- 1College of Pharmacy, Guizhou University, Guiyang, China
- 2State Key Laboratory for Infectious Disease Prevention and Control, Chinese Centre for Disease Control and Prevention, National Institute for Communicable Disease Control and Prevention, Beijing, China
- 3Guangdong Key Laboratory for Diagnosis and Treatment of Emerging Infectious Diseases, Shenzhen Third People's Hospital, Southern University of Science and Technology, Shenzhen, China
Background: China is a high-burden country of tuberculosis. The proportion of diseases caused by non-tuberculous mycobacteria (NTM) has increased, seriously affecting the prevention, control, and management of tuberculosis (TB) and posing a significant threat to human health. However, there is a lack of an organized monitoring system for NTM such as that used for tuberculosis. Comprehensive data on patient susceptibility, dominant species, and drug resistance profiles are needed to improve the treatment protocols and the management of NTM.
Methods: Primary research reports of NTM clinical specimens from mainland China published between January 1, 2000 and May 31, 2019 were retrieved from four online resources (BIOSIS, Embase, PubMed, and Web of Science) and three Chinese medical literature databases (CNKI, Wanfang, and Vip) as the Preferred Reporting Items for Systematic Reviews and Meta-Analyses.
Results: In total, 339 publications were included in the systematic review, 129 were used in the drug susceptibility analysis, and 95 were used in the meta-analysis. Traditional culture using Lowenstein–Jensen slants combined with P-nitrobenzene acid and thiophene-2-carboxylic acid hydrazine differential medium and proportional method was most commonly used for the isolation, identification, and drug susceptibility testing of NTM in China. The crude isolation rate for NTM among TB suspected cases was 4.66–5.78%, while the proportion of NTM among Mycobacterium isolates was 11.57%. Mycobacterium abscessus and Mycobacterium avium complex were the most common clinical NTM species. NTM only showed general sensitivity to ethambutol, linezolid, clofazimine, amikacin, tobramycin, and clarithromycin.
Conclusions: The prevalence of NTM in China has shown a decreasing trend. M. abscessus was replaced as the dominant species by Mycobacterium intracellulare over the course of the study. The geographic diversity of different species showed the effects of environmental and economic factors on the distribution of NTM and indicated that there were important factors still not identified. While there were only a limited number of antibiotics to which NTM showed any sensitivity, the drug resistance profiles of the isolates were highly variable and thus more caution should be taken when empirically treating NTM infection.
Introduction
Non-tuberculous mycobacteria (NTM) species spread among five genera (Mycobacterium, Mycobacteroides, Mycolicibacillus, Mycolicibacter, and Mycolicibacterium) of the family Mycobacteriaceae (1). To date, more than 250 taxa (species and subspecies) have been identified as NTM and are ubiquitously distributed throughout aquatic and terrestrial environments. Although most NTM species are non-pathogenic and rarely observed in clinical samples, up to 60 NTM species have repeatedly been recovered from clinical specimens and proved to cause human diseases (2, 3).
The incidence and the disease burden of NTM infection have increased significantly worldwide as a result of changes in demographics, advances in radiological diagnosis of pulmonary abnormalities and identification techniques of Mycobacterium species, and the control and decline of tuberculosis (TB). The prevalence of NTM even exceeded Mycobacterium tuberculosis, the causative agent of TB, in the United States (4). Moreover, among suspected multidrug-resistant TB patients who did not respond to 2–3 months of treatment with first-line anti-TB drugs, about 30% were actually NTM infection (5), with the actual NTM infection rates likely to be higher. Patients with impaired immunity as a result of malignancies, organ transplantation, and HIV infection, those with chronic pulmonary diseases, and the elderly are at a higher risk of NTM infection (4, 6, 7). NTM co-infection with HIV and M. tuberculosis has negatively impact on TB management.
Because NTM infection is not a legally required notifiable disease, coupled with the cumbersome operation of NTM identification methods, there is not such a sophisticated and organized TB monitoring system for NTM in China, the same as is the case in Europe and most other countries (8). An epidemiological analysis of NTM depends mainly on systematic review and meta-analysis. Because NTM are a large heterogeneous group of microorganisms, patient susceptibility, dominant species, and drug sensitivity, including anti-tuberculosis drugs, vary greatly within species. However, such information has significant reference value for the treatment and the management of these infections, especially in low- and middle-income countries without sufficient resources or laboratory equipment to conduct the clinical tests. Thus, in the current study, we systematically reviewed and analyzed reports on NTM from mainland China, published within the last 20 years, to explore the epidemic characteristics and trends in NTM prevalence, distribution, and drug resistance in China.
Materials and Methods
Search Strategy and Inclusion and Exclusion Criteria
The study was carried out according to the Preferred Reporting Items for Systematic Reviews and Meta-Analyses. All studies involving NTM clinical specimens from mainland China and published between January 1, 2000 and May 31, 2019 were retrieved from four online resources (BIOSIS, Embase, PubMed, and Web of Science) and three Chinese medical literature databases (CNKI, Wanfang, and Vip). The screening terms included the combination of “China/Chinese” and medical subheadings (MeSH) or key words (NTM, atypical mycobacterium, atypical mycobacterial infection, non-tuberculous, non-tuberculous mycobacterium, non-tuberculosis, non-tuberculous, as well as the names of the 249 NTM species and subspecies reported to date). Additional studies were added by retrospective searches.
Only original articles were included in the analysis. The inclusion criteria required that the studies clearly described the NTM isolation and identification methods used. The samples should be from patients in mainland China, with a definite sample size and number of NTM isolated. For reports on drug sensitivity, standardized methods of drug sensitivity testing (DST) should be used and breakpoints for drug resistance determination should be noted. Among duplicate studies from the same source conducted at the same location and time, only the study with the largest sample size was included. For studies using NTM isolates collected from imported patients, the environment or animal was excluded. Review articles and conference abstracts without the full text were also excluded.
Data Extraction and Management
The following data were extracted from the included full-text publications: sample source, patient characteristics, collection time, location, methods of identification/culture and DST, number of cases and strains isolated (NTM and total mycobacteria, if applicable), NTM species, breakpoints for drug resistance determination, drug resistance rates, authors, and affiliations. Two researchers independently performed the literature search and data entry. Inconsistencies were rechecked to obtain consensus.
Data Analysis
Stata 14 (9) and NoteExpress 3.0 (AEGEAN, Beijing, China) were used for data management and meta-analysis. Heterogeneity was examined using the I2 statistic. Funnel plots were used to quantitatively assess publication bias. The pooled prevalence of NTM and each species with corresponding 95% confidence intervals (CIs) was calculated using forest plots in a random-effect model. Stratified statistical analyses were subsequently performed with respect to species, isolation time, and geographic area.
Results
Screening Results and Characteristics of the Included Studies
A total of 8,137 publications were obtained by database search using the combination terms set for this study. After eliminating duplicates and irrelevant studies based on the title and abstract evaluations, 2,058 publications were carried forward for full-text evaluation. As a result, 1,719 papers were further excluded, leaving 339 papers for inclusion in the systematic review, including 12 in English and 327 in Chinese (Figure 1).
The publications used for the analyses included 55 case reports, 141 studies with <50 isolates, 70 with between 50 and 100 isolates, and 73 with >100 isolates. Geographically, the included studies covered 29 of the 31 provinces of mainland China. In total, 296 publications were reports from a single hospital, 43 covered multiple hospitals/centers, and 19 included samples from multiple provinces. Among the papers, 136 described single-year studies and 203 were multi-year studies, of which 36 contained data from >5 years. In addition, 67 publications involved only one NTM species, while 170 publications included species composition information. Of the 339 papers, 139 publications also included DST data.
NTM Culture and Identification Methods
Traditional culture on Lowenstein–Jensen (L–J) slants combined with P-nitrobenzene acid and thiophene-2-carboxylic acid hydrazine differential medium was the most commonly used isolation and identification method for NTM over the 20-year study period in mainland China (Supplementary Tables 1, 2). The use of commercial BACTEC™ medium (MGIT™ 960, 460, 9120, and FX40) and MacC agar increased in 2010, with use of the latter medium almost surpassing that of the L–J slants for NTM culture over the past 5 years. Molecular detection methods, particularly polymerase chain reaction (PCR)-based analysis and sequencing of conserved genes, have been applied to NTM species identification since 2005. Among 87 studies (9,628 NTM strains) using the PCR-sequencing identification method, 57 (7,284 NTM strains) conducted multi-locus analysis and 30 (2,344 NTM strains) used single-gene sequencing. Among molecular studies, 16S rRNA, hsp65, and rpoB were the three most commonly used target genes.
NTM Prevalence and Species Recovered From Clinical Specimens
Over the 20-year period from 2000 to 2019, 13,653 NTM isolates were recovered from 293,011 clinical suspected TB samples (patients with TB signs or symptoms, including pulmonary and extrapulmonary TB) in mainland China. In these publications, 58 papers also reported the total isolations of mycobacteria, which was 8,973 NTM among 77,964 mycobacteria from 219,829 samples. Thus, the crude isolation rate for NTM of TB suspects was 4.66%, with the proportion of NTM among Mycobacterium isolates at 11.57% in China. Excluding case reports, there was significant heterogeneity among the studies included in the meta-analysis (X2 = 17,579.19, I2 = 99.5%, P < 0.001). After excluding the studies with <100 NTM isolates, the isolation rate for NTM increased to nearly 5.78%. However, even if only studies with >200 NTM isolates were included, the heterogeneity remained statistically significant. Therefore, a random-effect model was used for the meta-analysis (Table 1 and Figure 2). In addition, stratified statistical analyses were conducted. From 2000 to 2019, the 5-year isolation rates for NTM in TB suspects were 3.01 (2.67–3.36), 6.71 (6.22–7.20), 3.01 (2.84–3.17), and 7.91% (7.32–8.49%), respectively. The proportion of NTM among the Mycobacterium isolates were 8.15, 22.03, 8.08, and 18.20%, respectively, but there was no significant difference between the two highest proportions (X2 = 5.97, P > 0.001). In the three economic zones of China, coastal, central, and western, the separation rate of NTM was higher in coastal areas than in inland areas, which showed a statistical geographic difference.
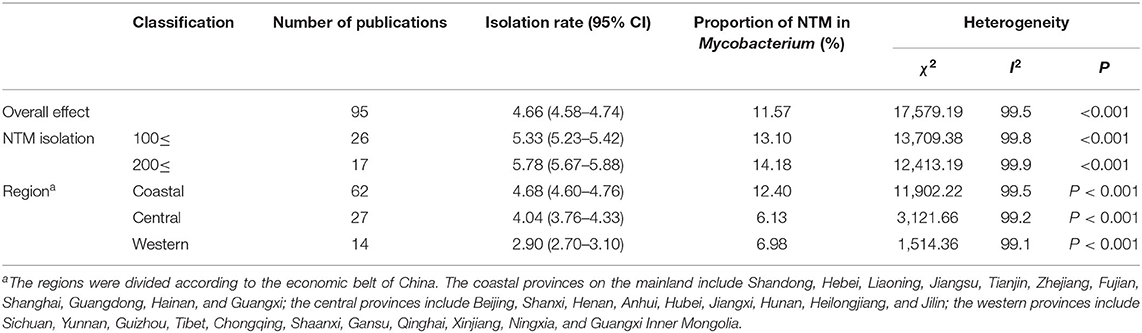
Table 1. Non-tuberculous mycobacteria (NTM) isolation rates in mainland China and heterogeneity among studies included in the meta-analysis.
Overall, 26,407 NTM isolates from 237 studies were identified as belonging to 64 NTM species. Twenty-eight species had <10 isolates, 16 species had 10–50 isolates, five species had 50–100 isolates, and 15 species had >100 isolates. Mycobacterium abscessus and species belonging to the Mycobacterium avium complex (MAC) were always among the top three most prevalent species in the 20-year period (Figure 3 and Supplementary Table 3). At the species level, M. abscessus was the most prevalent NTM species in clinical samples from China prior to 2015. After 2015, even though the isolates decreased compared with the previous 5 years, M. intracellulare became the most prevalent NTM species after a significant decrease in the prevalence of M. abscessus. If analyzed at the species group (M. abscessus/chelonae and MAC) level, MAC were the dominant NTM species in China across the 20-year study period. In addition, the prevalence of Mycobacterium chelonae decreased, while that of Mycobacterium kansasii significantly increased. The largest numbers of NTM isolates were recovered from Guangdong, Zhejiang, Shanghai, Beijing, and Jiangsu provinces (Figure 3 and Supplementary Table 3). Interestingly, the proportions of the 10 most dominant NTM species differed among these provinces, with differences even observed among the three neighboring provinces, Zhejiang, Jiangsu, and Shanghai.
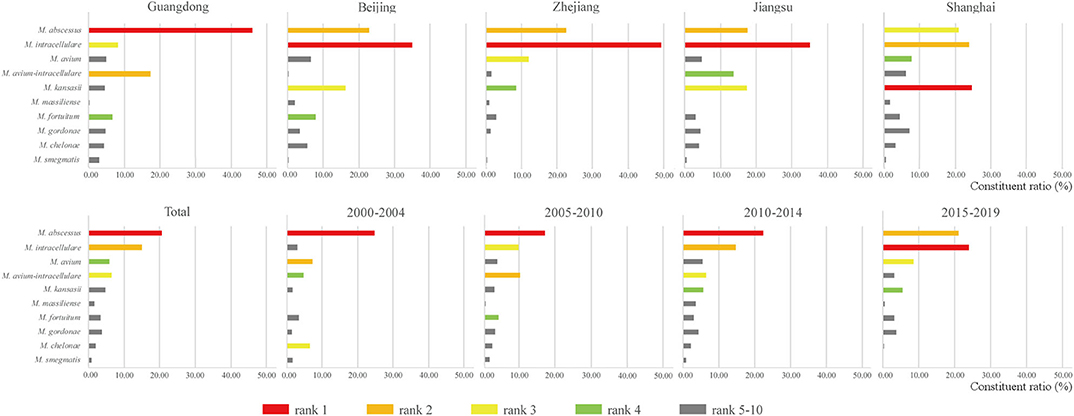
Figure 3. Most common clinical non-tuberculous mycobacteria (NTM) species in the five provinces with the highest NTM isolation and the four 5-year periods.
Pre-existing Conditions and Clinical Symptoms of NTM Patients
A total of 70 papers and 1,689 patients were included in the analysis of the pre-existing conditions of NTM infections. The most common pre-existing conditions were HIV infection (41.33%), bronchiectasis (16.87%), previous pulmonary tuberculosis (15.87%), chronic obstructive pulmonary disease (11.37%), diabetes (5.33%), pleurisy (3.14%), hypoproteinemia (2.66%), anemia (1.84%), and hypertension (1.60%). The main risk factors for NTM in China were different from those reported in Europe (chronic obstructive pulmonary disease, bronchiectasis, and asthma) and in the United States (fibrocavitary disease and consolidating infiltrates) (10).
From 449 HIV co-infection patients, a total of 26 NTM species were recovered. In addition, four co-infection cases were positive for two different NTM species. NTM can cause bacteremia as well as skin/soft tissue, bone, joint, and eye infections, with different NTM species more commonly associated with different clinical manifestations. Only one species, M. abscessus, was reported in all reports of eye infection (Table 2).
DST Methods and Antibiotic Resistance of Chinese NTM Isolates
Overall, six DST methods were used in the included studies. The numbers of publications and NTM strains tested using each method are summarized by time period in Table 3, and the breakpoints used are summarized in Supplementary Table 4. The results showed that, since 2010, an increasing number of studies have assessed the antibiotic sensitivity of NTM. The gold standard DST method for mycobacterium, proportional method, and the traditional absolute concentration method were the most common testing methods used among these studies. However, the microporous plate method, which is easier to conduct and can obtain minimum inhibitory concentration values, has been used more frequently since 2015.
Although NTM are generally resistant to first-line anti-TB drugs, they are usually included in NTM DST. In the included publications, 65 NTM species were screened using a total of 41 different drugs. Among the 10 most common clinical NTM species, up to 27 drugs were tested against >50 NTM isolates from a single species across 141 studies. An analysis of antibiotic resistances rates among the 10 most prevalent NTM species (Figure 4 and Supplementary Table 5) showed that there were very few drugs to which the NTM isolates showed a high degree of sensitivity. Among the most common clinical species, M. avium showed the lowest levels of drug sensitivity (26.9%, 7/26, average drug resistance rate <50%), followed by M. abscessus (33.3%, 9/27), M. avium-intracellulare (37.5%, 6/16), M. fortuitum (37.5%, 9/24), M. chelonae (37.5%, 9/24), and M. intracellulare (38.5%, 10/26). Only M. kansasii and Mycobacterium gordonae were sensitive to more than half of the tested drugs, at 52% (13/25) and 66.7% (16/24), respectively. Among the first-line anti-TB drugs, almost all NTM species were highly resistant to isoniazid. MAC, M. kansasii, and M. gordonae were moderately sensitive to rifampicin. Ethambutol was relatively the most sensitive first-line anti-TB drug for NTM. Among other drugs, NTM only showed general sensitivity to linezolid (group A drug), clofazimine (group B drug), amikacin (group C drug), tobramycin, and clarithromycin, which could be used as clinically recommended drugs for the treatment of NTM.
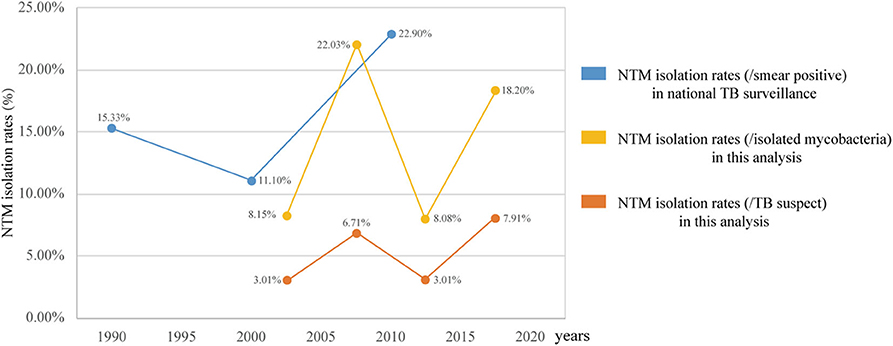
Figure 4. Antibiotic resistance rates of the top 10 clinical non-tuberculous mycobacteria species. The red lines indicate the average rates, while the black dots indicate the actual resistance rates reported in the papers. Inh, isoniazid; Rif, rifampicin; Emb, ethambutol; Lfx, levofloxacin; Mxf, moxifloxacin; Lzd, linezolid; Cfz, clofazimine; Cs, cycloserine; Imp, imipenem; Mpm, meropenem; Am, amikacin; Str, Streptomycin; Pto, protionamide; PAS, aminosalicylic acid; Cm, capreomycin; Km, kanamycin; Tbm, tobramycin; Smz, sulfamethoxazole; Pa, pasinizid; Rap, rifapentine; Rfb, rifabutin; Cfx, cefoxitin; Ofx, ofloxacin; Cip, ciprofloxacin; Gat, gatifloxacin; Azi, azithromycin; Clar, clarithromycin.
Discussion
In 1979, the first Chinese national TB surveillance program reported that NTM accounted for 4.3% (TB, 717/100,000) of smear-positive clinical cultures. This isolation rate increased to 15.35% (TB, 535/100,000) in the third surveillance (1990), 11.1% (TB, 367/100,000) in the fourth surveillance (2000), and 22.9% (TB, 459/100,000) in the fifth surveillance (2010) (11). As shown in Figure 5, the trends in the prevalence of NTM, as determined in the current study between 2000 and 2010, were consistent with the national monitoring. These data indicated that the prevalence of NTM in China is much higher than that in European countries (0.2–2.9/100,000) (2, 12–14) and in the United States (8.7–13.9/100,000). In addition, the proportion of NTM among mycobacterial isolates in China was higher than that reported in India (6.5%, 2000–2012) (15) and in Iran (10.2%, 1999–2014) (16) but lower than that in Northern India (29%, 2013–2015) (17).
Figure 5 also clearly shows that the changes in NTM prevalence in China are not continuous but rather show periodic fluctuation. Two increasing periods were observed after the two significant decline periods of 2000–2004 and 2010–2015. The NTM isolation rates (/TB suspect and /isolated mycobacteria) between the two decline periods have no statistically significant difference, the same as the NTM isolation rates (/isolated mycobacteria) between the two increasing periods. Although the 95% CIs of the two peaks of the NTM isolation rates (/TB suspects), 6.22–7.20 and 7.32–8.49, do not overlap and indicate statistical significance, the difference is only 1.2%. This analysis indicates that the proportions of NTM in TB are relatively constant in both the increasing and the declining periods. However, the estimated TB incidence (all forms) per 100,000 population in China has gradually dropped from 100 to 85, 72, and 63, down by 37.5% over the same time periods (18). Therefore, although the global NTM prevalence rates are increasing, the NTM infections in the populations in China should be a generally decreasing trend. Moreover, greater awareness of NTM and improved imaging and culture techniques can increase the rates of diagnosis, further implicitly increasing NTM prevalence rates. The decrease of NTM prevalence is perhaps even more so than indicated by the data.
NTM are generally environmental bacteria, so it is reasonable that specific environmental factors relating to soil, water, and temperature can affect the distribution of NTM species and the risk of NTM disease, leading to specific geographic characteristics and time variation. Research showed that MAC was the most common species worldwide, accounting for 34–61% of all NTM isolates (19), followed by M. gordonae, M. xenopi, and M. kansasii, and M. fortuitum was the most common rapid mycobacterial grower (20). Regional analyses showed that M. kansasii was the most common species in Slovakia and Poland, M. xenopi in Central, Southern, and Western Europe, M. malmoense in Northern Europe, and M. abscessus complex in North America, Taiwan, Australia, and South Korea (19, 21). At the species group level, MAC was the most prevalent group in China, the same as the rest of the world, although with a less proportion accounting for it (27.46%). However, our results revealed that it is more appropriate to conduct analyses at the species level, which more clearly reflects changes in the prevalence of different species. For example, in China, a shift in the most predominant species from M. abscessus to M. intracellulare occurred after 2015, with certain differences also noted in the NTM drug resistance spectra.
The geographical distribution of NTM has a general pattern of greater abundance near the coast compared with that inland. For example, the incidence rates of NTM-associated pulmonary disease in Europe were 0.4/100,000 and 0.2/100,000 for coastal and continental regions, respectively (2). Our study revealed a similar distribution pattern in China (11), with an incidence rate of 4.68% in coastal regions compared with 2.9% in western inland regions. In addition to differences in environmental factors, the economic level of the coastal economic zone is higher than that of the inland zone. As a result, the laboratory conditions and the testing capacity of labs in coastal areas are higher than those of labs in inland areas, which is an important contributing factor to differences in prevalence in China. Among the five provinces with the greatest NTM prevalence rates, Guangdong and the four other provinces are more than 10° apart in latitude; thus, it is reasonable that they would show differences in dominant species composition compared with the other provinces. Unexpectedly, the NTM species compositions of three adjoining provinces, Jiangsu, Zhejiang, and Shanghai, were not the same, with the middle province, Shanghai, showing the most divergent composition. Therefore, in addition to environmental factors, we predict that there are also undiscovered factors affecting the distribution of NTM.
Aside from the limited number of drugs to which NTM showed sensitivity, our study exposed two problems with NTM drug sensitivity testing. First, the resistance rates of NTM strains varied greatly between studies. For example, while an NTM species may show 100% resistance to a drug in one study, it could then show 100% sensitivity in another study. Among the 210 drug resistance rates reported for the top 10 NTM species, only 20.48% of rates (43/210) showed a range <25%. While the variations in drug resistance rates were the lowest for M. abscessus and M. gordonae, only one third of the reported rates were within a variation range of 25%. Therefore, when drug sensitivity testing results are absent or unavailable, physicians should exercise caution when selecting empirical medication for the treatment of NTM infection. Second, we uncovered a lack of drug resistance data for M. massiliense.
In conclusion, although the NTM culture and identification methods used in mainland China are still traditional, there is increasing focus on NTM. The current study presents a comprehensive 20-year history of NTM in mainland China, which will further our understanding and aid in the prevention of NTM-associated diseases. The analysis presented here is a valuable reference for clinicians and public health policymakers.
Author Contributions
LZ and DX independently performed the literature identification and data entry. HL rechecked the data. LZ carried out the data analysis and prepared the first draft of the article. RW conceived the project, analyzed and interpreted the data, and prepared the figures and the manuscript. ZY and KW revised the manuscript. All the authors had full access to all study data and approved the final version of the manuscript for submission.
Funding
This work was supported by the National Mega-project for Innovative Drugs (grant no. 2019ZX09721001-007-001).
Conflict of Interest
The authors declare that the research was conducted in the absence of any commercial or financial relationships that could be construed as a potential conflict of interest.
Supplementary Material
The Supplementary Material for this article can be found online at: https://www.frontiersin.org/articles/10.3389/fpubh.2020.00295/full#supplementary-material
References
1. Gupta RS, Lo B, Son J. Phylogenomics and comparative genomic studies robustly support division of the genus Mycobacterium into an emended genus Mycobacterium and four novel genera. Int J Syst Evol Microbiol. (2018) 9:68. doi: 10.3389/fmicb.2018.00067
2. Van Der Werf MJ, Kodmon C, Katalinic-Jankovic V, Kummik T, Soini H, Richter E, et al. Inventory study of non-tuberculous mycobacteria in the European Union. BMC Infect Dis. (2014) 14:62. doi: 10.1186/1471-2334-14-62
3. Mortaz E, Moloudizargari M, Varahram M, Movassaghi M, Garssen J, Kazempour Dizagie M, et al. What immunological defects predispose to non-tuberculosis mycobacterial infections? Iran J Allergy Asthma Immunol. (2018) 17:100–9. Available online at: https://ijaai.tums.ac.ir/index.php/ijaai/article/view/1623
4. Adjemian J, Frankland TB, Daida YG, Honda JR, Olivier KN, Zelazny A, et al. Epidemiology of nontuberculous mycobacterial lung disease and tuberculosis, Hawaii, USA. Emerg Infect Dis. (2017) 23:439–47. doi: 10.3201/eid2303.161827
5. Shahraki AH, Heidarieh P, Bostanabad SZ, Khosravi AD, Hashemzadeh M, Khandan S, et al. Multidrug-resistant tuberculosis may be nontuberculous mycobacteria. Eur J Intern Med. (2015) 26:279–84. doi: 10.1016/j.ejim.2015.03.001
6. Wang L, Zhang H, Ruan Y, Chin DP, Xia Y, Cheng S, et al. Tuberculosis prevalence in China, 1990–2010; a longitudinal analysis of national survey data. Lancet. (2014) 383:2057–64. doi: 10.1016/S0140-6736(13)62639-2
7. Halstrom S, Price P, Thomson RM. Environmental mycobacteria as a cause of human infection. Int J Mycobacteriol. (2015) 4:81–91. doi: 10.1016/j.ijmyco.2015.03.002
8. Wassilew N, Hoffmann H, Andrejak C, Lange C. Pulmonary disease caused by non-tuberculous mycobacteria. Respiration. (2016) 91:386–402. doi: 10.1159/000445906
10. Weiss CH, Glassroth J. Pulmonary disease caused by nontuberculous mycobacteria. Expert Rev Respir Med. (2012) 6:597–612. doi: 10.1586/ers.12.58
11. Yu X, Liu P, Liu G, Zhao L, Hu Y, Wei G, et al. The prevalence of non-tuberculous mycobacterial infections in mainland China: systematic review and meta-analysis. J Infect. (2016) 73:558–67. doi: 10.1016/j.jinf.2016.08.020
12. Andrejak C, Thomsen VO, Johansen IS, Riis A, Benfield TL, Duhaut P. Nontuberculous pulmonary mycobacteriosis in Denmark: incidence and prognostic factors. Am J Respir Crit Care Med. (2010) 181:514–21. doi: 10.1164/rccm.200905-0778OC
13. Moore JE, Kruijshaar ME, Ormerod LP, Drobniewski F, Abubakar I. Increasing reports of non-tuberculous mycobacteria in England, Wales and Northern Ireland, 1995-2006. BMC Public Health. (2010) 10:612. doi: 10.1186/1471-2458-10-612
14. Ringshausen FC, Apel RM, Bange FC, De Roux A, Pletz MW, Rademacher J, et al. Burden and trends of hospitalisations associated with pulmonary non-tuberculous mycobacterial infections in Germany, 2005-2011. BMC Infect Dis. (2013) 13:231. doi: 10.1186/1471-2334-13-231
15. Raveendran R, Oberoi JK, Wattal C. Multidrug-resistant pulmonary & extrapulmonary tuberculosis: A 13 years retrospective hospital-based analysis. Ind J Med Res. (2015) 142:575–82. doi: 10.4103/0971-5916.171285
16. Nasiri MJ, Dabiri H, Darban-Sarokhalil D, Hashemi Shahraki A. Prevalence of non-tuberculosis mycobacterial infections among tuberculosis suspects in Iran: systematic review and meta-Analysis. PLoS ONE. (2015) 10:e0129073. doi: 10.1371/journal.pone.0129073
17. Umrao J, Singh D, Zia A, Saxena S, Sarsaiya S, Singh S, et al. Prevalence and species spectrum of both pulmonary and extrapulmonary nontuberculous mycobacteria isolates at a tertiary care center. Int J Mycobacteriol. (2016) 5:288–93. doi: 10.1016/j.ijmyco.2016.06.008
18. World Health Organization. WHO TB Burden Estimates. Geneva. (2020). Available online at: https://www.who.int/tb/country/data/download/en/
19. Cowman S, Van Ingen J, Griffith DE, Loebinger MR. Non-tuberculous mycobacterial pulmonary disease. Eur Respir J. 6:210–20. doi: 10.1183/13993003.00250-2019
20. Sarro YD, Kone B, Diarra B, Kumar A, Kodio O, Fofana DB, et al. Simultaneous diagnosis of tuberculous and non-tuberculous mycobacterial diseases: time for a better patient management. Clin Microbiol Infect Dis. (2018) 3:1–8. doi: 10.15761/CMID.1000144
Keywords: non-tuberculous mycobacteria, atypical mycobacteria, prevalence, antibiotic resistance, dominant species, geographic distribution
Citation: Zhou L, Xu D, Liu H, Wan K, Wang R and Yang Z (2020) Trends in the Prevalence and Antibiotic Resistance of Non-tuberculous Mycobacteria in Mainland China, 2000–2019: Systematic Review and Meta-Analysis. Front. Public Health 8:295. doi: 10.3389/fpubh.2020.00295
Received: 14 March 2020; Accepted: 04 June 2020;
Published: 28 July 2020.
Edited by:
Zhongheng Zhang, Sir Run Run Shaw Hospital, ChinaReviewed by:
Pieter-Jan Ceyssens, Sciensano, BelgiumPaulo Bettencourt, University of Oxford, United Kingdom
Anita Luise Michel, University of Pretoria, South Africa
Copyright © 2020 Zhou, Xu, Liu, Wan, Wang and Yang. This is an open-access article distributed under the terms of the Creative Commons Attribution License (CC BY). The use, distribution or reproduction in other forums is permitted, provided the original author(s) and the copyright owner(s) are credited and that the original publication in this journal is cited, in accordance with accepted academic practice. No use, distribution or reproduction is permitted which does not comply with these terms.
*Correspondence: Ruibai Wang, d2FuZ3J1aWJhaSYjeDAwMDQwO2ljZGMuY24=; Zaichang Yang, eWFuZ3phaWNoYW5nJiN4MDAwNDA7MTI2LmNvbQ==