- 1Unit of Pharmacogenetics and Clinical Psychopharmacology, Centre for Psychiatric Neuroscience, Department of Psychiatry, Lausanne University Hospital, Prilly, University of Lausanne, Lausanne, Switzerland
- 2Centre of Psychiatric Epidemiology and Psychopathology, Department of Psychiatry, Lausanne University Hospital, Prilly, University of Lausanne, Lausanne, Switzerland
- 3Unit of Population Epidemiology, Department of Community Medicine and Primary Care and Emergency Medicine, University Hospital of Geneva, Geneva, Switzerland
- 4Division of Chronic Diseases, Institute of Social and Preventive Medicine, Lausanne University Hospital, University of Lausanne, Lausanne, Switzerland
- 5Service of Old Age Psychiatry, Department of Psychiatry, Lausanne University Hospital, Prilly, University of Lausanne, Lausanne, Switzerland
- 6Service of General Psychiatry, Department of Psychiatry, Lausanne University Hospital, Prilly, University of Lausanne, Lausanne, Switzerland
- 7School of Pharmaceutical Sciences, University of Geneva, Geneva, Switzerland
Importance: Multiple studies conducted in the general population identified an association between self-reported coffee consumption and plasma lipid levels. To date, no study assessed whether and which plasma methylxanthines (caffeine and/or its metabolites, i.e., paraxanthine, theophylline, and theobromine) are associated with plasma lipids. In psychiatric patients, an important coffee consumption is often reported and many psychotropic drugs can induce a rapid and substantial increase of plasma lipid levels.
Objective: To determine whether plasma methylxanthines are associated with metabolic parameters in psychiatric patients receiving treatments known to induce metabolic disturbances.
Design, Setting, and Participants: Data were obtained from a prospective study including 630 patients with metabolic parameters [i.e., body mass index (BMI), total cholesterol (TC), low-density lipoprotein cholesterol (LDL-C), high-density lipoprotein cholesterol (HDL-C), non-high-density lipoprotein cholesterol (non-HDL-C), and fasting triglycerides (TG)] monitored routinely during psychotropic treatment.
Exposures: Plasma methylxanthines levels.
Main Outcomes and Measures: Metabolic variables including BMI and plasma lipid levels.
Results: Multivariate analyses indicated that BMI, TC, HDL-C, and non-HDL-C increased significantly with increasing total methylxanthines (pcorrected ≤ 0.05). In addition, compared to patients with plasma caffeine concentration in the lowest quartile, those with caffeine concentration in the highest quartile were twice more prone to suffer from non-HDL hypercholesterolemia (pcorrected = 0.05), five times more likely to suffer from hypertriglyceridemia (pcorrected = 0.01) and four times more susceptible to be overweight (pcorrected = 0.01).
Conclusions and Relevance: This study showed that plasma caffeine and other methylxanthines are associated with worsening of metabolic parameters in patients receiving psychotropic treatments known to induce metabolic disturbances. It emphasizes that important caffeine consumption could be considered as an additional environmental risk factor for metabolic worsening in patients receiving such treatments.
Introduction
Coffee is one of the most commonly consumed beverages worldwide and its influence on multiple human health features has been extensively demonstrated (1). A large body of epidemiological studies has examined the association of coffee consumption with various diseases, often yielding inconsistent results. Recent reviews integrating numerous meta-analyses have drawn comprehensive conclusions on the overall effects of coffee consumption. In particular, one recent umbrella review, i.e., a review gathering existing research syntheses of different outcomes for the same intervention, showed that coffee consumption has beneficial effects on a broad range of chronic diseases including cancers, neurological diseases, and cardiovascular diseases (2). However, potential detrimental effects of caffeine consumption on certain health outcomes were also reported, as for instance on serum lipids.
For the last 30 years, multiple epidemiological studies considering association between coffee consumption and serum lipids resulted in inconsistent findings (3–18). More recently, a meta-analysis identified significant positive associations between coffee consumption and serum lipid levels in population-based cohorts, despite an important heterogeneity among considered studies (19). Thus, on average, drinking coffee for 45 days was associated with significant increases of total cholesterol (TC), low-density lipoprotein cholesterol (LDL-C), and triglyceride (TG) of 8.1 mg/dl (0.21 mmol/l), 5.4 mg/dl (0.14 mmol/l), and 12.6 mg/dl (0.14 mmol/l), respectively (19). Interestingly, a dose-response influence of coffee intake on serum lipid levels was recognized, suggesting that the influence of caffeine and/or other coffee components on blood lipid levels may be dose-dependent. Consistent with this hypothesis, methylxanthines including caffeine and its metabolites (i.e., paraxanthine, theobromine, and theophylline) were related with multiple biological effects such as an increased fat oxidation, mobilization of glycogen in muscle as well as an increased lipolysis (20, 21). However, methylxanthines are found in many other widespread-consumed products than coffee (e.g., tea, energy drinks, and chocolate) (22) and their concentration in coffee is highly variable (23). Thus, as previous studies all considered self-reported coffee consumption to evaluate the influence of caffeine and of other methylxanthines on various outcomes of interest, the observed effects may not have been sufficiently accurate.
In psychiatric patients, an important coffee consumption has been reported (24), and higher rates of high caffeine consumers were observed in psychiatric patients compared to individuals from the general population (25–27), which may enhance the risk of developing hyperlipidemia. Sedative and/or anticholinergic effects induced by psychotropic drugs may constitute one possible argument to explain a higher coffee consumption in psychiatric patients than in the general population. In addition, the use of psychotropic medications such as antipsychotics (most atypical but also some typical), mood stabilizers (e.g., lithium and valproate), and some antidepressants (e.g., mirtazapine) can increase the risk of developing metabolic disorders including obesity and dyslipidemia (28–31). These factors, combined with additional risk factors including psychiatric disease-related factors, unhealthy lifestyle, and poverty (32, 33), may therefore contribute to the excessive susceptibility of psychiatric patients for developing cardiovascular diseases.
To the best of our knowledge, no study has yet investigated whether plasma methylxanthine levels are associated with plasma lipid levels and body mass index (BMI), neither in the general nor in the psychiatric population. In the present study, we measured plasma levels of caffeine and its metabolites in a large cohort of psychiatric patients receiving psychotropic treatments known to induce metabolic disturbances, aiming to examine whether plasma methylxanthine levels are associated with lipid parameters and BMI.
Methods
Study Design
Since 2007, a longitudinal observational study (PsyMetab) has been ongoing at the Department of Psychiatry of the Lausanne University Hospital as described elsewhere (34). Patients with informed consent starting or already receiving a psychotropic treatment known to have a potential risk to induce metabolic disturbances (i.e., antipsychotics, mood stabilizers, and some antidepressants, as listed in S1 Table) were included, as described in the flowchart (S1 Figure). More information in Supplementary Material.
Lipid and Methylxanthine Parameters
The majority of blood samples (i.e., 66%) were drawn in fasting conditions. Non-fasting blood samples (i.e., within 6 h following last meal) were excluded only for triglyceride analysis (not for total, HDL- and LDL-cholesterol) (35, 36). LDL-cholesterol was calculated using the Friedewald formula only if triglyceride levels were lower than 3.5 mmol/l (37, 38). In the present study, dyslipidemia was defined as hypercholesterolemia (TC ≥ 5 mmol/l) and/or HDL hypocholesterolemia (HDL-C < 1 mmol/l) and/or LDL hypercholesterolemia (LDL-C ≥ 3 mmol/l) and/or hypertriglyceridemia (TG ≥ 2 mmol/l) and/or if patients were treated with lipid-lowering drugs (S2 Table). More information on quantification of lipids and of methylxanthines (i.e., caffeine, paraxanthine, theophylline, and theobromine) is available in Supplementary Material.
Statistical Analyses
Wilcoxon-Mann-Whitney rank-sum tests and Chi-squared tests were conducted to compare continuous and categorical variables, respectively, across patient groups (e.g., patients stratified by methylxanthine quartiles). Plasma levels of methylxanthine were log-transformed to reduce their variability. The xtmixed procedure in Stata 14 was used to fit linear mixed effect models on plasma lipid levels adjusting for age, sex, smoking status, psychotropic medication, treatment duration, BMI (whenever appropriate), and plasma methylxanthine levels. The fitted linear mixed effect model had a random effect at the subject level.
In order to determine whether and which stratified analyses could be conducted, statistical interactions of possible confounding factors [i.e., age, gender, smoking status, psychotropic medication, treatment duration, and BMI (or TC whenever applicable)] with methylxanthine levels on metabolic outcomes (i.e., lipid levels and BMI) were tested by including interaction terms in linear mixed models adjusting for covariates.
Odds ratio of disturbed metabolic outcomes (e.g., dyslipidemia and overweight) by quartiles of plasma methylxanthines were conducted using logistic mixed effects models adjusting for age, sex, smoking status, psychotropic medication, treatment duration, and BMI or TC, whenever applicable.
Statistical significance was defined as a p-value ≤ 0.05. P-values were adjusted for multiple testing using the false discovery rate (FDR) approach. Statistical analyses were performed using Stata 14 (StataCorp, College Station TX, USA) and R environment for statistical computing version 3.3.1.
Results
Demographics and Plasma Levels of Lipids and Methylxanthines
Demographic and clinical characteristics of the psychiatric sample are displayed in Table 1. Six hundred and thirty patients without missing data were included. Median age was 39 years [interquartile range (IQR): 27–54], psychotic disorders (F20–F24; F28; F29) were the most frequent diagnosis (49%) and quetiapine was the most frequently prescribed psychotropic drug (29%). Half of the patients smoked (50%) and 70% of the selected observation for each single patient was collected within the first 6 months of treatment.
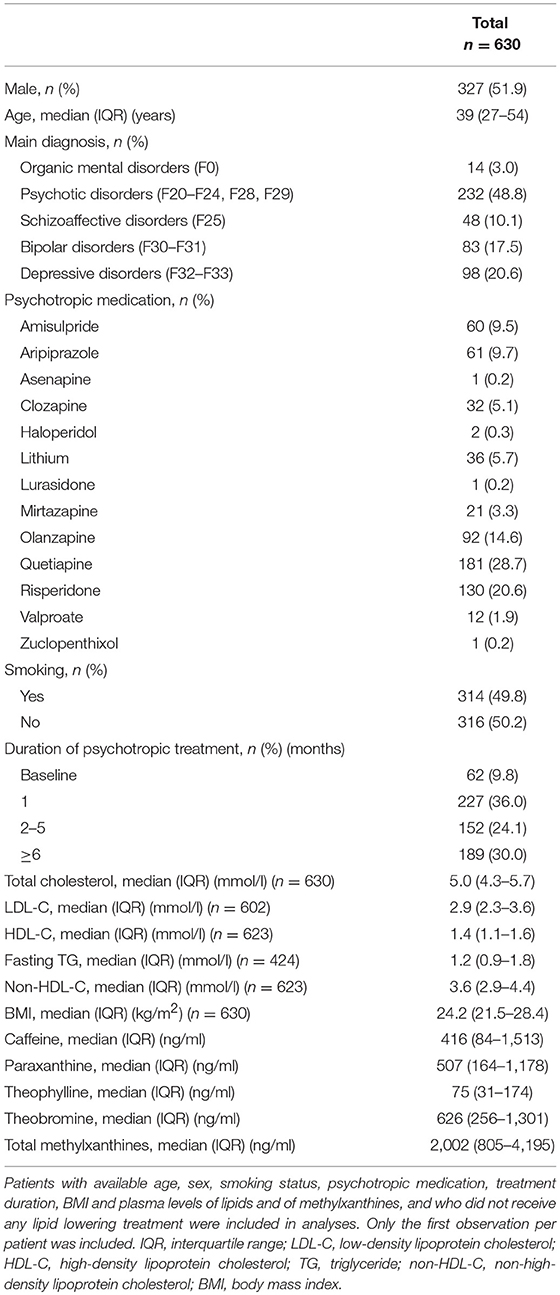
Table 1. Demographic parameters and plasma levels of lipids and of methylxanthines in patients receiving psychotropic drugs.
The evolution of metabolic parameters and of methylxanthine plasma levels during treatment with psychotropic drugs is presented in S3 Table and in Table 2, respectively. Although patients might differ across periods of treatment, a significant worsening of TC, LDL-C, and non-HDL-C levels occurred during treatment with psychotropic drugs. Thus, TC, LDL-C levels, and non-HDL-C increased from 4.8, 2.8, and 3.4 mmol/l at baseline to 5.1, 3.0, and 3.7 mmol/l after 6 months of treatment (p = 0.02, 0.03, and 0.01), respectively. Of note, no significant worsening was observed for HDL-C or TG, possibly attributable to an insufficient power due to the lower number of observations and/or to a lower variation of these parameters. Interestingly, plasma levels of total methylxanthines increased significantly during treatment with psychotropic drugs (pcorrected ≤ 0.05).
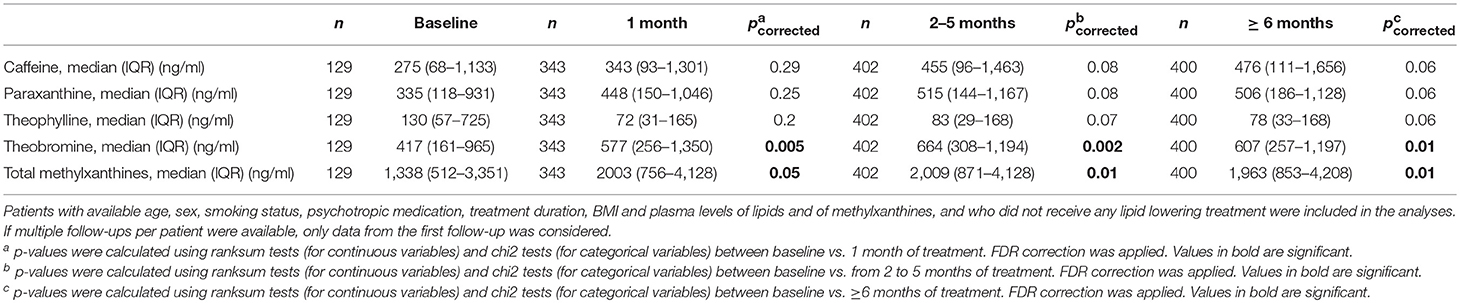
Table 2. Evolution of metabolic (including lipid) and of methylxanthine parameters during psychotropic treatment known to induce metabolic disturbances.
Methylxanthines increased significantly with increasing age (pcorrected ≤ 0.005, S4 Table and S2 Figure) except theobromine. S4 Table shows that women were more prone to have high levels of all methylxanthines but theobromine (pcorrected ≤ 0.04). More interestingly, BMI and all plasma lipid levels but LDL-C significantly increased with increasing quartiles of all methylxanthines (pcorrected ≤ 0.05), except for theobromine. S3.1–S3.6 Figures display distribution of plasma lipid levels and of BMI according to plasma levels of methylxanthines. In accordance with results from methylxanthine quartiles analyses, BMI, and plasma levels of TC, LDL-C, TG, and non-HDL-C increased with increasing levels of methylxanthines, while the association between HDL-C and methylxanthine levels was less apparent. Of note, prevalence of hypercholesterolemia and overweight increased significantly with increasing quartiles of all methylxanthines but theobromine (pcorrected ≤ 0.04; S5 Table). S6 Table shows that neither diagnosis nor psychotropic medication groups were associated with methylxanthine levels. Finally, trends of higher levels of methylxanthines except theobromine were observed in patients receiving clozapine or olanzapine [i.e., drugs with high concomitant affinities for AchM and H1 receptors (39)] as compared to patients who received drugs with less potent anticholinergic and sedative effects (pcorrected = 0.06, S7 Table).
Associations of Plasma Methylxanthines With Metabolic Parameters
Multivariate analyses adjusting for previously mentioned covariates indicated significant associations between plasma methylxanthines and metabolic parameters (S8 Table). In particular, each of the four methylxanthines and their sum (i.e., caffeine, paraxanthine, theophylline, theobromine, and total methylxanthines) were positively associated with TC, HDL-C, and BMI (pcorrected ≤ 0.02, ≤ 0.02, and ≤ 0.02, respectively), but not with LDL-C and TG. In addition, non-HDL-C was positively associated with all methylxanthines except caffeine (pcorrected ≤ 0.05). More information are in Supplementary Material. Further adjusted analyses considering methylxanthine quartiles indicated similar findings, showing significant differences of metabolic variables across methylxanthine quartiles (Table 3). In particular, BMI, TC, HDL-C, and non-HDL-C) were significantly increased with increasing total methylxanthine levels (pcorrected ≤ 0.05). As shown in Figures 1A–C, the odds of displaying non-HDL hypercholesterolemia, hypertriglyceridemia, and overweight was more than doubled for patients in the highest quartile of plasma methylxanthines as compared to those in the lowest quartile (pcorrected ≤ 0.05, pcorrected ≤ 0.03, and pcorrected ≤ 0.047, respectively). In particular, compared to patients with caffeine concentration in the lowest quartile, those with caffeine concentration in the highest quartile were twice more likely to display non-HDL hypercholesterolemia (pcorrected = 0.05), five times more likely to display hypertriglyceridemia (pcorrected = 0.01) and four times more prone to be overweight (pcorrected = 0.01).
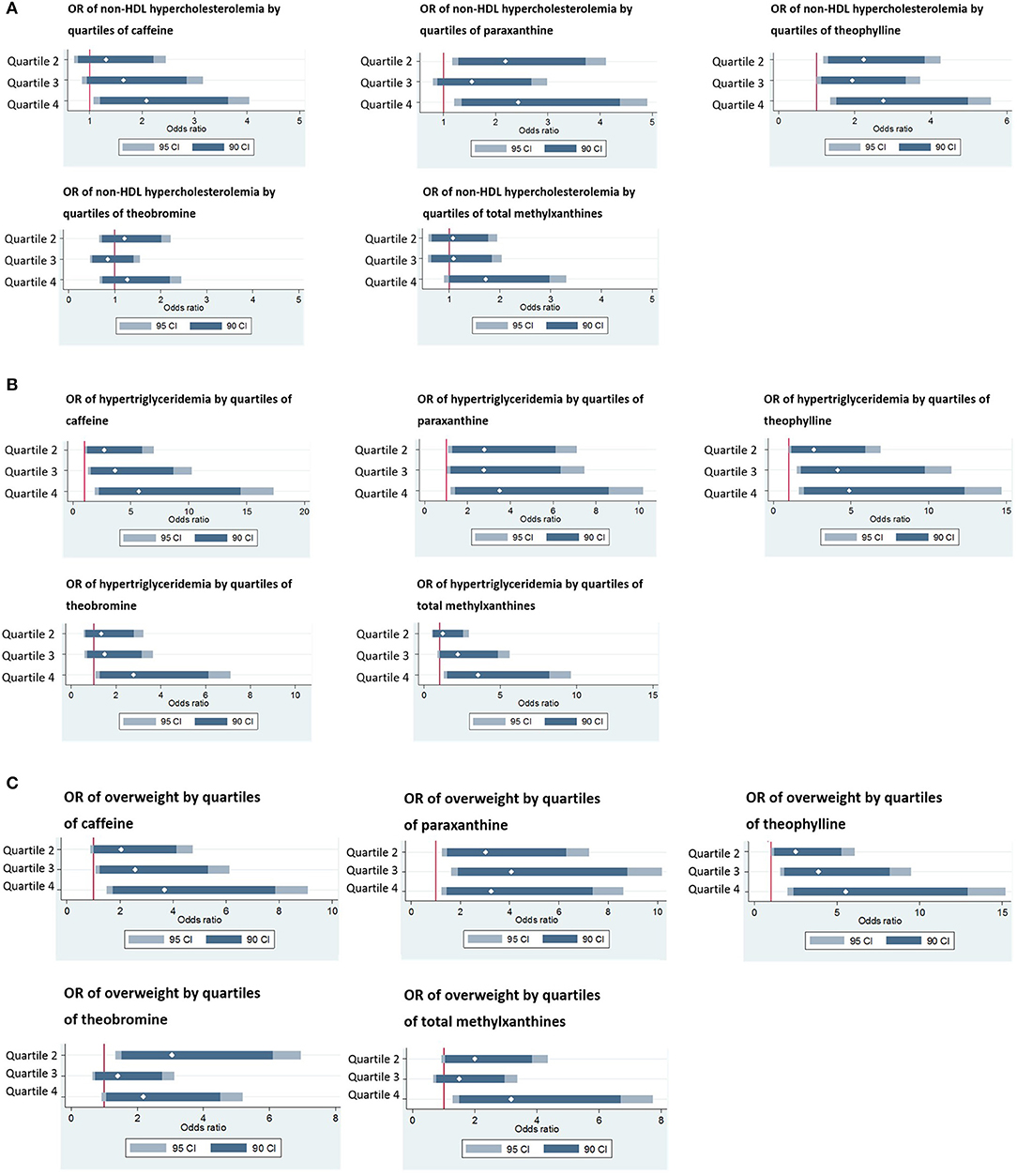
Figure 1. (A) Odds ratio of non-HDL hypercholesterolemia (i.e., abnormal levels of plasma non-HDL cholesterol) by quartiles of plasma methylxanthines. Quartile 1 was considered as the reference. Odds ratio were adjusted for age, sex, smoking status, psychotropic medication, treatment duration and BMI. 95 CI: 95% confidence interval. 90 CI: 90% confidence interval. White diamonds represent estimates of adjusted odd ratio. Log caffeine: Q1: ≤ 4.43 ng/ml; Q2: >4.43– ≤ 6.03 ng/ml; Q3: >6.03– ≤ 7.32 ng/ml; Q4: >7.32 ng/ml; Log paraxanthine: Q1: ≤ 5.1 ng/ml; Q2: >5.1– ≤ 6.23 ng/ml; Q3: >6.23– ≤ 7.07 ng/ml; >7.07 ng/ml; Log theophylline: Q1: ≤ 3.43 ng/ml; Q2: >3.43– ≤ 4.31 ng/ml; Q3: >4.31– ≤ 5.16 ng/ml; Q4: >5.16 ng/ml; Log theobromine: Q1: ≤ 5.55 ng/ml; Q2: >5.55– ≤ 6.44 ng/ml; Q3: >6.44– ≤ 7.17 ng/ml; Q4: >7.17 ng/ml; Log total methylxanthine: Q1: ≤ 6.69 ng/ml; Q2: >6.69– ≤ 7.6 ng/ml; Q3: >7.6– ≤ 8.34 ng/ml; Q4: >8.34 ng/ml. Non-HDL hypercholesterolemia was defined as plasma levels of total cholesterol ≥4 mmol/l, according to ESH/ESC guidelines. (B) Odds ratio of hypertriglyceridemia by quartiles of plasma methylxanthines. Quartile 1 was considered as the reference. Odds ratio (95% CI) were adjusted for age, sex, smoking status, psychotropic medication, treatment duration, and BMI. 95 CI: 95% confidence interval. 90 CI: 90% confidence interval. White diamonds represent estimates of adjusted odd ratio. Log caffeine: Q1: ≤ 4.43 ng/ml; Q2: >4.43– ≤ 6.03 ng/ml; Q3: >6.03– ≤ 7.32 ng/ml; Q4: >7.32 ng/ml; Log paraxanthine: Q1: ≤ 5.1 ng/ml; Q2: >5.1– ≤ 6.23 ng/ml; Q3: >6.23– ≤ 7.07 ng/ml; >7.07 ng/ml; Log theophylline: Q1: ≤ 3.43 ng/ml; Q2: >3.43– ≤ 4.31 ng/ml; Q3: >4.31– ≤ 5.16 ng/ml; Q4: >5.16 ng/ml; Log theobromine: Q1: ≤ 5.55 ng/ml; Q2: >5.55– ≤ 6.44 ng/ml; Q3: >6.44– ≤ 7.17 ng/ml; Q4: >7.17 ng/ml; Log total methylxanthine: Q1: ≤ 6.69 ng/ml; Q2: >6.69– ≤ 7.6 ng/ml; Q3: >7.6– ≤ 8.34 ng/ml; Q4: >8.34 ng/ml. Hypertriglyceridemia was defined as plasma levels of triglyceride ≥2 mmol/l, according to ESH/ESC guidelines. Only patients in fasting conditions were considered in these analyses. (C) Odds ratio of overweight by quartiles of plasma methylxanthines. Quartile 1 was considered as the reference. Odds ratio (95% CI) were adjusted for age, sex, smoking status, psychotropic medication, treatment duration and total cholesterol levels. 95 CI: 95% confidence interval. 90 CI: 90% confidence interval. White diamonds represent estimates of adjusted odd ratio. Log caffeine: Q1: ≤ 4.43 ng/ml; Q2: >4.43– ≤ 6.03 ng/ml; Q3: >6.03– ≤ 7.32 ng/ml; Q4: >7.32 ng/ml; Log paraxanthine: Q1: ≤ 5.1 ng/ml; Q2: >5.1– ≤ 6.23 ng/ml; Q3: >6.23– ≤ 7.07 ng/ml; >7.07 ng/ml; Log theophylline: Q1: ≤ 3.43 ng/ml; Q2: >3.43– ≤ 4.31 ng/ml; Q3: >4.31– ≤ 5.16 ng/ml; Q4: >5.16 ng/ml; Log theobromine: Q1: ≤ 5.55 ng/ml; Q2: >5.55– ≤ 6.44 ng/ml; Q3: >6.44– ≤ 7.17 ng/ml; Q4: >7.17 ng/ml; Log total methylxanthine: Q1: ≤ 6.69 ng/ml; Q2: >6.69– ≤ 7.6 ng/ml; Q3: >7.6– ≤ 8.34 ng/ml; Q4: >8.34 ng/ml. Overweight was defined as body mass index ≥25 kg/m2.
Interaction tests were conducted to determine whether any demographic or clinical variable interacted with plasma methylxanthines on metabolic parameters (i.e., on plasma lipid levels and/or on BMI) in order to recognize which stratified analyses would be beneficial. Analyses on plasma lipid levels reported no significant interaction between gender, smoking status, psychotropic treatment duration or psychotropic treatment category, and methylxanthine concentration on lipids, suggesting that stratification by these four variables would not provide additional information and that the association between methylxanthine levels and metabolic parameters appears to be similar across categories of these four variables (S9 Table). However, age and BMI interacted significantly with methylxanthines on plasma lipid levels and smoking interacted significantly with methylxanthines on BMI (S9 Table; S4 Figure). More information are in Supplementary Material.
Discussion
In the present sample of psychiatric patients, plasma levels of methylxanthines followed an asymmetric right-handed curve, with a small proportion of patients (approximatively half of patients in the fourth quartile, corresponding to around 1/8 of all patients) having particularly elevated methylxanthine concentrations. This right-skewed distribution is in accordance with an observational study conducted in similar settings showing that 13% of psychiatric patients presented a markedly high coffee consumption (24). In the present study, distribution of methylxanthines from blood samples collected in non-fasting conditions was similar than those from blood sample drawn in fasting conditions (data not shown). This suggests that the fasting status did not influence blood levels of methylxanthines.
In accordance with previous studies, metabolic parameters including lipid levels and BMI worsened significantly during treatment with psychotropic drugs (33, 40, 41). In parallel, plasma levels of methylxanthines increased during psychotropic treatment, in accordance with a study suggesting that caffeine consumption may be related to increased thirst due to anticholinergic effects of certain psychotropic drugs (42). In addition, further analyses in the present sample showed that patients receiving drugs with important sedative and anticholinergic effects [i.e., drugs with high concomitant affinities for AchM and H1 receptors, namely clozapine and olanzapine (39)] tended to have higher levels of methylxanthines except theobromine as compared to patients who received drugs with less potent anticholinergic and sedative effects. These findings support the hypothesis of an increased coffee consumption to overcome sedative effects and/or an increased thirst.
The main finding of the present study is that metabolic parameters including BMI and plasma lipid levels were positively associated with plasma levels of methylxanthines in multivariate analyses adjusting for possible confounding variables. These results are consistent with secondary findings from a recent large population-based study on arterial stiffness, showing increased metabolic parameters (i.e., BMI and plasma lipid levels) with increasing quartiles of methylxanthines (43). In addition, our results are in accordance with the most recent meta-analysis evaluating association between coffee consumption and lipid levels, which recognized that, in general population-based cohorts, drinking coffee was associated on average with significant increase of lipid levels (e.g., TC by 0.21 mmol/l) (19). This influence was possibly attributable to biological effects of caffeine (e.g., increased fat oxidation and an increased lipolysis) (20). Of note, in the present study and in agreement with a previous study in the general population (43), increasing quartiles of methylxanthines were associated with higher levels of beneficial cholesterol (i.e., HDL-C). Because TC, TG, and non-HDL-C levels increased to the same extent with increasing methylxanthine quartiles, it seems unlikely that the cardiovascular advantage provided by increased HDL-C levels would counteract detrimental influence of the three other lipid parameters (i.e., TC, TG, and non-HDL-C). Concerning the present positive association between methylxanthine levels and BMI, recent reviews evaluating the influence of coffee consumption on metabolic outcomes observed conflicting results, i.e., that coffee consumption and caffeine intake are associated with a decreased risk for metabolic syndrome (2, 44). Possible mechanisms to explain this reduced risk include that caffeine decreases body weight by increasing energy expenditure (45), and that this molecule induces changes in the structure of gut microbiota (i.e., a decreased Firmicutes to Bacteroidetes ratio), in favor of an anti-obesity profile (46). On the other hand, a recent mendelian randomization study suggested that high caffeine intake is causally associated with high BMI and contrariwise with a lower risk of obesity (47). Thus, the association between methylxanthine levels, BMI, and obesity is still controversial and results from previous studies emphasize the need of future studies to better understand mechanisms underlying these associations.
Plasma levels of methylxanthines increased with the patients' age and reached a plateau around 50 years of age, underlying the importance of considering age as a confounding variable in multivariate analyses. These findings are consistent with population-based studies showing that caffeine intake is age-dependent (48, 49) and with another study showing that the maximum number of cups of coffee per person per day reaches a plateau between 30 and 59 years old (50). In the present study, theobromine levels remained stable across age, showing similar concentrations between teenagers and adults. As coffee intake in teenager individuals was reported to be lower than in adults (42), elevated theobromine concentrations in young patients may be attributed to consumption of other products than coffee, including chocolate foods and beverages, which provide low caffeine but constitute the major source of dietary theobromine (51). Moreover, theobromine accounts for only 7–8% of caffeine metabolism (52, 53) and in the present study, the calculated theobromine/caffeine ratio was close to 1, further supporting that the present plasma levels of theobromine result from other sources than from caffeine metabolism exclusively.
Several interactions were observed between methylxanthines and some clinical variables on metabolic parameters (i.e., on plasma lipid levels and on BMI). For instance, age and BMI interacted significantly with some methylxanthines on some lipid phenotypes, showing that the association between plasma methylxanthines and plasma lipid levels was exclusively applicable in young and lean patients. These findings are consistent with previous studies showing that young and lean patients have a greater risk to develop metabolic side effects than older and heavier patients and that clinical and/or genetic susceptibilities are more easily revealed in these patients than in other patients (33, 34, 54). In the present study, another relevant interaction was observed: positive associations between plasma methylxanthines and BMI were restricted to non-smoking patients. This finding is consistent with another study evaluating the association between caffeine intake and hypertension that reported an influence of caffeine on hypertension in non-smokers but not in smokers (55). One hypothesis to explain this restrictive association is that by inducing CYP1A2 [i.e., the main enzyme responsible for caffeine metabolism (56)], smoking alters plasma concentrations of methylxanthines and may blind the association between methylxanthines and lipid levels. In addition, smoking has been shown to stimulate fat metabolism (57), which can have an impact on BMI and suppress the contribution of methylxanthines on this metabolic variable.
Interestingly, most of our positive findings involved caffeine as well as other methylxanthines (i.e., paraxanthine and theophylline) but not theobromine. These results are in accordance with recent findings (43) and can be partly attributed to the fact that theobromine is less active than caffeine. Thus, theobromine has two- to three-fold lower affinity than caffeine for adenosine receptors A1 and A2A (58) and penetrates the blood-brain barrier less readily than caffeine (59).
Results of the present study should be considered with the following limitations. First, although this study was longitudinal, no link of causality could be drawn between methylxanthines and metabolic parameters. Mendelian randomization analyses would be a valuable approach to evaluate causality. However, much larger sample sizes (typically ≥ 10,000 participants) are required for such analyses (60). Future studies including a higher number of participants should therefore be conducted in order to better understand these associations. Second, although most important factors were considered in the present study, biological half-life of caffeine varies among individuals and is determined by multiple genetic, physiological, and environmental factors (61) that we could not take into account. In addition, time elapsed between the last consumption of caffeine products and the blood draw was not available, which may have increased the variability of methylxanthine levels. However, methylxanthine levels measured at distinct periods of psychotropic treatment in similar individuals were comparable. In addition, considering the sum of all methylxanthines allowed us to take into account inter-individual differences in caffeine metabolism. It must also be mentioned that the present study has been performed in patients receiving psychotropic treatment known to induce metabolic disturbances and the results are therefore not necessarily valid in non-treated psychiatric patients or in patients treated with drugs with no metabolic effects. Strengths of the present study include its naturalistic setting and its relatively large sample size. In addition, plasma levels of methylxanthine were measured instead of using self-reported caffeine consumption, which enhances accuracy of our results.
In conclusion, the present study showed that plasma levels of methylxanthines are positively associated with lipids and BMI in patients receiving psychotropic treatment known to induce metabolic disturbances. In such patients, caffeine consumption could therefore be an additional environmental risk factor that may further worsen their metabolic condition, especially in young patients who are particularly vulnerable to metabolic side effects. However, further research is needed to better understand how the detrimental effect of caffeine on lipids elevation may be counteracted by its beneficial effect on HDL-C. In addition, studies in patients who receive psychotropic treatment inducing metabolic abnormalities and who warrant specific attention (e.g., young patients with elevated coffee consumption) should be initiated to determine whether dietary advices on gradual diminution of coffee consumption should improve their cardiometabolic health.
Ethics Statement
This study was carried out in accordance with the Declaration of Helsinki, the good epidemiological practice written by the Swiss Society of public health, the Swiss law, and local requirements. The study protocol was approved by the Ethic committee of Vaud (CER-VD) with written informed consent from all subjects.
Disclosure
CE received honoraria for conferences or teaching CME courses from Astra Zeneca, Forum für Medizinische Fortbildung, Janssen-Cilag, Lundbeck, Mepha, Otsuka, Sandoz, Servier, Vifor-Pharma, and Zeller in the past 3 years, and for writing a review article for the journal Dialogues in clinical neurosciences (Servier) He received an unrestricted educational research grant from Takeda in the past 3 years. AvG received honoraria for a conference or workshop participation from Vifor and Schwabe in the previous 3 years. SC received honoraria for teaching CME courses from Forum für Medizinische Fortbildung in the past 3 years.
Author Contributions
CE had full access to all of the data in the study and takes responsibility for the integrity of the data and the accuracy of the data analysis. Study concept and design was provided by CE. Acquisition of data was provided by AG, AL, CD, and by AD, FV, NA, SC, AvG, and PC. Analysis and interpretation was provided by AD, IG, MB, and MG-R. Drafting of the manuscript was provided by AD. Critical revision of the manuscript for important intellectual content was provided by all authors. Statistical analysis was provided by AD and MG-R. CE and PC obtained funding for the study. Administrative, technical, or material support was provided by AvG, PC, and CE.
Funding
This work has been funded in part by the Swiss National Research Foundation (CE and PC: 320030-120686, 324730-144064, and 320030-173211). The funding sources had no role in the writing of the manuscript or in the decision to submit it for publication.
Conflict of Interest Statement
The authors declare that the research was conducted in the absence of any commercial or financial relationships that could be construed as a potential conflict of interest.
Acknowledgments
The authors are grateful to C. Brogli and C. Darbellay for logistical assistance, E. Retamales for bibliographical help, A-C. Aubert, A. Vullioud, G. Viret, M. Brawand, M. Brocard, M. Delessert, N. Cochard, and S. Jaquet for sample analysis. The authors thank the nursing and medical staff who were involved in the metabolic monitoring program.
Supplementary Material
The Supplementary Material for this article can be found online at: https://www.frontiersin.org/articles/10.3389/fpsyt.2018.00573/full#supplementary-material
References
1. Higdon JV, Frei B. Coffee and health: a review of recent human research. Crit Rev Food Sci Nutr. (2006) 46:101–23. doi: 10.1080/10408390500400009
2. Grosso G, Godos J, Galvano F, Giovannucci EL Coffee, caffeine, and health outcomes: an umbrella review. Annu Rev Nutr. (2017) 37:131–56. doi: 10.1146/annurev-nutr-071816-064941
3. D'Amicis A, Scaccini C, Tomassi G, Anaclerio M, Stornelli R, Bernini A. Italian style brewed coffee: effect on serum cholesterol in young men. Int J Epidemiol. (1996) 25:513–20. doi: 10.1093/ije/25.3.513
4. Burr ML, Limb ES, Sweetnam PM, Fehily AM, Amarah L, Hutchings A. Instant coffee and cholesterol: a randomised controlled trial. Eur J Clin Nutr. (1995) 49:779–84.
5. Fried RE, Levine DM, Kwiterovich PO, Diamond EL, Wilder LB, Moy TF, et al. The effect of filtered-coffee consumption on plasma lipid levels. Results of a randomized clinical trial. JAMA (1992) 267:811–5. doi: 10.1001/jama.1992.03480060057030
6. Superko HR, Bortz W Jr Williams, PT Albers, JJ, Wood PD. Caffeinated and decaffeinated coffee effects on plasma lipoprotein cholesterol, apolipoproteins, and lipase activity: a controlled, randomized trial. Am J Clin Nutr. (1991) 54:599–605. doi: 10.1093/ajcn/54.3.599
7. van Dusseldorp M, Katan MB, van Vliet T, Demacker PN, Stalenhoef AF. Cholesterol-raising factor from boiled coffee does not pass a paper filter. Arterioscler. Thromb. (1991) 11:586–93. doi: 10.1161/01.ATV.11.3.586
8. Rosmarin PC, Applegate WB, Somes GW. Coffee consumption and serum lipids: a randomized, crossover clinical trial. Am J Med. (1990) 88:349–56. doi: 10.1016/0002-9343(90)90488-Y
9. Burr ML, Gallacher JE, Butland BK, Bolton CH, Downs LG. Coffee, blood pressure and plasma lipids: a randomized controlled trial. Eur J Clin Nutr. (1989) 43:477–83.
10. Bak AA, Grobbee DE. The effect on serum cholesterol levels of coffee brewed by filtering or boiling. N Engl J Med. (1989) 321:1432–7.
11. Aro A, Tuomilehto J, Kostiainen E, Uusitalo U, Pietinen P. Boiled coffee increases serum low density lipoprotein concentration. Metab Clin Exp. (1987) 36:1027–30. doi: 10.1016/0026-0495(87)90021-7
12. Førde OH, Knutsen SF, Arnesen E, Thelle DS. The Tromso heart study: coffee consumption and serum lipid concentrations in men with hypercholesterolaemia: an randomised intervention study. Br Med J. (1985) 290:893–5. doi: 10.1136/bmj.290.6472.893
13. Aro A, Kostiainen E, Huttunen JK, Seppälä, E, Vapaatalo H. Effects of coffee and tea on lipoproteins and prostanoids. Atherosclerosis (1985) 57:123–8. doi: 10.1016/0021-9150(85)90144-3
14. Grubben MJ, Boers GH, Blom HJ, Broekhuizen R, de Jong R, van, Rijt L, et al. Unfiltered coffee increases plasma homocysteine concentrations in healthy volunteers: a randomized trial. Am J Clin Nutr. (2000) 71:480–4. doi: 10.1093/ajcn/71.2.480
15. Yamashita K, Yatsuya H, Muramatsu T, Toyoshima H, Murohara T, Tamakoshi K. Association of coffee consumption with serum adiponectin, leptin, inflammation and metabolic markers in Japanese workers: a cross-sectional study. Nutr Diabetes. (2012) 2:e33. doi: 10.1038/nutd.2012.6
16. Balk L, Hoekstra T, Twisk J. Relationship between long-term coffee consumption and components of the metabolic syndrome: the Amsterdam Growth and Health Longitudinal Study. Eur J Epidemiol. (2009) 24:203–9. doi: 10.1007/s10654-009-9323-1
17. Naidoo N, Chen C, Rebello SA, Speer K, Tai ES, Lee J, et al. Cholesterol-raising diterpenes in types of coffee commonly consumed in Singapore, Indonesia and India and associations with blood lipids: a survey and cross sectional study. Nutr J. (2011) 10:48. doi: 10.1186/1475-2891-10-48
18. Nystad T, Melhus M, Brustad M, Lund E. The effect of coffee consumption on serum total cholesterol in the Sami and Norwegian populations. Public Health Nutr. (2010) 13:1818–25. doi: 10.1017/S1368980010000376
19. Cai L, Ma D, Zhang Y, Liu Z, Wang P. The effect of coffee consumption on serum lipids: a meta-analysis of randomized controlled trials. Eur J Clin Nutr. (2012) 66:872–7. doi: 10.1038/ejcn.2012.68
20. Ranheim T, Halvorsen B. Coffee consumption and human health–beneficial or detrimental?–Mechanisms for effects of coffee consumption on different risk factors for cardiovascular disease and type 2 diabetes mellitus. Mol Nutr Food Res. (2005) 49:274–84. doi: 10.1002/mnfr.200400109
21. Riksen NP, Smits P, Rongen GA. The cardiovascular effects of methylxanthines. Handb Exp Pharmacol. (2011) 200:413–37. doi: 10.1007/978-3-642-13443-2_16
22. Frary CD, Johnson RK, Wang MQ. Food sources and intakes of caffeine in the diets of persons in the United States. J Am Diet Assoc. (2005) 105:110–3. doi: 10.1016/j.jada.2004.10.027
23. Fox GP, Wu A, Yiran L, Force L. Variation in caffeine concentration in single coffee beans. J Agric Food Chem. (2013) 61:10772–8. doi: 10.1021/jf4011388
24. Rihs M, Muller C, Baumann P. Caffeine consumption in hospitalized psychiatric patients. Eur Arch Psychiatry Clin Neurosci. (1996) 246:83–92. doi: 10.1007/BF02274898
25. Furlong FW. Possible psychiatric significance of excessive coffee consumption. Canad Psychiatr Assoc J. (1975) 20:577–83.
26. Greden JF, Fontaine P, Lubetsky M, Chamberlin K. Anxiety and depression associated with caffeinism among psychiatric inpatients. Am J Psychiatry (1978) 135:963–6.
27. Victor BS, Lubetsky M, Greden JF. Somatic manifestations of caffeinism. J Clin Psychiatry (1981) 42:185–8.
28. Correll CU, Detraux J, De Lepeleire J, De Hert M. Effects of antipsychotics, antidepressants and mood stabilizers on risk for physical diseases in people with schizophrenia, depression and bipolar disorder. World Psychiatry (2015) 14:119–36. doi: 10.1002/wps.20204
29. Olfson M, Marcus SC, Corey-Lisle P, Tuomari AV, Hines P, L'Italien GJ. Hyperlipidemia following treatment with antipsychotic medications. Am J Psychiatry (2006) 163:1821–5. doi: 10.1176/ajp.2006.163.10.1821
30. Vancampfort D, Vansteelandt K, Correll CU, Mitchell AJ, De Herdt A, Sienaert P, et al. Metabolic syndrome and metabolic abnormalities in bipolar disorder: a meta-analysis of prevalence rates and moderators. Am J Psychiatry (2013) 170:265–74. doi: 10.1176/appi.ajp.2012.12050620
31. Vancampfort D, Correll CU, Wampers M, Sienaert P, Mitchell AJ, De Herdt A, et al. Metabolic syndrome and metabolic abnormalities in patients with major depressive disorder: a meta-analysis of prevalences and moderating variables. Psychol Med. (2014) 44:2017–28. doi: 10.1017/S0033291713002778
32. Correll CU, Lencz T, Malhotra AK. Antipsychotic drugs and obesity. Trends Mol Med. (2011) 17:97–107. doi: 10.1016/j.molmed.2010.10.010
33. De Hert M, Detraux J, van Winkel R, Yu W, Correll CU. Metabolic and cardiovascular adverse effects associated with antipsychotic drugs. Nat Rev Endocrinol. (2012) 8:114–26. doi: 10.1038/nrendo.2011.156
34. Choong E, Quteineh L, Cardinaux JR, Gholam-Rezaee M, Vandenberghe F, Dobrinas M, et al. Influence of CRTC1 polymorphisms on body mass index and fat mass in psychiatric patients and the general adult population. JAMA Psychiatry (2013) 70:1011–9. doi: 10.1001/jamapsychiatry.2013.187
35. Doran B, Guo Y, Xu J, Weintraub H, Mora S, Maron DJ, et al. Prognostic value of fasting versus nonfasting low-density lipoprotein cholesterol levels on long-term mortality: insight from the National Health and Nutrition Examination Survey III (NHANES-III). Circulation (2014) 130:546–53. doi: 10.1161/CIRCULATIONAHA.114.010001
36. Nordestgaard BG, Varbo A. Triglycerides and cardiovascular disease. Lancet (2014) 384:626–35. doi: 10.1016/S0140-6736(14)61177-6
37. Third Report of the National Cholesterol Education Program (NCEP) Expert panel on detection, and and, treatment of high blood cholesterol in adults (adult treatment panel III) final report Circulation (2002) 106:3143–21.
38. Friedewald, WT, Levy RI, Fredrickson DS. Estimation of the concentration of low-density lipoprotein cholesterol in plasma, without use of the preparative ultracentrifuge. Clin Chem. (1972) 18:499–502.
39. Kusumi I, Boku S, Takahashi Y. Psychopharmacology of atypical antipsychotic drugs: From the receptor binding profile to neuroprotection and neurogenesis. Psychiatry Clin Neurosci. (2015) 69:243–58. doi: 10.1111/pcn.12242
40. Delacrétaz A, Vandenberghe F, Gholam-Rezaee M, Saigi Morgui N, Glatard A, Thonney J et al. Early changes of blood lipid levels during psychotropic drug treatment as predictors of long-term lipid changes and of new onset dyslipidemia. J Clin Lipidol. (2017) 12:219–9. doi: 10.1016/j.jacl.2017.10.002
41. Vandenberghe F, Gholam-Rezaee M, Saigí-Morgui N, Delacrétaz A, Choong E, Solida-Tozzi A, et al. Importance of early weight changes to predict long-term weight gain during psychotropic drug treatment. J Clin Psychiatry (2015) 76:e1417–23. doi: 10.4088/JCP.14m09358
42. Stephenson PE. Physiologic and psychotropic effects of caffeine on man. A review. J Am Diet Assoc. (1977) 71:240–7.
43. Ponte B, Pruijm M, Ackermann D, Ehret G, Ansermot N, Staessen JA, et al. Associations of urinary caffeine and caffeine metabolites with arterial stiffness in a large population-based study. Mayo Clin Proc. (2018) 93:586–96. doi: 10.1016/j.mayocp.2017.12.010
44. Poole R, Kennedy OJ, Roderick P, Fallowfield JA, Hayes PC, Parkes J. Coffee consumption and health: umbrella review of meta-analyses of multiple health outcomes. BMJ (2017) 359:j5024. doi: 10.1136/bmj.j5024
45. Greenberg JA, Boozer CN, Geliebter A. Coffee, diabetes, and weight control. Am J Clin Nutr. (2006) 84:682–93. doi: 10.1093/ajcn/84.4.682
46. Pan MH, Tung YC, Yang G, Li S, Ho CT. Molecular mechanisms of the anti-obesity effect of bioactive compounds in tea and coffee. Food Funct. (2016) 7:4481–91. doi: 10.1039/C6FO01168C
47. Nordestgaard AT, Thomsen M, Nordestgaard BG. Coffee intake and risk of obesity, metabolic syndrome and type 2 diabetes: a Mendelian randomization study. Int J Epidemiol. (2015) 44:551–65. doi: 10.1093/ije/dyv083
48. Mitchell DC, Knight CA, Hockenberry J, Teplansky R, Hartman TJ. Beverage caffeine intakes in the U.S. Food Chem Toxicol. (2014) 63:136–42. doi: 10.1016/j.fct.2013.10.042
49. Tran NL, Barraj LM, Bi X, Jack MM. Trends and patterns of caffeine consumption among US teenagers and young adults, NHANES 2003-2012. Food Chem Toxicol. (2016) 94:227–42. doi: 10.1016/j.fct.2016.06.007
50. Greden JF. WA Caffeine. In: Lowinson JH, Ruiz P, Millman RB, Langrod JG, editors, Substance Abuse: A Comprehensive Textbook. Baltimore, MD: Williams & Wilkins (1992), p. 357–70.
51. Shively CA, Tarka SM. Methylxanthine composition and consumption patterns of cocoa and chocolate products. Progr Clin Biol Res. (1984) 158:149–78.
52. Kot M, Daniel WA. The relative contribution of human cytochrome P450 isoforms to the four caffeine oxidation pathways: an in vitro comparative study with cDNA-expressed P450s including CYP2C isoforms. Biochem Pharmacol. (2008) 76:543–51. doi: 10.1016/j.bcp.2008.05.025
53. Thorn CF, Aklillu E, McDonagh EM, Klein TE, Altman RB. PharmGKB summary: caffeine pathway. Pharmacogenet Genom. (2012) 22:389–95. doi: 10.1097/FPC.0b013e3283505d5e
54. Delacrétaz A, Preisig M, Vandenberghe F, Saigi Morgui N, Quteineh L, Choong E et al. Influence of MCHR2 and MCHR2-AS1 genetic polymorphisms on body mass index in psychiatric patients and in population-based subjects with present or past atypical depression. PLoS ONE (2015) 10:e0139155. doi: 10.1371/journal.pone.0139155
55. Guessous I, Dobrinas M, Kutalik Z, Pruijm M, Ehret G, Maillard M, et al. Caffeine intake and CYP1A2 variants associated with high caffeine intake protect non-smokers from hypertension. Hum Mol Genet. (2012) 21:3283–92. doi: 10.1093/hmg/dds137
56. Zevin S, Benowitz NL. Drug interactions with tobacco smoking. An update. Clin Pharmacokinet. (1999) 36:425–38. doi: 10.2165/00003088-199936060-00004
57. Harris KK, Zopey M, Friedman TC. Metabolic effects of smoking cessation. Nat Rev Endocrinol. (2016) 12:299–308. doi: 10.1038/nrendo.2016.32
58. Ferre S. An update on the mechanisms of the psychostimulant effects of caffeine. J Neurochem. (2008) 105:1067–79. doi: 10.1111/j.1471-4159.2007.05196.x
59. Svenningsson P, Nomikos GG, Fredholm BB. The stimulatory action and the development of tolerance to caffeine is associated with alterations in gene expression in specific brain regions. J Neurosci. (1999) 19:4011–22. doi: 10.1523/JNEUROSCI.19-10-04011.1999
60. Burgess S. Sample size and power calculations in Mendelian randomization with a single instrumental variable and a binary outcome. Int J Epidemiol. (2014) 43:922–9. doi: 10.1093/ije/dyu005
Keywords: psychotropic drugs, lipids, plasma caffeine level, plasma methylxanthines, metabolic parameters, secondary effects
Citation: Delacrétaz A, Vandenberghe F, Glatard A, Levier A, Dubath C, Ansermot N, Crettol S, Gholam-Rezaee M, Guessous I, Bochud M, von Gunten A, Conus P and Eap CB (2018) Association Between Plasma Caffeine and Other Methylxanthines and Metabolic Parameters in a Psychiatric Population Treated With Psychotropic Drugs Inducing Metabolic Disturbances. Front. Psychiatry 9:573. doi: 10.3389/fpsyt.2018.00573
Received: 09 July 2018; Accepted: 19 October 2018;
Published: 09 November 2018.
Edited by:
Marijn Lijffijt, Baylor College of Medicine, United StatesReviewed by:
Elena Martín-García, Universidad Pompeu Fabra, SpainOscar Prospéro-García, Universidad Nacional Autónoma de México, Mexico
Copyright © 2018 Delacrétaz, Vandenberghe, Glatard, Levier, Dubath, Ansermot, Crettol, Gholam-Rezaee, Guessous, Bochud, von Gunten, Conus and Eap. This is an open-access article distributed under the terms of the Creative Commons Attribution License (CC BY). The use, distribution or reproduction in other forums is permitted, provided the original author(s) and the copyright owner(s) are credited and that the original publication in this journal is cited, in accordance with accepted academic practice. No use, distribution or reproduction is permitted which does not comply with these terms.
*Correspondence: Chin B. Eap, Y2hpbi5lYXBAY2h1di5jaA==