- 1Medical Imaging Department,Jining Psychiatric Hospital, Jining, China
- 2Psychiatric Department, Jining Psychiatric Hospital, Jining, China
- 3Medical Imaging Department, Affiliated Hospital of Jining Medical College, Jining, China
- 4Mental Diseases Prevention and Treatment Institute of Chinese PLA, No. 988 Hospital of Joint Logistic Support Force, Jiaozuo, China
- 5Mental Rehabilitation Department, Jining Psychiatric Hospital, Jining, China
Emotional abnormality in major depressive disorder (MDD) is generally regarded to be associated with functional dysregulation in the affective network (AN). The present study examined the changes in characteristics of AN connectivity of MDD patients before and after repetitive transcranial magnetic stimulation (rTMS) treatment over the left dorsolateral prefrontal cortex, and to further assess how these connectivity changes are linked to clinical characteristics of patients. Functional connectivity (FC) in the AN defined by placing seeds in the bilateral amygdale was calculated in 20 patients with MDD before and after rTMS, and in 20 healthy controls (CN). Furthermore, a linear regression model was used to obtain correlations between FC changes and Hamilton depression scale (HAMD) changes in MDD before and after rTMS. Before rTMS, compared with CN, MDD exhibited significantly lower FC between left insula (INS.L), right superior and inferior frontal gyrus (SFG.R and IFG.R), right inferior parietal lobule (IPL.R), and amygdala, and showed an increment of FC between the bilateral precuneus and amygdala in AN. After rTMS, MDD exhibited a significant increase in FC in the INS.L, IFG.R, SFG.R, IPL.R, and a significant reduction in FC in the precuneus. Interestingly, change in FC between INS.L and left amygdala was positively correlated with change in HAMD scores before and after rTMS treatment. rTMS can enhance affective network connectivity in MDD patients, which is linked to emotional improvement. This study further suggests that the insula may be a potential target region of clinical efficacy for MDD to design rationale strategies for therapeutic trials.
Introduction
Emotional abnormality is a crucial feature of major depressive disorder (MDD) and is also associated with functional dysregulation in the amygdala affective network (AN) (1–3). Furthermore, numerous studies have indicated that brain circuits and network dysfunction can cause depressive symptoms and disability (4, 5). Magnetic resonance imaging (MRI) has been considered to be a non-invasive imaging technology that can help establish relationships between brain circuit and human behavior (5). Therefore, it is essential to understand the relationship between depressive symptom and imaging feature for amelioration in the clinic in the course of MDD.
In resting-state functional connectivity (FC) MRI of MDD, the AN have attracted a great deal of interest. The AN is a frontolimbic circuit comprising brain areas in the amygdala, the subgenual anterior cingulate cortex, the hippocampus, the hypothalamus, orbitofrontal cortex (6, 7), the fusiform gyrus, and the medial frontal gyrus (3). The role of the AN is considered to be the processing emotions and mediating motivated behaviors (6, 7). Recently, numerous MRI studies have reported that MDD patients present dysfunctional AN, including lower FC between the bilateral precuneus and the left amygdala (3), lower FC between the orbitofrontal cortex and the amygdala that are negatively associated with depression scores (8), lower FC between amygdala and posterior insula that are negatively related to increasing severity of behavioral and emotional dysregulation (9), and reduced connectivity between the amygdala and the “positive network” (10). These studies suggest that some crucial brain areas are specifically related to processing and regulating emotions. Indeed, a recently published article has identified symptom-specific targets for MDD, and they thought that different depression symptom had different specific brain circuits (11). However, little is known about the consensus circuit, or the relationship between depressive symptom and AN circuits for amelioration in the clinic.
Transcranial magnetic stimulation (TMS) has been widely used in recent years. Previous studies have widely applied TMS to promote the improvement of cognition, enhancement of neural activity, restoration of network connectivity, and amelioration of negative symptom in neuropsychiatric disorders (4, 12–15), but the mechanism of TMS action is still mostly unclear. Furthermore, some studies have begun to investigate causal relationships between network markers and clinical phenomena in schizophrenia (4). Repetitive transcranial magnetic stimulation (rTMS) is considered as a non-invasive brain stimulation method to modulate cortical excitability (12). Furthermore, rTMS has been approved by the food and drug administration (FDA) to treat the symptoms of MDD. However, it is mostly unknown if AN network target pathology is causally linked to psychiatric symptoms and if the TMS manipulation of network target can reflect the symptom modification of patients with MDD.
The present study examined the changes in the patterns of AN connectivity of patients with MDD before and after rTMS treatment, and further assessed how these connectivity changes are linked to clinical characteristics of patients. We hypothesized that MDD patients would exhibit lower affective network connectivity (for example, lower FC between the amygdala and the insula). We also hypothesized that high-frequency excitatory rTMS could enhance the emotional network connectivity (for example, increase the FC the amygdala and the insula) in MDD patients. Furthermore, it was further hypothesized that changes in AN induced by rTMS are linked to modifications of depressive symptoms of MDD.
Materials and Methods
Subjects
Forty MDD patients (who met DSM-5 criteria, right-handed) and 20 healthy controls (CN, right-handed) were recruited from inpatients at the Medical Imaging Department of Jining Psychiatric Hospital (Table 1). The inclusion and exclusion criteria of subjects can be seen in the published studies (3, 16, 17). The depressed group consisted of consecutively recruited subjects who met the Diagnostic and Statistical Manual of Mental Disorders, 5th Edition (DSM-5) criteria for major depression without psychotic features and had a score of 20 or higher on the 17-item Chinese Hamilton Depression Scale (HAMD) (18). The healthy controls (CN) were recruited through advertisement and were required to have no history or presence of any psychiatric disorder. The subjects signed written informed consent was taken from all subjects. This study was approved by the Human Participants Ethics Committee at the Medical Imaging Department of Jining Psychiatric Hospital. All patients were randomly assigned to the rTMS group (N = 20) and the sham group (N = 20). The rTMS (or sham) was applied daily on weekdays between Monday and Friday, at the same time of the day, in a course comprising of 25 sessions. Participants received treatment of rTMS or sham over four weeks. Note: the CN subjects were only used to identify brain regions affected by MDD by comparing the network connectivity between CN and MDD and didn’t receive rTMS.
Neuropsychological and MRI assessments were performed on Monday 1 morning at baseline and after 4-week treatment (rTMS or sham). All researchers performing patients’ evaluations were blind to their experimental arm belonging.
Individuals were excluded if they had severe suicide risk, a history of stroke, impaired thyroid function, multiple sclerosis, or degenerative diseases; metastatic cancer, brain tumors, unstable cardiac, hepatic, or renal disease, myocardial infarction, or stroke within the three months preceding the study; metal implants. MDD subjects with history of comorbid Axis I diagnosis were excluded.
MRI Data Acquisition
MRI images were acquired using a 1.5T Avanto Siemens scanner (Siemens, Erlangen, Germany) with a 12-channel head coil. Resting-state functional images including 240 volumes were obtained by gradient-recalled echo-planar imaging (GRE-EPI) sequence (EPI: TR = 2,000 ms, TE = 25 ms, FA = 90°, matrix = 64 × 64, FOV = 240 mm × 240 mm, thickness = 4.0 mm, no gap, number of slices = 36, voxel size = 3.75 × 3.75 × 4 mm3). High-resolution T1-weighted axial images covering the whole brain were acquired by 3D magnetization prepared rapid gradient echo (MPRAGE) sequence (TR = 1,900 ms, TE = 2.48 ms; FA = 9°, matrix = 256 × 256, FOV = 250 × 250 mm, thickness = 1.0 mm, no gap, number of slices = 176, voxel size = 1 × 1 × 1 mm3).
rTMS Protocol
rTMS was delivered using a Magstim Rapid2 magnetic stimulator with a 70-mm figure-8-shaped coil. In all participants, rTMS was applied over the left dorsolateral prefrontal cortex (DLPFC). For stimulation of the DLPFC, the tip of the intersection of the two coil loops was lined up with the F3 sites of the 10–20 electroencephalogram system (19).
The rTMS was applied, using trains of 1,000 stimuli at 10 Hertz (Hz) frequency and an intensity of 90% of the motor threshold (MT). The MT was defined as the lowest intensity (as assessed with single-pulse TMS) producing motor evoked potentials of greater than 50 μV in at least five out of 10 trials in the relaxed first dorsal interosseous (FDI) muscle of the contralateral (right) hand (20). No significant differences were found in MT values between CNs (61 ± 6.3%) and MDD patients (60 ± 7.0%) (CN only perform an MT to compare to MDD and didn’t receive rTMS treatment). Participants received 25 sessions of either rTMS or sham stimulation over the left DLPFC. Each daily stimulation session consisted of 40 trains of 4 s duration with an interval of 56 s. The entire session lasted approximately 25 min twice each day (once at 8 o’clock morning and once at 4 o’clock afternoon), and the interval between daily sessions is 8 h. The total sessions are 1,000 (25 sessions/time * 2 times /day * 5 days/week * 4 weeks), and The total trains are 40000 (40 trains/session * 25 sessions/time * 2 times /day * 5 days/week * 4 weeks). The sham rTMS blocks were conducted, with the coil held close to the DLPFC, but angled away. We chose 90% of MT, although the FDA approved protocol suggests 120% of MT. This reason specified regarding this choice as follows: our subjects were made up of people with 17-item HAMD scores greater than 20, that is, all subjects are major depressive disorder. In the early stages, the use of 120% of MT in some of the patients was attempted, but these subjects felt uncomfortable or emotionally disturbed. Furthermore, some studies have also shown that 90% of MT can improve HAMD scores significantly (21, 22). Finally, to meet safety recommendations, 90% of MT was found most suitable for use.
The sham rTMS group were conducted, with the coil held close to the left DLPFC but angled away (13).
Image Preprocessing
Data analyses of groups were conducted with Matlab (Math Works Inc., Natick, MA, USA), and Data Processing & Analysis for Brain Imaging (DPABI) (23) based on SPM8. To eliminate T1 equilibration effects, the first ten volumes were discarded. Images were then time-shifted and motion-corrected (24, 25). Participants with head motion more than 2 mm maximum displacement in any direction of x, y, and z or 2◦ of any angular motion throughout the course of scan were excluded from the present study. The resulting images were linearly normalized into Montreal Neurological Institute (MNI) space using a 12-parameter affine approach and an EPI template image. Functional images were resampled to 2 × 2 × 2 mm3 voxels and spatially smoothed (6 mm full-width half-maximum Gaussian kernel). Linear detrending and temporal band-pass filtering (0.01–0.08 Hz) were applied to reduce the effect of low-frequency drifts and high-frequency physiological noise. Finally, several nuisance variables, including six head motion parameters, global mean signal (26), CSF signal, and WM signal were removed by multiple linear regression analysis. There were no significant differences between groups in head motion parameters (t = 0.12, p >0.05 for CN vs. MDD; t = 1.16, p >0.05 for pre-rTMS group vs. post- rTMS group; t = 1.34, p >0.05 for pre-sham group vs. post-sham group)) (24, 25).
Functional Connectivity Analysis
The seed region of interest (ROI) located in the left and right amygdala (Figures 1A and 2A) was determined by using automated anatomic labelling template (27) implemented with wfu_PickAtlas software and functional connectivity analysis was referred to the previously published study (3). We extracted the individual time courses for each seed as the reference time course, and then performed a voxelwise cross-correlation analysis between the seed region and the whole brain within the grey matter (GM) mask.
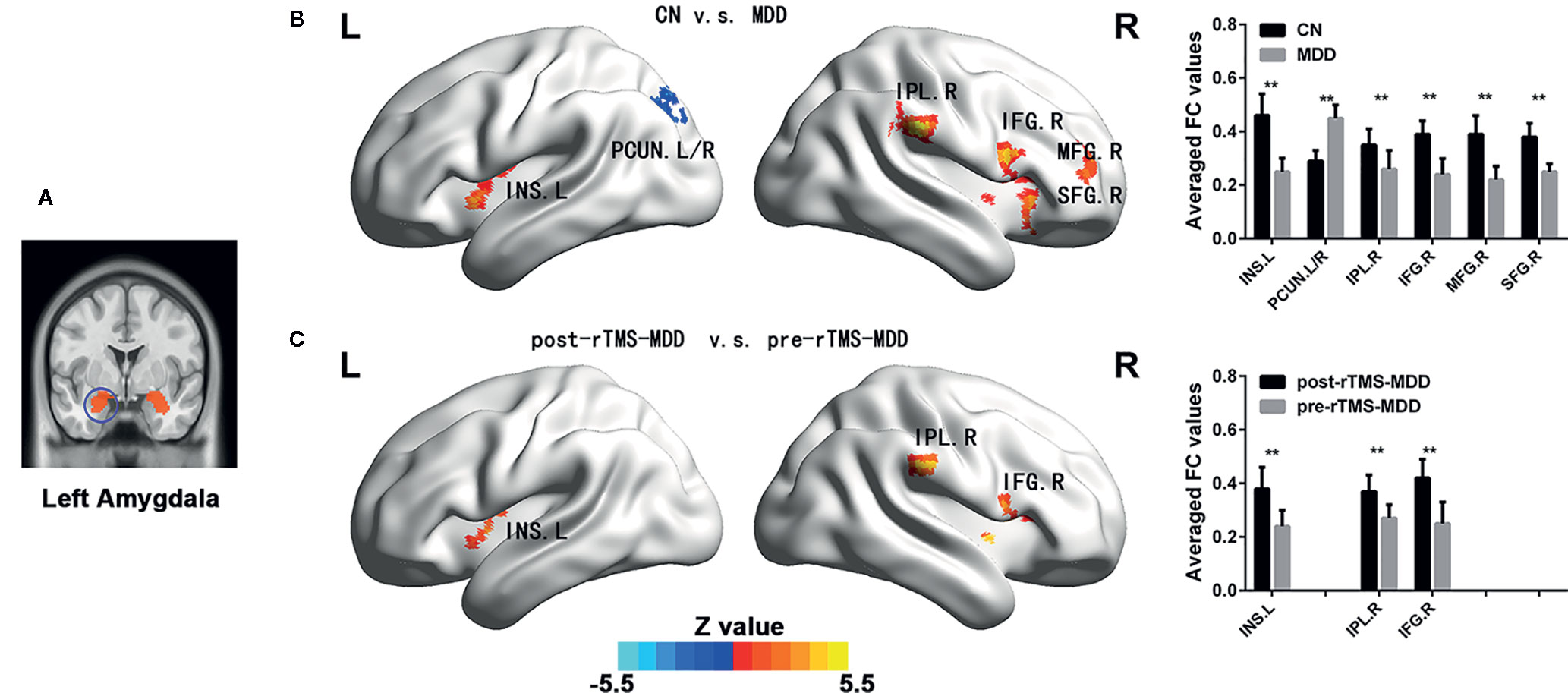
Figure 1 Comparisons of functional connectivity of the left affective network between MDD patients before and after treatment and CN subjects. (A) indicating left amygdala ROI. (B) brain different regions of the left amygdala functional connectivity between CN and MDD, and pre-rTMS-MDD and post-rTMS-MDD. (C) The bar chart shows quantitative differences in left amygdala functional connectivity of these differential brain regions. The statistical maps were managed by False Discovery Rate (FDR) for multiple comparisons to a significant level of p <0.01, with cluster size over 160 mm3. CN, controls; MDD, major depressive disorder; pre-rTMS-MDD, MDD before rTMS treatment; post-rTMS-MDD, MDD after rTMS treatment; PCUN.L/R, left/right Precuneus; INS.L, left Insula; IFG.R, right Inferior Frontal Gyrus; IPL.R,right Inferior Parietal Lobule; SFG.R, right Superior Frontal Gyrus. **p <0.01 with FDR for multiple comparisons.
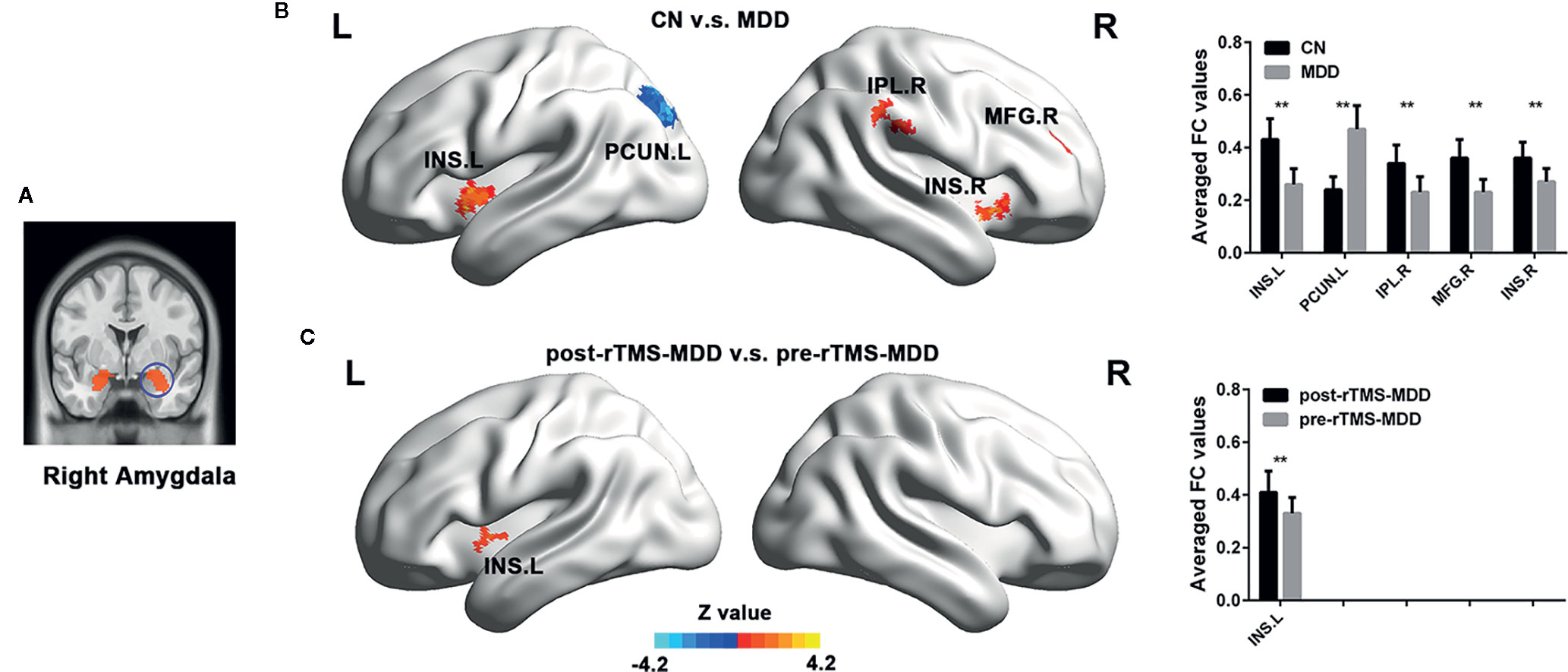
Figure 2 Comparisons of functional connectivity of the right affective network between MDD patients before and after treatment and CN subjects. (A) indicating the right amygdala ROI. (B) brain different regions of the right amygdala functional connectivity between CN and MDD, and pre-rTMS-MDD and post-rTMS-MDD. (C) The bar chart shows quantitative differences in right amygdala functional connectivity of these differential brain regions. The statistical maps were managed by FDR for multiple comparisons to a significant level of p <0.01, with cluster size over 160 mm3. CN, controls; MDD, major depressive disorder; pre-rTMS-MDD, MDD before rTMS treatment; post-rTMS-MDD, MDD after rTMS treatment; PCUN.L, left Precuneus; INS.L, left Insula; INS.R, right Insula; IPL.R, right Inferior Parietal Lobul. **p <0.01 with FDR for multiple comparisons.
Statistical Analysis
Demographic and Neuropsychological Data
The demographic data and clinical variables were compared using two-tailed analysis of variance, Two-samples t-test and chi-square test between MDD and CN.
Comparisons of Affective Network Connectivities Between CN and all MDD Subjects
To explain the changes that are related to MDD during rTMS treatment, we performed a voxel-wise analysis. Using a voxel-wise analysis, we compare CN to all 40 MDD (both the rTMS group and the sham group before rTMS) to identify brain regions affected by MDD. Using general mixed linear model analysis, we assessed the differences of the affective network connectivities between all MDD subjects and CN with age, gender, education level and voxel-wise GM volume map treated as covariates. Then a mask was made based on these different brain regions related to MDD. The statistical maps were managed by False Discovery Rate (FDR) for multiple comparisons to a significant level of p <0.01, with cluster size over 160 mm3.
Comparisons of Affective Network Connectivities Between Pre-rTMS (or Sham) Group and Post- rTMS (or Sham) Group
Using general linear mixed model analysis, we assessed the differences of the affective network connectivities between pre-rTMS group and post- rTMS group (or pre-sham group v.s. post-sham group) within the above-referenced mask with age, gender, education level and voxel-wise GM volume map treated as covariates. The statistical maps were managed by FDR for multiple comparisons to a significant level of p <0.01, with cluster size over 160 mm3.
Correlations Between Changes of Connectivity and HAMD Scores Pre- Versus Post-rTMS
For each participant, the change in affective network connectivity was calculated to examine the correlations between FC changes post- versus pre-rTMS and HAMD scores pre- versus post-rTMS. The relationships between FC changes and HAMD changes in MDD before and after rTMS was also investigated using a linear regression model (independent variable: FC changes, dependent variable: HAMD changes).
Results
Demographic and Neuropsychological Characteristics
No significant differences were observed in age, gender, or education level between the MDD patients and CN groups (all p >0.05, Table 1).
Comparison of Affective Network Connectivities Between CN and All MDD Subjects
In the left affective network, compared with CN, MDD subjects showed significantly lower FC between left insula (INS.L), right inferior frontal gyrus (IFG.R), right superior frontal gyrus (SFG.R), right inferior parietal lobule (IPL.R), right middle frontal gyrus (MFG.R) and left amygdala, and showed significantly higher FC between bilateral PreCUN and left amygdala (Figures 1A, B and Table 2).
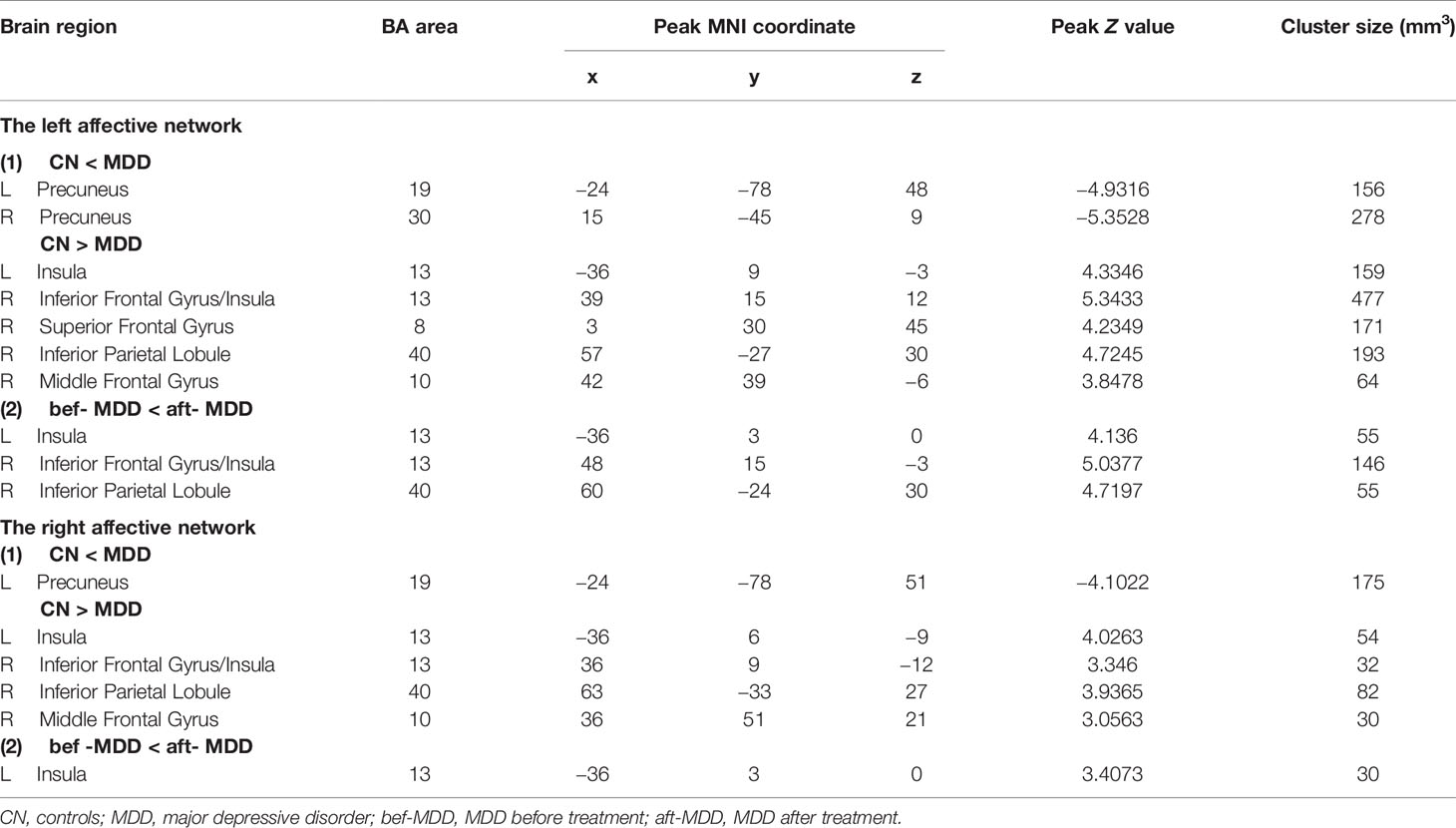
Table 2 Functional connectivity of the affective network between MDD and CN, and bef-MDD and aft-MDD.
In the right affective network, compared with CN, MDD subjects exhibited significantly lower FC between INS.L, IFG.R/INS.R, IPL.R, MFG.R and right amygdala, and exhibited a significantly higher FC between left precuneus (PCUN.L) and right amygdala (Figure 2B and Table 2).
Comparison of Affective Network Connectivities Between Pre-rTMS (or Sham) and Post-rTMS (or Sham) in MDD Patients
In the left affective network, compared with pre-rTMS-MDD, post-rTMS-MDD exhibited a significant increase of FC between INS.L, IFG.R/ right insula (INS.R), IPL.R and left amygdala (Figures 1A, C and Table 2). Compared with post-sham-rTMS-MDD, post-rTMS-MDD exhibited a significant rise in FC between INS.L, IFG.R/ right insula (INS.R), IPL.R and left amygdale.
In the right affective network, compared with pre-rTMS-MDD, post-rTMS-MDD exhibited a significant increase of FC between INS.L and right amygdala (Figures 2A, C and Table 2). Compared with post-sham-rTMS-MDD, post-rTMS-MDD exhibited a significant increase of FC between INS.L and right amygdala.
Furthermore, there were no changes for AN functional connectivity between pre-sham group vs. post-sham group.
Correlations Between Changes of Connectivity and HAMD Scores Pre- Versus Post-rTMS or Pre-Sham Versus Post-Sham-rTMS
As shown in Figure 3, the change in FC between INS.L and left amygdala was positively correlated with the changes in HAMD scores before and after TMS treatment (p <0.001). Furthermore, there were no correlations between changed FC and the changes in HAMD scores (25.50 v.s. 24.95) before and after sham TMS treatment (p >0.05).
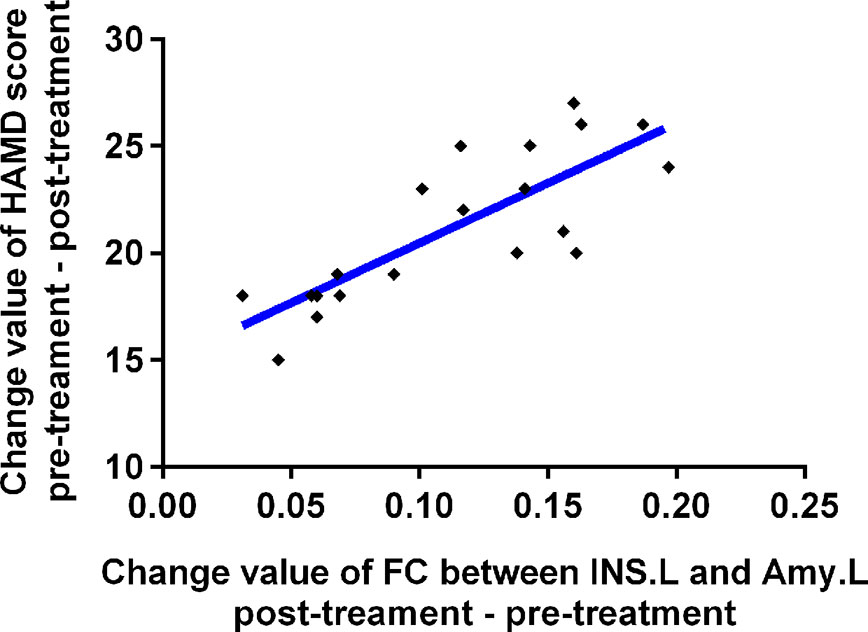
Figure 3 Relationships between change value of HAMD scores and change value of affective network connectivity between MDD patients before and after treatment. MDD, major depressive disorder; FC, functional connectivity; bef-MDD, MDD before treatment; aft-MDD, MDD after treatment; INS.L, left Insula; Amy.L, left Amygdala; HAMD, Hamilton depression scale.
Discussion
This study investigated the changing patterns of amygdala connectivity of MDD patients before and after rTMS treatment, and illuminated brain circuit or the relationship between the network and human behaviour in MDD. Firstly, compared to CN, MDD subjects showed significantly lower FC between INS.L, IPL.R, IFG.R, MFG.R, SFG.R and amygdala, and exhibited significantly higher FC between the bilateral precuneus and the left amygdala before rTMS. Secondly, after rTMS, MDD showed a significant increased FC in the INS.L, IFG.R/INS.R, and IPL.R. Finally, changes in FC between INS.L and left amygdala were positively correlated with changes in HAMD scores before and after rTMS treatment. Altogether, this study promotes our further understanding of the possible anatomical basis of MDD associated with emotional dysregulation.
Before rTMS treatment, this study exhibited lower FC between the left insula and amygdala in MDD patients, which are in substantial agreement with previous studies (9). It has been indicated that emotional dysregulation in MDD patients is related to FC between amygdala and insula supporting emotion regulation (28). Some anatomical studies have reported that anterior insula is connected with the amygdala by subgenual ACC, which plays a crucial role in generating negative mood states (29). Functional neuroimaging studies in the resting brain have also demonstrated that the anterior insular cortex subserves the emotional functions (30, 31). Furthermore, the present study also exhibited lower FC between the right superior and inferior frontal gyrus, right inferior parietal lobule and amygdala. Our findings also suggest that MDD patients show an impairment of salience and emotion perception, and impairment of emotional controlling and goal-directed behavior, which is in concordance with previous studies (30, 32, 33). Indeed, some recent studies have also reported the therapeutic effects in AN (34) and frontostriatal network (35) in patients with MDD. However, our findings are inconsistent with a recent study (34), who investigated the effect of rTMS on neural connections in depressed patients. Although they also showed changes in amygdala connectivity, their findings showed the more subgenual anterior cingulate cortex role while our study mainly found reduced connectivities of the left insula, right superior and inferior frontal gyrus, right inferior parietal lobule in AN. This reason for the differences may be as follows. The disease severity of our subjects (mean HAMD-17 score: 26.95 for active rTMS, and 25.5 for sham rTMS) were more severe than those of their study (mean HRSD-17 score: 16 for active rTMS, and 13.1 for sham rTMS). Indeed, a series of studies have shown different emotional processing between minor depression and major depression and different mechanisms of electrophysiological damage (36).
Interestingly, our study showed increased FC between the bilateral precuneus and amygdala in MDD patients, which indicates increased connectivity could be associated with the persistently negative mood state and rumination (3). The precuneus is consistently considered to participate in the processing of episodic memory (37) and to be implicated in the encoding of self-relevant information (38, 39). A large number of studies have indicated that the precuneus is a crucial node of the so-called default mode network (DMN) (40, 41). Accumulating evidence has indicated that the DMN is involved in self-referential processing, including self-prospecting and internal monitoring, autobiographical memory retrieval, future planning, and theory of mind (40, 42, 43). Therefore, our findings further suggest that patients with MDD may fail to restrain negative self-thoughts (44). This finding is inconsistent with our earlier results done at another research centre, which showed reduced FC between the left amygdala and the bilateral precuneus in MDD patients (3). It could be due to the difference in the mean age of subjects in the current study (46.75 years) v.s. the previous studies (32.11 years). Indeed, previous studies have reported that MDD presented age-related abnormalities of grey and white matter (45), and age-related changes in physiological functioning (46). Furthermore, the mean duration of illness in this study (25.00 months) was longer than those of previous studies (mean = 0.7 years). Previous studies have also indicated that duration of illness can affect adverse consequences in terms of outcome for individuals with MDD (47). Therefore, it is reasonable to assume that these inconsistencies in results are due to differences in age of subjects and the duration of their illness.
After rTMS treatment, our study showed significantly increased FC in these above-mentioned brain regions (INS.L, IFG.R, SFG.R, and IPL.R) showing altered connectivity in the affective network. Furthermore, these frontoparietal structures are classified as domain-general control regions, that is so-called cognitive control network (48, 49). Our findings suggest that rTMS can improve functional connectivities of brain regions in interaction hubs between the affective network and executive function network in MDD patients, which provides a potentially reproducible model of TMS-based rescue of a breakdown of connectivity. Some studies have reported that insula is connected with prefrontal cortices, which plays a role in emotional controlling and goal-directed behaviour (33). AN is considered to participate in emotional processing and mediating motivated behaviors (7, 50), emotional controlling and goal-directed behaviour (33). Meanwhile, orbital fronto-insular cortices are part of both the salience network and the AN (51). A recently published article has identified symptom-specific targets for MDD, and they thought that different depression symptom had different specific brain circuits (11). It is reasonable to speculate that the AN might be partially connected with the salience network in MDD patients. The excitatory rTMS plays a therapeutic improvement role in through stimulation of the DLPFC, which is connected with the salience network and the AN. Altogether, our findings suggest that non-invasive treatment of AN network dysfunction by stimulating the DLPFC is an effective strategy to improve depressive symptoms in MDD patients.
Interestingly, this study also found that changes in FC between INS.L and left amygdala was positively correlated with changes in HAMD scores before and after rTMS treatment, which indicates the existence of at least one network circuit linked directly to depressive symptoms. Indeed, it has been hypothesized that emotional dysregulation is considered to contribute to altered FC between the amygdala and insula (28), and it is reported that an insula-centered neural network is considered to support salience and emotion perception (30, 32). The insula is also believed to be connected with prefrontal cortices, which plays a role in emotional controling and goal-directed behavior (33). Therefore, it is reasonable to speculate that the rTMS-induced improvement of depressive symptoms supports the standpoint that DLPFC is directly implicated in the onset of depressive symptom in MDD patients. According to a neurobiological view, rTMS may induce relevant modulations to activate the changes in synaptic plasticity over a DLPFC–amygdala–insula circuit, which leads to clinical improvement. Therefore, the precise localiZation of a DLPFC–amygdala–insula circuit is critical in identifying the underlying neural substrates of the disabling depressive symptoms in patients with MDD. Our results provide experimental evidence in support of such a circuit, which, when directly modulated, rescues these deficits.
However, this has some limitations. Firstly, the longitudinal data was not available for the controls. In future studies, the longitudinal data of controls should be designed to ensure that the network changes observed pre/post-treatment are due to rTMS and not merely within the range of normal variation in FC, although FC is not expected to change within a short period. Secondly, this sham stimulation may affect our findings. The current sham rTMS studies are conducted using coils specially designed to deliver sham stimulation. However, tilting the coil away from DLPFC still provides low-intensity stimulation due to the magnetic field at the edge of the coil. Therefore, this limitation should be kept in mind. Finally, amygdala network connectivity is not the same as AN connectivity. The amygdala is associated with multiple functional networks, including the so-called salience network, which is involved in attentional and cognitive control in domains beyond affect. Although we have explained that TMS can improve functional connectivities of brain regions in interaction hubs between amygdala affective network and other networks (i.e. executive function network, DMN, and salience network and so on) in MDD patients, our interpretation of the results needs to be cautious.
The present study provides novel evidence that rTMS may be an effective strategy for improving the depressive symptom of patients with MDD, which may be useful in further characterizing network–symptom relationship in MDD. While our results demonstrate a target biological substrate for the treatment of depressive symptoms, we do not suggest that DLPFC–amygdala–insula-targeted TMS is the sole intervention that can do so. Our results raise the possibility that functional connectivity may be a useful marker of efficacy for other therapeutic interventions in the treatment of depressive symptoms. It further suggests that the amygdala–insula circuit may be a potential interventional target circuit of clinical efficacy for MDD to design rationale strategies for therapeutic trials.
Data Availability Statement
The datasets generated for this study are available on request to the corresponding authors.
Ethics Statement
The studies involving human participants were reviewed and approved by Human Participants Ethics Committee at the Medical Imaging Department of Jining Psychiatric Hospital. The patients/participants provided their written informed consent to participate in this study.
Author Contributions
F-JC, C-ZG, H-FD, and XZ contributed study concept and design. NZ, H-FD, A-LZ, and XZ acquired, analyzed, or interpreted data. F-JC and H-FD drafted and revised the manuscript. All authors contributed to the article and approved the submitted version.
Conflict of Interest
The authors declare that the research was conducted in the absence of any commercial or financial relationships that could be construed as a potential conflict of interest.
References
1. Mayberg HS. Modulating dysfunctional limbic-cortical circuits in depression: towards development of brain-based algorithms for diagnosis and optimised treatment. Br Med Bull (2003) 65:193–207. doi: 10.1093/bmb/65.1.193
2. Rigucci S, Serafini G, Pompili M, Kotzalidis GD, Tatarelli R. Anatomical and functional correlates in major depressive disorder: the contribution of neuroimaging studies. World J Biol Psychiatry (2010) 11:165–80. doi: 10.1080/15622970903131571
3. Wang YL, Yang SZ, Sun WL, Shi YZ, Duan HF. Altered functional interaction hub between affective network and cognitive control network in patients with major depressive disorder. Behav Brain Res (2016) 298:301–9. doi: 10.1016/j.bbr.2015.10.040
4. Brady RO Jr., Gonsalvez I, Lee I, Ongur D, Seidman LJ, Schmahmann JD, et al. Cerebellar-Prefrontal Network Connectivity and Negative Symptoms in Schizophrenia. Am J Psychiatry (2019) 176:512–20. doi: 10.1176/appi.ajp.2018.18040429
5. Bebko G, Bertocci MA, Fournier JC, Hinze AK, Bonar L, Almeida JR, et al. Parsing dimensional vs diagnostic category-related patterns of reward circuitry function in behaviorally and emotionally dysregulated youth in the Longitudinal Assessment of Manic Symptoms study. JAMA Psychiatry (2014) 71:71–80. doi: 10.1001/jamapsychiatry.2013.2870
6. Yeo BT, Krienen FM, Sepulcre J, Sabuncu MR, Lashkari D, Hollinshead M, et al. The organization of the human cerebral cortex estimated by intrinsic functional connectivity. J Neurophysiol (2011) 106:1125–65. doi: 10.1152/jn.00338.2011
7. Lindquist KA, Wager TD, Kober H, Bliss-Moreau E, Barrett LF. The brain basis of emotion: a meta-analytic review. Behav Brain Sci (2012) 35:121–43. doi: 10.1017/S0140525X11000446
8. Kim SM, Park SY, Kim YI, Son YD, Chung US, Min KJ, et al. Affective network and default mode network in depressive adolescents with disruptive behaviors. Neuropsychiatr Dis Treat (2016) 12:49–56. doi: 10.2147/NDT.S95541
9. Bebko G, Bertocci M, Chase H, Dwojak A, Bonar L, Almeida J, et al. Decreased amygdala-insula resting state connectivity in behaviorally and emotionally dysregulated youth. Psychiatry Res (2015) 231:77–86. doi: 10.1016/j.pscychresns.2014.10.015
10. Luking KR, Repovs G, Belden AC, Gaffrey MS, Botteron KN, Luby JL, et al. Functional connectivity of the amygdala in early-childhood-onset depression. J Am Acad Child Adolesc Psychiatry (2011) 50:1027–1041 e1023. doi: 10.1016/j.jaac.2011.07.019
11. Siddiqi SH, Taylor SF, Cooke D, Pascual-Leone A, George MS, Fox MD. Distinct Symptom-Specific Treatment Targets for Circuit-Based Neuromodulation. Am J Psychiatry (2020) 177:435–46. doi: 10.1176/appi.ajp.2019.19090915
12. Rossi S, Hallett M, Rossini PM, Pascual-Leone A, Safety of TMSCG. Safety, ethical considerations, and application guidelines for the use of transcranial magnetic stimulation in clinical practice and research. Clin Neurophysiol (2009) 120:2008–39. doi: 10.1016/j.clinph.2009.08.016
13. Turriziani P, Smirni D, Zappala G, Mangano GR, Oliveri M, Cipolotti L. Enhancing memory performance with rTMS in healthy subjects and individuals with Mild Cognitive Impairment: the role of the right dorsolateral prefrontal cortex. Front Hum Neurosci (2012) 6:62:62. doi: 10.3389/fnhum.2012.00062
14. Koch G, Bonni S, Pellicciari MC, Casula EP, Mancini M, Esposito R, et al. Transcranial magnetic stimulation of the precuneus enhances memory and neural activity in prodromal Alzheimer’s disease. Neuroimage (2017) 169:302–11. doi: 10.1016/j.neuroimage.2017.12.048
15. Rabey JM, Dobronevsky E. Repetitive transcranial magnetic stimulation (rTMS) combined with cognitive training is a safe and effective modality for the treatment of Alzheimer’s disease: clinical experience. J Neural Transm (Vienna) (2016) 123:1449–55. doi: 10.1007/s00702-016-1606-6
16. Chen J, Ma W, Zhang Y, Yang LQ, Zhang Z, Wu X, et al. Neurocognitive impairment of mental rotation in major depressive disorder: evidence from event-related brain potentials. J Nerv Ment Dis (2014) 202:594–602. doi: 10.1097/NMD.0000000000000167
17. Chen J, Yang L, Ma W, Wu X, Zhang Y, Wei D, et al. Ego-rotation and object-rotation in major depressive disorder. Psychiatry Res (2013) 209:32–9. doi: 10.1016/j.psychres.2012.10.003
18. Zheng YP, Zhao JP, Phillips M, Liu JB, Cai MF, Sun SQ, et al. Validity and reliability of the Chinese Hamilton Depression Rating Scale. Br J Psychiatry (1988) 152:660–4. doi: 10.1192/bjp.152.5.660
19. Turriziani P, Smirni D, Oliveri M, Semenza C, Cipolotti L. The role of the prefrontal cortex in familiarity and recollection processes during verbal and non-verbal recognition memory: an rTMS study. Neuroimage (2010) 52:348–57. doi: 10.1016/j.neuroimage.2010.04.007
20. Rossini PM, Burke D, Chen R, Cohen LG, Daskalakis Z, Di Iorio R, et al. Non-invasive electrical and magnetic stimulation of the brain, spinal cord, roots and peripheral nerves: Basic principles and procedures for routine clinical and research application. An updated report from an I.F.C.N. Committee. Clin Neurophysiol (2015) 126:1071–107. doi: 10.1016/j.clinph.2015.02.001
21. Williams NR, Sudheimer KD, Bentzley BS, Pannu J, Stimpson KH, Duvio D, et al. High-dose spaced theta-burst TMS as a rapid-acting antidepressant in highly refractory depression. Brain (2018) 141:e18. doi: 10.1093/brain/awx379
22. Sonmez AI, Camsari DD, Nandakumar AL, Voort JLV, Kung S, Lewis CP, et al. Accelerated TMS for Depression: A systematic review and meta-analysis. Psychiatry Res (2019) 273:770–81. doi: 10.1016/j.psychres.2018.12.041
23. Yan CG, Wang XD, Zuo XN, Zang YF. DPABI: Data Processing & Analysis for (Resting-State) Brain Imaging. Neuroinformatics (2016) 14:339–51. doi: 10.1007/s12021-016-9299-4
24. Power JD, Barnes KA, Snyder AZ, Schlaggar BL, Petersen SE. Spurious but systematic correlations in functional connectivity MRI networks arise from subject motion. Neuroimage (2012) 59:2142–54. doi: 10.1016/j.neuroimage.2011.10.018
25. Van Dijk KR, Sabuncu MR, Buckner RL. The influence of head motion on intrinsic functional connectivity MRI. Neuroimage (2012) 59:431–8. doi: 10.1016/j.neuroimage.2011.07.044
26. Fox MD, Zhang D, Snyder AZ, Raichle ME. The global signal and observed anticorrelated resting state brain networks. J Neurophysiol (2009) 101:3270–83. doi: 10.1152/jn.90777.2008
27. Tzourio-Mazoyer N, Landeau B, Papathanassiou D, Crivello F, Etard O, Delcroix N, et al. Automated anatomical labeling of activations in SPM using a macroscopic anatomical parcellation of the MNI MRI single-subject brain. Neuroimage (2002) 15:273–89. doi: 10.1006/nimg.2001.0978
28. Phillips ML, Ladouceur CD, Drevets WC. A neural model of voluntary and automatic emotion regulation: implications for understanding the pathophysiology and neurodevelopment of bipolar disorder. Mol Psychiatry (2008) 13:829, 833–857. doi: 10.1038/mp.2008.65
29. Mayberg HS, Liotti M, Brannan SK, McGinnis S, Mahurin RK, Jerabek PA, et al. Reciprocal limbic-cortical function and negative mood: converging PET findings in depression and normal sadness. Am J Psychiatry (1999) 156:675–82. doi: 10.1176/ajp.156.5.675
30. Dupont S, Bouilleret V, Hasboun D, Semah F, Baulac M. Functional anatomy of the insula: new insights from imaging. Surg Radiol Anat (2003) 25:113–9. doi: 10.1007/s00276-003-0103-4
31. Cauda F, D’Agata F, Sacco K, Duca S, Geminiani G, Vercelli A. Functional connectivity of the insula in the resting brain. Neuroimage (2011) 55:8–23. doi: 10.1016/j.neuroimage.2010.11.049
32. Dolan RJ. Emotion, cognition, and behavior. Science (2002) 298:1191–4. doi: 10.1126/science.1076358
33. Cloutman LL, Binney RJ, Drakesmith M, Parker GJ, Lambon Ralph MA. The variation of function across the human insula mirrors its patterns of structural connectivity: evidence from in vivo probabilistic tractography. Neuroimage (2012) 59:3514–21. doi: 10.1016/j.neuroimage.2011.11.016
34. Taylor SF, Ho SS, Abagis T, Angstadt M, Maixner DF, Welsh RC, et al. Changes in brain connectivity during a sham-controlled, transcranial magnetic stimulation trial for depression. J Affect Disord (2018) 232:143–51. doi: 10.1016/j.jad.2018.02.019
35. Kang JI, Lee H, Jhung K, Kim KR, An SK, Yoon KJ, et al. Frontostriatal Connectivity Changes in Major Depressive Disorder After Repetitive Transcranial Magnetic Stimulation: A Randomized Sham-Controlled Study. J Clin Psychiatry (2016) 77:e1137–43. doi: 10.4088/JCP.15m10110
36. Wu X, Chen J, Jia T, Ma W, Zhang Y, Deng Z, et al. Cognitive Bias by Gender Interaction on N170 Response to Emotional Facial Expressions in Major and Minor Depression. Brain Topogr (2016) 29:232–42. doi: 10.1007/s10548-015-0444-4
37. Cavanna AE, Trimble MR. The precuneus: a review of its functional anatomy and behavioural correlates. Brain (2006) 129:564–83. doi: 10.1093/brain/awl004
38. Kjaer TW, Nowak M, Lou HC. Reflective self-awareness and conscious states: PET evidence for a common midline parietofrontal core. Neuroimage (2002) 17:1080–6. doi: 10.1006/nimg.2002.1230
39. Amft M, Bzdok D, Laird AR, Fox PT, Schilbach L, Eickhoff SB. Definition and characterization of an extended social-affective default network. Brain Struct Funct (2015) 220:1031–49. doi: 10.1007/s00429-013-0698-0
40. Buckner RL, Andrews-Hanna JR, Schacter DL. The brain’s default network: anatomy, function, and relevance to disease. Ann N Y Acad Sci (2008) 1124:1–38. doi: 10.1196/annals.1440.011
41. Raichle ME, MacLeod AM, Snyder AZ, Powers WJ, Gusnard DA, Shulman GL. A default mode of brain function. Proc Natl Acad Sci U S A (2001) 98:676–82. doi: 10.1073/pnas.98.2.676
42. Spreng RN, Mar RA, Kim AS. The common neural basis of autobiographical memory, prospection, navigation, theory of mind, and the default mode: a quantitative meta-analysis. J Cognit Neurosci (2009) 21:489–510. doi: 10.1162/jocn.2008.21029
43. Northoff G, Bermpohl F. Cortical midline structures and the self. Trends Cognit Sci (2004) 8:102–7. doi: 10.1016/j.tics.2004.01.004
44. Aghajani M, Veer IM, van Tol MJ, Aleman A, van Buchem MA, Veltman DJ, et al. Neuroticism and extraversion are associated with amygdala resting-state functional connectivity. Cognit Affect Behav Neurosci (2014) 14:836–48. doi: 10.3758/s13415-013-0224-0
45. Soriano-Mas C, Hernandez-Ribas R, Pujol J, Urretavizcaya M, Deus J, Harrison BJ, et al. Cross-sectional and longitudinal assessment of structural brain alterations in melancholic depression. Biol Psychiatry (2011) 69:318–25. doi: 10.1016/j.biopsych.2010.07.029
46. Sacchet MD, Camacho MC, Livermore EE, Thomas EAC, Gotlib IH. Accelerated aging of the putamen in patients with major depressive disorder. J Psychiatry Neurosci (2017) 42:164–71. doi: 10.1503/jpn.160010
47. Dell’Osso B, Cremaschi L, Grancini B, De Cagna F, Benatti B, Camuri G, et al. Italian patients with more recent onset of Major Depressive Disorder have a shorter duration of untreated illness. Int J Clin Pract (2017) 71:e12926. doi: 10.1111/ijcp.12926
48. Seeley WW, Menon V, Schatzberg AF, Keller J, Glover GH, Kenna H, et al. Dissociable intrinsic connectivity networks for salience processing and executive control. J Neurosci (2007) 27:2349–56. doi: 10.1523/JNEUROSCI.5587-06.2007
49. Smith SM, Fox PT, Miller KL, Glahn DC, Fox PM, Mackay CE, et al. Correspondence of the brain’s functional architecture during activation and rest. Proc Natl Acad Sci U S A (2009) 106:13040–5. doi: 10.1073/pnas.0905267106
50. Grillner S, Hellgren J, Menard A, Saitoh K, Wikstrom MA. Mechanisms for selection of basic motor programs–roles for the striatum and pallidum. Trends Neurosci (2005) 28:364–70. doi: 10.1016/j.tins.2005.05.004
Keywords: major depressive disorder, affective network, repetitive transcranial magnetic stimulation, fMRI, amygdala
Citation: Chen F-j, Gu C-z, Zhai N, Duan H-f, Zhai A-l and Zhang X (2020) Repetitive Transcranial Magnetic Stimulation Improves Amygdale Functional Connectivity in Major Depressive Disorder. Front. Psychiatry 11:732. doi: 10.3389/fpsyt.2020.00732
Received: 24 March 2020; Accepted: 13 July 2020;
Published: 31 July 2020.
Edited by:
Ping Li, Qiqihar Medical University, ChinaReviewed by:
Jennifer Barredo, Providence VA Medical Center, United StatesQiwen Mu, North Sichuan Medical College, China
Katharine Dunlop, University of Toronto, Canada
Ephrem Takele Zewdie, University of Calgary, Canada
Copyright © 2020 Chen, Gu, Zhai, Duan, Zhai and Zhang. This is an open-access article distributed under the terms of the Creative Commons Attribution License (CC BY). The use, distribution or reproduction in other forums is permitted, provided the original author(s) and the copyright owner(s) are credited and that the original publication in this journal is cited, in accordance with accepted academic practice. No use, distribution or reproduction is permitted which does not comply with these terms.
*Correspondence: Fu-jian Chen, chen3165659@163.com ; Chuan-zheng Gu, guchuanzheng120@163.com; Xiao Zhang, woshixiao_81@163.com