- 1Centre & Discipline of Child and Adolescent Psychiatry, Psychosomatics and Psychotherapy, Faculty of Health and Medical Sciences, The University of Western Australia, Perth, WA, Australia
- 2Brain and Behaviour, Telethon Kids Institute, Perth, WA, Australia
- 3Specialised Child and Adolescent Mental Health Services, Department of Health, Perth, WA, Australia
- 4School of Psychological Sciences, Faculty of Science, The University of Western Australia, Perth, WA, Australia
- 5Community Child and Adolescent Mental Health Services, Department of Health, Perth, WA, Australia
- 6Department of Child and Adolescent Psychiatry, Psychosomatic Medicine and Psychotherapy, Jena University Hospital, Friedrich Schiller University, Jena, Germany
Serotonin (5-HT) is widely implicated as a key neurotransmitter relevant to a range of psychiatric disorders and psychological processes. The role of central nervous 5-HT function underlying these processes can be examined through serotonergic challenge methodologies. Acute tryptophan depletion (ATD) is a key challenge method whereby a diminished dietary intake of tryptophan—the amino acid precursor to brain 5-HT synthesis—results in temporary diminished central nervous 5-HT synthesis. While this particular methodology has been used in adult populations, it was only recently that modifications were made to enable the use of ATD in child and adolescent populations. Additionally, the Moja-De modification of the ATD challenge methodology has demonstrated benefits over other ATD techniques used previously. The aim of this protocol paper is to describe the ATD Moja-De methodology in detail, its benefits, as well as studies that have been conducted to validate the procedure in child and adolescent samples. The ATD Moja-De protocol provides a potential methodology for investigating the role of central nervous 5-HT via manipulation of brain tryptophan availability in human psychopathology from a developmental viewpoint.
Introduction
Serotonin (5-HT) is a neurotransmitter that is involved in a variety of psychiatric disorders, such as depressive disorders, anxiety disorders, and attention deficit hyperactivity disorder (ADHD). ADHD is a highly prevalent psychiatric disorder. Recent research has shown that, apart from changes in central nervous dopaminergic activity as well as other neurotransmitter systems (1) the underlying neurobiology of ADHD as a disorder is also subject to changes in 5-HT neurotransmission (2–9). Research on the role of the amino acid (AA) tryptophan (TRP, the physiological precursor of 5-HT) and related brain 5-HT synthesis) have used acute tryptophan depletion (ATD). ATD is a physiological serotonergic challenge procedure that has been widely used over the last decade to investigate the role of 5-HT in a variety of disorders, in both children and adolescents (10, 11), and adults (9, 12). Additionally, ATD allows investigations into the role of 5-HT on various behavioral and cognitive processes. ATD is a safe, transient method of reducing brain 5-HT synthesis by both reducing the central nervous availability of the 5-HT precursor AA TRP and increasing competition for the active transport mechanism that delivers TRP into the brain. In addition, the AAs administered stimulate protein synthesis in the liver, thereby taking TRP from plasma stores and subsequently contributing to the depletion or diminished availability of TRP across the blood brain barrier (BBB). These effects are temporary and are quickly reversed by resumption of a normal diet with adequate levels of TRP. Historically, several ATD protocols have been used since the technique was first applied in humans in 1977, and these methods have included a varying number and/or amount of AAs (13, 14). Until recently, however, there had been no safe and effective short-term neurochemical challenge procedure available to deplete brain 5-HT synthesis in young people, such as children and adolescents.
The more recent development of a new modification of an ATD procedure allows for this particular ATD protocol to be administered to children and adolescents in a safe and effective manner. This new body weight adapted ATD protocol is named Moja-De (a modification of the ATD protocol by Moja and colleagues), and it indirectly accounts for baseline levels of the 5-HT-precursor AA TRP because baseline TRP was shown to be correlated with body weight (15). This new technique therefore allows investigation of the effects of a short-term decrease in brain 5-HT synthesis in young people.
Acute Tryptophan Depletion and the Development of the Moja-De Protocol
ATD is based on the premise that the depletion of TRP, the physiological precursor AA of central nervous 5-HT synthesis, leads to depletion of central nervous 5-HT. TRP is an essential AA that mammals cannot synthesize and must be acquired from dietary sources. Dietary TRP can be directly used by the gut microbiota to synthesize ligands, kynurenine via the kynurenine pathway or peripheral 5-HT through TRP hydroxylase 1 enzyme in the enterochromaffin cells of the gastric mucosa. Thus, gut microbiota can serve as important modulators of TRP availability (4). Plasma TRP levels are influenced by the balance between the dietary intake of TRP and its removal from the plasma by protein synthesis. Most of the TRP in plasma is bound to albumin. Under normal physiological conditions the free TRP portion accounts for 10-15% of total TRP as shown by more recent research (16). The free TRP portion is available for transport into the central nervous system (CNS). Hence, central nervous TRP availability may be more accurately predicted by free TRP than total (free + protein bound) TRP plasma levels. TRP is transported across the BBB by an active transporter (L1), at which TRP and all other large neutral AAs (LNAAs: e.g., valine, leucine, isoleucine, methionine, phenylalanine, and tyrosine) also compete. Once in the brain, TRP is converted into 5-hydroxytryptophan (5-HTP) by the enzyme TRP hydroxylase 2 (the rate-limiting enzyme of central nervous 5-HT synthesis). 5-HTP is then decarboxylated by the enzyme aromatic acid decarboxylase to 5-HT.
There are three factors that are important in determining the rate of brain 5-HT synthesis, and thus three points at which brain 5-HT synthesis may be influenced. These three factors include: (1) The concentration of free TRP in the plasma, which is dependent on dietary intake and/or restriction of TRP, (2) the amount of free TRP that is able to cross the BBB via competition for the LNAA transporter with other AAs, (3) the inhibition of the TRP hydroxylase 2 enzyme, [e.g., by para-chlorophenylalanine (PCPA); a rate limiting enzyme for TRP hydroxylase 2, which therefore impacts on central 5-HT synthesis). Central nervous 5-HT synthesis can be influenced by interfering with any or all of these factors. However, the best ATD results in humans with regards to depletion magnitude were achieved using a combination of dietary restriction (point 1), and via methods to reduce competitive binding of TRP via the LNAA transporter and across the BBB (point 2). Consequently, the ATD technique uses a combination of a low TRP diet and a TRP-deficient protein load containing large amounts of the other LNAAs to produce maximal central nervous TRP depletion (i.e., the administered AAs compete with endogenous TRP on the uptake into the brain over the BBB). A further mechanism that impacts brain TRP availability in the brain is passive diffusion, in both directions, across the BBB. However, this particular mechanism only contributes to a rather small degree (17–19).
The 7-amino-acid ATD mixture as described by Moja et al. (20), was associated with a marked reduction in plasma TRP levels. This protocol was later modified for administration to young people (coined Moja-De, with “De” being related to the last name of Professor Lothar Demisch who suggested and developed this ATD modification) with a body-weight-adapted dosing scheme, and a lower amount of methionine relative to conventional mixtures. The reduction in methionine was to reduce unwanted side effects, such as vomiting and nausea, that were frequently observed in adult populations using alternative formulations (21).
The ATD Moja-De protocol administers AA within an aqueous suspension, in which the relevant AA quantities are dosed in accordance with the participants’ body weights. The AA quantities of the ATD Moja-De beverage are (dosage per 10 kg of body weight): L-phenylalanine (PHE 1.32 g), L-leucine (LEU 1.32 g), L-isoleucine (ILE 0.84 g), L-methionine (MET 0.5 g), L-valine (VAL 0.96 g), L-threonine (THR 0.6 g), and L-lysine (LYS 0.96 g). The TRP Balanced Control (BAL) beverage contains the same AA quantities with an additional 0.7 g of L-TRP per 10 kg of body weight. The chemical properties and descriptions of the eight AAs used as part of the ATD Moja-De and the BAL protocol are summarized in Table 1. Table 1 additionally shows the AAs that comprise the ATD Moja-De mixture (AAs 1 to 7) as well as the amounts of each AA in grams per 10 kg of body weight, as per the body weight adapted protocol. The final beverage composition is included, including excipients. Table 1 also shows AAs that comprise the BAL mixture, which differs from the ATD mixture only with the addition of the AA L-TRP. The final beverage composition is also included. Specific manufacturing, packaging, and storage details are also provided.
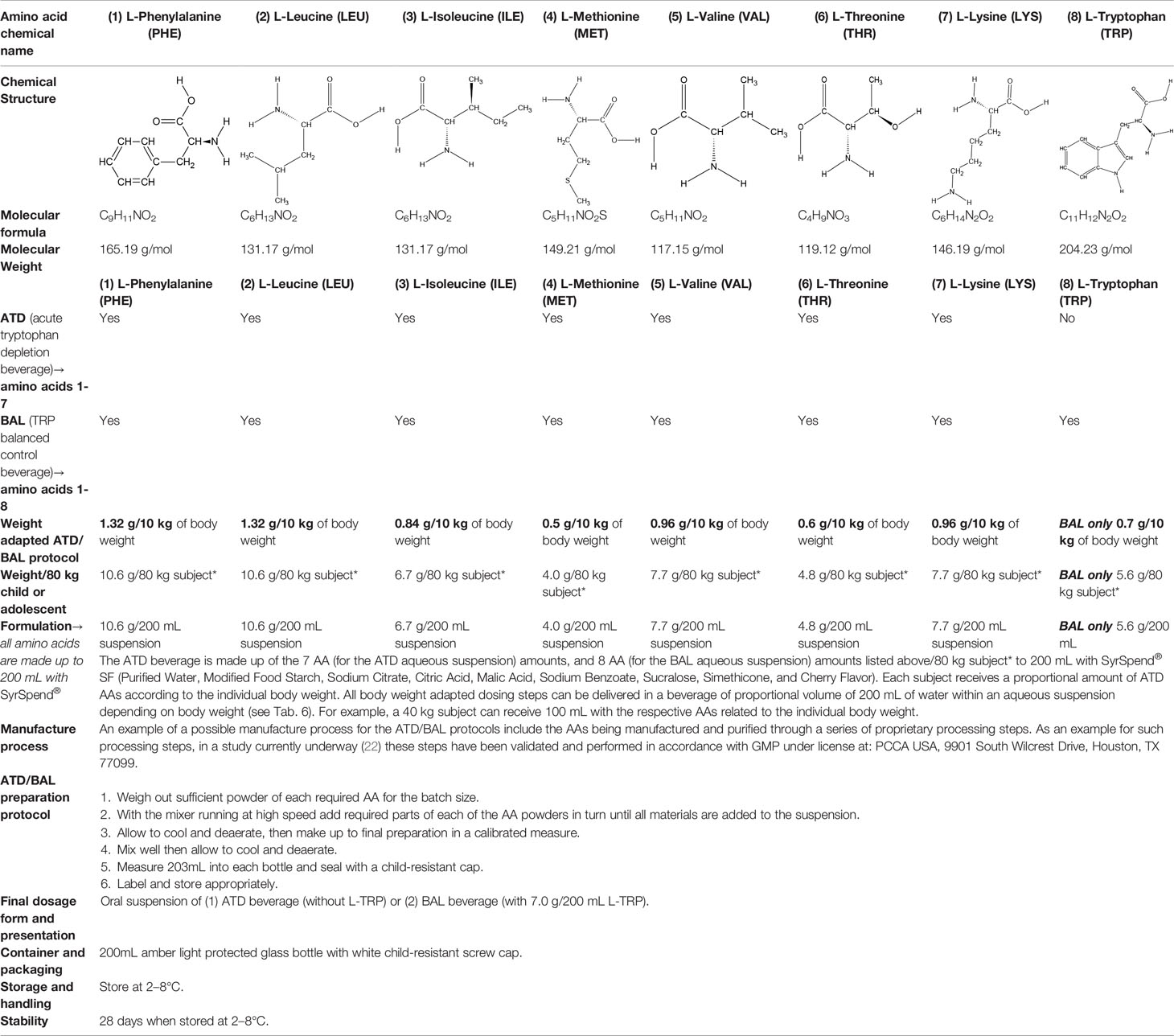
Table 1 Chemical properties and descriptions of the AAs and amounts (grams/10kg body weight) that make up the ATD Moja-De and BAL mixture and a brief description regarding manufacturing, packaging and storage details for the AA.
Amino Acids Used in the Moja-De ATD Mixture:
L-Phenylalanine
L-Phenylalanine (PHE) is an essential aromatic AA in humans (provided by the diet/ood). PHE plays a key role in the biosynthesis of other AAs, and is important in the structure and function of many proteins and enzymes. PHE is converted into tyrosine, which is used in the biosynthesis of dopamine and norepinephrine neurotransmitters. PHE is an odorless white crystalline powder, with a slightly bitter taste and a pH (1% aqueous solution) of 5.4 to 6. It has a water solubility of 26900 mg/L at 25°C.
Leucine
Leucine (LEU) is one of nine essential AAs in humans (provided by the diet/food). LEU is important for protein synthesis and many metabolic functions. LEU contributes to regulation of blood-sugar levels; growth and repair of muscle and bone tissue; growth hormone production; and wound healing. LEU is available in many foods and diets, and deficiency is rare. LEU is an odorless white crystalline powder, with a water solubility of 21,500 mg/L at 25°C.
L-Isoleucine
L-Isoleucine (ILE) is one of nine essential AAs in humans (present in dietary proteins). L-ILE is a branched-chain aliphatic AA found in many proteins, and it is an isomer of LEUCINE. ILE has diverse physiological functions, such as assisting wound healing, detoxification of nitrogenous wastes, stimulating immune function, and promoting secretion of several hormones. Necessary for hemoglobin formation and regulating blood sugar and energy levels, ILE is concentrated in muscle tissues in humans. ILE is an odorless white crystalline powder, with a bitter taste and a water solubility of 34,400 mg/L at 25°C.
L-Methionine
L-Methionine (MET) is a further example of nine essential AAs in humans (provided by the diet/food), MET is required for growth and tissue repair. A sulphur-containing AA, MET improves the tone and pliability of skin, hair, and strengthens nails. Involved in many detoxifying processes, sulphur provided by MET protects cells from pollutants, slows cell aging, and is essential for absorption and bio-availability of selenium and zinc. MET chelates heavy metals, such as lead and mercury, aiding their excretion. It also acts as a lipotropic agent, and prevents excess fat buildup in the liver. MET is a white crystalline powder with a faint odor and sulphurous taste. It has a pH of (1% aqueous solution) of 5.6 to 6.1, and a water solubility of 56,600 mg/L at 25°C.
L-Valine
L-Valine (VAL) is an aliphatic and extremely hydrophobic essential AA in humans related to LEU and found in many proteins, mostly in the interior of globular proteins helping to determine three-dimensional structure. A glycogenic AA, VAL maintains mental vigor, muscle coordination, and emotional calm. VAL is obtained from soy, cheese, fish, meats, and vegetables, and VAL supplements are used for muscle growth, tissue repair, and energy. VAL is a white crystalline powder, and has a water solubility of 58,500 mg/L at 25°C.
L-Threonine
L-Threonine (THR) is an essential AA in humans (provided by food) and is an important residue of many proteins, such as tooth enamel, collagen, and elastin. It is an important AA for the nervous system, and also plays an important role in porphyrin and fat metabolism and prevents fat buildup in the liver. THR is a white crystalline powder, and has a water solubility of 97,000 mg/L at 25°C.
L-Lysine
L-Lysine (LYS) is one of nine essential AAs in humans required for growth and tissue repair, and is supplied by many foods and diets, especially red meats, fish, and dairy products. LYS is a colorless crystalline powder with a sweet/bitter taste. It has a water solubility of 1,000,000 mg/L at 25°C.
L-Tryptophan
L-Tryptophan (TRP) is the least plentiful of all 22 AAs and an essential AA in humans (provided by the diet/food), TRP is found in most proteins and a precursor of 5-HT. TRP is converted to 5-hydroxy-tryptophan (5-HTP), which in turn is converted to 5-HT, a neurotransmitter essential in regulating appetite, sleep, mood, and pain. TRP is present in dairy products, meats, brown rice, fish, and soybeans. TRP is an odorless white to slightly yellowish-white crystalline powder, with a slightly bitter taste. It has a water solubility of 13,400 mg/L at 25°C. A 1% solution in water has a pH of 5.5 to 7.
In the administration of the ATD Moja-De Protocol, the aqueous suspension is often administered to young people following an overnight protein fast. The protein fast decreases the dietary intake of TRP, and the LNAAs in the beverage compete with endogenous TRP for uptake into the CNS over the BBB. This subsequently leads to decreased substrate availability for central nervous 5-HT synthesis for a short period of time (approx. 5–7 h). In addition, the administered AAs stimulate protein synthesis in the liver, which takes additional TRP from plasma stores and also contributes to the depletion. The BAL beverage consists of all eight AAs, and causes no significant alteration in brain 5-HT synthesis, as supported by research conducted in rodents (23).
Validation of the Atd Moja-De/Bal Body Weight-Adapted Protocol
Animal Models
There are two animal model studies that have successfully validated the ATD Moja-De/BAL body weight adapted protocol (23, 24). Biskup & colleagues (23) validated a refined body weight adapted ATD protocol called Moja-De in two strains of mice, while Sanchez & colleagues (24) examined the neurochemical effects of three developed formulas (ATD Moja-De for 5-HT, phenylalanine/tyrosine depletion or PTD for dopamine (DA) and a combined monoamine depletion mixture or CMD) on brain 5-HT and DA function in mice. Both studies concluded that the Moja-De protocol lowered brain TRP and significantly decreased central nervous 5-HT synthesis.
Human Validation Studies
There are eight individual study sample populations that have used the ATD Moja-De protocol across healthy and clinical populations of adults and adolescents, and these have generated other associated research papers (see Table 2). Given Moja-De’s very likely changed side effect profile compared to other ATD mixtures (which is presumably related to a lower concentration of methionine and the administration of a body weight adjusted ATD protocol and amount of AAs, as well as an overall reduced total amount of AA’s), it is the only ATD protocol so far that has been used in children and adolescents. Across these studies, the ATD Moja-De protocol has been well tolerated overall, with limited side effects/adverse events, and very few drop-outs in studies. In addition, the body-weight-adjusted ATD protocol has been proven to successfully lead to TRP depletion and a decreased CNS of 5-HT, which has enabled Moja-De to be a valid tool to explore the central nervous serotonergic system and related aspects of TRP synthesis. Many of the studies using the Moja-De ATD modification focused on ADHD or ADHD-related symptoms. The following table summarises the findings so far.
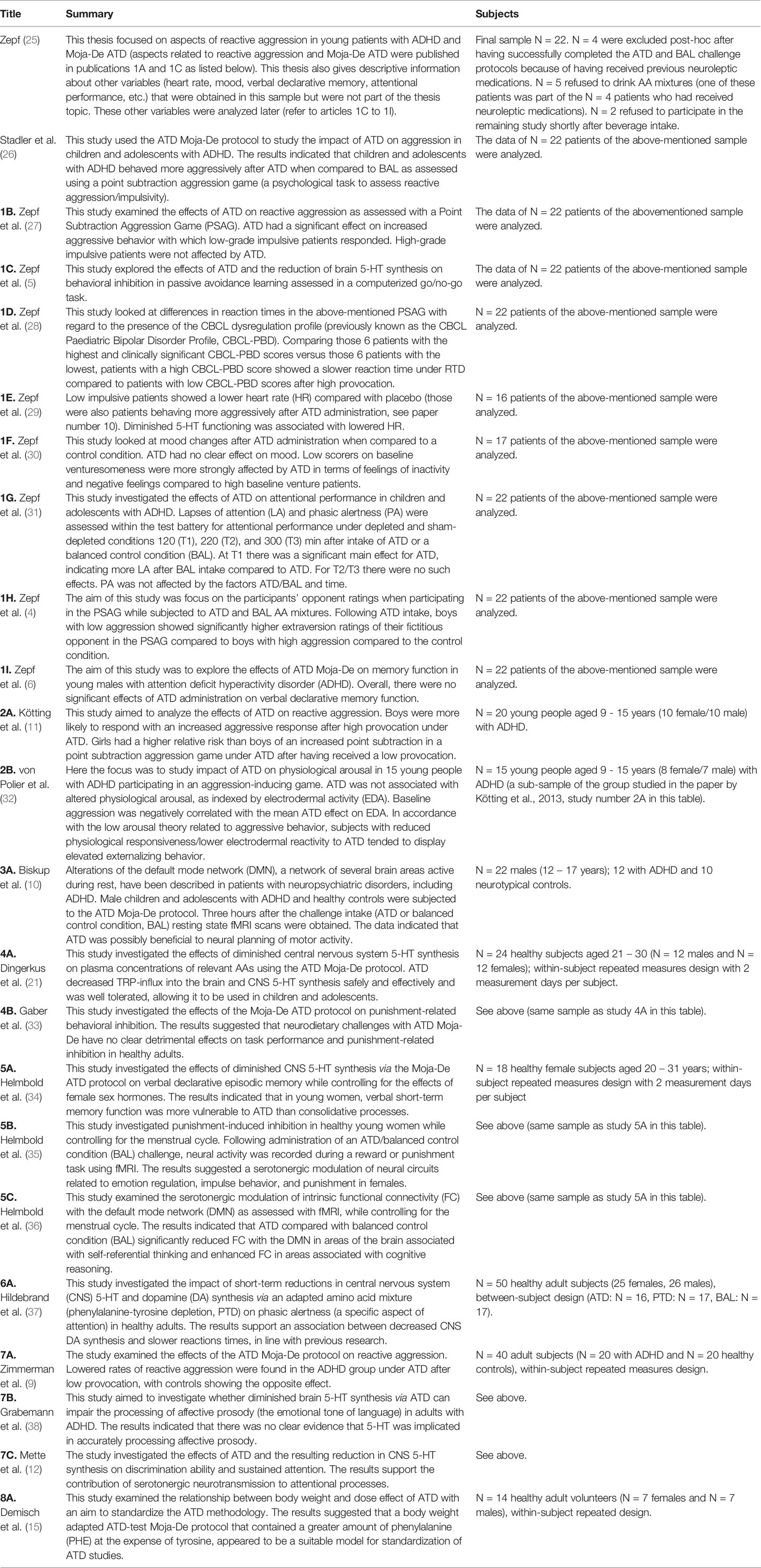
Table 2 Summary of studies employing the ATD Moja-De Protocol in healthy and clinical populations (young people and adults).
Contraindications and Side Effects
The ATD Moja-De protocol should be administered according to the individual body weight, and the studies above demonstrate safety, in addition to depletion-related efficacy and/or behavioral effect-related efficacy (for example, effects on behavioral parameters like reactive aggressive behaviors). The AAs contained in the ATD and the BAL beverages are derived from a normal diet. The beverages are contraindicated for use in participants with known hypersensitivity to the active substance or any of the excipients, as well as in participants with known changes in, or disorders of AA metabolism and participants with psychotic features. Every use in human populations needs to be carefully evaluated (risk vs. benefits analysis) before the beverages are used.
The known side effects of acute ATD Moja-De are mild and short-lived and include an unpleasant taste, nausea, and/or vomiting (22) and lowered mood (27). These are expected to be transient but nevertheless, clear risk mitigation strategies should be in place, such as the administration of a TRP replacement meal following the ATD Moja-De protocol.
Discussion
ATD protocols other than Moja-De have been used to investigate central nervous 5-HT function of healthy subjects and across a range of diverse clinical populations, particularly in adults (39). The ATD Moja-De protocol was adapted from the seven AA ATD mixture formulated by Moja et al. to account for the individual’s body weight (20), and the Moja-De AA mixture also contains AA’s with a high affinity to the L-1 transporter at the BBB, thus allowing efficient competitive antagonism with regards to central nervous TRP uptake. This relatively new ATD protocol indirectly accounts for baseline levels of TRP, the precursor for central nervous 5-HT. Additionally, the Moja-De protocol contains a lower amount of methionine (which contains sulphur) and also an overall smaller amount of AA’s compared to other ATD protocols, and this has likely reduces side-effects such as vomiting and nausea (21). Combined, adjusting for body weight, reducing the overall amount of AAs, and reducing methionine levels has led to the development of a safe and effective neurochemical challenge procedure for the use in young people.
Administration of the ATD Moja-De protocol in both animal and human models of neuropsychiatric disorders have consistently demonstrated lowered brain TRP and significantly decreased central nervous 5-HT synthesis. Given this, the ATD Moja-De protocol can be further extended and applied to investigate central nervous 5-HT functioning in related neuropsychiatric disorders as well as behavioral, physiological, and neuropsychological processes in young people. A specific example includes the ATD Moja-De protocol being employed as a method to investigate predictors of treatment response to selective serotonin reuptake inhibitor (SSRI) administration in adolescents with major depressive disorder (22).
Ethics Statement
This is a methods paper that reviewed a series of studies. Please see each individual paper for the name and affiliation of the respective ethics committee that approved each individual study.
Author Contributions
FZ conceptualized this manuscript and was significantly involved in the evaluation of the protocol and subsequent studies. FZ, RS, JW, KR, PR, SM, and HM contributed to the writing of this manuscript.
Conflict of Interest
In the last 10 years, FZ was the recipient of an unrestricted award donated by the American Psychiatric Association (APA), the American Psychiatric Institute for Research and Education (APIRE), and AstraZeneca (Young Minds in Psychiatry Award). FZ has also received research support from the European Union, German Federal Ministry for Economics and Technology, the German Society for Social Paediatrics and Adolescent Medicine, the Paul and Ursula Klein Foundation, the Dr. August Scheidel Foundation, the IZKF fund of the University Hospital of RWTH Aachen University, the Telethon Perth Children’s Hospital Research Fund (TPCHR); the Princess Margaret Foundation, and a travel stipend donated by the GlaxoSmithKline Foundation. FZ was the recipient of an unrestricted educational grant, travel support, and speaker honoraria by Shire Pharmaceuticals, Germany. In addition, FZ has received support from the Raine Foundation for Medical Research (Raine Visiting Professorship), and editorial fees from Co-Action Publishing (Sweden)/Taylor & Francis Publishing (USA).
The remaining authors declare that the research was conducted in the absence of any commercial or financial relationships that could be construed as a potential conflict of interest.
Acknowledgments
We would like to take the opportunity and thank all study participants and their families for their involvement. In addition, we would like to thank all involved team members for their work in the above-mentioned studies. Finally, we would like to thank the respective funding agencies of the above-mentioned studies.
References
1. Fernández-Jaén A, López-Martín S, Albert J, Fernández-Mayoralas DM, Fernández-Perrone AL, de La Peña MJ, et al. Cortical thickness differences in the prefrontal cortex in children and adolescents with ADHD in relation to dopamine transporter (DAT1) genotype. Psychiatry Res: Neuroimaging (2015) 233(3):409–17. doi: 10.1016/j.pscychresns.2015.07.005
2. Park S, Lee J-M, Kim J-W, Cho D-Y, Yun H, Han D, et al. Associations between serotonin transporter gene (SLC6A4) methylation and clinical characteristics and cortical thickness in children with ADHD. Psychol Med (2015) 45(14):3009–17. doi: 10.1017/S003329171500094X
3. Hou Y-w, Xiong P, Gu X, Huang X, Wang M, Wu J. Association of serotonin receptors with attention deficit hyperactivity disorder: a systematic review and meta-analysis. Curr Med Sci (2018) 38(3):538–51. doi: 10.1007/s11596-018-1912-3
4. Zepf F, Gaber T, Bubenzer S, Baurmann D, Helmbold K, Vloet T, et al. Reduced central nervous 5-HT neurotransmission in youth with ADHD influences ratings of a virtual opponentsextraversion aâ, “Effects of trait-aggression. J Pediatr Sci (2011) 3(2):1–12. doi: 10.1002/hup.1002
5. Zepf F, Holtmann M, Stadler C, Demisch L, Schmitt M, Wöckel L, et al. Diminished serotonergic functioning in hostile children with ADHD: tryptophan depletion increases behavioural inhibition. Pharmacopsychiatry (2008) 41(02):60–5. doi: 10.1055/s-2007-1004593
6. Zepf F, Landgraf M, Biskup C, Dahmen B, Poustka F, Wöckel L, et al. No effect of acute tryptophan depletion on verbal declarative memory in young persons with ADHD. Acta Psychiatr Scand (2013) 128(2):133–41. doi: 10.1111/acps.12089
7. Zepf F, Poustka F. 5-HT functioning and aggression in children with ADHD and disruptive behaviour disorders. Hum Psychopharmacol: Clin Exp (2008) 23(5):438–. doi: 10.1002/hup.948
8. Zepf F, Stadler C, Demisch L, Schmitt M, Landgraf M, Poustka F. Serotonergic functioning and trait-impulsivity in attention-deficit/hyperactivity-disordered boys (ADHD): influence of rapid tryptophan depletion. Hum Psychopharmacol: Clin Exp (2008) 23(1):43–51. doi: 10.1002/hup.896
9. Zimmermann M, Grabemann M, Mette C, Abdel-Hamid M, Ueckermann J, Kraemer M, et al. The effects of acute tryptophan depletion on reactive aggression in adults with attention-deficit/hyperactivity disorder (ADHD) and healthy controls. PloS One (2012) 7(3):e32023. doi: 10.1371/journal.pone.0032023
10. Biskup C, Helmbold K, Baurmann D, Klasen M, Gaber T, Bubenzer-Busch S, et al. Resting state default mode network connectivity in children and adolescents with ADHD after acute tryptophan depletion. Acta Psychiatr Scand (2016) 134(2):161–71. doi: 10.1111/acps.12573
11. Kötting W, Bubenzer S, Helmbold K, Eisert A, Gaber T, Zepf F. Effects of tryptophan depletion on reactive aggression and aggressive decision-making in young people with ADHD. Acta Psychiatr Scand (2013) 128(2):114–23. doi: 10.1111/acps.12001
12. Mette C, Zimmermann M, Grabemann M, Abdel-Hamid M, Uekermann J, Biskup C, et al. The impact of acute tryptophan depletion on attentional performance in adult patients with ADHD. Acta Psychiatr Scand (2013) 128(2):124–32. doi: 10.1111/acps.12090
13. Young SN. Acute tryptophan depletion in humans: a review of theoretical, practical and ethical aspects. J Psychiatry Neurosci: JPN (2013) 38(5):294. doi: 10.1503/jpn.120209
14. Hood SD, Bell CJ, Nutt DJ. Acute tryptophan depletion. part I: rationale and methodology. Aust New Zealand J Psychiatry (2005) 39(7):558–64. doi: 10.1080/j.1440-1614.2005.01627.x
15. Demisch L, Kewitz A, Schmeck K, Sadigorsky S, Barta S, Dierks T, et al. Methodology of rapid tryptophan depletion (RTD): impact of gender and body weight. Eur Arch Psychiatry Clin Neurosci (2002) 252(Suppl 2):I/25.
16. Comai S, Bertazzo A, Brughera M, Crotti S. Tryptophan in Health and Disease. Advances in Clinical Chemistry. G. Makowski. (Global, Elsevier) (2019). 95, 232.
17. D’Mello JF. Amino acids in animal nutrition. (United Kingdom: CABI Publishing) (2003). doi: 10.1079/9780851996547.0000
18. Kewitz A. Biochemische untersuchungen zur optimierung des “Rapid Tryptophan Depletion-Test” (RTD)—-eine physiologische methode zur akuten verminderung der zentralnervösen serotonin-synthese in der psychobiologischen forschun. Johann Wolfgang Goethe-Universität;: Frankfurt am Main, Germany (2002).
19. Zepf FD. Principles of rapid tryptophan depletion and its use in research on neuropsychiatric disorders. Amino Acids Hum Nutr Health (2012), 418–26. doi: 10.1079/9781845937980.0418
20. Moja EA, Stoff DM, Gessa GL, Castoldi D, Assereto R, Tofanetti O. Decrease in plasma tryptophan after tryptophan-free amino acid mixtures in man. Life Sci (1988) 42(16):1551–6. doi: 10.1016/0024-3205(88)90013-6
21. Dingerkus V, Gaber T, Helmbold K, Bubenzer S, Eisert A, Sánchez C, et al. Acute tryptophan depletion in accordance with body weight: influx of amino acids across the blood–brain barrier. J Neural Transm (2012) 119(9):1037–45. doi: 10.1007/s00702-012-0793-z
22. Stewart RM, Hood SD, Rao P, Moore JK, Runions KC, Murphy SE, et al. Using acute tryptophan depletion to investigate predictors of treatment response in adolescents with major depressive disorder: study protocol for a randomised controlled trial. Trials (2018) 19(1):434. doi: 10.1186/s13063-018-2791-4
23. Biskup CS, Sánchez CL, Arrant A, Van Swearingen AE, Kuhn C, Zepf FD. Effects of acute tryptophan depletion on brain serotonin function and concentrations of dopamine and norepinephrine in C57BL/6J and BALB/cJ mice. PLoS One (2012) 7(5):e35916. doi: 10.1371/journal.pone.0035916
24. Sánchez CL, Van Swearingen AE, Arrant AE, Kuhn CM, Zepf FD. Dietary manipulation of serotonergic and dopaminergic function in C57BL/6J mice with amino acid depletion mixtures. J Neural Transm (2014) 121(2):153–62. doi: 10.1007/s00702-013-1083-0
25. Zepf F. Untersuchung zentralnervöser serotonerger Funktionen mit Hilfe des »Rapid Tryptophan Depletion-Test« (RTD) bei männlichen Kindern und Jugendlichen mit Aufmerksamkeits- Defizit/Hyperaktivitäts Syndrom (ADHS) –Einfluss einer akut verminderten Serotoninsynthese auf laborexperimentelle Impulsivität und Aggression. Germany (2008).
26. Stadler C, Zepf FD, Demisch L, Schmitt M, Landgraf M, Poustka F. Influence of rapid tryptophan depletion on laboratory-provoked aggression in children with ADHD. Neuropsychobiology (2007) 56(2-3):104–10. doi: 10.1159/000112951
27. Zepf FD, Stadler C, Demisch L, Schmitt M, Landgraf M, Poustka F. Serotonergic functioning and trait-impulsivity in attention-deficit/hyperactivity-disordered boys (ADHD): influence of rapid tryptophan depletion. Hum Psychopharmacol: Clin Exp (2008) 23(1):43–51. doi: 10.1002/hup.896
28. Zepf F, Wöckel L, Poustka F, Holtmann M. Diminished 5-HT functioning in CBCL pediatric bipolar disorder-profiled ADHD patients versus normal ADHD: susceptibility to rapid tryptophan depletion influences reaction time performance. Hum Psychopharmacol: Clin Exp (2008) 23(4):291–9. doi: 10.1002/hup.934
29. Zepf FD, Holtmann M, Stadler C, Wöckel L, Poustka F. Reduced serotonergic functioning changes heart rate in ADHD. J Neural Transm (2009) 116(1):105–8. doi: 10.1007/s00702-008-0146-0
30. Zepf FD, Holtmann M, Stadler C, Magnus S, Wöckel L, Poustka F. Diminished central nervous 5-HT neurotransmission and mood self-ratings in children and adolescents with ADHD: no clear effect of rapid tryptophan depletion. Hum Psychopharmacol: Clin Exp (2009) 24(2):87–94. doi: 10.1002/hup.1002
31. Zepf F, Gaber T, Baurmann D, Bubenzer S, Konrad K, Herpertz-Dahlmann B, et al. Serotonergic neurotransmission and lapses of attention in children and adolescents with attention deficit hyperactivity disorder: availability of tryptophan influences attentional performance. Int J Neuropsychopharmacol (2010) 13(7):933–41. doi: 10.1017/S146114571000012X
32. Von Polier G, Biskup C, Kötting W, Bubenzer S, Helmbold K, Eisert A, et al. Change in electrodermal activity after acute tryptophan depletion associated with aggression in young people with attention deficit hyperactivity disorder (ADHD). J Neural Transm (2014) 121(4):451–5. doi: 10.1007/s00702-013-1119-5
33. Gaber TJ, Dingerkus VL, Crockett MJ, Bubenzer-Busch S, Helmbold K, Sánchez CL, et al. Studying the effects of dietary body weight-adjusted acute tryptophan depletion on punishment-related behavioral inhibition. Food Nutr Res (2015) 59(1):28443. doi: 10.3402/fnr.v59.28443
34. Helmbold K, Bubenzer S, Dahmen B, Eisert A, Gaber T, Habel U, et al. Influence of acute tryptophan depletion on verbal declarative episodic memory in young adult females. Amino Acids (2013) 45(5):1207–19. doi: 10.1007/s00726-013-1582-1
35. Helmbold K, Zvyagintsev M, Dahmen B, Bubenzer-Busch S, Gaber T, Crockett M, et al. Effects of serotonin depletion on punishment processing in the orbitofrontal and anterior cingulate cortices of healthy women. Eur Neuropsychopharmacol (2015) 25(6):846–56. doi: 10.1016/j.euroneuro.2015.02.007
36. Helmbold K, Zvyagintsev M, Dahmen B, Biskup C, Bubenzer-Busch S, Gaber T, et al. Serotonergic modulation of resting state default mode network connectivity in healthy women. Amino Acids (2016) 48(4):1109–20. doi: 10.1007/s00726-015-2137-4
37. Hildebrand P, Königschulte W, Gaber TJ, Bubenzer-Busch S, Helmbold K, Biskup CS, et al. Effects of dietary tryptophan and phenylalanine–tyrosine depletion on phasic alertness in healthy adults–a pilot study. Food Nutr Res (2015) 59(1):26407. doi: 10.3402/fnr.v59.26407
38. Grabemann M, Mette C, Zimmermann M, Heinrich V, Uekermann J, Wiltfang J, et al. No clear effects of acute tryptophan depletion on processing affective prosody in male adults with ADHD. Acta Psychiatr Scand (2013) 128(2):142–8. doi: 10.1111/acps.12130
Keywords: acute tryptophan depletion, ATD, Moja-De, central nervous 5-HT, serotonin challenge procedure, ATD Moja-De in child and adolescent populations, attention deficit hyperactivity disorder
Citation: Stewart RM, Wong JWY, Mahfouda S, Morandini HAE, Rao P, Runions KC and Zepf FD (2020) Acute Tryptophan Depletion Moja-De: A Method to Study Central Nervous Serotonin Function in Children and Adolescents. Front. Psychiatry 10:1007. doi: 10.3389/fpsyt.2019.01007
Received: 15 September 2019; Accepted: 20 December 2019;
Published: 06 March 2020.
Edited by:
Hans Willi Clement, University of Freiburg, GermanyReviewed by:
Stefano Comai, Vita-Salute San Raffaele University, ItalyDietmar Fuchs, Innsbruck Medical University, Austria
Copyright © 2020 Stewart, Wong, Mahfouda, Morandini, Rao, Runions and Zepf. This is an open-access article distributed under the terms of the Creative Commons Attribution License (CC BY). The use, distribution or reproduction in other forums is permitted, provided the original author(s) and the copyright owner(s) are credited and that the original publication in this journal is cited, in accordance with accepted academic practice. No use, distribution or reproduction is permitted which does not comply with these terms.
*Correspondence: Florian D. Zepf, Zmxvcmlhbi56ZXBmQG1lZC51bmktamVuYS5kZQ==