- 1Department of Neurology, Xuan Wu Hospital Capital Medical University, Beijing, China
- 2Department of Neurology, Third Affiliated Hospital, Beijing University of Chinese Medicine, Beijing, China
- 3Department of Clinical Psychology, Beijing Anding Hospital, Beijing, China
- 4Nanjing Municipal Hospital of Traditional Chinese Medicine, Nanjing, China
- 5Department of Neurology, Fengtai Integrated Chinese and Western Medicine Hospital of Beijing, Beijing, China
- 6Department of Radiology, Guang’ anmen Hospital China Academy of Chinese Medical Sciences, Beijing, China
- 7Department of Psychiatry and Behavioral Sciences, Johns Hopkins University School of Medicine, Baltimore, MD, United States
Childhood Maltreatment (CM) is an important risk factor for major depressive disorder (MDD). Previous studies using emotional task-state functional magnetic resonance (task-state fMRI) found that altered brain function in prefrontal-limbic regions was the key neuropathological mechanism in adult MDD patients with experience of early-life maltreatment. However, to the best of our knowledge, there is no published study investigating brain function in MDD patients with CM experience using resting-state fMRI (rs-fMRI). In present study, we aimed to detect altered resting-state brain activity in MDD patients with CM experience, and identify significantly activated brain regions, which may provide new insights into the neural mechanism underlying the relationship between MDD and CM experience. The results showed MDD patients with CM experience were associated with increased amplitude of low-frequency fluctuation (ALFF) and altered function connection (FC) in the prefrontal cortex, when compared to MDD patients without CM. Of note, left frontal middle gyrus (LFEG) was found as a specific brain region which differentiates MDD patients with CM from patients without CM. These results suggest that rs-fMRI is a useful method in studying the correlation between MDD and CM experience and altered function of LFEG in resting-state may explain the correlation between MDD and CM experience.
Introduction
Major depressive disorder (MDD) has become the single largest contributor to nonfatal health loss globally in 2015 (1). The causality of MDD is heterogeneous. Although stress, poor family relationship and social support in adulthood have been thoroughly studied as environmental risks for MDD, unpleasant psychical or physical experiences during childhood were often overlooked. Childhood maltreatment (CM) has recently gained greater attention because it may confer susceptibility to depression in later-life. Clinical evidence from retrospective and prospective cohort studies suggests that CM could markedly increase the risk of MDD (2–5). Furthermore, it has been reported that approximately 65% of chronically depressed patients have a history of CM, which is associated with more relapses and heightened therapy resistance (6, 7). Therefore, it is important to identify the neural mechanisms underlying the impact of CM on MDD pathophysiology, for pursuing early intervention and mechanism-based treatment strategies.
CM has been proved to affect brain function and development in MDD patients (8–11). Task- and resting-state functional magnetic resonance imaging (fMRI) has been wildly used to non-invasively evaluate functional brain activity for identification of specific brain regions and neural circuits associated with disease conditions. Previous studies using emotional task-state Fmri (12–15) showed altered activation of prefrontal-limbic regions, including ventromedial prefrontal cortex (vmPFC), anterior cingulate cortex (ACC), amygdale and hippocampus, in MDD patients with CM.
Nonetheless, to our best knowledge, resting-state brain function in MDD patients with CM still remains to be investigated. In the present study, we utilized rs-fMRI to examine the neuropathological mechanisms of drug-naïve MDD with CM experience. We hypothesize that altered blood oxygenation level dependency (BOLD) in certain brain regions may be correlated with CM experience in MDD patients and these brain areas may include subregions of the prefrontal-limbic system, which has previously been reported to be associated with MDD patients with CM using task-state fMRI.
Methods
Participants
Fifteen MDD patients with CM and fifteen patients without CM were recruited from the outpatient clinic of Xuanwu Hospital Capital Medical University, Third Affiliated Hospital of Beijing University of Chinese Medicine, and Beijing Anding Hospital. All of the patients were diagnosed with modified structure clinical interview for DSM-V (16) by two senior clinical psychiatrists, and were rated with a 17-item Hamilton depression scale (HAMD). All MDD patients were drug-naïve and in their first episode of illness. These patients were right-handed and would be excluded if they had another major psychiatric illness, neurological illness, head injury, alcohol or drug abuse. CM was assessed by a short form childhood trauma questionnaire (CTQ-SF) (17).
Seventeen age-, gender- and education-matched healthy controls (HC) were recruited from community-based advertising through flyers posted at hospital and university campuses. They were also interviewed with the Structured Clinical Interview for DSM-V. All HC were right-handed, free of depression and any other psychiatric or neurological illness and had no history of head injury, alcohol or drug abuse.
Data Acquisition
T1-weighted and resting-state fMRI data were acquired using a 3T Siemens Trio scanner (Magnetom Allegra, Siemens, Erlangen, Germany) in the Beijing Guang’ anmen Hospital China Academy of Chinese Medical Sciences. The scanning sessions included the following: (i) three-dimensional T1-weighted whole-brain images: 3D-MPRAGE sequence, Repetition Time (TR)/Echo Time (TE) = 2300/3ms, 176 sagittal slices. (ii) Rs-fMRI scans contain 180 functional volumes, using a T2-weigthed Echo Planar Imaging sequence, TR/TE = 2,000/30 ms, flip angle = 90°, acquisition matrix = 64 × 64 axial slices = 40, thickness/gap = 3/0 mm, Voxel size: 3.0 × 3.0 × 3.0mm (3), Field of view = 210 × 210 mm. During the scanning, subjects laid supine in the scanner with their heads fixed with foam pads to decrease head motion. They were informed to close their eyes but remain awake, and a simple inquiry was conducted to exclude any sleeping periods.
Pre-Processing
Image preprocessing and statistical analysis were performed using the Data Processing Assistant for Resting-state fMRI (DPARSF, http://www.rfmri.org/DPARSF) toolkits (18), Resting State fMRI Data Analysis Toolkit 1.8 version (REST, https://www.nitrc.org/projects/rest/) (19) and SPM8 software (SPM8, http://www.fil.ion.ucl.ac.uk/spm/) (20).
Images were drafted by REST and BrainNet Viewer toolkit. Data pre-processing was performed by DPARSF toolkits. The steps were as follows: (i) Raw DICOM data were converted to the NifTI format; (ii) To allow for instrumental stabilization of the initial signal, first 10 images were discarded; (iii) Images were slice-timing and 3D motion corrected for head motions, we excluded images if patients’ and HC’s head movement data in translational and rotational planes i.e. exceeded 2mm or 2° and 1mm or 1°; (iv) Images were normalized based on the Montreal Neurological Institute (MNI) Space with Smoothing Method (Full Width at Half Maximum, FWHM 4mm); (v) rs-fMRI data were processed with linear detrending and band-pass filtering.
ALFF and FC Analysis
After pre-processing, very low-frequency drift and high-frequency noise was first filtered (band-pass, 0.01∼0.08Hz), and then a Fast Fourier Transform (FFT) was used to convert the frequency domain. This averaged square root was termed Amplitude of Low-Frequency Fluctuation (ALFF) at the given voxel (21). Furthermore, in order to eliminate the physiological signals, fractional ALFF (fALFF) was also performed. In the following FC analysis, according to our present results and referenced by previous CM task-fMRI study results (22), the left orbital part of inferior frontal gyrus, left anterior cingulated and paracingulate gyri, left middle frontal gyrus and left inferior parietal, extending to supramarginal and angular gyri, were chosen as a region of interest (ROI). After, seed-to-voxel functional connectivity was performed.
Statistical Analysis
Subjects’ demographic information, including age, gender, education level, and their matched HC groups were analyzed by One-way ANCOVA. Gender related differences were detected by Chi-square tests. ALFF, fALFF and FC results were performed in correlation with CM scale using Pearson Correlation. The statistical significance level was set at p < 0.05. All statistical tests were performed using SPSS 18.0 (SPSS Inc., IL, USA).
The technologists who performed fMRI data analysis were blind to the subjects. Significant brain activation in the whole brain was computed using one-sample t-test in REST (Threshold p < 0.05) for every group. Voxel-wise group comparisons were detected with two-sample t-test (AlphaSim correction p < 0.01; continuous voxels > 16). The precise anatomical position in the brain, with statistical significance on the corresponding MNI coordinate, was identified using the Viewer in REST. Voxel-wise FC analyses revealed the Pearson correlation coefficients between the seeds and the rest of the whole brain areas. Fisher r-to-z transformation were used to transform FC values into z-values. The group differences in the functional connectivity (AlphaSim correction p < 0.01; continuous voxels > 16) were disclosed using two sample t-tests.
Results
Demographic and Clinical Characteristics of the Study Group
MDD patients (n = 15 MDD with CM and n = 15 MDD without CM) and matched HC (n = 17) participated in this study. As a subject in MDD without CM group was excluded for a big head motion, there were 14 subjects in MDD without CM group in practice. The demographic information, HAMD scores, and CM scores for these groups were shown in Table 1. There were no statistical differences in age, gender, and years of education between the groups. The MDD with or without CM showed higher HAMD scores compared to those in matched HC (MDD with CM: 26.33 ± 7.99; MDD without CM: 24.14 ± 4.88, HC: 1.06 ± 1.19, p < 0.01), whereas no significant difference in HAMD scores between CM and without CM groups was observed. MDD with CM had a higher CM score than MDD without CM and HC (MDD with CM: 63.33 ± 4.03; MDD without CM: 31.16 ± 5.63, HC: 30.83 ± 4.02, p < 0.01), and there was no significant difference found between MDD without CM and HC.
ALFF and fALFF Analysis
Intergroup differences of results from ALFF analysis were shown in Table 2. Compared to HC group, MDD with CM showed increased ALFF in the left orbital part of inferior frontal gyrus (-45, 18, -9. BA47/38), left middle frontal gyrus (-36, 39, 21. BA10/9/46), left medial of superior frontal gyrus (-3, 48, 33. BA9), left supplementary motor area (-3, -3, 75. BA6), left anterior cingulated and paracingulate gyri (-3, 45, 9. BA32), left supramarginal gyrus (-60, -27, 39. BA1/2), left inferior parietal, extending to supramarginal and angular gyri (-45, -48, 57. BA40/7), right orbital part of middle frontal gyrus (48, 51, -6. BA47/10/45), right triangular part of inferior frontal gyrus (51, 33, 18. BA46) and right dorsolateral part of superior frontal gyrus (21, 48, 36. BA9/8) (Figure 1). Increased ALFF in MDD without CM, compared to HC group, was observed in left triangular part of inferior frontal gyrus (-48, 48, 3. BA10/46/47), left middle frontal gyrus (-24, 51, 36. BA9), left inferior parietal, extending to supramarginal and angular gyri (-45, -51, 48. BA40/39), left precuneus (-6, -72, 57. BA7), left middle occipital gyrus (-27, -96, 12. BA19), right orbital part of inferior frontal gyrus (54, 42, -6. BA10/47/45), right medial part of superior frontal gyrus (18, 66, 12. BA10/9/6/8), right supplementary motor area (3, -6, 72. BA6), right precuneus (3, -66, 45. BA7), right angular (57, -60, 24. BA39) and right temporal role: superior temporal gyrus (45, 18, -15. BA38/47/34/28) (Figure 2).
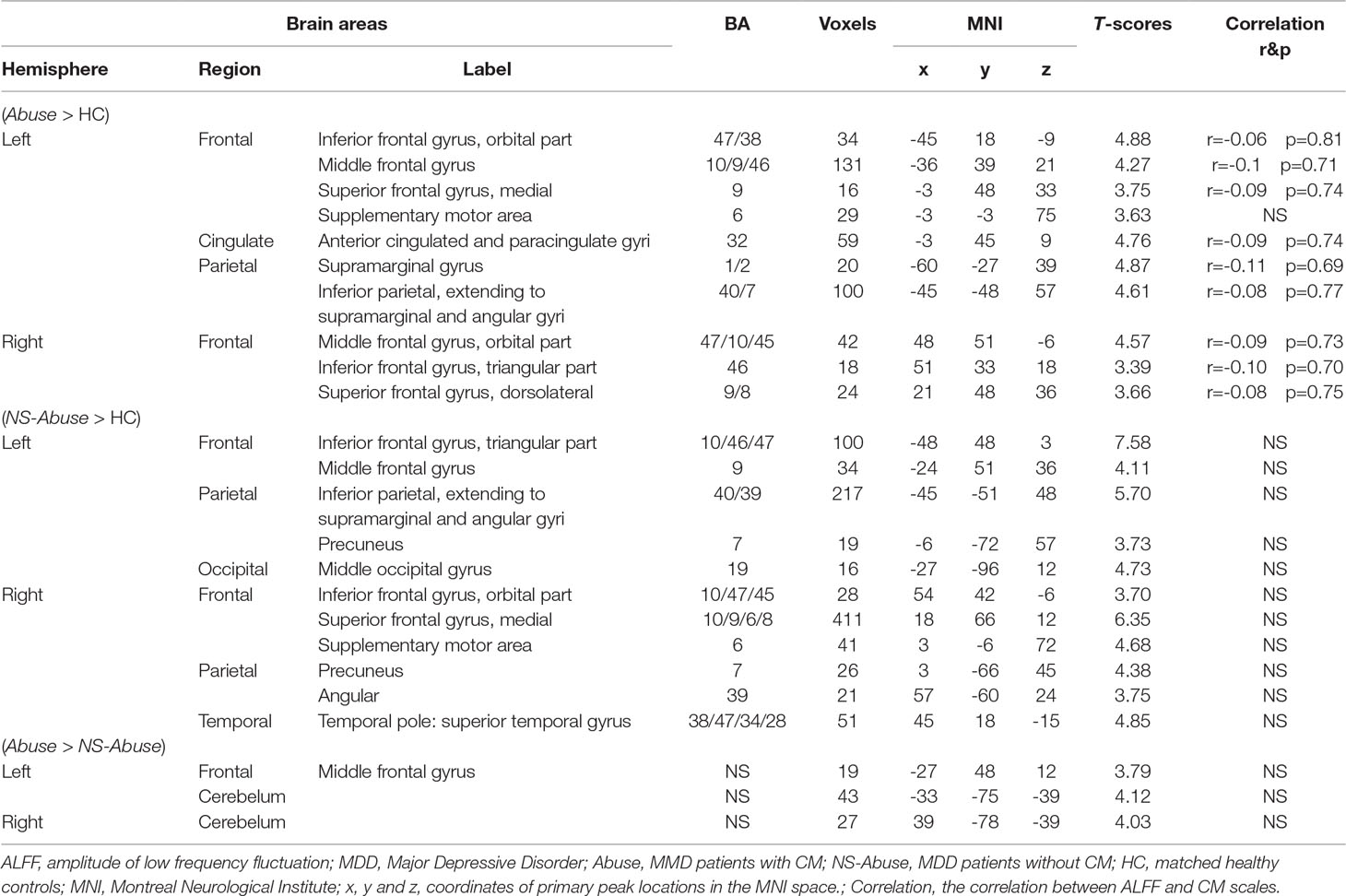
Table 2 The comparison of ALFF in MDD with CM, MDD patients without CM and controls (AlphaSim-corrected, p < 0.01).
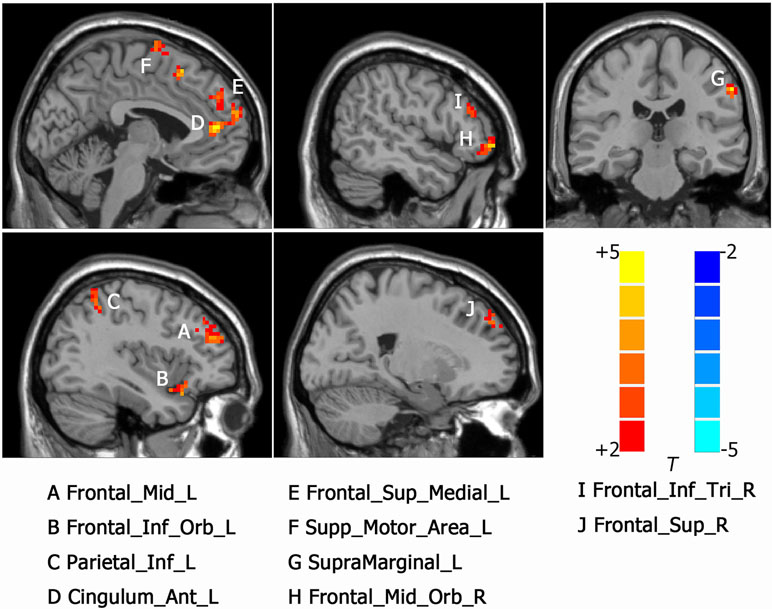
Figure 1 Activated brain regions showed by rs-fMRI using method of Amplitude of Low-Frequency Fluctuation (ALFF) in MDD patients with childhood maltreatment (CM) compared with the health control (HC). A: Frontal_Mid_L, left middle frontal gyrus; B: Frontal_Inf_Orb_L, left inferior frontal gyrus, orbital part; C: Parietal_Inf_L, left inferior parietal, but supramarginal and angular gyri; D: Cingulum_Ant_L, left anterior cingulated and paracingulate gyri; E: Frontal_Sup_Medial_L, left superior frontal gyrus, medial; F: Supp_Motor_Area_L, left supplementary motor area; G: SupraMarginal_L, left supramarginal gyrus; H: Frontal_Mid_Orb_R, right superior frontal gyrus, medial orbital; I: Frontal_Inf_Tri_R, right inferior frontal gyrus, triangular part. J: Frontal_Sup_R, right superior frontal gyrus, dorsolateral.
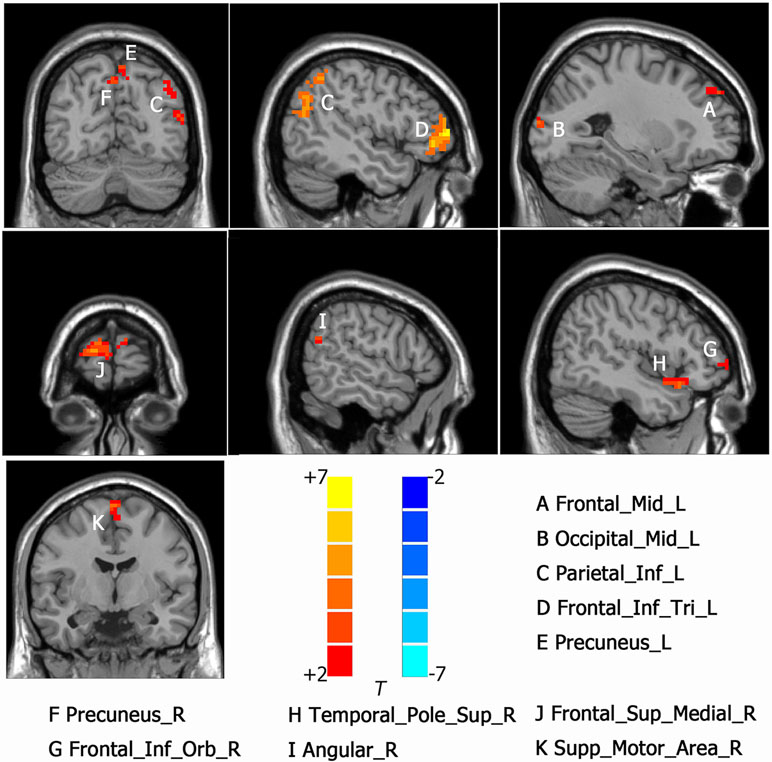
Figure 2 Activated brain regions showed by rs-fMRI using method of ALFF in MDD patients without CM compared with the HC. A: Frontal_Mid_L, left middle frontal gyrus; B: Occ:pital_Mid_L, left middle occipital gyrus; C: Parietal_Inf_L, left inferior parietal, but supramarginal and angular gyri; D: Frontal_Inf_Tri_L; left inferior frontal gyrus, triangular part, E: Precuneus_R, left precuneus; F: Precuneus_R, right precuneus; G: Frontal_Inf_Orb_R, right inferior frontal gyrus, orbital part; H Temporal_Pole_Sup_R, right temporal role: superior temporal gyrus; I: Angular_R, right angular; J: Frontal_Sup_Medial_R, right superior frontal gyurs, medial; K: Supp_Motor_Area_R, right supplementary motor area.
Compared to MDD without CM, increased ALFF was observed in the left frontal middle frontal gyrus (-27, 48, 12. NS), left cerebellum (-33, -75, -39. NS) and right cerebellum (39, -78, -39. NS) in MDD with CM (Figure 3).
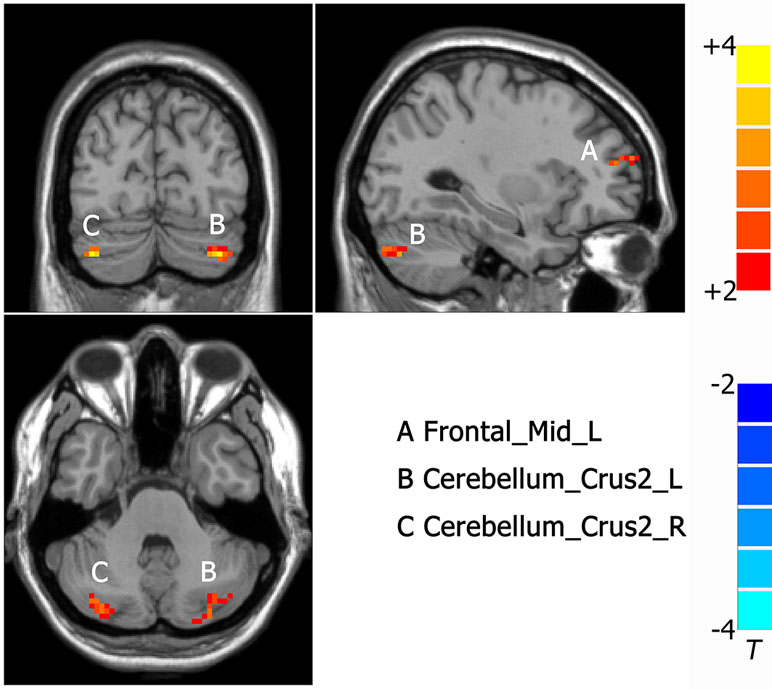
Figure 3 Activated brain regions showed by rs-fMRI using method of ALFF in MDD patients with CM compared with patients without CM. A: Frontal_Mid_L, left middle frontal gyrus; B: Cerebelum_Crus2_L, left cerebellum; C: Cerebelum_Crus2_R, right cerebellum.
Intergroup differences detected in fALFF analysis were shown in Table 3. Compared to HC group, MDD with CM showed increased fALFF in left cuneus (-9, -84, 18. BA19) (Figure 4). Increased fALFF in MDD without CM, compared to HC group, was observed in left middle temporal gyrus (-54, -57, 9. BA39) (Figure 4).
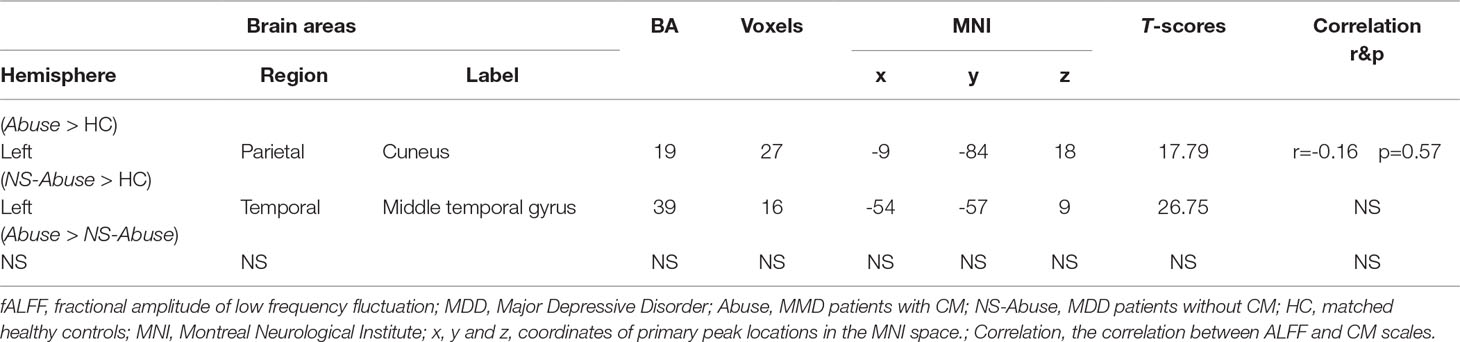
Table 3 The comparison of fALFF in MDD with CM, MDD patients without CM and controls (AlphaSim-corrected, p < 0.01).
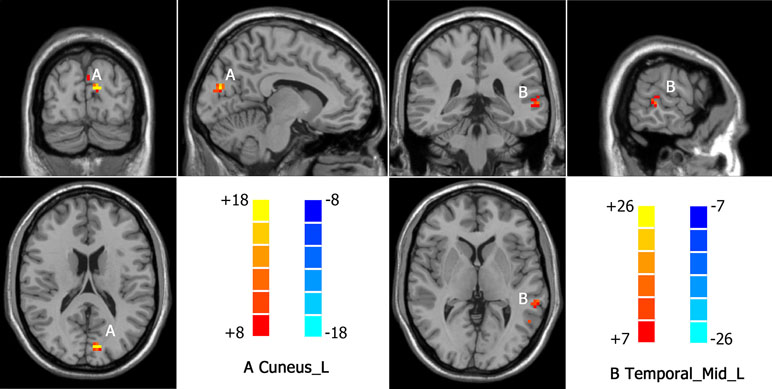
Figure 4 Increased fALFF in MDD patients with CM and patients without CM compared with healthy controls(HC). A: Cuneus_L, left cuneus; B: Temporal_Mid_L, left middle temporal gyrus.
FC Analysis
Intergroup differences observed in FC analysis were shown in Table 4. Left middle frontal gyrus where ALFF was significantly changed between MDD with CM, compared with MDD without CM, was taken as ROI. The left anterior cingulated and paracingulate gyri, left orbital part of inferior frontal gyrus and left inferior parietal, extending to supramarginal and angular gyri where ALFF was altered in MDD with CM and MDD without CM, when compared to HC, were also taken as ROIs.
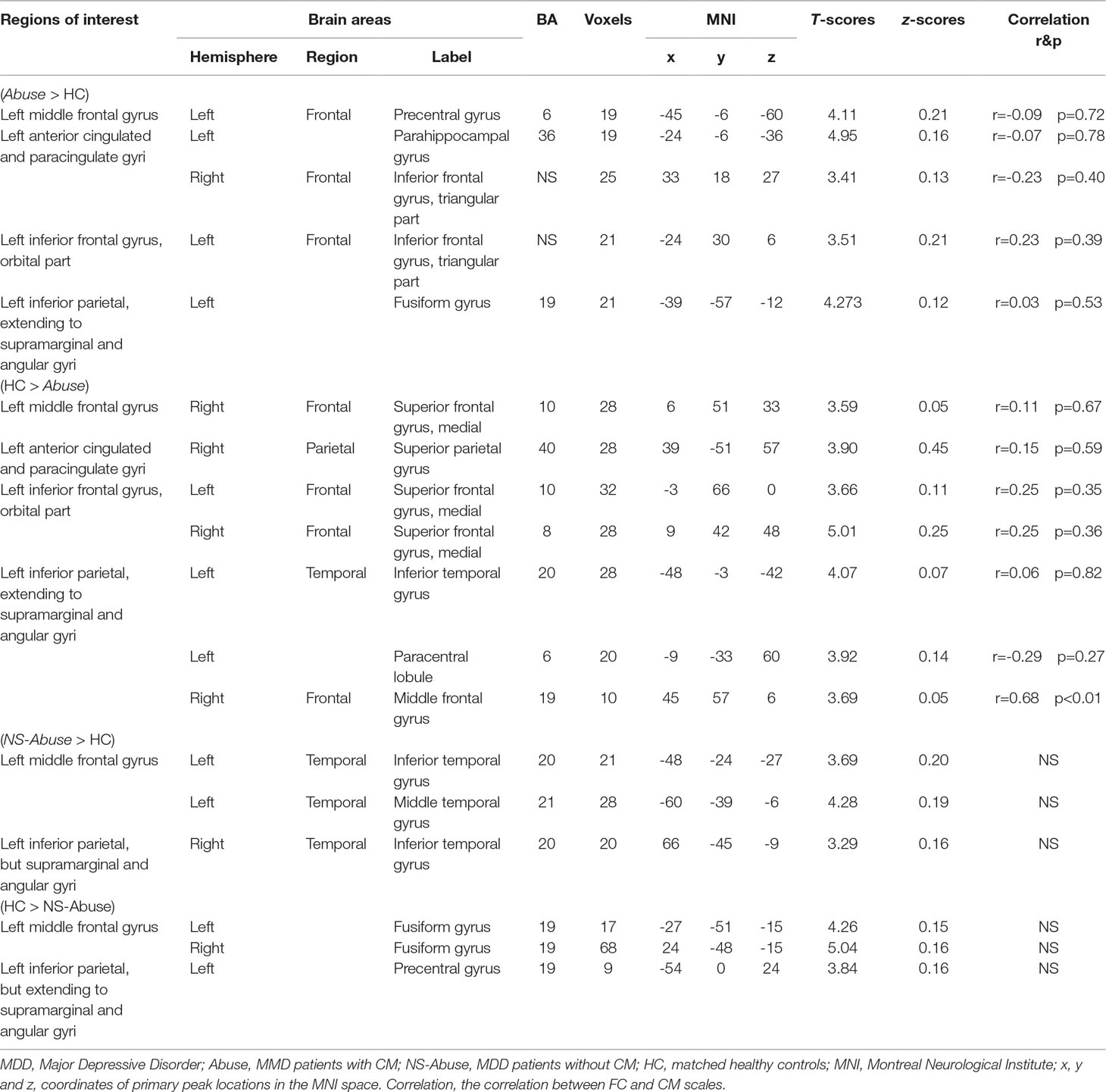
Table 4 The comparison of functional connectivity in MDD with CM, MDD patients without CM to HC (AlphaSim-corrected, p < 0.01).
In MDD with CM group, positive FC was observed between left middle frontal gyrus and left precentral gyrus (-45, -6, -60. BA6), and negative FC was observed in right medial of superior frontal gyrus (6, 51, 33. BA10). The left anterior cingulated and paracingulate gyri had positive FC with left parahippocampal gyrus (-24, -6, -36. BA36) and right triangular part of inferior frontal gyrus (33, 18, 27. NS). Negative FC was observed in right superior parietal gyrus (39, -51, 57, BA40). Left orbital part of inferior frontal gyrus had positive FC with left triangular part of inferior frontal gyrus (-24, 30, 6. NS) and negative FC was observed in left medial of superior frontal gyrus (-3, 66, 0. BA10) and right medial of superior frontal gyrus (9, 42, 48. BA8). The left inferior parietal, extending to supramarginal and angular gyri, had positive FC with left Fusiform gyrus (-39, -57, -12. BA19). Negative FCs were found in left inferior temporal gyrus (-48, -3, -42. BA20), left paracentral lobule (-9, -33, 60. BA19), and right middle frontal gyrus (45, 57, 6. BA19) (Figure 5).
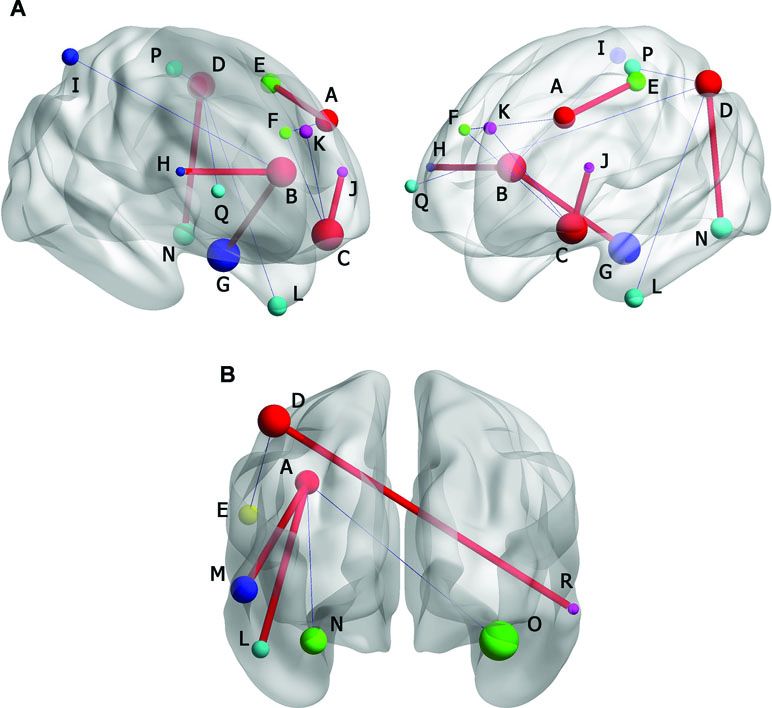
Figure 5 (A) Brain functional connectivity (FC) of rs-fMRI in MDD without CM compared with HC. (B) FC in MDD patients with CM compared with HC. Size of balls represent the t-scores of every brain regions; the blue thin edge represents the negative FC and the red bold edge represents the positive FC between different brain regions; A: left middle frontal gyrus; B: left anterior cingulated and paracingulate gyri; C: left inferior frontal gyrus, orbital part; D: left inferior parietal, but supramarginal and angular gyri; E: left precentral gyrus; F: right superior frontal gyrus, medial; G: left parphippocampal gyrus; H: right inferior frontal gyrus, triangular part; I: right superior parietal gyrus; J: left inferior frontal gyrus, triangular part; K: left superior frontal gyrus, medial; L: left inferior temporal gyrus; M: left middle temporal gyrus; N: left fusiform gyrus; O: right fusiform gyrus; P: left paracentral lobule; Q: right middle frontal gyrus; R: right inferior temporal gyrus.
In MDD without CM, positive FC was observed between left middle frontal gyrus and left inferior temporal gyrus (-48, -24, -27. BA20), left middle temporal gyrus (-60, -39, -6. BA21), whereas negative FC was observed in left fusiform gyrus (-27, -51, -15. BA19) and right fusiform gyrus (24, -48, -15. BA19). The left inferior parietal, extending to supramarginal and angular gyri, had positive FC with right inferior temporal gyrus (66, -45, -9. BA20). Negative FC was found in left precentral gyrus (-54, 0, 24. BA19) (Figure 5).
Correlation between Brain Functional Alteration
Pearson Correlation showed that FC alteration between the left inferior, extending to supramarginal and angular gyri, and right middle frontal gyrus, had a positive correlation with CM scale (r = 0.68, p < 0.01). All details are shown in Table 2, 3, 4.
Discussion
The current study has investigated the impact of maltreatment during early-life in MDD patients by examining functional activation and connectivity during resting state. To the best of our knowledge, this is the first study that visualizes the whole brain ALFF profiles of MDD with CM during spontaneous brain activity using rs-fMRI. Moreover, FC also has been adopted to detect special brain connectivity. The purpose of this study is to elucidate the mechanism of brain function underlying correlations between MDD and CM experience, based on ALFF and FC results. We also aimed to discover distinct brain regions which could differentiate MDD patients with CM experience from patients without CM.
Firstly, we found that under scan of rs-fMRI, compared with HC, MDD patients with CM had enhanced ALFF in prefrontal-limbic regions, left orbital part of inferior frontal gyrus, right orbital part of middle frontal gyrus, which is similar to the results from previous task-state fMRI studies in MDD with CM (23, 24). In MDD patients without CM, compared with HC, increased ALFF only in the right orbital part of inferior frontal gyrus was found. A depressive patient who had CM experience had more activated OFC than MDD without CM in resting state. Furthermore, brain activity in the right dorsolateral prefrontal cortex (DLPFC) was increased in MDD with CM, but not in MDD without CM. DLPFC had been targeted in transcranial magnetic therapy for MDD. DLPFC is involved in emotional process during the suppression stage, and increased FC was reported in vmPFC (25) and orbitofrontal cortex (OFC) (26). OFC is considered anatomically synonymous with the vmPFC (27–29). The orbitofrontal cortex (OFC) is well-known as a key region for regulating emotion, and any damage in OFC would result in changes in emotion, personality, behavior, and social conduct (30). The loss of volume of OFC was reported in MDD (31, 32). In the present study, both increased OFC and DLPFC were found in MDD with CM. In addition, both precuneus and angular were activated, which play great role in depression (33).
Our results show that ALFF of left anterior cingulated and paracingulate gyri, which belong to the ACC of limbic system, was increased in MDD with CM, whereas no increased ALFF in any subregion of limbic system was detected in MDD without CM. Previous studies indicated that people with CM had a smaller ACC volume than those without CM (34, 35). Task-state fMRI studies demonstrated that vmPFC/ACC activation plays key roles in processing fear, appraising negative emotions and regulating emotional responses via the limbic system (36–39). Thus, hyperactivity of ACC may underlie fear dysregulation in MDD with CM, compared with patients without CM. Although abnormal function of amygdale (40) and hippocampus (41) are reportedly associated with MDD and CM, we found no alteration in ALFF in the amygdale and hippocampus. Interestingly, altered ALFF in these brain regions in MDD patients with CM was reported using negative emotional discrimination under task-state fMRI (13, 42, 43). The discrepancy between the results may be explained by the following reasons: for the amygdale, vmPFC have direct white matter fiber projection to the amygdale (44, 45) and have a top-down, inhibitory effect on the amygdale, because OFC and ACC were both found to have increased ALFF in our study, thus the function of amygdale might be inhibited by OFC; as for the hippocampus, relative to the prefrontal cortex (PFC), it matures earlier from perceptive of evolution, thus the hippocampus is less vulnerable to CM experience (23, 46). Moreover, other factors, such as limited sample number, different states (rest instead of task), and different measurements (task activation vs. amplitude), may also affect the difference.
Secondly, our FC study showed that the left inferior frontal gyrus (orbital part) had increased FC with left inferior frontal gyrus (triangular part), and decreased FCs with bilateral superior medial frontal gyrus. Also, the left anterior cingulated, paracingulate gyri had increased FC with left parahippocampal gyrus, and decreased FC with right superior parietal gyrus in MDD with CM, compared with HC, whereas no FC was observed in these ROIs in MDD without CM. Our results also revealed the dysfunction of OFC in MDD with CM, which was consistent with previous FC studies showing increased connection between sub-regions within the orbital and prefrontal cortex (47–49), specific brain areas playing critical roles in MDD’s aberrant networks. Our results showed that the anterior cingulated and paracingulate gyri had decreased FC with superior parietal gyrus, and had increased FC with parahippocampal gyrus, which was similar to results from previous research (50). Given that ACC was a key node in default-mode network and the parahippocampal gyrus essentially involved in memory encoding, aberrant connectivity in MDD with CM may be involved in episodic memory related to experience of CM.
Lastly, after comparing the ALFF between MDD patients with CM and without CM, notably, altered ALFF in the left middle frontal gyrus (LMFG) and cerebellum was unexpectedly detected in MDD with CM compared to those without CM. MFG is a part of frontal lobe which has advanced cognitive function and participates in integrating emotion and information from the internal and external environment, and extracting episodic memory (51, 52). LMFG is located in dorsolateral prefrontal cortex, which has inhibitive (53) and recalling (54) function in psychological disease. LMFG may play a role in extracting unpleasant memory of early-life CM experience, especially memory of disagreeable verbal information, and impaired LMFG function may affect MDD onset. As for cerebellum, its volume declines in patients with MDD (55) and is involved in the modulation of emotional processing and may act as ‘emotional pacemaker’ (56) in MDD. Thus, MDD patients with CM may have a greater increase in brain activity in recalling past sufferings and emotional experience, than patients without CM.
Conclusion
Our study revealed altered resting-state brain activation in drug-naïve MDD patients with CM experience. The approach using rs-fMRI may be useful to investigate neural mechanisms into how CM affects developmental trajectory of brain maturation, leading to MDD in the later life.
Limitations
There are two major limitations. Firstly, for Chinese patients with traditional conservative concept in sex, it is difficult to collect any information regarding sex abuse during their childhood. Secondly, CM contains heterogeneous conditions that include emotional abuse, sex abuse, physical abuse, emotional neglect, and physical neglect. We did not separately analyze our results depending on the subtypes of CM in MDD patients due to limited sample number. These limitations will be a line with future inquiry being pursued by our group.
Ethics Statement
This study was approved by the Ethical Committee at the Third Affiliated Hospital of Beijing University of Chinese Medicine (protocol number: 2015BZHYLL0140). In accordance with the Declaration of Helsinki, all subjects were given written informed consent.
Author Contributions
ZX, JZ and DW participated in the design of the study, conducted the analyses, and wrote the manuscript. SZ collected the clinical information and performed the HAMD assessment. TW helped with the design and coordination of the study and wrote the manuscript. XR participated in fMRI data collection. XZ and AK contributed to interpretation of the data and drafting the manuscript. MQ and JF conceived and coordinated the design of the study, and wrote the manuscript. All authors read and approved the final manuscript.
Funding
This work was supported by the National Natural Science Foundation of China (Grant No. 81573905) and Excellent Project of Beijing Municipal Science and Technology Commission (Grant No. Z141107002514080).
Conflict of Interest Statement
The authors declare that the research was conducted in the absence of any commercial or financial relationships that could be construed as a potential conflict of interest.
References
1. WHO. Depression and other common mental disorders: global health estimates. World Health Organization (2017).
2. Anda RF, Whitfield CL, Felitti VJ, Chapman D, Edwards VJ, Dube SR, et al. Adverse childhood experiences, alcoholic parents, and later risk of alcoholism and depression. Psychiatr Serv (2002) 53:1001–9. doi: 10.1176/appi.ps.53.8.1001
3. Scott KM, Smith DR, Ellis PM. Prospectively ascertained child maltreatment and its association with DSM-IV mental disorders in young adults. Arch Gen Psychiatry (2010) 67:712–9. doi: 10.1001/archgenpsychiatry.2010.71
4. Green JG, McLaughlin KA, Berglund PA, Gruber MJ, Sampson NA, Zaslavsky AM, et al. Childhood adversities and adult psychiatric disorders in the national comorbidity survey replication I: associations with first onset of DSM-IV disorders. Arch Gen Psychiatry (2010) 67:113–23. doi: 10.1001/archgenpsychiatry.2009.186
5. Danese A, Moffitt TE, Harrington H, Milne BJ, Polanczyk G, Pariante CM, et al. 27. Adverse childhood experiences predict adult risk factors for age-related disease: depression, inflammation, and clustering of metabolic risk markers. Brain Behav Immun (2009) 23:S32–S32. doi: 10.1016/j.bbi.2009.06.032
6. Keller MB, Lavori PW, Mueller TI, Endicott J, Coryell W, Hirschfeld RM, et al. Time to recovery, chronicity, and levels of psychopathology in major depression: A 5-year prospective follow-up of 431 subjects. Arch Gen Psychiatry (1992) 49(10):809–16. doi: 10.1001/archpsyc.1992.01820100053010
7. Wiersma JE, Hovens JG, van Oppen P, Giltay EJ, van Schaik DJ, Beekman AT, et al. The importance of childhood trauma and childhood life events for chronicity of depression in adults. J Clin Psychiatry (2009) 70(7):983–9. doi: 10.4088/JCP.08m04521
8. Danese A, McEwen BS. Adverse childhood experiences, allostasis, allostatic load, and age-related disease. Physiol Behav (2012) 106:29–39. doi: 10.1016/j.physbeh.2011.08.019
9. Ito Y, Teicher MH, Glod CA, Harper D, Magnus E, Gelbard HA. Increased prevalence of electrophysiological abnormalities in children with psychological, physical, and sexual abuse. J Neuropsychiatry Clin Neurosci (1993) 5:401–8. doi: 10.1176/jnp.5.4.401
10. Schiffer F, Teicher MH, Papanicolaou AC. Evoked potential evidence for right brain activity during the recall of traumatic memories. J Neuropsychiatry Clin Neurosci (1995) 7:169–75. doi: 10.1176/jnp.7.2.169
11. Teicher MH. Scars that won’t heal: the neurobiology of child abuse. Sci Am (2002) 286:68–75. doi: 10.1038/scientificamerican0302-68
12. Fonzo GA, Flagan TM, Sullivan S, Allard CB, Grimes EM, Simmons AN, et al. Neural functional and structural correlates of childhood maltreatment in women with intimate-partner violence-related posttraumatic stress disorder. Psychiatry Res (2013) 211:93–103. doi: 10.1016/j.pscychresns.2012.08.006
13. Dannlowski U, Kugel H, Huber F, Stuhrmann A, Redlich R, Grotegerd D, et al. Childhood maltreatment is associated with an automatic negative emotion processing bias in the amygdala. Hum Brain Mapp (2013) 34:2899–909. doi: 10.1002/hbm.22112
14. De Bellis MD, Keshavan MS, Spencer S, Hall J. N-Acetylaspartate concentration in the anterior cingulate of maltreated children and adolescents with PTSD. Am J Psychiatry (2000) 157:1175–77. doi: 10.1176/appi.ajp.157.7.1175
15. Opel N, Redlich R, Zwanzger P, Grotegerd D, Arolt V, Heindel W, et al. Hippocampal atrophy in major depression: a function of childhood maltreatment rather than diagnosis? Neuropsychopharmacology (2014) 39:2723–31. doi: 10.1038/npp.2014.145
16. American Psychiatric Association. Diagnostic and statistical manual of mental disorders, 5th Edition. American Psychiatric Publishing (2013). doi: 10.1176/appi.books.9780890425596
17. Bernstein DP, Stein JA, Newcomb MD, Walker E, Pogge D, Ahluvalia T, et al. Development and validation of a brief screening version of the childhood trauma questionnaire. Child Abuse Negl (2003) 27:169–90. doi: 10.1016/S0145-2134(02)00541-0
18. Chao-Gan Y, Yu-Feng Z. DPARSF: A MATLAB toolbox for “pipeline” data analysis of resting-state fMRI. Front Syst Neurosci (2010) 4:13. doi: 10.3389/fnsys.2010.00013
19. Song XW, Dong ZY, Long XY, Li SF, Zuo XN, Zhu CZ, et al. REST: a toolkit for resting-state functional magnetic resonance imaging data processing. PloS one (2011) 6:e25031. doi: 10.1371/journal.pone.0025031
20. Xia M, Wang J, Yong H. BrainNet viewer: a network visualization tool for human brain connectomics. PloS one (2013) 8:e68910. doi: 10.1371/journal.pone.0068910
21. Zou QH, Zhu CZ, Yang Y, Zuo XN, Long XY, Cao QJ, et al. An improved approach to detection of amplitude of low-frequency fluctuation (ALFF) for resting-state fMRI: fractional ALFF. J Neurosci Methods (2008) 172:137–41. doi: 10.1016/j.jneumeth.2008.04.012
22. Teicher MH, Samson JA, Anderson CM, Ohashi K. The effects of childhood maltreatment on brain structure, function and connectivity. Nat Rev Neurosci (2016) 17:652. doi: 10.1038/nrn.2016.111
23. Hart H, Lim L, Mehta MA, Simmons A, Mirza KAH, Rubia K. Altered fear processing in adolescents with a history of severe childhood maltreatment: an fMRI study. Psychol Med (2018) 48:1092–101. doi: 10.1017/S0033291716003585
24. Wang L, Paul N, Stanton SJ, Greeson JM, Smoski MJ. Loss of sustained activity in the ventromedial prefrontal cortex in response to repeated stress in individuals with early-life emotional abuse: implications for depression vulnerability. Front Psychol (2013) 4:320. doi: 10.3389/fpsyg.2013.00320
25. Koenigs M, Grafman J. The functional neuroanatomy of depression: distinct roles for ventromedial and dorsolateral prefrontal cortex. Behav Brain Res (2009) 201:239–43. doi: 10.1016/j.bbr.2009.03.004
26. Frodl T, Bokde AL, Scheuerecker J, Lisiecka D, Schoepf V, Hampel H, et al. Functional connectivity bias of the orbitofrontal cortex in drug-free patients with major depression. Biol Psychiatry (2010) 67:161–7. doi: 10.1016/j.biopsych.2009.08.022
27. Phillips LH, MacPherson S, Della Sala S. Age, cognition and emotion: the role of anatomical segregation in the frontal lobes. In Grafman J (Ed.), Handbook of Neuropsychology: the frontal lobes. Amsterdam: Elsevier Science (2002) p. 73–97.
28. Gorka AX, Hanson JL, Radtke SR, Hariri AR. Reduced hippocampal and medial prefrontal gray matter mediate the association between reported childhood maltreatment and trait anxiety in adulthood and predict sensitivity to future life stress. Biol Mood Anxiety Disord (2014) 4:12–12. doi: 10.1186/2045-5380-4-12
29. Chugani HT, Behen ME, Muzik O, Juhász C, Nagy F, Chugani DC, et al. Local brain functional activity following early deprivation: a study of postinstitutionalized Romanian orphans. Neuroimage (2001) 14:1290–301. doi: 10.1006/nimg.2001.0917
30. Kringelbach ML, Rolls ET. The functional neuroanatomy of the human orbitofrontal cortex: evidence from neuroimaging and neuropsychology. Prog Neurobiol (2004) 72:341–72. doi: 10.1016/j.pneurobio.2004.03.006
31. Lacerda AL, Keshavan MS, Hardan AY, Yorbik O, Brambilla P, Sassi RB, et al. Anatomic evaluation of the orbitofrontal cortex in major depressive disorder. Biol Psychiatry (2004) 55:353–8. doi: 10.1016/j.biopsych.2003.08.021
32. Ballmaier M, Toga AW, Blanton RE, Sowell ER, Lavretsky H, Peterson J, et al. Anterior cingulate, gyrus rectus, and orbitofrontal abnormalities in elderly depressed patients: an MRI-based parcellation of the prefrontal cortex. Am J Psychiatry (2004) 161:99. doi: 10.1176/appi.ajp.161.1.99
33. Cheng W, Rolls ET, Qiu J, Yang D, Ruan H, Wei D, et al. Functional connectivity of the precuneus in unmedicated patients with depression. Biol Psychiatry: Cogn Neurosci Neuroimag (2018) 12:1040–9. doi: 10.1016/j.bpsc.2018.07.008
34. Cohen RA, Grieve S, Hoth KF, Paul RH, Sweet L, Tate D, et al. Early life stress and morphometry of the adult anterior cingulate cortex and caudate nuclei. Biol Psychiatry (2006) 59:975–82. doi: 10.1016/j.biopsych.2005.12.016
35. Jensen SK, Dickie EW, Schwartz DH, Evans CJ, Dumontheil I, Paus T, et al. Effect of early adversity and childhood internalizing symptoms on brain structure in young men. Jama Pediatr (2015) 169:938–46. doi: 10.1001/jamapediatrics.2015.1486
36. Phelps EA, Delgado MR, Nearing KI, Ledoux JE. Extinction learning in humans: role of the amygdala and vmPFC. Neuron (2004) 43:897–905. doi: 10.1016/j.neuron.2004.08.042
37. Milad MR, Quirk GJ, Pitman RK, Orr SP, Fischl B, Rauch SL, et al. A role for the human dorsal anterior cingulate cortex in fear expression. Biol Psychiatry (2007) 62:1191–4. doi: 10.1016/j.biopsych.2007.04.032
38. Hänsel A, Känel RV. The ventro-medial prefrontal cortex: a major link between the autonomic nervous system, regulation of emotion, and stress reactivity? Biopsychosoc Med (2008) 2:21–21. doi: 10.1186/1751-0759-2-21
39. Etkin A, Egner T, Kalisch R. Emotional processing in anterior cingulate and medial prefrontal cortex. Trends Cogn Sci (2011) 15:85–93. doi: 10.1016/j.tics.2010.11.004
40. Yuan H, Young KD, Phillips R, Zotev V, Misaki M, Bodurka J, et al. Resting-state functional connectivity modulation and sustained changes after real-time functional magnetic resonance imaging neurofeedback training in depression. Brain Connect (2014) 4:690. doi: 10.1089/brain.2014.0262
41. Macqueen G, Frodl T. The hippocampus in major depression: evidence for the convergence of the bench and bedside in psychiatric research? Mol Psychiatry (2011) 16:252. doi: 10.1038/mp.2010.80
42. McCrory EJ, De Brito SA, Sebastian CL, Mechelli A, Bird G, Kelly PA, et al. Heightened neural reactivity to threat in child victims of family violence. Curr Biol (2011) 21:R947–R948. doi: 10.1016/j.cub.2011.10.015
43. Grant MM, Cannistraci C, Hollon SD, Gore J, Shelton R. Childhood trauma history differentiates amygdala response to sad faces within MDD. J Psychiatr Res (2011) 45:886–95. doi: 10.1016/j.jpsychires.2010.12.004
44. Sah P, Faber ES, Lopez DAM, Power J. The amygdaloid complex: anatomy and physiology. Physiol Rev (2003) 83:803–34. doi: 10.1152/physrev.00002.2003
45. Holland PC, Gallagher M. Amygdala-frontal interactions and reward expectancy. Curr Opin Neurobiol (2004) 14:148–55. doi: 10.1016/j.conb.2004.03.007
46. Lupien SJ, McEwen BS, Gunnar MR, Heim C. Effects of stress throughout the lifespan on the brain, behaviour and cognition. Nature Reviews Neuroscience (2009) 10:434–45. doi: 10.1038/nrn2639
47. Price JL, Drevets WC. Neurocircuitry of mood disorders. Neuropsychopharmacology (2010) 35:192. doi: 10.1038/npp.2009.104
48. Pizzagalli DA. Frontocingulate dysfunction in depression: toward biomarkers of treatment response. Neuropsychopharmacology (2011) 36:183–206. <br/>doi: 10.1038/npp.2010.166
49. Hamani C, Mayberg H, Stone S, Laxton A, Haber S, Lozano AM. The subcallosal cingulate gyrus in the context of major depression. Biol Psychiatry (2011) 69:301–8. doi: 10.1016/j.biopsych.2010.09.034
50. van der Werff SJ, Pannekoek JN, Veer IM, van Tol MJ, Aleman A, Veltman DJ, et al. Resting-state functional connectivity in adults with childhood emotional maltreatment. Psychol Med (2013) 43:1825–36. doi: 10.1017/S0033291712002942
51. Frith CD, Frith U. Interacting minds—a biological basis. Science (1999) 286:1692–5. doi: 10.1126/science.286.5445.1692
52. Liang MJ, Zhou Q, Yang KR, Yang XL, Fang J, Chen WL, et al. Identify changes of brain regional homogeneity in bipolar disorder and unipolar depression using resting-state fMRI. PloS one (2013) 8:e79999. doi: 10.1371/journal.pone.0079999
53. Heitzeg MM, Nigg JT, Hardee JE, Soules M, Steinberg D, Zubieta JK, et al. Left middle frontal gyrus response to inhibitory errors in children prospectively predicts early problem substance use. Drug Alcohol Depend (2014) 141:51–7. doi: 10.1016/j.drugalcdep.2014.05.002
54. Manenti R, Cotelli M, Robertson IH, Miniussi C. Transcranial brain stimulation studies of episodic memory in young adults, elderly adults and individuals with memory dysfunction: a review. Brain Stimul (2012) 5:103–9. doi: 10.1016/j.brs.2012.03.004
55. Frodl TS, Koutsouleris N, Bottlender R, Born C, Jäger M, Scupin I, et al. Depression-related variation in brain morphology over 3 years: effects of stress? Arch Gen Psychiatry (2008) 65:1156. doi: 10.1001/archpsyc.65.10.1156
Keywords: major depressive disorder, childhood maltreatment, resting state, fMRI, prefrontal-limbic system
Citation: Xu Z, Zhang J, Wang D, Wang T, Zhang S, Ren X, Zhu X, Kamiya A, Fang J and Qu M (2019) Altered Brain Function in Drug-Naïve Major Depressive Disorder Patients With Early-Life Maltreatment: A Resting-State fMRI Study. Front. Psychiatry 10:255. doi: 10.3389/fpsyt.2019.00255
Received: 14 September 2018; Accepted: 03 April 2019;
Published: 24 April 2019.
Edited by:
Fushun Wang, Nanjing University of Chinese Medicine, ChinaReviewed by:
Ting Xu, Child Mind Institute, United StatesZhanjun Zhang, Beijing Normal University, China
Copyright © 2019 Xu, Zhang, Wang, Wang, Zhang, Ren, Zhu, Kamiya, Fang and Qu. This is an open-access article distributed under the terms of the Creative Commons Attribution License (CC BY). The use, distribution or reproduction in other forums is permitted, provided the original author(s) and the copyright owner(s) are credited and that the original publication in this journal is cited, in accordance with accepted academic practice. No use, distribution or reproduction is permitted which does not comply with these terms.
*Correspondence: Miao Qu, qumiaotcm@126.com
Jiliang Fang, fangmgh@163.com
†These authors contributed equally to this work.