- 1Centre for Mental Health, School of Health Sciences, Swinburne University, Hawthorn, VIC, Australia
- 2Melbourne Brain Centre @ Royal Melbourne Hospital, Parkville, VIC, Australia
- 3School of Psychology and Public Health, Latrobe University, Bundoora, VIC, Australia
People with depression have shown alterations in processing emotional information and working memory functionality. There is some evidence that emotional content may interact with working memory update processes, however neurological correlates are current unknown. In this preliminary study we utilized a novel version of the emotional variant of the n-back working memory task in fMRI. We examined BOLD response of 14 healthy controls and 13 depressed participants in response to happy, sad, and neutral displays of facial affect. No accuracy or reaction time differences were found between the two groups. The depressed group showed significantly decreased BOLD response to happy faces compared to the control group areas of the dorsal striatum and anterior cingulate. Significant, moderate, positive associations were found between right caudate activation with anxiety score and anterior cingulate activation with depression score in those with depression. Our novel task was able to elicit group level differences in emotional processing during working memory update. These results suggest those with depression fail to differentiate between positive emotional stimuli and stimuli with no emotional content.
Introduction
Depression is a common mood disorder that significantly impacts the lives of an estimated 16% of the global population (1, 2). While primarily associated with a host of physical and internal emotional states, alterations to cognition and processing of emotional information are also commonly reported (3–6). One area of impaired cognitive ability in depression that has received widespread examination is working memory (WM). WM is thought to be a limited capacity sensory register and temporarily holds incoming information for manipulation and more permanent storage in long term memory (LTM) (7). As new information is brought in, existing information is either passed to LTM or discarded, making successful updating an important part of general cognition. Multiple brain regions have been identified with normal WM functioning (8–13) including the medial cerebellum, bilateral medial parietal, bilateral premotor, bilateral dorsolateral, and ventrolateral prefrontal cortex (DLPFC & VLPFC, respectively) and bilateral frontal poles. Storage and updating of information within WM can be examined using the n-back, a task where the participant is presented with a series of discrete stimuli and asked to match the current stimulus to one shown n items prior (14). The n-back is an extremely versatile task base, able to utilize multiple stimuli types yet still consistently activate fronto-parieto-cerebellum regions (15, 16) and consistently shows larger recruitment of these areas with increased load (17).
In depression, the n-back task has been used during fMRI scans to examine WM function. Typically, those with depression show larger activation in frontal areas (18–20) which suggest the need to recruit additional neuronal resources to compensate for deficiencies in update. Behavioral evidence for this however has been mixed, fMRI studies have tended to find no significant differences in reaction times (RT) or accuracy (18–23) while purely behavioral studies utilizing identical tasks (24, 25) have found those with depression perform significantly worse than controls.
The processing of stimuli containing emotionally salient information is another area in which differences have been suggested between those with depression compared to healthy controls (4). One commonly reported change from behavioral studies is a bias toward negative stimuli. For example, studies examining processing of words have found those with major depressive disorder (MDD) showed longer RT when attempting to process words that contain negative or depressive related words (26). When processing facial affect, those with depression show a similar bias toward faces displaying negative emotions, and could more quickly identify faces showing emotions such as sadness and disgust, but seem unable to effectively break off attention from the stimuli (27–29). Depressed participants are also more likely to rate neutral faces as sad and happy faces more negatively than controls (30).
Functional studies of emotional processing in depression using a variety of tasks and have found differences in BOLD activity in the putamen, amygdalae / parahippocampal area, insula, subgenual, and anterior cingulate and the ventromedial PFC (VMPF) (31–37). One study examining implicit processing of emotional expression in depression (38) found differential patterns of activity in processing sad and happy faces compared to controls. Those with depression showed increased activity in the right fusiform gyrus, left putamen, and left parahippocampal/amgydalae areas when presented with sad faces and decreased activity in bilateral fusiform and right putamen to happy emotional expressions.
Examining the interactions between negative affect and WM on cognitive performance, Joormann and Gotlib (39) found those with depression showed longer decision times when presented with negative non-target words using a modified version of the Sternberg test. Levens and Gotlib (40), using an emotional variant of the n-back task found depressed participants displayed faster RT when viewing sad faces but also significantly longer to respond to any faces following the presentation of sad faces. This suggests those with depression have attentional bias toward sad displays of facial affect which facilitates more efficient behavioral response. Additionally, this may indicate that when attention has been captured by negative information, WM processes may have more difficulty clearing the negative information thus producing less effective updating to incorporate new information. Thus, recruitment of additional neural resources within frontal regions similar to load effects could be required order to maintain satisfactory performance (18), however no studies have examined this directly.
Therefore, we created an emotional variant of the n-back task for use in fMRI to examine potential neural correlates of WM interruption in facial affective processing in depression. We hypothesized the task would elicit group differences in neural response to facial affect, when comparing the depressed group with controls. Furthermore, we hypothesized the depressed group would show faster RT to emotionally negative (sad) stimuli and increased response in frontal and limbic regions compared to the control group. We also predicted the control group would show similar behavioral and BOLD response, but to emotionally positive (happy) faces.
Materials and Methods
This pilot study was approved by the Alfred Hospital and Swinburne University of Technology ethics committees in accordance with the declaration of Helsinki.
Participants and Prescan Procedure
Participants were recruited from the public via social media, advertising in outpatient clinics, patient support groups and referral by mental health professionals. Inclusion in the study required participants to be between the ages of 18–65 with no history of/or suspected neurological disorder, drug or alcohol abuse. Participants were also required to have normal or corrected to normal vision to see the stimuli while in the scanner. In addition, for inclusion in the depression group, participants were required to have their diagnosis confirmed by their treating mental health care professional (psychologist/psychiatrist/GP) and be experiencing a depressed episode. Depression participants were excluded if they had a co-morbid diagnosis, such as bipolar disorder or schizophrenia; anxiety disorders were acceptable, however no participant reported a current diagnosis.
Inclusion was also based on a cut-off score from the depression sub scale of the Depression, Anxiety, Stress Scale 21 (DASS-21) a 21-item questionnaire that measures symptoms associated with depression, anxiety and stress. The DASS-21 has been shown to have good psychometric properties and is strongly correlated with other depression symptoms scales such as the Beck Depression Inventory (41). The DASS-21 is clinically sensitive to severity of state depressive symptoms (42) and their changes over time over time (43).
Control participants were excluded from the study if they scored above a “mild” rating (12+) on the depression sub scale, while those with depression were excluded if they scored below a “moderate” rating (<14). General fMRI safety exclusion criteria were also enforced.
On the day of the scan, participants were asked to fill in a questionnaire package containing demographics questions and a second sitting of the DASS-21. Data from participants whose scores were not within the cut-offs for their respective groups were not included in the study. Participants were then given time outside the scanner to practice the task until they achieved an accuracy of 65% or greater. Those participants requiring corrected vision were fitted with MR compatible prescriptive goggles (mediglasses; http://www.crsltd.com) and tested using a standard Snellen eye chart to confirm 6/6 vision.
Initially 17 controls and 15 depressed participants were recruited, with none reporting inability to perceive colors. Data from 3 controls (2 female) and 2 depressed participants (both male) were not included due to not satisfying the cut-off criteria. The remaining 14 controls and 13 depression participant's demographic information is shown in Table 1.
Control participants scored within the normal range depression, anxiety, and stress symptoms, while the depressed group were in the severe range for all three (41). Of the nine participants on antidepressant medications, four were on selective serotonin reuptake inhibitors, four on serotonin-norepinephrine reuptake inhibitors and one on anti-psychotics. No participants reported changes within the last 6 months to the type and amount of medication they were taking and none reported a history of electroconvulsive therapy.
3-Back Task
We designed a 3-back version of the well validated n-back task (11) that incorporated two types of stimuli, facial affect and basic visual patterns. The basic visual stimuli were intended to act as a WM baseline, to leave only regions relating to face and emotional processing. Previous studies (44, 45) have found robust emotional effects using a similar contrast. A 3-back design was used due to suggestions by Harvey et al. (18) that hyper frontal responses in depression can only be elicited by tasks requiring large cognitive effort.
Task Design and Presentation
Participants were asked to determine whether the sex of the faces or the color of the patterns on the currently presented image was the same or different as the one presented three images ago and ignore the emotion and identity of faces or pattern design. Responses for same or different were obtained through a button box. The first three trials were marked as different with the following stimuli requiring a response of same or different.
The blocked design n-back task consisted of 32 blocks recorded in two runs (16 per run). Each block was 16.2 s in length and consisted of 9 trials, with each trial made up of a 2 s fixation cross and 1.6 s presentation of the stimuli, either faces (male and female faces displaying one of three emotional expressions) or patterns (basic stimuli of patterns and colors with one of three designs). Blocks alternated between faces and patterns conditions, with one emotion (happy, sad, neutral) or pattern design type (horizontal, vertical, checked) presented per block. Participants were asked to determine whether the sex of the faces or the color of the patterns on the currently presented image was the same or different as the one presented three images ago and ignore the emotion and identity of faces or pattern design. Responses for same or different were obtained through a button box. The first three trials were marked as different with the following stimuli requiring a response of same or different. Six blocks of happy emotional expressions and horizontal orientated gratings were recorded over the two sessions (three per session), while the sad, neutral, vertical, and checked blocks were presented five times over the two runs (either two or three presentations per run). Each session was 8.37 min in length with total scan time 16.74 min.
The faces condition contained 33 happy, sad and neutral faces (16 female) from the NimStim collection (http://www.macbrain.org/resources.htm), a freely available collection of emotional face stimuli with good internal validity and reliability (46). Faces were closed mouth and contained within a black border. Patterned stimuli were made using Adobe Photoshop CS2 (http://www.photoshop.com) and consisted of three black geometric designs on a colored background—horizontal stripes, vertical stripes, or a checked pattern. Backgrounds consisted of 2 colors, purple or yellow with patterns made of widths ranging from 5 mm line widths to 12 in 1 mm increments (7 increments per pattern, 21 in total). Stimuli were presented using E-Prime version 2 (www.pst.com) and presented on a 24-inch (60.96 cm) Cambridge research systems BOLDscreen fMRI (http://www.crsltd.com). Participants viewed the images through a mirror mounted on the head coil, roughly 10 cm from the eyes and 100 cm from the monitor. Figure 1 displays the stimuli presentation sequence.
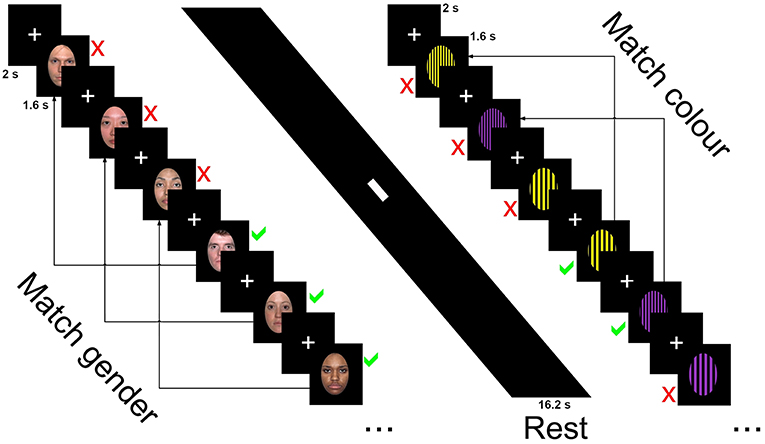
Figure 1. Stimuli presentation sequence for the 3-back task showing neutral faces, rest, and horizontal grating conditions.
Behavioral Analysis
Behavioral data and correlations between fMRI and clinical data were analyzed using the python 2.7 packages scipy and pyvttbl (https://github.com/rogerlew/pyvttbl). Custom permutation tests (47) based on mixed ANOVAs (48) two tailed Wilcoxon signed-rank post-hoc tests (49) and two tailed Spearman correlation coefficients (modified from the method proposed by (50) were used for null hypothesis testing. The single step maxT method (51) was used for correction of multiple comparisons in post-hoc and correlation analyses. For each test, 10,000 re-shuffles were used. Scripts are available from http://github.com/petergoodin/montecarlo_funcs or on request.
fMRI Acquisition
Data were collected with a Siemens Tim Trio 3T using a standard 32 channel head coil. A high resolution MPRAGE scan (208 slices, TR = 1,800 ms, TE = 2.79 ms, inversion time = 900 ms, slice thickness = 0.7, in plane resolution of 0.7 mm3, FOV 210 mm, flip angle 9°, 320 frequency/phase encoding steps) was collected for registration to the functional data and normalization to a standardized space. Whole brain fMRI data were collected using an echo planar imaging (EPI) sequence, AC/PC aligned axial acquisition (TR = 3,000 ms, TE = 30 ms) with 168 volumes containing 51 slices (slice thickness = 2.5 mm with a 0.5 mm gap between slices, in plane resolution of 3 mm3, 74 × 74 matrix, flip angle = 90°, 74 frequency/phase encoding steps, bandwidth = 1648 Hz per pixel, GRAPPA factor 2).
fMRI Analysis
fMRI data preprocessing and modeling was carried out using FEAT and MELODIC, part of the FSL 5.0.9 package (www.fmrib.ox.ac.uk/fsl). Functional data were unwarped using fieldmaps acquired prior to each run (TE = 30, blip direction = negative) and registered to a 2 mm MNI space template brain using FLIRT. Preprocessing consisted of motion correction using MCFLIRT, slice timing correction (52) using Fourier-space time-series phase-shifting, application of a 6 mm full width half maximum (FWHM) smoothing kernel and intensity normalization across volumes. Optimal high pass filtering was calculated automatically in FEAT using the first level design matrix and used Gaussian-weighted least-squares straight line fitting with sigma = 45 s (0.022 Hz).
Independent Component Analysis (ICA) using MELODIC was used to decompose the data to manually remove artifacts. Components were deemed to be artifactual if: time series that did not resemble the known blocked onset/offset times of the stimuli; power spectral densities were clustered toward higher frequencies, extremely low frequencies or had a relatively uniform distribution. Components were also deemed artifactual if their spatial characteristics resembled known artifact types (e.g., radio frequency artifact producing a random speckled pattern, motion artifact showing activation around the edge of the brain or large extremely smooth regions with no tissue boundaries, cardiac/vasculature artifact showing BOLD response localized in vessels such as the carotid and middle cerebral arteries, and responses within white matter and cerebrospinal fluid filled regions including ventricles).
First level regressors were convolved with a double gamma function with inclusion of temporal derivatives. Each emotion condition (happy, sad, and neutral) were contrasted against the mean response of the patterned conditions. A second level fixed effects analysis was used to combine the two runs and the resulting contrast of parameter estimates (COPE). Higher level, within and between-group contrasts were examined using Randomize (53). Pooled one sample t-test permutation analyses were performed for each emotional condition to show location of activation using threshold-free cluster enhancement (TFCE) (54) and 10,000 re-shuffles. Two sample contrasts (control minus depressed) were also performed for each emotional condition to examine for differences of emotional processing on BOLD response during the n-back task. The Harvard Oxford atlas (55) was used for neuroanatomical labeling. Group level difference significant cluster mean estimates were extracted across individuals for correlation analyses with behavioral data (56).
For all analyses, a maxT corrected alpha of 0.05 or less was considered the threshold to signify a result being of sufficiently low probability as occurring under the null distribution to warrant consideration as a potentially legitimate effect (significant).
Results
Behavioral Data
A two sided Fisher's exact test showed no significant imbalance of sex between the control and depressed group (Odds ratio = 2.5, p = 0.419). Permutation analysis showed no significant difference in age (t-obs = 0.21, p = 0.816) and revealed the expected increased symptom scores for depression, anxiety and stress scales (all t-obs > 7.01, all p < 0.001) for the depressed group.
Table 2 outlines the means (M) and standard deviations (SD) for accuracy and RT, between the control and depression groups across the six conditions and between condition significant differences.
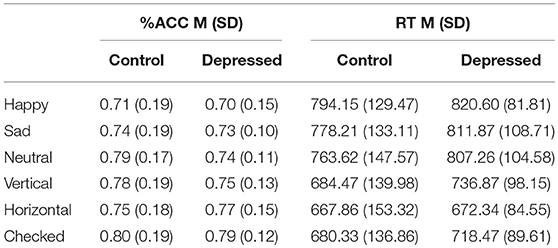
Table 2. Mean (SD) for accuracy (%ACC) and reaction time (RT) of control and Depressed participants across happy, sad, neutral faces and vertical, horizontal, checked pattern conditions.
Potential main effects and interaction differences in n-back accuracy and reaction time were tested using separate permutation tests using 3 (emotional condition) × 2 (Group) mixed ANOVAs. Results showed significant main effects of condition for both accuracy (F-obs = 9.00, p < 0.001) and reaction time (F-obs = 27.17, p < 0.001) but not for group (F-obs < 1.00, p > 0.40). There was no significant group interaction for either accuracy or reaction time (F-obs < 1.30, p > 0.27). The permutation results are shown in Figure 2.
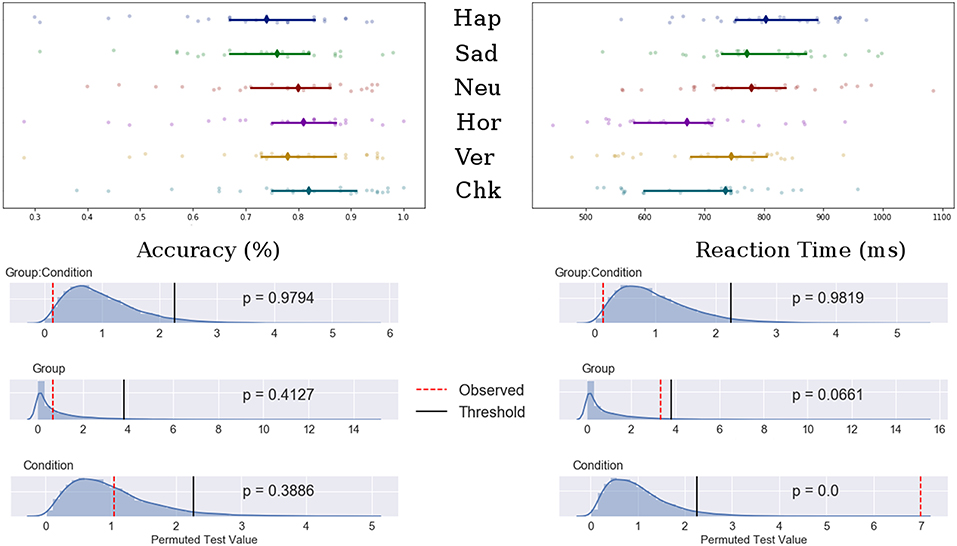
Figure 2. Top: Median value for accuracy (Left) and reaction time (Right) for happy, sad, neutral, horizontal, vertical, and checked conditions. Bars indicate 95% confidence interval bootstrapped 10,000 times, points represent individual participant data. Bottom: Permuted null distribution with observed (red) and threshold (black) values marked as vertical bars.
Post-hoc analyses showed decreased accuracy for the happy condition compared to all patterned and neutral conditions (t-obs < −3.40, p < 0.01), sad and neutral to checked pattern conditions (t-obs < −2.81, p < 0.05) and sad to neutral conditions (t-obs = −2.59, p = 0.48). Accuracy was decreased for the vertical compared to the checked pattern conditions (t-obs = −2.64, p = 0.043). RT were significantly increased for the emotional conditions compared to patterned conditions (t-obs > 3.01, p < 0.001). No differences were found in reaction times between emotions. The vertical condition stimuli were reacted to slower compared to horizontal conditions stimuli (t-obs = 3.01, p = 0.041).
Task fMRI Data
fMRI responses to each emotion pooled across the two groups showed activation within face and emotional processing regions including the fusiform gyrus, temporal poles, amgydalae and inferior frontal gyrus (57) (see Figure 3).
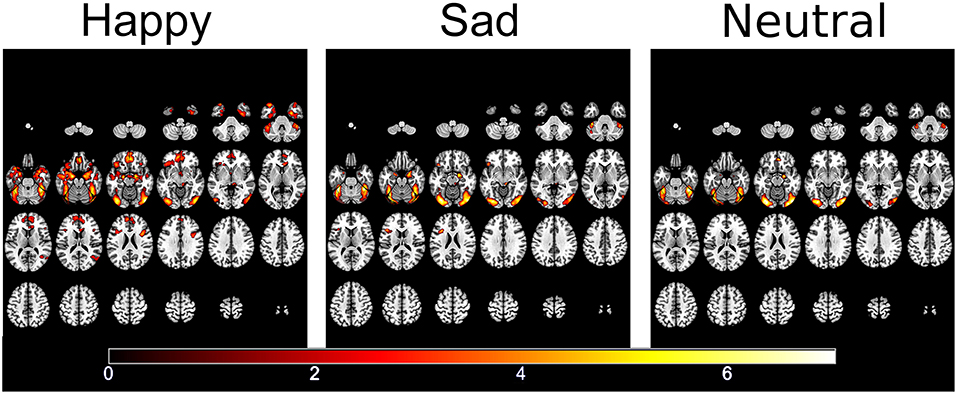
Figure 3. Significantly activated areas for happy, sad, and neutral faces compared to the patterned baseline.
Between group analyses showed significantly increased activation for control compared to depressed participants in response to happy faces within the striatum (left and right caudate, left putamen), genu of the corpus collosum and the right rostral anterior cingulate (rACC). Extracted contrast estimates showed control participants had activation greater than the patterns baseline, while depressed participants generally showed responses similar to or less than baseline. Areas showing significant differences between the groups and individual contrast estimate values are shown in Figure 4. No significant differences were found between groups for sad and neutral conditions (see Supplemental Material for cluster sizes, peak locations, and peak value).
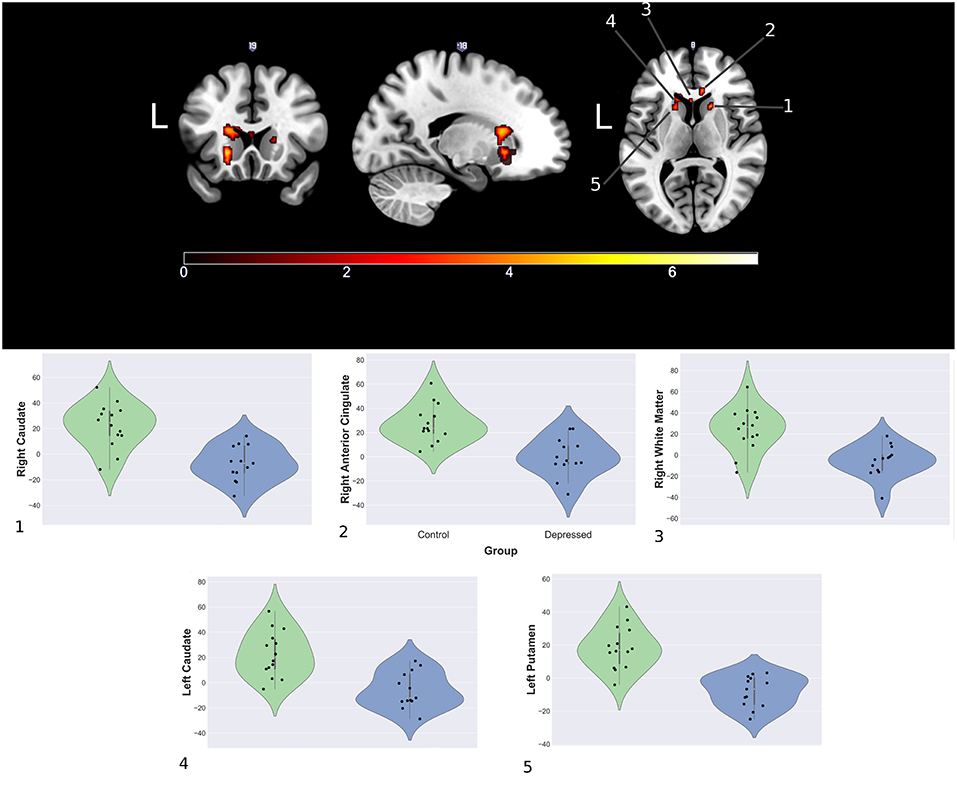
Figure 4. Significantly increased areas of activation in the control group compared to depression during presentation of happy faces (Top) and mean cluster estimates (Bottom).
Clinical—fMRI Correlations
Post-hoc spearman correlation analyses on mean cluster estimates and clinical scores found two significant, moderately positive monotonic correlations for the depressed group. Increased right rACC response were associated with increased DASS-21 depression scale score (ρ = 0.60, p = 0.036), while increased right caudate response correlated with increased with DASS-21 anxiety scale score (ρ = 0.69, p = 0.013). There were no significant clinical and fMRI correlations for the control group. Further analysis showed the depressed group had significantly increased correlations between the rACC and DASS-21 depression score (Δ ρ = 0.57, p = 0.009) and the right caudate with anxiety (Δ ρ = 0.755, p ≤ 0.001) when compared to controls. Figure 5 shows the correlation matrix for the depressed group and scatterplots between the rACC and DASS depression score and the right caudate with anxiety (see Supplemental Material for the control group's correlations).
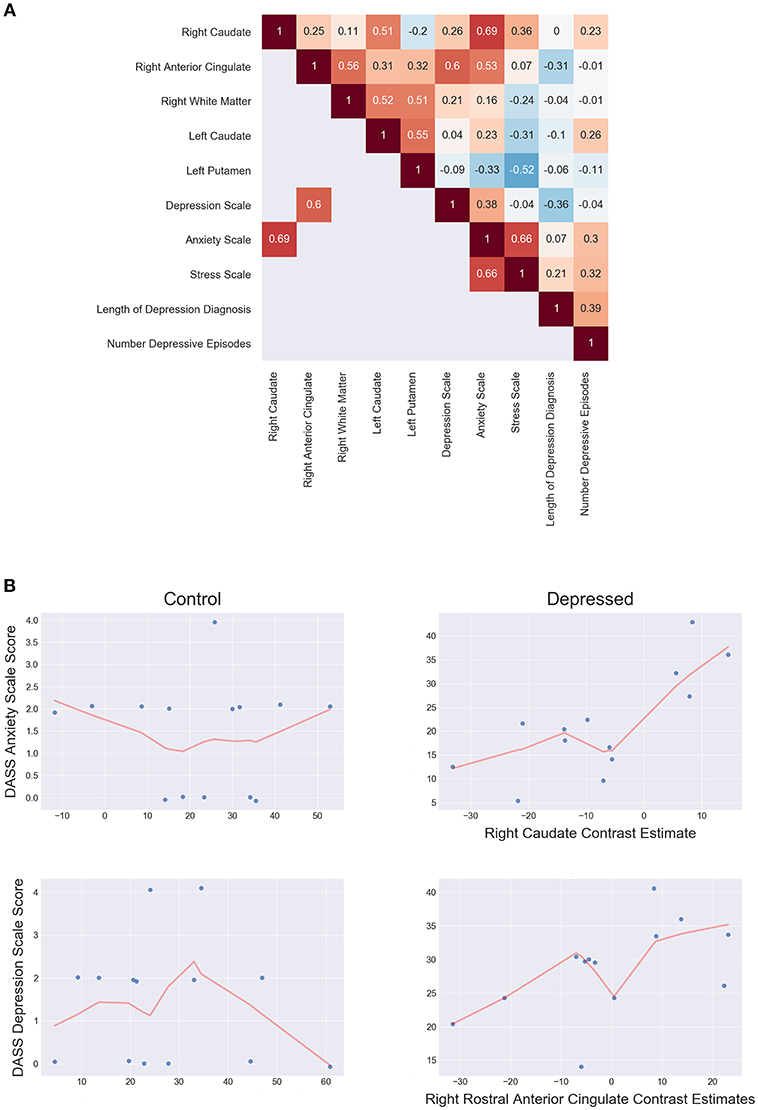
Figure 5. (A) Depressed correlation matrix of Spearman's ρ shows unthresholded correlations in the top triangle and thresholded (p < 0.05 two tailed) correlations on the bottom. (B) Scatterplots of controls (left) and depressed (right) for DASS anxiety scale score and right caudate estimates (top) and DASS Depression scale score and right rostral anterior cingulate estimates (bottom). LOESS line (red; fraction = 0.5) indicates the direction of the monotonic relationship.
Discussion
Our novel n-back task was able to elicit group level BOLD differences in facial affectual processing when comparing depressed and control groups, which was as predicted. To our knowledge, this is the first study that has examined BOLD response to emotional faces during working memory update.
Contrary to expectations, the depressed group did not show the predicted decrease in reaction time (RT) or increased BOLD response to sad faces in frontal regions when compared to controls. Controls also did not show faster RT and increased frontal BOLD to happy faces. Depressed participants displayed decreased BOLD activation in several regions of the striatum and the rACC in response to happy faces when engaged in the n-back task. Extracted contrast estimates showed the average depressed group response to happy was similar to the baseline patterns condition. Additionally, depressed participants also showed significant, moderate, positive monotonic correlations between DASS-21 depression symptom score with the rACC, and the DASS-21 anxiety symptom score with the right caudate.
We were unable to detect the hypothesized differences in RT between happy and sad faces. Slower RT to negative stimuli in those with depression have been previously reported in behavioral studies (4, 40), however in this study we found no significant difference between the control and depressed group. This consistent with other emotional expression n-back studies conducted using fMRI (18, 20, 21). We were also unable to detect changes within the frontal and limbic systems as hypothesized. Altered frontal and limbic activity, especially the ventromedial prefrontal cortex (VMPFC) and amygdalae reactivity are a commonly reported functional association with depression (58–60), however we did not detect any significant differences between the two groups contrary to our hypothesis.
This study found decreased response in the striatum in response to happy faces in the depressed group. Part of the dopamine innervated basal ganglia, the striatum has is associated addiction, motivation and inhibitory behaviors (61–63). The dorsal striatum, which includes the caudate and putamen is considered to link frontal “top-down” and more posterior “bottom-up” driven systems involved in attention, memory, social and emotional processing, integrating information, and functioning in a goal directed, motivated capacity (64). Fiber tracking studies have found projections from the putamen connect to the primary motor cortex and supplementary motor area (65, 66) while the caudate has dense connections with largely frontal regions, including the prefrontal cortex (67), an area strongly associated with WM (11).
Like the dorsal striatum, the anterior cingulate has been associated with a substantial amount of stimuli responsivity and behavioral outcomes (68, 69) however focused studies and parcellation of the region (70) has allowed for some delineation of functioning within subregions (71, 72). Projections from the rACC connect largely with the prefrontal cortex in addition to tracts between the rACC and limbic regions, including the amygdala (73). Functional connectivity has shown anti-correlations between the rACC with motor and dorsolateral prefrontal regions, while medial structures such as the limbic and orbitofrontal cortex (OFC) show positive correlations (74). It has been suggested that the region may be involved with emotional awareness or orientating attentional resources to the processing of emotional stimuli (75), of which preference in healthy individuals may be given to happy faces (76, 77).
Our results showed the depressed group had lower fMRI response to happy faces when compared to controls. Additionally, the decreased response was not greater than the baseline, which may indicate that as a group, the depressed cohort failed to differentiate between visual displays of positive emotion and visual information of no emotional salience. Depression is associated with alterations in emotional processing, which can include decreased recognition of positive emotion, such as happy faces (35, 40, 78). Several fMRI studies have shown changes in functional connectivity between the dorsal striatum and other regions of the brain affect emotional processing in depression (79–81). For example, Yang et al. (82) have shown decreased activation in the extended regions including the striatum only when viewing positive emotional stimuli. Furthermore, they found this decreased activity and resting state functional connectivity correlated with clinical scores.
Additionally, several functional imaging meta-analyses (58–60, 83) have found the rACC, caudate and putamen display decreased BOLD signal to positive emotional stimuli in depressed individuals. Murrough et al. (84) found depressed participants also displayed decreased response to happy faces compared to healthy controls in the right caudate. When administered a single dose of ketamine, activation in the caudate increased significantly compared to baseline levels. This suggests the striatum may be involved in emotional blunting to positive affect. The Positive Attenuation Hypothesis (PAH), posits those with depression have decreased response to positive emotional stimuli compared to healthy controls, stemming from depressed individual's tendency to exhibit low positive mood (85, 86). Decreased response to positive emotional stimuli may arise from motivational deficits (87). Given the dorsal striatum's association with reward processing, attention and motivation and its role in emotional processing the results of our study suggest those with depression may have decreased reward response and motivation to engage with positive stimuli during working memory update, potentially due to inability to differentiate between emotional conditions. Whether this is a prodromal response or a trait caused by depressive episode onset is unknown.
Positive monotonic associations were observed between depression symptom score with the rACC and anxiety symptom score with the right caudate. Several studies have found functional connectivity to the striatum to largely frontal regions is associated with clinical variables (82, 88, 89) however this is the first study to show region specific correlations with clinical variables during a working memory task. Of note, these associations were significantly larger for the depressed group compared to the control group, who showed weak associations between these regions and clinical scores. Given the positive nature of the relationship, this suggests as activation in these regions for depressed participants increases, so too does the clinical symptom scores. However, the control group showed increased activation in these regions compared to the depressed group but did not show any association. These results may suggest there may be altered functionality in the striatum of those with depression that extends beyond simple differences in activation. It may be beneficial for future studies to validate this dissociation with larger sample.
Some limitations to the study need to be acknowledged. This study had a small sample size with 13 depressed and 14 control participants included in the final analysis. This typically leads to overfitting to the observed data and inability to generalize the results to the wider populations of interest. For these reasons we utilized permutation testing which allows for inference regarding the samples directly with good control of type 1 error and with confidence of the reported p-value directly linked to the number of reshuffles (53). While our results may not be able to be directly applied on the depressed population, they may still be used as to inform further study and be validated through replication, like other studies that utilize the null hypothesis testing framework (90). We did not detect the predicted decreased reaction time and increased frontal activation for depressed participants to sad faces. This may be due to the small sample size of this study which was insufficient to detect the effect size of interest and stratify depression behavioral subtypes. McIntyre et al. (91) has suggested overt WM deficits in behavioral outcomes such as accuracy and RT in depression have small to medium effects sizes. Furthermore, they suggested that distinct deficits in working memory occur only within 20–30% of those with the disorder. The small participant numbers found in many neuroimaging studies, including this one, would be unable to differentiate between such small effect sizes. Additionally, despite showing severe levels of depressive symptoms as measured by the DASS21, the depressed group were all recruited from the general public and were able to function within wider society, taking part in normal activities such as attending work, school, and raising families. These participants were able to organize their time, attend and engage in the testing session. This suggests the depressed participants recruited for this study may have developed successful methods to compensate for their disorder and, in turn, this may have influenced the behavioral results.
We were also unable to detect difference in amygdalae response between groups. This may have been due our assumption that the presentation of faces using a blocked design would elicit a consistent response in the amygdalae over time. However, amygdalae response to constant presentation of affectual stimuli may habituate (92). The constant presentation of faces with the same emotional expression during a block may have attenuated amygdalae response. Further, the decreased amygdalae response may have also been associated with lack of detected difference in the VMPFC. Roy et al. (93) suggested a role for the VMPFC is inhibition of subcortical / limbic responses. As the response of the amygdalae attenuated, it is possible the inhibitory response of the VMPFC was also decreased. Revising the design to be event related may help to identify if this was the cause.
In this study, 4 of the 13 depressed participants (~30%) were on pharmacological treatment for their condition. This may have been another reason for the lack of difference between the depressed and control group. Medication effects have also been reported to alter response in the caudate nucleus and anterior cingulate during a verbal n-back task (23), with medication reported to increase the BOLD response in these areas. In our study we found the depressed group displayed significantly decreased response to happy faces compared to controls in these regions, so assuming a similar response had medicated participants not been included, we may have observed an even more exaggerated decrease in BOLD response.
In conclusion, this study aimed to examine neural correlates of WM update when processing displays of facial affect using a novel emotional n-back variation. We found the depressed group showed a failure to activate to positive emotional stimuli compared to healthy controls in the dorsal striatum and rACC. Additionally, the right caudate activity associated with increased depressive symptom score. This suggests the task we developed to probe WM update in depression to facial affect can elicit an emotional response between control and depressed participants while performing the n-back task. Furthermore, our preliminary results suggest those with depression exhibit altered functionality of the dopaminergic dorsal striatum which contributes to depressed mood through attenuation of positive emotional stimuli during WM update. Further examination into the dorsal striatum's relationship with emotional processing in working memory may contribute to our understanding of decreased responsiveness to positive information in depression.
Author Contributions
PG, SR, and JC conceived and designed the study. PG oversaw data collection and integrity, wrote the permutation methods to analyse the behavioral data, analyzed behavioral, and fMRI data, and wrote the manuscript drafts. SR and JC provided feedback on the manuscript drafts. MH provided domain knowledge and feedback on the manuscript drafts. GL provided extensive domain knowledge and feedback on the manuscript drafts.
Funding
This study was partially funded by the Barbara Dicker Foundation.
Conflict of Interest Statement
The authors declare that the research was conducted in the absence of any commercial or financial relationships that could be construed as a potential conflict of interest.
Acknowledgments
The authors acknowledge the facilities and scientific and technical assistance of the National Imaging Facility, a National Collaborative Research Infrastructure Strategy (NCRIS) capability, at the Swinburne Neuroimaging Facility, Swinburne University of Technology. The authors wish to thank David Liley, Jerome Maller, E.M. and M.E.G for their assistance.
Supplementary Material
The Supplementary Material for this article can be found online at: https://www.frontiersin.org/articles/10.3389/fpsyt.2019.00060/full#supplementary-material
References
1. Kessler RC, Bromet EJ. The epidemiology of depression across cultures. Ann Rev Pub Health. (2013) 34:119–38. doi: 10.1146/annurev-publhealth-031912-114409
2. Andrade L, Caraveo-anduaga JJ, Berglund P, Bijl RV, Graaf RD, Vollebergh W, et al. The epidemiology of major depressive episodes: results from the International Consortium of Psychiatric Epidemiology (ICPE) Surveys. Int J Methods Psychiat Res. (2003) 12:3–21. doi: 10.1002/mpr.138
3. Austin MC, Mitchell P, Goodwin GM. Cognitive deficits in depression: Possible implications for functional neuropathology. Br J Psychiat. (2001) 178:200–6. doi: 10.1192/bjp.178.3.200
4. Elliott R, Zahn R, Deakin JF, Anderson IM. Affective cognition and its disruption in mood disorders. Neuropsychopharmacology. (2010) 36:153–82. doi: 10.1038/npp.2010.77
5. Gotlib IH, Joormann J. Cognition and depression: current status and future directions. Ann Rev Clin Psychol. (2010) 6:285–312. doi: 10.1146/annurev.clinpsy.121208.131305
6. McClintock SM, Husain MM, Greer TL, Cullum CM. Association between depression severity and neurocognitive function in major depressive disorder: a review and synthesis. Neuropsychology. (2010) 24:9–34. doi: 10.1037/a0017336
7. Baddeley A. Working memory: looking back and looking forward. Nat Rev Neurosci. (2003) 4:829–39. doi: 10.1038/nrn1201
8. Braver TS, Barch DM, Kelley WM, Buckner RL, Cohen NJ, Miezin FM, et al. Direct comparison of prefrontal cortex regions engaged by working and long-term memory tasks. Neuroimage. (2001) 14:48–59. doi: 10.1006/nimg.2001.0791
9. Martinkauppi S, Rämä P, Aronen HJ, Korvenoja A, Carlson S. Working memory of auditory localization. Cereb Cortex. (2000) 10:889–98. doi: 10.1093/cercor/10.9.889
10. Neta M, Whalen PJ. Individual differences in neural activity during a facial expression vs. identity working memory task. Neuroimage. (2011) 56:1685–92. doi: 10.1016/j.neuroimage.2011.02.051
11. Owen AM, McMillan KM, Laird AR, Bullmore E. N-back working memory paradigm: a meta-analysis of normative functional neuroimaging studies. Hum Brain Mapp. (2005) 25:46–59. doi: 10.1002/hbm.20131
12. Savini N, Brunetti M, Babiloni C, Ferretti A. Working memory of somatosensory stimuli: an fMRI study. Int J Psychophysiol. (2012) 86:220–8. doi: 10.1016/j.ijpsycho.2012.09.007
13. Rottschy C, Langner R, Dogan I, Reetz K, Laird AR, Schulz JB, et al. Modelling neural correlates of working memory: a coordinate-based meta-analysis. NeuroImage. (2012) 60:830–46. doi: 10.1016/j.neuroimage.2011.11.050
14. Kirchner WK. Age differences in short-term retention of rapidly changing information. J Exp Psychol. (1958) 55:352–8. doi: 10.1037/h0043688
15. Brahmbhatt SB, McAuley T, Barch DM. Functional developmental similarities and differences in the neural correlates of verbal and nonverbal working memory tasks. Neuropsychologia. (2008) 46:1020–31. doi: 10.1016/j.neuropsychologia.2007.11.010
16. Nystrom LE, Braver TS, Sabb FW, Delgado MR, Noll DC, Cohen JD. Working memory for letters, shapes, and locations: fMRI evidence against stimulus-based regional organization in human prefrontal cortex. Neuroimage. (2000) 11:424–46. doi: 10.1006/nimg.2000.0572
17. Callicott JH, Mattay VS, Bertolino A, Finn K, Coppola R, Frank JA, et al. Physiological characteristics of capacity constraints in working memory as revealed by functional MRI. Cereb Cort. (1999) 9:20–6. doi: 10.1093/cercor/9.1.20
18. Harvey PO, Fossati P, Pochon JB, Levy R, LeBastard G, Lehéricy S, et al. Cognitive control and brain resources in major depression: An fMRI study using the n-back task. Neuroimage. (2005) 26:860–9. doi: 10.1016/j.neuroimage.2005.02.048
19. Matsuo K, Glahn DC, Peluso MAM, Hatch JP, Monkul ES, Najt P, et al. Prefrontal hyperactivation during working memory task in untreated individuals with major depressive disorder. Mol Psychiat. (2007) 12:158–66. doi: 10.1038/sj.mp.4001894
20. Rose EJ, Simonotto E, Ebmeier KP. Limbic over-activity in depression during preserved performance on the n-back task. Neuroimage. (2006) 29:203–15. doi: 10.1016/j.neuroimage.2005.07.002
21. Fitzgerald PB, Srithiran A, Benitez J, Daskalakis ZZ, Oxley TJ, Kulkarni J, et al. An fMRI study of prefrontal brain activation during multiple tasks in patients with major depressive disorder. Hum Brain Mapp. (2008) 29:490–501. doi: 10.1002/hbm.20414
22. Kerestes R, Ladouceur CD, Meda S, Nathan PJ, Blumberg HP, Maloney K, et al. Abnormal prefrontal activity subserving attentional control of emotion in remitted depressed patients during a working memory task with emotional distracters. Psychol Med. (2011) 42:29–40. doi: 10.1017/S003329171100109
23. Walsh ND, Williams SC, Brammer MJ, Bullmore ET, Kim J, Suckling J, et al. A longitudinal functional magnetic resonance imaging study of verbal working memory in depression after antidepressant therapy. Biol Psychiat. (2007) 62:1236–43. doi: 10.1016/j.biopsych.2006.12.022
24. Harvey PO, Le Bastard G, Pochon JB, Levy R, Allilaire JF, Dubois B, et al. Executive functions and updating of the contents of working memory in unipolar depression. J Psychiat Res. (2004) 38:567–76. doi: 10.1016/j.jpsychires.2004.03.003
25. Rose EJ, Ebmeier KP. Pattern of impaired working memory during major depression. J Aff Dis. (2006) 90:149–61. doi: 10.1016/j.jad.2005.11.003
26. Li M, Feng L, Liu X, Zhang M, Fu B, Wang G, et al. Emotional working memory in patients with major depressive disorder. J Int Med Res. (2018) 46:1734–46. doi: 10.1177/0300060518758225
27. Gilboa-Schechtman E, Ben-Artzi E, Jeczemien P, Marom S, Hermesh H. Depression impairs the ability to ignore the emotional aspects of facial expressions: evidence from the Garner task. Cogn Emot. (2004) 18:209–31. doi: 10.1080/02699930341000176a
28. Gotlib IH, Krasnoperova E, Yue DN, Joormann J. Attentional biases for negative interpersonal stimuli in clinical depression. J Abnorm Psychol. (2004) 113:121–35. doi: 10.1037/0021-843X.113.1.121
29. Joormann J, Gotlib IH. Selective attention to emotional faces following recovery from depression. J Abnorm Psychol. (2007) 116:80–5. doi: 10.1037/0021-843X.116.1.80
30. Douglas KM, Porter RJ. Recognition of disgusted facial expressions in severe depression. Br J Psychiat. (2010) 197:156–7. doi: 10.1192/bjp.bp.110.078113
31. Frodl T, Scheuerecker J, Albrecht J, Kleemann AM, Müller-Schunk S, Koutsouleris N, et al. Neuronal correlates of emotional processing in patients with major depression. World J Biol Psychiat. (2009) 10:202–8. doi: 10.1080/15622970701624603
32. Fu CH, Mourao-Miranda J, Costafreda SG, Khanna A, Marquand AF, Williams SR, et al. Pattern classification of sad facial processing: toward the development of neurobiological markers in depression. Biol Psychiat. (2008) 63:656–62. doi: 10.1016/j.biopsych.2007.08.020
33. Fu CH, Williams SC, Cleare AJ, Brammer MJ, Walsh ND, Kim J, et al. Attenuation of the neural response to sad faces in major depression by antidepressant treatment: a prospective, event-related functional magnetic resonance imaging study. Arch Gen Psychiat. (2004) 61:877–89. doi: 10.1001/archpsyc.61.9.877
34. Gotlib IH, Sivers H, Gabrieli JD, Whitfield-Gabrieli S, Goldin P, Minor KL, et al. Subgenual anterior cingulate activation to valenced emotional stimuli in major depression. Neuroreport. (2005) 16:1731–4. doi: 10.1097/01.wnr.0000183901.70030.82
35. Sheline YI, Barch DM, Donnelly JM, Ollinger JM, Snyder AZ, Mintun MA. Increased amygdala response to masked emotional faces in depressed subjects resolves with antidepressant treatment: an fMRI study. Biol Psychiat. (2001) 50:651–8. doi: 10.1016/S0006-3223(01)01263-X
36. Wang L, LaBar KS, Smoski M, Rosenthal MZ, Dolcos F, Lynch TR, et al. Prefrontal mechanisms for executive control over emotional distraction are altered in major depression. Psychiat Res. (2008) 163:143–55. doi: 10.1016/j.pscychresns.2007.10.004
37. Werner NS, Meindl T, Materne J, Engel RR, Huber D, Riedel M, et al. Functional MRI study of memory-related brain regions in patients with depressive disorder. J Aff Dis. (2009) 119:124–31. doi: 10.1016/j.jad.2009.03.003
38. Surguladze S, Brammer MJ, Keedwell PA, Giampietro V, Young AW, Travis MJ, et al. A differential pattern of neural response toward sad versus happy facial expressions in major depressive disorder. Biol Psychiat. (2005) 57:201–9. doi: 10.1016/j.biopsych.2004.10.028
39. Joormann J, Gotlib IH. Updating the contents of working memory in depression: interference from irrelevant negative material. J Abnorm Psychol. (2008) 117:182–92. doi: 10.1037/0021-843X.117.1.182
40. Levens SM, Gotlib IH. Updating positive and negative stimuli in working memory in depression. J Exp Psychol Gen. (2010) 139:654–64. doi: 10.1037/a0020283
41. Lovibond PF, Lovibond SH. The structure of negative emotional states: comparison of the Depression Anxiety Stress Scales (DASS) with the beck depression and anxiety inventories. Behav Res Ther. (1995) 33:335–43. doi: 10.1016/0005-7967(94)00075-U
42. Antony MM, Bieling PJ, Cox BJ, Enns MW, Swinson RP. Psychometric properties of the 42-item and 21-item versions of the Depression Anxiety Stress Scales in clinical groups and a community sample. Psychol Assess. (1998) 10:176–81. doi: 10.1037/1040-3590.10.2.176
43. Page AC, Hooke GR, Morrison DL. Psychometric properties of the Depression Anxiety Stress Scales (DASS) in depressed clinical samples. Br J Clin Psychol. (2007) 46:283–97. doi: 10.1348/014466506X158996
44. Hariri A, Drabant E, Munoz K, Kolachana B, Mattay V, Egan E, et al. A susceptibility gene for affective disorders and the response of the human amygdala. Arch Gen Psychiat. (2005) 62:146–52. doi: 10.1001/archpsyc.62.2.146
45. Townsend JD, Eberhart NK, Bookheimer SY, Eisenberger NI, Foland-Ross LC, Cook IA, et al. fMRI activation in the amygdala and the orbitofrontal cortex in unmedicated subjects with major depressive disorder. Psychiat Res Neuroimag. (2010) 183:209–17. doi: 10.1016/j.pscychresns.2010.06.001
46. Tottenham N, Tanaka JW, Leon AC, McCarry T, Nurse M, Hare TA, et al. The NimStim set of facial expressions: judgments from untrained research participants. Psychiatry Res. (2009) 168:242–9. doi: 10.1016/j.psychres.2008.05.006
47. Ludbrook J, Dudley H. Why permutation tests are superior to t and F tests in biomedical research. Am Statist. (1998) 52:127–32. doi: 10.2307/2685470
48. Manly BFJ. Randomization, Bootstrap and Monte Carlo methods in Biology. 3rd Edn. London: Chapman & Hall.
49. Ge Y, Dudoit S, Speed TP. Resampling-based multiple testing for microarray data analysis. Test. (2003) 12:1–77. doi: 10.1007/BF02595811
50. Ikpotokin O, Edokpa IW. Correlation analysis: the bootstrap approach. Int J Adv Res. (2013) 1:21–39.
51. Tsai C-A, Chen JJ. Kernel estimation for adjusted p-values in multiple testing. Comput Statis Data Anal. (2007) 51:3885–97. doi: 10.1016/j.csda.2006.03.007
52. Sladky R, Friston KJ, Tröstl J, Cunnington R, Moser E, Windischberger C. Slice-timing effects and their correction in functional MRI. Neuroimage. (2011) 58:588–94. doi: 10.1016/j.neuroimage.2011.06.078
53. Winkler AM, Ridgway GR, Webster MA, Smith SM, Nichols TE. Permutation inference for the general linear model. NeuroImage. (2014) 92:381–97. doi: 10.1016/j.neuroimage.2014.01.060
54. Smith SM, Nichols TE. Threshold-free cluster enhancement: addressing problems of smoothing, threshold dependence and localisation in cluster inference. NeuroImage. (2009) 44:83–98. doi: 10.1016/j.neuroimage.2008.03.061
55. Desikan RS, Ségonne F, Fischl B, Quinn BT, Dickerson BC, Blacker D, et al. An automated labeling system for subdividing the human cerebral cortex on MRI scans into gyral based regions of interest. Neuroimage. (2006) 31:968–80. doi: 10.1016/j.neuroimage.2006.01.021
56. Poldrack RA, Fletcher PC, Henson RN, Worsley KJ, Brett M, Nichols TE. Guidelines for reporting an fMRI study. Neuroimage. (2008) 40:409–14. doi: 10.1016/j.neuroimage.2007.11.048
57. Lindquist KA, Wager TD, Kober H, Bliss-Moreau E, Barrett LF. The brain basis of emotion: a meta-analytic review. Behav Brain Sci. (2012) 35:121–43. doi: 10.1017/S0140525X11000446
58. Palmer SM, Crewther SG, Carey LM. A meta-analysis of changes in brain activity in clinical depression. Front Hum Neurosci. (2015) 8:1045. doi: 10.3389/fnhum.2014.01045
59. Fitzgerald PB, Laird AR, Maller J, Daskalakis ZJ. A meta-analytic study of changes in brain activation in depression. Hum Brain Mapp. (2008) 29:683–95. doi: 10.1002/hbm.20426
60. Diener C, Kuehner C, Brusniak W, Ubl B, Wessa M, Flor H. A meta-analysis of neurofunctional imaging studies of emotion and cognition in major depression. Neuroimage. (2012) 61:677–85. doi: 10.1016/j.neuroimage.2012.04.005
61. Di Martino A, Scheres A, Margulies DS, Kelly AMC, Uddin LQ, Shehzad Z, et al. Functional connectivity of human striatum: a resting state fMRI study. Cereb Cort. (2008) 18:2735–47. doi: 10.1093/cercor/bhn041
62. Kober H, Barrett LF, Joseph J, Bliss-Moreau E, Lindquist K, Wager TD. Functional grouping and cortical–subcortical interactions in emotion: a meta-analysis of neuroimaging studies. Neuroimage. (2008) 42:998–1031. doi: 10.1016/j.neuroimage.2008.03.059
63. Wager TD, Davidson ML, Hughes BL, Lindquist MA, Ochsner KN. Prefrontal-subcortical pathways mediating successful emotion regulation. Neuron. (2008) 59:1037–50. doi: 10.1016/j.neuron.2008.09.006
64. Balleine BW, Delgado MR, Hikosaka O. The role of the dorsal striatum in reward and decision-making. J Neurosci. (2007) 27:8161–65. doi: 10.1523/JNEUROSCI.1554-07.2007
65. Draganski B, Kherif F, Klöppel S, Cook PA, Alexander DC, Parker GJM, et al. Evidence for segregated and integrative connectivity patterns in the human basal ganglia. J Neurosci. (2008) 28:7143–52. doi: 10.1523/JNEUROSCI.1486-08.2008
66. Lehéricy S, Ducros M, Krainik A, Francois C, Van de Moortele P-F, Ugurbil K, et al. 3-D diffusion tensor axonal tracking shows distinct SMA and Pre-SMA projections to the human striatum. Cereb Cort. (2004) 14:1302–9. doi: 10.1093/cercor/bhh091
67. Jarbo K, Verstynen TD. Converging structural and functional connectivity of orbitofrontal, dorsolateral prefrontal, and posterior parietal cortex in the human striatum. J Neurosci. (2015) 35:3865–78. doi: 10.1523/JNEUROSCI.2636-14.2015
68. Devinsky O, Morrell MA, Vogt B. Contributions of anterior cingulate cortex to behaviour. Brain. (1995) 118:279–306. doi: 10.1093/brain/118.1.279
69. Bush G, Luu P, Posner MI. Cognitive and emotional influences in anterior cingulate cortex. Trends Cognit Sci. (2000) 4:215–22. doi: 10.1016/S1364-6613(00)01483-2
70. Beckmann M, Johansen-Berg H, Rushworth MFS. Connectivity-based parcellation of human cingulate cortex and its relation to functional specialization. J Neurosci. (2009) 29:1175–90. doi: 10.1523/JNEUROSCI.3328-08.2009
71. Margulies DS, Kelly AMC, Uddin LQ, Biswal BB, Castellanos FX, Milham MP. Mapping the functional connectivity of anterior cingulate cortex. NeuroImage. (2007) 37:579–88. doi: 10.1016/j.neuroimage.2007.05.019
72. Stevens FL, Hurley RA, Taber KH, Hurley RA, Hayman LA, Taber KH. Anterior cingulate cortex: unique role in cognition and emotion. JNP. (2011) 23:121–5. doi: 10.1176/jnp.23.2.jnp121
73. Haber SN, Knutson B. The reward circuit: linking primate anatomy and human imaging. Neuropsychopharmacology. (2010) 35:4–26. doi: 10.1038/npp.2009.129
74. Yu C, Zhou Y, Liu Y, Jiang T, Dong H, Zhang Y, et al. Functional segregation of the human cingulate cortex is confirmed by functional connectivity based neuroanatomical parcellation. NeuroImage. (2011) 54:2571–81. doi: 10.1016/j.neuroimage.2010.11.018
75. Lane RD, Reiman EM, Axelrod B, Yun L-S, Holmes A, Schwartz GE. Neural correlates of levels of emotional awareness: evidence of an interaction between emotion and attention in the anterior cingulate cortex. J Cognitive Neurosci. (1998) 10:525–35. doi: 10.1162/089892998562924
76. Fusar-Poli P, Allen P, Lee F, Surguladze S, Tunstall N, Fu CH, et al. Modulation of neural response to happy and sad faces by acute tryptophan depletion. Psychopharmacology. (2007) 193:31–44. doi: 10.1007/s00213-007-0757-4
77. Vogt BA. Pain and emotion interactions in subregions of the cingulate gyrus. Nat Rev Neurosci. (2005) 6:533–44. doi: 10.1038/nrn1704
78. Beck AT. Thinking and depression. i. idiosyncratic content and cognitive distortions. Arch Gen Psychiat. (1963) 9:324–33. doi: 10.1001/archpsyc.1963.01720160014002
79. Heller AS, Johnstone T, Shackman AJ, Light SN, Peterson MJ, Kolden GG, et al. Reduced capacity to sustain positive emotion in major depression reflects diminished maintenance of fronto-striatal brain activation. Proc Natl Acad Sci USA. (2009) 106:22445–50. doi: 10.1073/pnas.0910651106
80. Hanson JL, Hariri AR, Williamson DE. Blunted ventral striatum development in adolescence reflects emotional neglect and predicts depressive symptoms. Biol Psychiat. (2015) 78:598–605. doi: 10.1016/j.biopsych.2015.05.010
81. Epstein J, Pan H, Kocsis JH, Yang Y, Butler T, Chusid J, et al. Lack of ventral striatal response to positive stimuli in depressed versus normal subjects. AJP. (2006) 163:1784–90. doi: 10.1176/ajp.2006.163.10.1784
82. Yang Y, Zhong N, Imamura K, Lu S, Li M, Zhou H, et al. Task and resting-state fMRI reveal altered salience responses to positive stimuli in patients with major depressive disorder. PLOS ONE. (2016) 11:e0155092. doi: 10.1371/journal.pone.0155092
83. Stuhrmann A, Suslow T, Dannlowski U. Facial emotion processing in major depression: a systematic review of neuroimaging findings. Biol Mood Anxiety Dis. (2011) 1:10. doi: 10.1186/2045-5380-1-10
84. Murrough JW, Collins KA, Fields J, DeWilde KE, Phillips ML, Mathew SJ, et al. Regulation of neural responses to emotion perception by ketamine in individuals with treatment-resistant major depressive disorder. Transl Psychiatry. (2015) 5:e509. doi: 10.1038/tp.2015.10
85. Bylsma LM, Morris BH, Rottenberg J. A meta-analysis of emotional reactivity in major depressive disorder. Clin Psychol Rev. (2008) 28:676–91. doi: 10.1016/j.cpr.2007.10.001
86. Rottenberg J, Gross JJ, Gotlib IH. Emotion context insensitivity in major depressive disorder. J Abnorm Psychol. (2005) 114:627–39. doi: 10.1037/0021-843X.114.4.627
87. Yang X, Huang J, Zhu C, Wang Y, Cheung EFC, Chan RCK, et al. Motivational deficits in effort-based decision making in individuals with subsyndromal depression, first-episode and remitted depression patients. Psychiat Res. (2014) 220:874–82. doi: 10.1016/j.psychres.2014.08.056
88. Gabbay V, Ely BA, Li Q, Bangaru SD, Panzer AM, Alonso CM, et al. Striatum-based circuitry of adolescent depression and anhedonia. J Am Acad Child Adolesc Psychiat. (2013) 52:628–41.e13. doi: 10.1016/j.jaac.2013.04.003
89. van Tol MJ, Veer IM, van der Wee NJA, Aleman A, van Buchem MA, Rombouts SARB, et al. Whole-brain functional connectivity during emotional word classification in medication-free major Depressive Disorder: Abnormal salience circuitry and relations to positive emotionality. NeuroImage Clin. (2013) 2:790–6. doi: 10.1016/j.nicl.2013.05.012
91. McIntyre RS, Cha DS, Soczynska JK, Woldeyohannes HO, Gallaugher LA, Kudlow P, et al. Cognitive deficits and functional outcomes in major depressive disorder: determinants, substrates and treatment interventions. Depress Anxiety. (2013) 30:515–27. doi: 10.1002/da.22063
92. Zald DH. The human amygdala and the emotional evaluation of sensory stimuli. Brain Res Rev. (2003) 41:88–123. doi: 10.1016/S0165-0173(02)00248-5
Keywords: fMRI, working memory, emotional processing, dorsal striatum, depression, n-back
Citation: Goodin P, Lamp G, Hughes ME, Rossell SL and Ciorciari J (2019) Decreased Response to Positive Facial Affect in a Depressed Cohort in the Dorsal Striatum During a Working Memory Task—A Preliminary fMRI Study. Front. Psychiatry 10:60. doi: 10.3389/fpsyt.2019.00060
Received: 02 August 2018; Accepted: 28 January 2019;
Published: 05 March 2019.
Edited by:
Qinghua He, Southwest University, ChinaReviewed by:
Haichao Zhao, Beihang University, ChinaMingxia Zhang, Chinese Academy of Sciences, China
Copyright © 2019 Goodin, Lamp, Hughes, Rossell and Ciorciari. This is an open-access article distributed under the terms of the Creative Commons Attribution License (CC BY). The use, distribution or reproduction in other forums is permitted, provided the original author(s) and the copyright owner(s) are credited and that the original publication in this journal is cited, in accordance with accepted academic practice. No use, distribution or reproduction is permitted which does not comply with these terms.
*Correspondence: Peter Goodin, peter.goodin@unimelb.edu.au