lesion group. The expression of the downstream targets of miR21 (PTEN and PDCD4) varied inversely, but the expression of pAKT and PI3K varied proportionately with its expression levels. Another study on arsenic-treated HaCaT cell line identified differential expression of 30 miRNAs of which miR-21, miR-200a, and miR-141 might play a role in skin carcinogenesis (106). Very few studies are so far reported regarding this area, and thus, it is indeed an interesting field for present day researchers to enlighten with newer findings.
Quite a few studies showed arsenic-induced alteration in post-translational histone modifications (PTHM) including H3K36me2, H3K36me3, H3K79me2, H3K27me3, H3K9me2, H3K18ac, H3K9me2, H4K16ac, etc. (107, 108, 108–111)]. Most of them are either in vitro or in mouse models, but population-based studies justifying difference between with and without skin lesion individuals are really scarce. Cantone et al. (112) identified that increased H3K4me2 and H3K9ac is associated with inhaled arsenic particulate matter in steel plant workers. Two different studies on arsenic-exposed Bangladesh population observed alteration in H3K9me2, H3K9ac, H3K4me3, H3K27me3, H3K27ac, and H3K36me2 moieties (113, 114). Pournara et al. (115) reported decreased H3K9me3 and unaltered H3K9ac among a population from Argentina, chronically exposed to arsenic through drinking water. A study on Chinese population exposed to arsenic from indoor coal combustion reported that modifications of H3K18ac, H3K9me2, and H3K36me3 are associated with the degree of oxidative damage and the severity of arsenicosis. However, none of the studies particularly differentiate the degree of altered PTHM between arsenic-exposed skin lesion phenotypes compared to those with no skin lesion. Recently, our group has reported about two different PTHMs among chronic arsenic-exposed population from India considering the skin lesion status (38, 116). We identified significant upregulation of H3K79me1 in individuals with arsenic-induced skin lesion, and H3K79me1 was found to be regulated by the upstream methyltransferase DOT1L. Again, significant downregulation of H3K36me3 was found in the arsenic-exposed with skin lesion individuals with an impairment of mismatch repair pathway activated by arsenic-induced DNA damage. Cell-line-based in vitro studies and animal model are necessary for the identification of detailed molecular mechanism and for the observation of the outcome of advanced therapeutics (113). However, it is noteworthy to mention that only population-based studies of arsenic toxicity may confer greater opportunities in epitherapeutic drug development and identification of early biomarker for arsenicosis.
One of the ready solutions of arsenic removal is the reduction at source and providing an alternative source of drinking water. Arsenic comes into our body mainly through drinking water sources and a variable dietary intake of food grown in contaminated areas (Figure 3, Supplementary Table 1). With more than 140 million individuals affected, it might not be possible to provide better infrastructure in the removal of arsenic for daily consumption of arsenic-safe diets. The huge economic burden due to the population size makes it difficult to implement the strategies. Though beneficial for the population, the cost of installation of new wells of 1,000 ft deep or more is prohibitive. Another major challenge is the maintenance of already installed filters. After few years of installation, these filters either did not work properly or gets saturated with arsenic.
This reduction can be done in two ways: (i) alternative arsenic-safe water source and (ii) treatment of arsenic-contaminated water. A comprehensive report is available with detailed pros and cons of the several methods (Wilsonweb, Water supply options2). Alternative sources include groundwater [deep tube well, shallow shrouded tube well (SST) and very shallow shrouded tube well (VSST), dug well, infiltration galley], surface water (protected ponds, pond sand filters, combined filters, household filters), and rain water harvesting. Apart from these alternative water sources, arsenic can also be removed from water by various treatments. For example, solar oxidation of arsenic kept in transparent bottles effectively reduce the arsenic load by increased oxidation from As(III+) to As(V+). The common rural practice of storing water for longer period of time in clear container reduces the arsenic concentration through sedimentation (Figure 4). Another effective way to remove arsenic is through coprecipitation and adsorption processes after reduction of As(III+) to As(V+) by bleaching powder (chlorine) or potassium permanganate. Aluminum alum, Al2(SO4)3.18H2O, ferric chloride, FeCl3, and ferric sulfate, Fe2(SO4)3.7H2O, are some of the commonly used coagulants. This technology can be used in our daily households with some simple adaptations—popularly known as Bucket Treatment Unit (BTU) (Wilsonweb, Water supply options2). Additionally, various sorptive media columns with activated alumina, iron-coated sand, granular ferric hydroxide, etc. are commercially available. The most efficient way to remove arsenic is the use of membrane techniques like reverse osmosis, nano filtration, and electrodialysis, but these are highly expensive for mass scale installation.
Rice being the staple food for billions of people, it significantly contributes more arsenic than any other food source (16, 117). Irrigation with arsenic-contaminated groundwater poses another major threat to public health through food-chain contamination. Conventional irrigation system in flooded paddies increases the arsenic uptake further (117). Recent studies have ascertained that the adaptation of different agronomic techniques can effectively reduce bioaccumulation in rice grains (118). For example, rice grown aerobically significantly has low arsenic accumulation compared to those grown in flooded condition (119). Again, another study reported the reduction in the total As during Sprinkler irrigation compared to traditional flooding (120). Few adaptations in cooking method can effectively lessen levels of arsenic within the grains. For example, a significant decrease was observed by thoroughly rinsing and then cooking the grains in an excessive amount of water, thereby increasing the percolation rate as arsenic is “mobile” in liquid water (117). A study in West Bengal showed that the traditional cooking method is most effective in lowering the concentration and the atab rice type with the lowest arsenic content (121). A recent review has discussed the possibility of genetically modified rice that would accumulate less arsenic within the rice grains (122). Very recently, rice varieties have been screened and developed that accumulate lesser arsenic, as consumption of arsenic-containing rice has been reported to cause genetic damage in humans (123–126). A recent review has discussed the possibility of genetically modified rice that would accumulate less arsenic within the rice grains (122).
Microbiological interactions are crucial for the mobilization of arsenic into the aqueous phase leading to arsenicosis (127). Several arsenate-resistant microbes (ARMs) reduce As(V) to As(III) to thrive on the extreme conditions with high arsenic exposure. Recently, scientists have isolated a microorganism, which uses arsenic to “breathe,” from Searles Lake with extremely unfavorable conditions (10 times saltier and 70 times more caustic, alkaline, pH 9.8) and loaded with toxic arsenic (~300 mg/L total arsenic) (Toxic Substances Hydrology Program, The U.S. Geological Survey3). These organisms could be used as a way of arsenic removal from water. Later, the applicability of the study remains undetermined (128). Water-usage switch from surface to groundwater to reduce the risk of microbial contamination increased the risk of arsenic contamination. Two rod-shaped Gram-positive bacteria was reported to remove 51.45 and 51.99% of arsenite and 53.29 and 50.37% of arsenate, respectively, from arsenic-containing culture media isolated from Purbasthali block of Burdwan, West Bengal, India (129).
Studies have found that administering dietary supplements like vitamin C, different spectra of medicinal plants, drugs, etc. have reduced the effects of arsenic toxicity, although such observations have not been reported or worked out in humans (130–132). Vitamin C or ascorbic acid is a good antioxidant, which explains the reduction in oxidative stress created by arsenic-induced generation of reactive oxygen species (ROS) and in turn ameliorates the ill effects of arsenic within the body. Activation of antioxidative defense system within the body may be better and quicker. Nrf2 pathway is one of the prime pathways for the activation of antioxidant enzymes in the body (133–135). Research has shown that tea polyphenol brings about systemic activation of the Nrf2 pathway (136–138). We discussed in earlier sections that arsenic exposure depletes the methylation pool within the system. Studies suggest that administration of SAM reverses and/or reduces the degree of arsenic-induced DNA damage and anomalies in vitro (126, 139). Hence, a concoction of dietary supplements and reduction in arsenic intake can lead to better lives of the population affected by arsenic (Figure 4).
Interindividual susceptibility plays a key role in arsenic toxicity, which is already evident from the multiple research outcomes. Other than the variation in arsenic biotransformation, host-specific genetic make-up, and epigenetic regulation, an array of other environmental and physiological factors including nutritional status, lifestyle, effect of any other inherited or sporadic disease stage, and coexposure toward other heavy metals may regulate the extent of arsenic-induced skin lesion. Differential rate of arsenic methylation has been reported in many studies, where primarily a correlative outcome suggests that a higher primary methylation index is related to risk of skin lesion (Reduction in Arsenic at Source, Table 1). However, the complex mechanism of biotransformation behind skin lesion manifestation still remains elusive. Association studies on SNP and arsenic exposure in different populations have identified risk variants for developing skin lesion, several types of cancer, and other adverse diseases (Adaptation of Cooking and Irrigation Practices, Supplementary Table 2). However, there is inconsistency in the results because of population from different geographic areas, ethnicity, source of exposure, sample size, etc. Some studies are also limited on a single population and thus needed further validation [Supplementary Table 2, e.g., arsenic-metabolism-related genes PEMT and DHFR SNPs were validated only in Bangladesh population (140); similarly CBS SNP was analyzed only in Argentina population by (19, 78); in case of DNMTs, Adiponectin, and INPP5A genes single reports were found to date by Seow et al. (140–142), respectively]. The role of epigenetic modification to explain differential susceptibility is one of the most recent advances in arsenic research, having an interesting future prospect in the development of epitherapeutics. Besides the arsenic-related health effects, there are several other problems among the exposed population living in the arsenic-affected countries like India and Bangladesh, such as socioeconomic status, orthodox beliefs and stigma, lack of awareness, and poor maintenance of infrastructure. One of the best ways to counter arsenic-induced health problems is the intake of a protein-rich diet, as study showed that low intake of protein, choline, or methionine can reduce arsenic metabolism and excretion through urine. With a very low income, such a diet is not possible by the majority of the exposed population, leading to an ever-increasing number of susceptible individuals. It is suggested that citrus-based fruits with loads of ascorbic acid can also help in countering such a toxic effect (143, 144). Furthermore, different social stigma, superstitions, and prejudices about the skin symptoms further worsen the situation. In our own studies (38, 93, 116), while performing random sampling in such affected areas, we have faced discontent and discomfort among individuals and family members to acknowledge the fact of skin lesions like skin darkening, black spots, nodules, gangrene, and cancer in limbs, which causes social isolation of the victims. This stigma makes it difficult for our onboard dermatologists to determine whether an individual's dermatological lesion is due to arsenic or something else. While the public health communities and individual research organizations attempted to reach these populations, they refuse to interact with them, as no direct benefit could be offered toward them. Though years of research have been done, clinical implementation remained unsuccessful due to lack of risk–benefit analysis. Large population size is another major hindrance to implement any preventive measure like potable water supply to population at risk. Till now, no comprehensive therapeutic strategy has been tested. The natural means includes reduction in arsenic load in the drinking water, consuming protein-rich diet; ascorbates and polyphenols contained in regular beverages like tea seem to be the likely source of relief. In many parts of India and Bangladesh, the rural population fails to avail of arsenic-free water or high-protein diet due to lack of knowledge, financial restraints, and poor government infrastructure, and, on top of that, social taboo has kept the situation worse. Governments of developing countries cannot support the huge financial burden to set up arsenic-free deep tube wells or to distribute the arsenic removal domestic filter at individual scale. Even though, in few areas, arsenic filtration units have been installed, the durability of these units is less due to lack of filter replacement, poor monitoring, and delayed implementation. Therefore, the combating strategy is preferred to be bottom–up approach, where people will be aware of alternative and safe water sources along with suggested modification in cooking and irrigation practice (Figure 1). A comprehensive plan of action needs to be implemented, which should be disseminated among the affected people through awareness sessions.
The problem of arsenic poising is intertwined with several other factors like social beliefs, geological risk factors, poverty, and lack of awareness apart from its associated health risks that further worsen the scenario. Arsenic-induced dermatological anomalies often causes social isolation of affected individuals due to beliefs that arsenic poisoning is a “curse” or may be “contagious” in nature. Researches across the globe are trying to develop a sustainable solution, which is still missing. A holistic multidisciplinary approach is needed to combat with the menace. A lot of data have been generated, and several aspects have been explored. Research is now focused more on mechanistic detailing of individual risk of toxicity and developing strategies to counter arsenic toxicity both at the molecular and environmental perspectives. It needs a conjoint/consensus and a multidisciplinary approach to counter this environmental menace, a slow killer and showing an ever-increasing presence over the years.
TS had written the manuscript, composed figures, and tables. PB (2nd author) contributed in manuscript writing and figure composition. SP had contributed in manuscript writing. PB (4th author) had supervised the work and given necessary inputs. All authors contributed to the article and approved the submitted version.
The authors declare that the research was conducted in the absence of any commercial or financial relationships that could be construed as a potential conflict of interest.
We are grateful to our research team for their support and cooperation.
The Supplementary Material for this article can be found online at: https://www.frontiersin.org/articles/10.3389/fpubh.2020.00464/full#supplementary-material
As, arsenic; DMAIII, dimethylarsonous acid; DMAv, dimethylarsonic acid; DNA, deoxyribonucleic acid; iAs, inorganic arsenic; miRNA, microRNA; MMAIII, monomethylarsonous acid; MMAv, monomethylarsonic acid; RNA, ribonucleic acid; SAM, S-adenosyl-methionine; WHO, World Health Organization.
1. ^https://www.ncbi.nlm.nih.gov/pubmed/ (accessed February 20, 2019).
2. ^http://wilsonweb.physics.harvard.edu/arsenic/conferences/Feroze_Ahmed/Sec_3.htm (accessed February 20, 2019).
3. ^https://toxics.usgs.gov/ (accessed February 20, 2019).
1. World Health Organization (2018). Available online at: http://www.who.int/news-room/fact-sheets/detail/arsenic (accessed February 20, 2019).
2. Naujokas MF, Anderson B, Ahsan H, Aposhian HV, Graziano JH, Thompson C, et al. The broad scope of health effects from chronic arsenic exposure: update on a worldwide public health problem. Environ Health Perspect. (2013) 121:295–302. doi: 10.1289/ehp.1205875
3. Coulson EJ, Remington RE, Lynch KM. Toxicity of naturally occurring arsenic in foods. Science. (1934) 80:230–1. doi: 10.1126/science.80.2071.230
4. Moxon AL. The effect of arsenic on the toxicity of seleniferous grains. Science. (1938) 88:81. doi: 10.1126/science.88.2273.81
5. Jang YC, Somanna Y, Kim H. Source, distribution, toxicity and remediation of arsenic in the environment – a review. Int J Appl Environ Sci. (2016) 11:559–81.
6. Colin P, Koenig P, Ouzzane A, Berthon N, Villers A, Biserte J, et al. Environmental factors involved in carcinogenesis of urothelial cell carcinomas of the upper urinary tract. BJU Int. (2009) 104:1436–40. doi: 10.1111/j.1464-410X.2009.08838.x
7. Saint-Jacques N, Parker L, Brown P, Dummer TJ. Arsenic in drinking water and urinary tract cancers: a systematic review of 30 years of epidemiological evidence. Environ Health. (2014) 13:44. doi: 10.1186/1476-069X-13-44
8. Sage AP, Minatel BC, Ng KW, Stewart GL, Dummer TJB, Lam WL, et al. Oncogenomic disruptions in arsenic-induced carcinogenesis. Oncotarget. (2017) 8:25736–55. doi: 10.18632/oncotarget.15106
9. National Research Council. Arsenic, Medical and Biological Effects of Environmental Pollutants. Washington, DC: The National Academies Press (1977). Available online at: https://www.nap.edu/catalog/9003/arsenic-medical-and-biological-effects-of-environmental-pollutants (accessed February 20, 2019).
10. Gu B, Schmitt J, Chen Z, Liang L, McCarthy JF. Adsorption and desorption of natural organic matter on iron oxide: mechanisms and models. Environ Sci Technol. (1994) 28:38–46. doi: 10.1021/es00050a007
11. Shankar S, Shikha SU. Arsenic contamination of groundwater: a review of sources, prevalence, health risks, and strategies for mitigation. Sci World J. (2005) 1–18. doi: 10.1155/2014/304524
12. Chatain V, Bayard R, Sanchez F, Moszkowicz P, Gourdon R. Effect of indigenous bacterial activity on arsenic mobilization under anaerobic conditions. Environ Int. (2005) 31:221–6. doi: 10.1016/j.envint.2004.09.019
13. Redman AD, Macalady DL, Ahmann D. Natural organic matter affects arsenic speciation and sorption onto hematite. Environ Sci Technol. (2002) 36:2889–96. doi: 10.1021/es0112801
14. Mondal D, Banerjee M, Kundu M, Banerjee N, Bhattacharya U, Giri AK, et al. Comparison of drinking water, raw rice and cooking of rice as arsenic exposure routes in three contrasting areas of West Bengal, India. Environ Geochem Health. (2010) 32:463–77. doi: 10.1007/s10653-010-9319-5
15. Roggenbeck BA, Banerjee M, Leslie EM. Cellular arsenic transport pathways in mammals. J Environ Sci. (2016) 49:38–58. doi: 10.1016/j.jes.2016.10.001
16. Bhattacharjee P, Chatterjee D, Singh KK, Giri AK. Systems biology approaches to evaluate arsenic toxicity and carcinogenicity: an overview. Int J Hyg Environ Health. (2013) 216:574–86. doi: 10.1016/j.ijheh.2012.12.008
17. Chi L, Gao B, Tu P, Liu C, Xue J, Lai Y, et al. Individual susceptibility to arsenic-induced diseases: the role of host genetics, nutritional status and the gut microbiome. Mamm Genome. (2018) 29:63–79. doi: 10.1007/s00335-018-9736-9
18. Shuyu AS, Mian X, Hafeez A, Wang X, Hussain HI, Iqbal Z, et al. Metabolism and toxicity of arsenicals in mammals. Environ ToxicolPharmacol. (2016) 48:214–24. doi: 10.1016/j.etap.2016.10.020
19. Steinmaus C, Yuan Y, Kalman D, Rey OA, Skibola CF, Dauphine D, et al. Individual differences in arsenic metabolism and lung cancer in a case-control study in Cordoba, Argentina. Toxicol Appl Pharmacol. (2010) 247:138–45. doi: 10.1016/j.taap.2010.06.006
20. Fängström B, Hamadani J, Nermell B, Grandér M, Palma B, Marie V. Impaired arsenic metabolism in children during weaning. Toxicol Appl Pharmacol. (2009) 239:208–14. doi: 10.1016/j.taap.2008.12.019
21. Agusa T, Iwata H, Fujihara J, Kunito T, Takeshita H, Minh TB, et al. Genetic polymorphisms in AS3MT and arsenic metabolism in residents of the Red River Delta, Vietnam. Toxicol Appl Pharmacol. (2009) 236:131–41. doi: 10.1016/j.taap.2009.01.015
22. Agusa T, Iwata H, Fujihara J, Kunito T, Takeshita H, Minh TB, et al. Genetic polymorphisms in glutathione S-transferase (GST) superfamily and arsenic metabolism in residents of the Red River Delta, Vietnam. Toxicol Appl Pharmacol. (2010) 242:352–62. doi: 10.1016/j.taap.2009.11.007
23. Raml R, Rumpler A, Goessler W, Vahter M, Li L, Ochi T, et al. Thio-dimethylarsinate is a common metabolite in urine samples from arsenic-exposed women in Bangladesh. Toxicol Appl Pharmacol. (2007) 222:374–80. doi: 10.1016/j.taap.2006.12.014
24. Taylor VF, Li Z, Sayarath V, Palys TJ, Morse KR, Scholz-Bright RA, et al. Distinct arsenic metabolites following seaweed consumption in humans. Sci Rep. (2017) 7:3920. doi: 10.1038/s41598-017-03883-7
25. Ghosh P, Banerjee M, De Chaudhuri S, Das JK, Sarma N, Basu A, et al. Increased chromosome aberration frequencies in the Bowen's patients compared to non-cancerous skin lesions individuals exposed to arsenic. Mutat Res. (2007) 632:104–10. doi: 10.1016/j.mrgentox.2007.05.005
26. Haque R, Mazumder DN, Samanta S, Ghosh N, Kalman D, Smith MM, et al. Arsenic in drinking water and skin lesions: dose-response data from West Bengal, India. Epidemiology. (2003) 14:174–82. doi: 10.1097/01.EDE.0000040361.55051.54
27. Alarifi S, Ali D, Alkahtani S, Siddiqui MA, Ali BA. Arsenic trioxide-mediated oxidative stress and genotoxicity in human hepatocellular carcinoma cells. Onco Targets Ther. (2013) 6:75–84. doi: 10.2147/OTT.S38227
28. Baidya K, Raj A, Mondal L, Bhaduri G, Todani A. Persistent conjunctivitis associated with drinking arsenic-contaminated water. J Ocul Pharmacol Ther. (2006) 22:208–11. doi: 10.1089/jop.2006.22.208
29. Kundu M, Ghosh P, Mitra S, Das JK, Sau TJ, Banerjee S, et al. Precancerous and non-cancer disease endpoints of chronic arsenic exposure: the level of chromosomal damage and XRCC3 T241M polymorphism. Mutat Res. (2011) 706:7–12. doi: 10.1016/j.mrfmmm.2010.10.004
30. Mathew L, Vale A, Adcock JE. Arsenical peripheral neuropathy. Pract Neurol. (2010) 10:34–8. doi: 10.1136/jnnp.2009.201830
31. Paul S, Das N, Bhattacharjee P, Banerjee M, Das JK, Sarma N, et al. Arsenic-induced toxicity and carcinogenicity: a two-wave cross-sectional study in arsenicosis individuals in West Bengal, India. J Expo Sci Environ Epidemiol. (2013) 23:156–62. doi: 10.1038/jes.2012.91
32. Calatayud M, Devesa V, Velez D. Differential toxicity and gene expression in Caco-2 cells exposed to arsenic species. Toxicol Lett. (2013) 218:70–80. doi: 10.1016/j.toxlet.2013.01.013
33. Chatterjee D, Bhattacharjee P, Sau TJ, Das JK, Sarma N, Bandyopadhyay AK, et al. Arsenic exposure through drinking water leads to senescence and alteration of telomere length in humans: a case-control study in West Bengal, India. Mol Carcinog. (2015) 54:800–9. doi: 10.1002/mc.22150
34. Hassani S, Khaleghian A, Ahmadian S, Alizadeh S, Alimoghaddam K, Ghavamzadeh A, et al. Redistribution of cell cycle by arsenic trioxide is associated with demethylation and expression changes of cell cycle related genes in acute promyelocytic leukemia cell line (NB4). Ann Hematol. (2018) 97:83–93. doi: 10.1007/s00277-017-3163-y
35. Li H, Engstrom K, Vahter M, Broberg K. Arsenic exposure through drinking water is associated with longer telomeres in peripheral blood. Chem Res Toxicol. (2012) 25:2333–9. doi: 10.1021/tx300222t
36. Liu JT, Bain LJ. Arsenic inhibits hedgehog signaling during P19 cell differentiation. Toxicol Appl Pharmacol. (2014) 281:243–53. doi: 10.1016/j.taap.2014.10.007
37. Bandyopadhyay AK, Paul S, Adak S, Giri AK. Reduced LINE-1 methylation is associated with arsenic-induced genotoxic stress in children. Biometals. (2016) 29:731–41. doi: 10.1007/s10534-016-9950-4
38. Bhattacharjee P, Sanyal T, Bhattacharjee S, Bhattacharjee P. Epigenetic alteration of mismatch repair genes in the population chronically exposed to arsenic in West Bengal, India. Environ Res. (2018) 163:289–96. doi: 10.1016/j.envres.2018.01.002
39. Jimenez-Villarreal J, Rivas-Armendariz DI, Pineda-Belmontes CP, Betancourt-Martinez ND, Macias-Corral MA, Guerra-Alanis AJ, et al. Detection of damage on single- or double-stranded DNA in a population exposed to arsenic in drinking water. Genet Mol Res. (2017) 16. doi: 10.4238/gmr16029241
40. Azizian-Farsani F, Rafiei G, Saadat M. Impact of sodium arsenite on chromosomal aberrations with respect to polymorphisms of detoxification and DNA repair genes. Int J Toxicol. (2014) 33:518–22. doi: 10.1177/1091581814557953
41. Hernandez A, Paiva L, Creus A, Quinteros D, Marcos R. Micronucleus frequency in copper-mine workers exposed to arsenic is modulated by the AS3MT Met287Thr polymorphism. Mutat Res Genet Toxicol Environ Mutagen. (2014) 759:51–5. doi: 10.1016/j.mrgentox.2013.09.010
42. Paul S, Bhattacharjee P, Mishra PK, Chatterjee D, Biswas A, Deb D, et al. Human urothelial micronucleus assay to assess genotoxic recovery by reduction of arsenic in drinking water: a cohort study in West Bengal, India. Biometals. (2013) 26:855–62. doi: 10.1007/s10534-013-9652-0
43. Ghosh P, Basu A, Mahata J, Basu S, Sengupta M, Das JK, et al. Cytogenetic damage and genetic variants in the individuals susceptible to arsenic-induced cancer through drinking water. Int J Cancer. (2006) 118:2470–8. doi: 10.1002/ijc.21640
44. Paul S, Giri AK. Epimutagenesis: a prospective mechanism to remediate arsenic-induced toxicity. Environ Int. (2015) 81:8–17. doi: 10.1016/j.envint.2015.04.002
45. Wei B, Yu J, Wang J, Yang L, Li H, Kong C, et al. The relationships between arsenic methylation and both skin lesions and hypertension caused by chronic exposure to arsenic in drinking water. Environ Toxicol Pharmacol. (2017) 53:89–94. doi: 10.1016/j.etap.2017.05.009
46. Zhang Q, Li Y, Liu J, Wang D, Zheng Q, Sun G. Differences of urinary arsenic metabolites and methylation capacity between individuals with and without skin lesions in Inner Mongolia, Northern China. Int J Environ Res Public Health. (2014) 11:7319–32. doi: 10.3390/ijerph110707319
47. McClintock TR, Chen Y, Bundschuh J, Oliver JT, Navoni J, Olmos V, et al. Arsenic exposure in Latin America: biomarkers, risk assessments and related health effects. Sci Total Environ. (2012) 429:76–91. doi: 10.1016/j.scitotenv.2011.08.051
48. Rasheed H, Kay P, Slack R, Gong YY. The effect of association between inefficient arsenic methylation capacity and demographic characteristics on the risk of skin lesions. Toxicol Appl Pharmacol. (2018) 339:42–51. doi: 10.1016/j.taap.2017.11.026
49. Yang J, Yan L, Zhang M, Wang Y, Wang C, Xiang Q. Associations between the polymorphisms of GSTT1, GSTM1 and methylation of arsenic in the residents exposed to low-level arsenic in drinking water in China. J Hum Genet. (2015) 60:387–94. doi: 10.1038/jhg.2015.39
50. Wei B, Yu J, Kong C, Li H, Yang L, Xia Y, et al. Effects of arsenic methylation and metabolism on the changes of arsenic-related skin lesions. Environ Sci Pollut Res Int. (2018) 25:24394–402. doi: 10.1007/s11356-018-2512-2
51. Gao J, Yu J, Yang L. Urinary arsenic metabolites of subjects exposed to elevated arsenic present in coal in Shaanxi Province, China. Int J Environ Res Public Health. (2011) 8:1991–2008. doi: 10.3390/ijerph8061991
52. Jansen RJ, Argos M, Tong L, Li J, Rakibuz-Zaman M, Islam MT, et al. Determinants and consequences of arsenic metabolism efficiency among 4,794 individuals: demographics, lifestyle, genetics, and toxicity. Cancer Epidemiol Biomark Prevent. (2016) 25:381–90. doi: 10.1158/1055-9965.EPI-15-0718
53. Tseng CH. A review on environmental factors regulating arsenic methylation in humans. Toxicol Appl Pharmacol. (2009) 35:338–50. doi: 10.1016/j.taap.2008.12.016
54. Fu S, Wu J, Li Y, Liu Y, Gao Y, Yao F, et al. Urinary arsenic metabolism in a Western Chinese population exposed to high-dose inorganic arsenic in drinking water: Influence of ethnicity and genetic polymorphisms. Toxicol Appl Pharmacol. (2014) 274:117–23. doi: 10.1016/j.taap.2013.11.004
55. Yu RC, Hsu KH, Chen CJ, Froines JR. Arsenic methylation capacity and skin cancer. Cancer Epidemiol Biomark. (2000) 9:1259–62.
56. Valenzuela OL, Borja-Aburto VH, Garcia-Vargas GG, Cruz-Gonzalez MB, Garcia-Montalvo EA, Calderon-Aranda ES, et al. Urinary trivalent methylated arsenic species in a population chronically exposed to inorganic arsenic. Environ Health Perspect. (2005) 113:250–4. doi: 10.1289/ehp.7519
57. Ahsan H, Chen Y, Kibriya MG, Slavkovich V, Parvez F, Jasmine F, et al. Arsenic metabolism, genetic susceptibility, and risk of premalignant skin lesions in Bangladesh. Cancer Epidemiol Biomarkers Prev. (2007) 16:1270–8. doi: 10.1158/1055-9965.EPI-06-0676
58. McCarty KM, Chen YC, Quamruzzaman Q, Rahman M, Mahiuddin G, Hsueh YM, et al. Arsenic methylation, GSTT1, GSTM1, GSTP1 polymorphisms, and skin lesions. Environ Health Perspect. (2007) 115:341–5. doi: 10.1289/ehp.9152
59. Su L, Cheng Y, Lin S, Wu C. Study of relationship between arsenic methylation and skin lesion in a population with long-term high arsenic exposure. Wei Sheng Yan Jiu. (2007) 36:336–40.
60. Lindberg AL, Rahman M, Persson LA, Vahter M. The risk of arsenic induced skin lesions in Bangladeshi men and women is affected by arsenic metabolism and the age at first exposure. Toxicol Appl Pharmacol. (2008) 230:9–16. doi: 10.1016/j.taap.2008.02.001
61. Lindberg AL, Sohel N, Rahman M, Persson LA, Vahter M. Impact of smoking and chewing tobacco on arsenic-induced skin lesions. Environ Health Perspect. (2010) 118:533–8. doi: 10.1289/ehp.0900728
62. Li X, Li B, Xu Y, Wang Y, Jin Y, Itoh T, et al. Arsenic methylation capacity and its correlation with skin lesions induced by contaminated drinking water consumption in residents of chronic arsenicosis area. Environ Toxicol. (2011) 26:118–23. doi: 10.1002/tox.20535
63. Wen J, Wen W, Li L, Liu H. Methylation capacity of arsenic and skin lesions in smelter plant workers. Environ Toxicol Pharmacol. (2012) 34:624–30. doi: 10.1016/j.etap.2012.07.003
64. Zhang Q, Wang D, Zheng Q, Zheng Y, Wang H, Xu Y, et al. Joint effects of urinary arsenic methylation capacity with potential modifiers on arsenicosis: a cross-sectional study from an endemic arsenism area in Huhhot Basin, northern China. Environ Res. (2014) 132:281–9. doi: 10.1016/j.envres.2014.04.036
65. Yang L, Chai Y, Yu J, Wei B, Xia Y, Wu K, et al. Associations of arsenic metabolites, methylation capacity, and skin lesions caused by chronic exposure to high arsenic in tube well water. Environ Toxicol. (2017) 32:28–36. doi: 10.1002/tox.22209
66. Wei B, Yu J, Yang L, Li H, Chai Y, Xia Y, et al. Arsenic methylation and skin lesions in migrant and native adult women with chronic exposure to arsenic from drinking groundwater. Environ Geochem Health. (2016) 39:89–98. doi: 10.1007/s10653-016-9809-1
67. Wei B, Yu J, Kong C, Li H, Yang L, Guo Z, et al. An investigation of the health effects caused by exposure to arsenic from drinking water and coal combustion: arsenic exposure and metabolism. Environ Sci Pollut Res Int. (2017) 24:25947–54. doi: 10.1007/s11356-017-0203-z
68. Niedzwiecki MM, Liu X, Zhu H, Hall MN, Slavkovich V, Ilievski V, et al. Serum homocysteine, arsenic methylation, and arsenic-induced skin lesion incidence in Bangladesh: a one-carbon metabolism candidate gene study. Environ Int. (2018) 113:133–42. doi: 10.1016/j.envint.2018.01.015
69. Packianathan C, Kandavelu P, Rosen BP. The Structure of an As(III) S-adenosylmethionine methyltransferase with 3-coordinately bound As(III) depicts the first step in catalysis. Biochemistry. (2018) 57:4083–92. doi: 10.1021/acs.biochem.8b00457
70. Das N, Giri A, Chakraborty S, Bhattacharjee P. Association of single nucleotide polymorphism with arsenic-induced skin lesions and genetic damage in exposed population of West Bengal, India. Mutat Res. (2016) 809:50–6. doi: 10.1016/j.mrgentox.2016.09.006
71. Pierce BL, Kibriya MG, Tong L, Jasmine F, Argos M, Roy S, et al. Genome-wide association study identifies chromosome 10q24.32 variants associated with arsenic metabolism and toxicity phenotypes in Bangladesh. PLoS Genet. (2012) 8:e1002522. doi: 10.1158/1538-7445.AM2012-2925
72. Beebe-Dimmer JL, Iyer PT, Nriagu JO, Keele GR, Mehta S, Meliker JR, et al. Genetic variation in glutathione S-transferase omega-1, arsenic methyltransferase and methylene-tetrahydrofolate reductase, arsenic exposure and bladder cancer: a case-control study. Environ Health. (2012) 11:43. doi: 10.1186/1476-069X-11-43
73. Hernández A, Xamena N, Surrallés J, Sekaran C, Tokunaga H, Quinteros D, et al. Role of the Met (287) Thr polymorphism in the AS3MT gene on the metabolic arsenic profile. Mutat Res. (2008) 637:80–92. doi: 10.1016/j.mrfmmm.2007.07.004
74. Chung CJ, Pu YS, Su CT, Chen HW, Huang YK, Shiue HS, et al. Polymorphisms in one-carbon metabolism pathway genes, urinary arsenic profile, and urothelial carcinoma. Cancer Causes Control. (2010) 21:1605–13. doi: 10.1007/s10552-010-9589-3
75. Steinmaus C, Moore LE, Shipp M, Kalman D, Rey OA, Biggs ML, et al. Genetic polymorphisms in MTHFR 677 and 1298, GSTM1 and T1, and metabolism of arsenic. J Toxicol Environ Health A. (2007) 70:159–70. doi: 10.1080/15287390600755240
76. Chen Y, Parvez F, Gamble M, Islam T, Ahmed A, Argos M, et al. Arsenic exposure at low-to-moderate levels and skin lesions, arsenic metabolism, neurological functions, and biomarkers for respiratory and cardiovascular diseases: review of recent findings from the Health Effects of Arsenic Longitudinal Study (HEALS) in Bangladesh. Toxicol Appl Pharmacol. (2009) 239:184–92. doi: 10.1016/j.taap.2009.01.010
77. De Chaudhuri S, Ghosh P, Sarma N, Majumdar P, Sau TJ, Basu S, et al. Genetic variants associated with arsenic susceptibility: study of purine nucleoside phosphorylase, arsenic (+3) methyltransferase, and glutathione S-transferase omega genes. Environ Health Perspect. (2008) 116:501–5. doi: 10.1289/ehp.10581
78. Porter KE, Basu A, Hubbard AE, Bates MN, Kalman D, Rey O, et al. Association of genetic variation in cystathionine-β-synthase and arsenic metabolism. Environ Res. (2011) 110:580–7. doi: 10.1016/j.envres.2010.05.001
79. Lindberg AL, Kumar R, Goessler W, Thirumaran R, Gurzau E, Koppova K, et al. Metabolism of low-dose inorganic arsenic in a central European population: influence of sex and genetic polymorphisms. Environ Health Perspect. (2007) 115:1081–6. doi: 10.1289/ehp.10026
80. Meza MM, Yu L, Rodriguez YY, Guild M, Thompson D, Gandolfi AJ, et al. Developmentally restricted genetic determinants of human arsenic metabolism: Association between urinary methylated arsenic and CYT19 polymorphisms in children. Environ Health Perspect. (2005) 113:775–81. doi: 10.1289/ehp.7780
81. Luo L, Li Y, Gao Y, Zhao L, Feng H, Wei W, et al. Association between arsenic metabolism gene polymorphisms and arsenic-induced skin lesions in individuals exposed to high-dose inorganic arsenic in northwest China. Sci Rep. (2018) 8:6578. doi: 10.1038/s41598-017-18925-3
82. McCarty KM, Ryan L, Houseman EA, Williams PL, Miller DP, Quamruzzaman Q, et al. A case-control study of GST polymorphisms and arsenic related skin lesions. Environ Health. (2007) 6:1–10. doi: 10.1186/1476-069X-6-5
83. Lin GF, Du H, Chen JG, Lu HC, Guo WC, Meng H, et al. Arsenic-related skin lesions and glutathione S-transferase P1 A1578G (Ile105Val) polymorphism in two ethnic clans exposed to indoor combustion of high arsenic coal in one village. Pharmacog Genomics. (2006) 16:863–71. doi: 10.1097/01.fpc.0000230415.82349.4b
84. Kaya-Akyüzlü D, Kayaaltl Z, Söylemezoğlu T. Influence of MRP1 G1666A and GSTP1 Ile105Val genetic variants on the urinary and blood arsenic levels of Turkish smelter workers. Environ. Toxicol. Pharmacol. (2016) 43:68–73. doi: 10.1016/j.etap.2016.02.013
85. Borghini A, Faita F, Mercuri A, Minichilli F, Bustaffa E, Bianchi F, Andreassi MG. Arsenic exposure, genetic susceptibility and leukocyte telomere length in an Italian young adult population. Mutagenesis. (2016) 31:539–46. doi: 10.1093/mutage/gew017
86. Smeester L, Rager JE, Bailey KA, Guan X, Smith N, García-Vargas G, et al. Epigenetic changes in individuals with arsenicosis. Chem Res Toxicol. (2011) 24:165–7. doi: 10.1021/tx1004419
87. Seow WJ, Kile ML, Baccarelli AA, Pan WC, Byun HM, Mostofa G, et al. Epigenome-wide DNA methylation changes with development of arsenic-induced skin lesions in Bangladesh: a case-control follow-up study. Environ Mol Mutagen. (2014) 55:449–56. doi: 10.1002/em.21860
88. Majumder M, Dasgupta UB, Guha Mazumder DN, Das N. Skin score correlates with global DNA methylation and GSTO1 A140D polymorphism in arsenic-affected population of Eastern India. Toxicol Mech Methods. (2017) 27:467–75. doi: 10.1080/15376516.2017.1323255
89. Pilsner RJ, Liu X, Ahsan H, Ilievski V, Slavkovich V, Levy D, et al. Genomic methylation of peripheral blood leukocyte DNA: influences of arsenic and folate in Bangladeshi adults. Am J Clin Nutr. (2007) 86:1179–86. doi: 10.1093/ajcn/86.4.1179
90. Chanda S, Dasgupta UB, Mazumder DG, Saha J, Gupta B. Human GMDS gene fragment hypermethylation in chronic high level of arsenic exposure with and without arsenic induced cancer. SpringerPlus. (2013) 2:1–12. doi: 10.1186/2193-1801-2-557
91. Gribble MO, Tang WY, Shang Y, Pollak J, Umans JG, Francesconi KA, et al. Differential methylation of the arsenic (III) methyltransferase promoter according to arsenic exposure. Arch Toxicol. (2013) 88:275–82. doi: 10.1007/s00204-013-1146-x
92. Janasik B, Reszka E, Stanislawska M, Jablonska E, Kuras R, Wieczorek E, et al. Effect of arsenic exposure on NRF2-KEAP1 pathway and epigenetic modification. Biol Trace Element Res. (2018) 185: 11–19. doi: 10.1007/s12011-017-1219-4
93. Sanyal T, Bhattacharjee P, Bhattacharjee S, Bhattacharjee P. Hypomethylation of mitochondrial D-loop and ND6 with increased mitochondrial DNA copy number in the arsenic-exposed population. Toxicology. (2018) 408:54–61. doi: 10.1016/j.tox.2018.06.012
94. Sanyal T, Paul M, Bhattacharjee S, Bhattacharjee P. Epigenetic alteration of mitochondrial biogenesis regulatory genes in arsenic exposed individuals (with and without skin lesions) and in skin cancer tissues: a case control study. Chemosphere. (2020) 258:127305. doi: 10.1016/j.chemosphere.2020.127305
95. Chanda S, Dasgupta UB, GuhaMazumder D, Gupta M, Chaudhuri U, Lahiri S, et al. DNA hypermethylation of promoter of gene p53 and p16 in arsenic-exposed people with and without malignancy. Toxicol Sci. (2006) 89:431–7. doi: 10.1093/toxsci/kfj030
96. Banerjee N, Paul S, Sau TJ, Das JK, Bandyopadhyay A, Banerjee S, et al. Epigenetic modifications of DAPK and p16 genes contribute to arsenic-induced skin lesions and nondermatological health effects. Toxicol Sci. (2013) 135:300–8. doi: 10.1093/toxsci/kft163
97. Paul S, Banerjee N, Chatterjee A, Sau TJ, Das JK, Mishra PK, et al. Arsenic-induced promoter hypomethylation and over-expression of ERCC2 reduces DNA repair capacity in humans by non-disjunction of the ERCC2-Cdk7 complex. Metallomics. (2014) 6:864–73. doi: 10.1039/c3mt00328k
98. Zhang J, Wang B, Li Z, Yan J, Yu Y, Guo Y, et al. A perspective of chronic low exposure of arsenic on non-working women: Risk of hypertension. Sci Total Environ. (2016) 580:69–73. doi: 10.1016/j.scitotenv.2016.11.204
99. Liao WT, You HL, Chai CY, Lee CH, Lan CCE, Chang SJ, et al. Cyclin D1 promoter−56 and−54 bp CpG un-methylation predicts invasive progression in arsenic-induced Bowen's disease. J Dermatol Sci. (2018) 89:191–7. doi: 10.1016/j.jdermsci.2017.10.003
100. Guo Z, Hu Q, Tian J, Yan L, Jing C, Xie HQ, et al. Proteomic profiling reveals candidate markers for arsenic-induced skin keratosis. Environ Pollut. (2016) 218:34–8. doi: 10.1016/j.envpol.2016.08.035
101. Argos M, Kibriya MG, Parvez F, Jasmine F, Rakibuz-Zaman M, Ahsan H. Gene expression profiles in peripheral lymphocytes by arsenic exposure and skin lesion status in a Bangladeshi population. Cancer Epidemiol Biomarkers Prev. (2006) 15:1367–75. doi: 10.1158/1055-9965.EPI-06-0106
102. Kibriya MG, Jasmine F, Parvez F, Argos M, Roy S, Paul-Brutus R, et al. Association between genome-wide copy number variation and arsenic-induced skin lesions: a prospective study. Environ Health. (2017) 16:1–13. doi: 10.1186/s12940-017-0283-8
103. Mir SA, Pinto SM, Paul S, Raja R, Nanjappa V, Syed N, et al. SILAC-based quantitative proteomic analysis reveals widespread molecular alterations in human skin keratinocytes upon chronic arsenic exposure. Proteomics. (2017) 17:1600257. doi: 10.1002/pmic.201600257
104. Al-Eryani L, Jenkins SF, States VA, Pan J, Malone JC, Rai SN, et al. miRNA expression profiles of premalignant and malignant arsenic-induced skin lesions. PLoS ONE. (2018) 13:e0202579. doi: 10.1371/journal.pone.0202579
105. Banerjee M, Sarkar J, Das JK, Mukherjee A, Sarkar AK, Mondal L, et al. Polymorphism in the ERCC2 codon 751 is associated with arsenic-induced premalignant hyperkeratosis and significant chromosome aberrations. Carcinogenesis. (2007) 28:672–6. doi: 10.1093/carcin/bgl181
106. Gonzalez H, Lema C, Kirken RA, Maldonado RA, Varela-Ramirez A, Renato J, et al. Arsenic-exposed keratinocytes exhibit differential microRNAs expression profile; potential implication of miR-21, miR-200a and miR-141 in melanoma pathway Horacio. Clin Cancer Drugs. (2015) 2:138–47. doi: 10.2174/2212697X02666150629174704
107. Howe CG, Gamble MV. Influence of arsenic on global levels of histone posttranslational modifications: a review of the literature and challenges in the field. Curr Environ Health Rep. (2016) 3:225–37. doi: 10.1007/s40572-016-0104-1
108. Ma L, Li J, Zhan Z, Chen L, Li D, Bai Q, et al. Specific histone modification responds to arsenic-induced oxidative stress. Toxicol Appl Pharmacol. (2016) 302:52–61. doi: 10.1016/j.taap.2016.03.015
109. Liu D, Wu D, Zhao L, Yang Y, Ding J, Dong L, et al. Arsenic trioxide reduces global histone H4 acetylation at lysine 16 through direct binding to histone acetyltransferase hMOF in human cells. PLoS ONE. (2015) 10:e0141014. doi: 10.1371/journal.pone.0141014
110. Suzuki T, Nohara K. Long-term arsenic exposure induces histone H3 Lys9 dimethylation without altering DNA methylation in the promoter region of p16INK4a and down-regulates its expression in the liver of mice. J Appl Toxicol. (2013) 33:951–8. doi: 10.1002/jat.2765
111. Tauheed J, Sanchez-Guerra M, Lee JJ, Paul L, Ibne Hasan MOS, Quamruzzaman Q, et al. Associations between post translational histone modifications, myelomeningocele risk, environmental arsenic exposure, and folate deficiency among participants in a case control study in Bangladesh. Epigenetics. (2017) 12:484–91. doi: 10.1080/15592294.2017.1312238
112. Cantone L, Nordio F, Hou L, Apostoli P, Bonzini M, Tarantini L, et al. Inhalable metal-rich air particles and histone H3K4 dimethylation and H3K9 acetylation in a cross-sectional study of steel workers. Environ Health Perspect. (2011) 119:964–9. doi: 10.1289/ehp.1002955
113. Howe CG, Liu X, Hall MN, Slavkovich V, Ilievski V, Parvez F, et al. Associations between blood and urine arsenic concentrations and global levels of post-translational histone modifications in Bangladeshi men and women. Environ. Health Persp. (2016) 124:1234–40. doi: 10.1289/ehp.1510412
114. Chervona Y, Hall MN, Arita A, Wu F, Sun H, Tseng HC, et al. Associations between arsenic exposure and global posttranslational histone modifications among adults in Bangladesh. Cancer Epidemiol Biomark Prevent. (2012) 21:2252–60. doi: 10.1158/1055-9965.EPI-12-0833
115. Pournara A, Kippler M, Holmlund T, Ceder R, Grafström R, Vahter M, et al. Arsenic alters global histone modifications in lymphocytes in vitro and in vivo. Cell Biol Toxicol. (2016) 32:275–84. doi: 10.1007/s10565-016-9334-0
116. Bhattacharjee P, Paul S, Bhattacharjee S, Giri AK, Bhattacharjee P. Association of H3K79 monomethylation (an epigenetic signature) with arsenic-induced. Mutat Res. (2018) 807:1–9. doi: 10.1016/j.mrfmmm.2017.11.001
117. Sohn E. Simple cooking methods flush arsenic out of rice, Preparing rice in a coffee machine can halve levels of the naturally occurring substance. Nature News. (2015). Available online at: https://www.nature.com/news/simple-cooking-methods-flush-arsenic-out-of-rice-1.18034 (accessed February 20, 2019).
118. Spanu A, Daga L, Orlandoni AM, Sanna G. The role of irrigation techniques in arsenic bioaccumulation in rice (Oryza sativa L.). Environ Sci Technol. (2012) 46:8333–40. doi: 10.1021/es300636d
119. Xu XY, McGrath SP, Meharg AA, Zhao FJ. Growing rice aerobically markedly decreases arsenic accumulation. Environ Sci Technol. (2008) 42:5574–9. doi: 10.1021/es800324u
120. Moreno-Jiménez E, Meharg AA, Smolders E, Manzano R, Becerra D, Sánchez-Llerena J, et al. Sprinkler irrigation of rice fields reduces grain arsenic but enhances cadmium. Sci Total Environ. (2014) 485:468–73. doi: 10.1016/j.scitotenv.2014.03.106
121. Signes A, Mitra K, Burlo F, Carbonell-Barrachina AA. Effect of cooking method and rice type on arsenic concentration in cooked rice and the estimation of arsenic dietary intake in a rural village in West Bengal, India. Food AdditContam Part A. (2008) 25:1345–52. doi: 10.1080/02652030802189732
122. Chen Y, Han YH, Cao Y, Zhu YG, Rathinasabapathi B, Ma LQ. Arsenic transport in rice and biological solutions to reduce arsenic risk from rice. Front Plant Sci. (2017) 8:268. doi: 10.3389/fpls.2017.00268
123. Banerjee M, Banerjee N, Bhattacharjee P, Mondal D, Lythgoe PR, Martinez M, et al. High arsenic in rice is associated with elevated genotoxic effects in humans. Sci Rep. (2013) 3:2195. doi: 10.1038/srep02195
124. Farrow EM, Wang J, Burken JG, Shi H, Yan W, Yang J, et al. Reducing arsenic accumulation in rice grain through iron oxide amendment. Ecotoxicol Environ Saf . (2015) 118:55–61. doi: 10.1016/j.ecoenv.2015.04.014
125. Norton GJ, Nigar M, Williams PN, Dasgupta T, Meharg AA, Price AH. Rice-arsenate interactions in hydroponics: a three-gene model for tolerance. J Exp Bot. (2008) 59:2277–84. doi: 10.1093/jxb/ern098
126. Ramirez T, Garcia-Montalvo V, Wise C, Cea-Olivares R, Poirier LA, Herrera LA. S-adenosyl-L-methionine is able to reverse micronucleus formation induced by sodium arsenite and other cytoskeleton disrupting agents in cultured human cells. Mutat Res. (2003) 528:61–74. doi: 10.1016/S0027-5107(03)00099-X
127. Oremland RS, Stolz JF. Arsenic, microbes and contaminated aquifers. Trends Microbiol. (2005) 13:45–9. doi: 10.1016/j.tim.2004.12.002
128. Cressey D. ‘Arsenic-life' bacterium prefers phosphorus after all, transport proteins show 4,000-fold preference for phosphate over arsenate. Nat. News. (2012). doi: 10.1038/nature.2012.11520
129. Dey U, Chatterjee S, Mondal NK. Isolation and characterization of arsenic-resistant bacteria and possible application in bioremediation. Biotechnol Rep. (2016) 10:1–7. doi: 10.1016/j.btre.2016.02.002
130. Bhattacharya S. Medicinal plants and natural products in amelioration of arsenic toxicity: a short review. Pharm Biol. (2017) 55:349–54. doi: 10.1080/13880209.2016.1235207
131. Ling S, Shan Q, Liu P, Feng T, Zhang X, Xiang P, et al. Metformin ameliorates arsenic trioxide hepatotoxicity via inhibiting mitochondrial complex I. Cell Death Dis. (2017) 8: e3159. doi: 10.1038/cddis.2017.482
132. Singh S, Rana SV. Amelioration of arsenic toxicity by L-Ascorbic acid in laboratory rat. J Environ Biol. (2007) 28:377–84.
133. Merry TL, Ristow M. Nuclear factor erythroid-derived 2-like 2 (NFE2L2, Nrf2) mediates exercise-induced mitochondrial biogenesis and the anti-oxidant response in mice. J Physiol. (2016) 594:5195–207. doi: 10.1113/JP271957
134. Ndisang JF. Synergistic interaction between heme oxygenase (HO) and nuclear-factor E2- related factor-2 (Nrf2) against oxidative stress in cardiovascular related diseases. Curr Pharm Des. (2017) 23:1465–70. doi: 10.2174/1381612823666170113153818
135. Srivastava R, Sengupta A, Mukherjee S, Chatterjee S, Sudarshan M, Chakraborty A, et al. In vivo effect of arsenic trioxide on Keap1-p62-Nrf2 signalling pathway in mouse liver: expression of antioxidant responsive element-driven genes related to glutathione metabolism. ISRN Hepatol. (2013) 2013:817693. doi: 10.1155/2013/817693
136. Han X, Zhang J, Xue X, Zhao Y, Lu L, Cui M, et al. Theaflavin ameliorates ionizing radiation-induced hematopoietic injury via the NRF2 pathway. Free RadicBiol Med. (2017) 113:59–70. doi: 10.1016/j.freeradbiomed.2017.09.014
137. Shibu MA, Kuo CH, Chen BC, Ju DT, Chen RJ, Lai CH, et al. Oolong tea prevents cardiomyocyte loss against hypoxia by attenuating p-JNK mediated hypertrophy and enhancing P-IGF1R, p-akt, and p-Bad(ser136) activity and by fortifying NRF2 antioxidation system. Environ Toxicol. (2018) 33:220–33. doi: 10.1002/tox.22510
138. Ye T, Zhen J, Du Y, Zhou JK, Peng A, Vaziri ND, et al. Green tea polyphenol (-)-epigallocatechin-3-gallate restores Nrf2 activity and ameliorates crescentic glomerulonephritis. PLoS ONE. (2015) 10:e0119543. doi: 10.1371/journal.pone.0119543
139. Ramirez T, Stopper H, Hock R, Herrera LA. Prevention of aneuploidy by S-adenosyl-methionine in human cells treated with sodium arsenite. Mutat Res. (2007) 617:16–22. doi: 10.1016/j.mrfmmm.2006.10.005
140. Seow WJ, Pan WC, Kile ML, Lin T, Baccarelli A, Quamruzzaman Q, et al. A distinct and replicable squamous cell carcinoma gene INPPA5 variant modifies susceptibility of arsenic-associated skin lesions in Bangladesh. Cancer. (2015) 121:2222. doi: 10.1002/cncr.29291
141. Yang SM, Huang CY, Shiue HS, Pu YS, Hsieh YH, Chen WJ, et al. Combined effects of DNA methyltransferase 1 and 3A polymorphisms and urinary total arsenic levels on the risk for clear cell renal cell carcinoma. Toxicol Appl Pharmacol. (2016) 305:103–10. doi: 10.1016/j.taap.2016.06.011
142. Hsueh YM, Chen WJ, Lin YC, Huang CY, Shiue HS, Yang SM, et al. Adiponectin gene polymorphisms and obesity increase the susceptibility to arsenic-related renal cell carcinoma. Toxicol Appl Pharmacol. (2018) 350:11–20. doi: 10.1016/j.taap.2018.04.034
143. Mondal R, Biswas S, Chatterjee A, Mishra R, Mukhopadhyay A, Bhadra RK, et al. Protection against arsenic-induced hematological and hepatic anomalies by supplementation of vitamin C and vitamin E in adult male rats. J Basic Clin Physiol Pharmacol. (2016) 27:643–52. doi: 10.1515/jbcpp-2016-0020
Keywords: arsenic toxicity, differential susceptibility, arsenic methylation, single nucleotide polymorphism, epigenetic pattern, sustainable remediation
Citation: Sanyal T, Bhattacharjee P, Paul S and Bhattacharjee P (2020) Recent Advances in Arsenic Research: Significance of Differential Susceptibility and Sustainable Strategies for Mitigation. Front. Public Health 8:464. doi: 10.3389/fpubh.2020.00464
Received: 20 April 2020; Accepted: 23 July 2020;
Published: 08 October 2020.
Edited by:
Radu Corneliu Duca, Laboratoire National de Santé (LNS), LuxembourgReviewed by:
Wojciech Wasowicz, Nofer Institute of Occupational Medicine, PolandCopyright © 2020 Sanyal, Bhattacharjee, Paul and Bhattacharjee. This is an open-access article distributed under the terms of the Creative Commons Attribution License (CC BY). The use, distribution or reproduction in other forums is permitted, provided the original author(s) and the copyright owner(s) are credited and that the original publication in this journal is cited, in accordance with accepted academic practice. No use, distribution or reproduction is permitted which does not comply with these terms.
*Correspondence: Pritha Bhattacharjee, Nzc3LnByaXRoYUBnbWFpbC5jb20=
Disclaimer: All claims expressed in this article are solely those of the authors and do not necessarily represent those of their affiliated organizations, or those of the publisher, the editors and the reviewers. Any product that may be evaluated in this article or claim that may be made by its manufacturer is not guaranteed or endorsed by the publisher.
Research integrity at Frontiers
Learn more about the work of our research integrity team to safeguard the quality of each article we publish.