- 1Department of Psychiatry, The Second Xiangya Hospital, Central South University, Changsha, China
- 2Mental Health Institute, The Second Xiangya Hospital, Central South University, Changsha, China
- 3Chinese National Clinical Research Center on Mental Disorders, Second Xiangya Hospital, Central South University, Changsha, China
- 4National Clinical Research Center on Mental Disorders, Changsha, China
- 5National Technology Institute on Mental Disorders, Changsha, China
- 6Hunan Key Laboratory of Psychiatry and Mental Health, Changsha, China
- 7Department of Psychological Sciences, Texas Tech University, Lubbock, TX, United States
- 8Department of Radiology, Hunan Provincial People's Hospital, Changsha, China
- 9Department of Psychiatry, Yale University School of Medicine, New Haven, CT, United States
Background: The medial prefrontal cortex (mPFC) contains various neurotransmitter systems and plays an important role in drug use. Broad body of literature on how methamphetamine (MA) affects the structure and metabolism in the animal's mPFC is emerging, while the effects on metabolites of mPFC among human is still unclear. In this study, proton magnetic resonance spectroscopy (1H MRS) was used to measure metabolites of mPFC in methamphetamine dependent subjects.
Methods: Sixty-one subjects with a history of MA dependence (fulfiled the Diagnostic and Statistical Manual of Mental Disorders, fourth edition criteria) and 65 drug-naïve control subjects (age19–45) completed 1H MRS scans using 3.0T Siemens MRI scanner. Single voxel spectra were acquired from the mPFC bilaterally using a point resolved spectroscopy sequence (PRESS). The 1H MRS data were automatically fit with linear combination model for quantification of metabolite levels of n-acetyl-aspartate (NAA), myo-inositol (mI), glycerophosphocholine plus phosphocholine(GPC+PC), phosphocreatine plus creatine (PCr+Cr), and glutamate (Glu). Metabolite levels were reported as ratios to PCr+Cr.
Results: The MA group showed a significant reduction in NAA/PCr+Cr ratio and elevation in Glu/PCr+Cr ratio and mI/PCr+Cr ratio, compared with healthy control. No significant correlation was found between metabolite ratios and MA use variables.
Conclusions: MA use is associated with a significant increased Glu/PCr+Cr ratio, mI/PCr+Cr ratio and reduced NAA/PCr+Cr ratio in the mPFC of MA dependence subjects. These findings suggest that Glu may play a key role in MA induced neurotoxicity.
Introduction
Methamphetamine is one of the most consumed amphetamine-type stimulants (ATS) worldwide. According to the World Drug Report 2017 (1), global methamphetamine seizures reached a new peak of 132 tons, increased 21% than previous year, accounting for 60–80% of ATS seizures annually. As MA use is spreading and the treatment demand is growing, evidence on effective treatment is scarce while MA represents the greatest global health burden among ATS (1). There is abundant evidence that MA cause long-lasting impairment to the brain both in preclinical and clinical research. In preclinical studies, chronic MA exposure activated microglials (2) and astrocytes, and increased inflammatory mediators and other oxidative stress related factors (3). While in human neuroimaging studies demonstrated that chronic MA use leads to serious brain changes, including dopaminergic (4, 5), monoaminergic (6), and serotoninergic (7, 8) neurotransmitter system, cerebral glucose metabolism (9, 10), structure and integrity (9–11).
1H magnetic resonance spectroscopy (1H MRS) provides an invasive method to explore the metabolites in the brain. Previous 1H MRS research on MA dependent (MAD) subjects shows alterations in n-acetyl-aspartate (NAA) (12–18), choline (Cho) (12, 13, 16, 17, 19), myo-inositol (mI) (13, 14), and glutamate (Glu) or Glx (meaning glutamate+glutamine) (15, 20) concentrations or the ratios of these metabolites to creatine (Cr). Most of these studies focused on anterior cingulate (12, 16–18), basal ganglia (13, 19), frontal gray (13) and white matter (13–15), with less evidence in the medial prefrontal cortex (mPFC).
The mPFC is a terminal region of the mesocorticolimbic dopamine system which has been reported to modulate reward seeking behavior (21, 22) and is associated with drug addiction (23). The functional connectivity of the mPFC is decreased in various mental disorders (24–26), including addiction (27, 28). Previous studies suggested ATS dependent subjects have smaller volume (29–31) and decreased gray matter density (32) in the mPFC. To date, there is no report on measuring metabolite levels in the mPFC in MA users relative to healthy subjects. The mPFC contains pyramidal glutamatergic neurons that project to numerous regions (33) and repeated amphetamine administration alters a-amino-3-hydroxy-5-methylisoxazole-4-propionic acid (AMPA) receptor subunits mRNA levels in rat mPFC (34). Thus, metabolite levels in the mPFC may be different between methamphetamine dependent (MAD) subjects and healthy chontrols, especially Glu. In this present study, we performed a semi-quantitative analysis to quantify the levels of NAA, mI, GPC+PC, and Glu of the mPFC in MAD subjects. In this study, we aimed to investigate whether MA use significantly altered metabolite ratios to PCr+Cr in the mPFC. It was hypothesized that first, MA use would be associated with altered metabolite levels in the mPFC, and second, the altered metabolite levels would be significantly correlated with MA use variables, including age of first MA use (years old), duration of MA use (months), and duration of abstinence from MA use (days). In addition, we explored possible relationship between cigarettes smoked per day (CPD), Beck Depression Inventory (BDI) score, State-Trait Anxiety Inventory (STAI) scores and the metabolite levels in MA users.
Method
Subjects
The data were collected as a part of the brain imaging study on methamphetamine-induced psychotic symptoms, a study hosted at the Second Xiangya Hospital of Central South University. One hundred and twenty-six subjects (61 MAD subjects and 65 drug-free healthy subjects, age 19–45) were enrolled in this study. Subjects between 18 and 45 years were entitled to participate in the study. MAD volunteers were recruited from The Kangda Voluntary Drug Rehabilitation Centers in Hunan Province. All MA users fulfiled the Diagnostic and Statistical Manual of Mental Disorders, fourth edition (DSM-IV) criteria (35) for lifetime MA dependence assessed by the Structured Clinical Interview (SCID) (36). MAD subjects were excluded if they met criteria for other substance dependence (excluding nicotine dependence) at any time. Subjects were required to abstain from MA for at least 48 h before scanning. Drug free healthy control subjects were recruited from community through advertising. Participants were excluded if they (i) had any general medical condition or neurological disorder, including infectious, hepatic or endocrine disease; (ii) had a history of severe head injury with skull fracture or loss of consciousness of more than 10 min; (iii) had any current or previous psychiatric disorder; (iv) had a family history of psychiatric disorder; (v) women during pregnant or breast-feed stage; (vi) had contraindications for MRI. Two licensed psychiatrist, at MD level, conducted all clinical interviews. Subjects were fully informed about the measurement and MRI scanning in the study. Written informed consent was given by all subjects. This study was approved by the Ethics Committee of the Second Xiangya Hospital, Central South University (No. S095, 2013) and was carried out in accordance with the Declaration of Helsinki. We used the BDI-II to measure the depression symptoms in the last week before undergoing MRI scans. STAI was used to measure anxiety level before MRI scanning (STAI-Y1), and anxiety level as a personal characteristic (STAI-Y2). The demographic characteristic were shown in Table 1.
Magnetic Resonance Data Acquisition
Structural MRI and MRS data were acquired with a Siemens Magnetom Trio 3.0 T MR scanner (Siemens, Erlangen, Germany) using an eight-channel standard quadrature headcoil at the Magnetic Resonance Center of Hunan provincial People's Hospital, China. Three-dimensional T1-weighted images were collected using a gradient echo sequence (repetition time = 2,000 ms, echo time = 2.26 ms, field of view = 256 × 256 mm, flip angle = 8°, matrix size = 256 × 256, number of slices = 176, slice thickness = 1 mm). Using these images, a single 1H MRS voxel was placed on the corpus callosumand centered on the intrahemispheric fissure, including medial superior frontal gyrus and anterior cingulate cortices, not containing the orbitofrontal cortex (see Figure 1). 1H MRS was performed using a short-echo point resolved spectroscopy sequence (PRESS; repetition time = 1,500 ms; echo time = 30 ms; voxel size 30 × 25 × 30 mm; number of scans = 256, spectral bandwidth = 1,200 Hz, the number of data points = 1,024 points). Water suppression was achieved using a chemical shift selective (CHESS) sequence.
1H MRS spectra were automatically fit with linear combination model (LCModel version 6.3–1B [LCMODEL Inc. CA (37)] at the Second Affiliated Hospital, Shantou University Medical College located in Shantou, Guangdong, China. Metabolite concentration for NAA, PCr+Cr, GPC+PC, mI and Glu were acquired using LCModel software (Figure 2). The signal-to-noise ratio (S/N) and full width at half maximum (FWHM) of each spectrum were checked for quality to ensure they were adequate for reliable peak fitting for the metabolites of interest. Only those spectra with FWHM ≤ 0.1 ppm and S/N ≥ 20 were retained. Furthermore, only those metabolite speaks satisfying the LCModel criterion less than 20% of Cramer-Rao lower bound (CRLB) value were reported here. PCr+Cr severs as a reference for other metabolite peaks on the assumption that its concentration is relatively constant. We first observed that there was no absolute PCr+Cr difference prior to forming ratios with respect to PCr+Cr. The common practice of normalization by PCr+Cr removd the across-subject variability, which arises from technical factors, such as coil loading. From these data, the metabolite ratios of NAA/PCr+Cr, mI/PCr+Cr, Glu/PCr+Cr, and GPC+PC/PCr+Cr were reported here.
Segmentation was performed on T1-weighted images using New Segment+DARTEL in Data Processing & Analysis of Brain Imaging (38). Estimation of tissue volume was collected from the normalized gray matter, white matter, and cerebro-spinal fluid (CSF) images using custom Matlab (The Mathworks, Inc.) code (http://www.cs.ucl.ac.uk/staff/G.Ridgway/vbm/get_totals.m). GM fractions, WM fractions and CSF fractions in the MAD subjects and controls were shown in Table 2. No statistical significance was found between the two groups.
Statistical Analysis
Statistical analysis was performed with SPSS 20 (IBM Inc. New York, USA). Assumption of normality of each variable was tested with the Shapiro–Wilk test. Because of non-normality of the data, CPD and BDI scores were compared using a Mann-Whitney U test, and gender with Chi-square test for independence. Metabolite concentrations were reported as mean ± standard deviation. General Linear Model multivariate analysis was used to evaluate group differences in metabolite ratios controlling for CPD and gray matter tissue fraction in the voxle. Correlation analyses between metabolite ratios and each of clinical parameters, including age, months of MA use, days of abstinence, age of onset of MA use, CPD, BDI, total STAI scores, STAI-Y1 score, and STAI-Y2 score were performed using Pearson's or Spearman's correlation analysis, followed by Bonferroni test. Statistical significance was defined at p < 0.05, two-tailed.
Results
Demographic Characteristic
The groups did not differ in gender, mean age, BMI, or years of education (Table 1). The CPD (p < 0.01) in MAD subjects was higher than those in healthy controls. The BDI score (p < 0.01) was higher in MAD subjects. There were no significant differences in the total STAI scores (p = 0.17), STAI-Y1 (p = 0.37), or STAI-Y2 (p = 0.12) between the two groups.
Tissue Composition Within Voxels of Interest
Segmentation indicates the fractional contribution of gray matter, white matter and CSF in the MAD group = 44% gray, 30% white and 23% CSF and in healthy control group = 46% gray, 32% white and 23% CSF. No differences in the fractions of gray (p = 0.06), white (p = 0.10), and CSF (p = 0.85) were detected between MAD subjects and controls.
FWHM, S/N, and CRLB Values of MRS Data
There was no difference between the MAD group and control group in FWHM (p = 0.23). There was significant difference in S/N between MAD group and healthy control group (p < 0.01). There were significant differences between the two groups in CRLB values for NAA (p < 0.01), mI (p < 0.01), GPC+PC (p = 0.01) and Glu (p < 0.01) (see Table 3).
1H MRS Metabolite Ratios
The MAD group and control group did not differ significantly in absolute PCr+Cr values (mean = 6.47 vs. 6.58, p = 0.44), which served as the denominator for the ratios tested. Compared with healthy controls, MAD subjects had significant decreased NAA/PCr+Cr ratios (mean = 1.12 vs. 1.17, p = 0.02), increased mI/PCr+Cr ratios (mean = 0.85 vs. 0.80, p < 0.01) and Glu/PCr+Cr ratios (mean = 1.03 vs. 0.95, p < 0.01) in the mPFC. There was not significant difference in GPC+PC/PCr+Cr between the two groups (mean = 0.27 vs. 0.27, p = 0.73) (see Table 4).
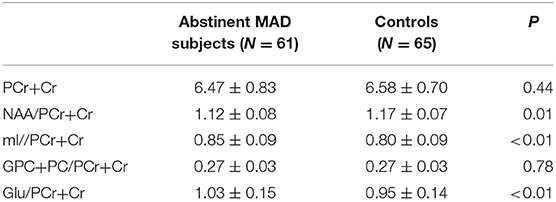
Table 4. Metabolite concentrations in the mPFC of MAD subjects (n = 61) and healthy controls(n = 65).
1H-MRS Metabolite Levels With MA Use, Age, CPD, and Anxiety
For the MA users, there were no significant correlation between ratios of metabolites and MA use variables. There were no significant correlation between age, CPD and metabolite ratios.
Discussion
There was no significant difference in PCr+Cr levels between MAD group and control group, so the differences in NAA/PCr+Cr, mI/PCr+Cr, and Glu/PCr+Cr between groups are probably due to the differences in NAA, mI and Glu levels between groups. The first finding of the present study is that the NAA/PCr+Cr ratio was increased in the mPFC of MAD subjects. Finding the decreased ratio of NAA/PCr+Cr among MAD subjects is consistent with previous studies (12, 16, 18, 39, 40) and as well as other psychiatric disorders (41). NAA is taken as a neuronal marker, and reflects neuronal integrity, viability and number (42). NAA plays a key role in enhancing mitochondrial energy production from Glu (42) and also reflects functional status of neuronal mitochondria (43). The changes of NAA/PCr+Cr may reflect adverse neuron function disorder. Besides, the pathological deletion of dendrite or degeneration of neuron may be related with decreased NAA. The present finding suggests that there is decreased neuronal integrity, viability, number, or mitochondraial dysfunction in the mPFC in MAD individuals, even though they have been abstinent from MA.
The second finding is that the ratio of mI/PCr+Cr was significantly increased in the mPFC of MAD subjects compared with healthy controls. The increased ratio of mI/PCr+Cr in MAD group is in line with previous studies, which were reported in frontal gray matter and white matter of MA users (13, 14, 44). Mostly mI is considered as a marker of glial (45). Some studies suggested that Ins is an osmoregulator (46) and contributes to glucose storage (47) and is a precursor in the PI-cycle second messenger system (47). Elevation of mI may suggest that proliferation of glial cells or inflammation due to the damage of neurons induced by methamphetamine, which is a marker of MA induced neurons damage after NAA decreased. MA has been reported to induced gliosis in vitro and in animal experiment. After acute administration of MA to rats (48) and vervet monkeys (49) induced glial activation, and gliosis remains after stopped exposure one and half a year (49).
The final finding is a significant increase in ratios of Glu/PCr+Cr in the mPFC of MAD subjects. The most consistent alteration across MA abuse was reduction in NAA, while change in Glu was inconsistent. Glx and Glu were reported to be lower in the mPFC (39), precuneus, posterior cingulate, and right inferior frontal cortex (20), while Glu was repored to be higher in frontal white matter of abstinent MAD subjects (15). But the Glu levels in the posterior gray matter did not differ with HC (15). In one study, in the frontal gray matter of the MA users, the Glx concentration reduced during early abstinence, reached relatively normal after 1–2 months, and exceeded normal levels in longer-term abstinence (50). In the Crocker's study, the concentration levels of Glu in the MA group was reduced relative to HC and schizophrenia patients (39). In another study, however, Glu levels in the ACC and DLPFC did not differ between MAD subjects and controls (12). Several variables may contribute to these inconsistencies. These studies evaluated different brain regions and used different field strength. Other variables include the length of time using MA, dose, frequency of use, duration of abstinence and the sample size.
This is a relatively large sample study to report of increased ratio of Glu/PCr+Cr in the mPFC among MAD subjects, although preclinical studies have found such findings (51–53). Glu is the major excitatory neurotransmitter, and most of Glu is present intracellularly. Extracellular Glu released from nerve terminals is taken up by Glu receptors and Glu transporters present presynaptically, postsynaptically and extrasynaptically in glial cells, preventing Glu excitotoxicity (54). Glu in the glial cells is converted to glutamine (Gln) through an ATP-dependent process in mitochondria. Gln is subsequently released from the glial cells and taken up by Gln transporters in neurons. Gln in neurons is converted back to Glu. This is the “glutamate–glutamine cycle,” which represents 40–50% of the total flux from the TCA cycle (54). Therefore, dysfunction of Glu receptors or Glu transporters may be a possible explanation for elevated Glu concentration in the mPFC. The elevated Glu concentration suggests that the Gln converted from Glu is decreased and decreased activities of glutamateric neurons. Meanwhile the increased extracellular Glu concentration is neurotoxic.
In the research conducted by Sailasuta et al., around 36% of normal glial tricarboxylic acid (TCA) cycle rate was significant reduced in frontal brain of abstinent MA abusers, which may impact the glutamate-glutamine cyle and thus result in accumulation of Glu (55). Therefore, the other possible explanation of the increased Glu concentration in our study is the consequence of dysfunction of glial cells.
Furthermore, the increased extracellular Glu concentration is considered as an important factor of relapse. Glutamateric signal system plays an important role in drug-seeking behavior. It has been reported that the activation of Glu transporter (56), or gene expression of the transporter in the nucleus accumbens (57) plays an inhibitory role in MA conditioned place preference (CPP), while mPFC is acritical region for reactivation of the MA-CPP memory (58). Glu released from the mPFC stimulates dopamine release in the ventral tegmental area and the nucleus accumbens (59), while dopaminergic system is considered as primary mechanism initiating drug reinstatement (60). In a preclinical study, the increased expression of the Glu transporter attenuated the MA-CPP, which reduced drug-seeking behavior (61).
Limitations
This study has several limitations. First, most of subjects in this study were men, making it impossible to explore the influence of gender confidently. Second, the characteristics of MAD subjects in this study, including the range of duration of MA use and abstinence, and the drug used in the abstinence would be possible confounding factors. Third, the study is cross-sectional, it is unclear whether alterations in these metabolites would reverse completely during continued abstinence or would persist. Furthermore, MAD subjects had a higher CPD than the controls, and this difference was significant. We noted this difference, controlled this covariate in statistical analysis process and discussed their possible impacts on our MRS results. We concluded that this difference in CPD between the MAD subjects and healthy controls is unlikely to be the reason for the statistically significant alterations of metabolites of the mPFC. Our study is a relatively large sample and we controlled the cigarette smoking when comparing the metabolites. Finally, there were significant differences between MAD subjects and controls in the S/N ratios and CRLB values. But the S/N ratios and CRLB values in both groups characterize relatively good quality of our data. Future studies should include more women MAD subjects, measure alterations longitudinally when MAD subjects using MA (if possible), at the beginning of abstinence, and after longer duration (6–24 months) of abstinence, and match subjects' cigarette smoking.
Conclusion
Our findings suggest that the alterations in ratios of Glu/PCr+Cr of the mPFC may underlie the pathophysiology of neurological injury in MA abuse. MA cause the Glu concentration elevation, which has neurotoxicity and may lead to NAA concentration decreased and mI increased. This study implicates that Glu plays an important role in MA dependent disorder, reducing Glu concentration or increasing the activity of Glu receptors in the mPFC may be of great clinical significance in the treatment.
Author Contributions
YL, JT, JLi, and TL contributed conception and design of the study. QW, CQ, and AX organized the database. QW performed the statistical analysis and wrote the first draft of the manuscript. JLo, YL, XW, YT, and JT revised the manuscript. TL, BY and WH advised on the statistical analysis, interpretation of findings, and reviewed drafts of the manuscript.
Funding
This study was supported by the National Natural Science Foundation of China (Grant No. 81371465 and 81671324 to TL, and 81671325 to YL), Natural Science Foundation of Hunan Province (Grant No. 14JJ4075 to JLo) and Hunan Provincial Innovation Foundation for Postgraduate (Grant No. CX2017B071 to QW).
Conflict of Interest Statement
The authors declare that the research was conducted in the absence of any commercial or financial relationships that could be construed as a potential conflict of interest.
Acknowledgments
We acknowledge all the professionals in Kangda Voluntary Drug Rehabilitation Centers who helped a lot in data collection. The authors thank all the subjects who participated in this study.
References
1. United Nations Office on Drugs and Crime. World Drug Report. United Nations publication, Sales No. 2 E.17.XI.6. (2017).
2. Thomas DM, Walker PD, Benjamins JA, Geddes TJ, Kuhn DM. Methamphetamine neurotoxicity in dopamine nerve endings of the striatum is associated with microglial activation. J Pharmacol Exp Ther. (2004)311:1–7. doi: 10.1124/jpet.104.070961
3. Yamamoto BK, Bankson MG. Amphetamine neurotoxicity: cause and consequence of oxidative stress. Crit Rev Neurobiol. (2005)17:87–117. doi: 10.1615/CritRevNeurobiol.v17.i2.30
4. Sekine Y, Minabe Y, Ouchi Y, Takei N, Iyo M, Nakamura K, et al. Association of dopamine transporter loss in the orbitofrontal and dorsolateral prefrontal cortices with methamphetamine-related psychiatric symptoms. Am J Psychiatry. (2003)160:1699–1701. doi: 10.1176/appi.ajp.160.9.1699
5. Volkow ND, Chang L, Wang GJ, Fowler JS, Leonido-Yee M, Franceschi D, et al. Association of dopamine transporter reduction with psychomotor impairment in methamphetamine abusers. Am J Psychiatry. (2001)158:377–82. doi: 10.1176/appi.ajp.158.3.377
6. McFadden LM, Stout KA, Vieira-Brock PL, Allen SC, Nielsen SM, Wilkins DG, et al. Methamphetamine self-administration acutely decreases monoaminergic transporter function. Synapse. (2012)66:240–5. doi: 10.1002/syn.21506
7. Sekine Y, Ouchi Y, Takei N, Yoshikawa E, Nakamura K, Futatsubashi M, et al. Brain serotonin transporter density and aggression in abstinent methamphetamine abusers. Arch Gen Sychiatry. (2006)63:90–100. doi: 10.1001/archpsyc.63.1.90
8. Kish SJ, Fitzmaurice PS, Boileau I, Schmunk GA, Ang LC, Furukawa Y, et al. Brain serotonin transporter in human methamphetamine users. Psychopharmacology (Berl). (2009)202:649–61. doi: 10.1007/s00213-008-1346-x
9. Volkow ND, Chang L, Wang GJ, Fowler JS, Franceschi D, Sedler MJ, et al. Higher cortical and lower subcortical metabolism in detoxified methamphetamine abusers. Am J Psychiatry (2001)158:383–389. doi: 10.1176/appi.ajp.158.3.383
10. Berman SM, Voytek B, Mandelkern MA, Hassid BD, Isaacson A, et al. Changes in cerebral glucose metabolism during early abstinence from chronic methamphetamine abuse. Mol Psychiatry (2008)13:897–908. doi: 10.1038/sj.mp.4002107
11. Chung A, Lyoo IK, Kim SJ, Hwang J, Bae SC, Sung YH, et al. Decreased frontal white-matter integrity in abstinent methamphetamine abusers. Int J Neuropsychopharmacol. (2007)10:765–75. doi: 10.1017/S1461145706007395
12. Howells FM, Uhlmann A, Temmingh H, Sinclair H, Meintjes E, Wilson D, et al. (1)H-magnetic resonance spectroscopy ((1H)-MRS) in methamphetamine dependence and methamphetamine induced psychosis. Schizophr Res. (2014)153:122–8.doi: 10.1016/j.schres.2014.01.029
13. Ernst T, Chang L, Leonido-Yee M, Speck O. Evidence for long-term neurotoxicity associated with methamphetamine abuse: a 1H MRS study. Neurology (2000)54:1344–9. doi: 10.1212/WNL.54.6.1344
14. Sung YH, Cho SC, Hwang J, Kim SJ, Kim H, Bae S, et al. Relationship between N-acetyl-aspartate in gray and white matter of abstinent methamphetamine abusers and their history of drug abuse: a proton magnetic resonance spectroscopy study. Drug Alcohol Depend. (2007)88:28–35. doi: 10.1016/j.drugalcdep.2006.09.011
15. Sailasuta N, Abulseoud O, Hernandez M, Haghani P, Ross BD. Metabolic abnormalities in abstinent methamphetamine dependent subjects. Subst Abuse. (2010)2014:9–20. doi: 10.4137/SART.S4625
16. Nordahl TE, Salo R, Natsuaki Y, Galloway GP, Waters C, Moore CD, et al. Methamphetamine users in sustained abstinence: a proton magnetic resonance spectroscopy study. Arch Gen Psychiatry. (2005)62:444–52. doi: 10.1001/archpsyc.62.4.444
17. Salo R, Nordahl TE, Natsuaki Y, Leamon MH, Galloway GP, Waters C, et al. Attentional control and brain metabolite levels in methamphetamine abusers. Biol Psychiatry (2007)61:1272–80. doi: 10.1016/j.biopsych.2006.07.031
18. Nordahl TE, Salo R, Possin K, Gibson DR, Flynn N, Leamon M, et al. Low N-acetyl-aspartate and high choline in the anterior cingulum of recently abstinent methamphetamine-dependent subjects: a preliminary proton MRS study. Psychiatry Res. (2002)116:43–52. doi: 10.1016/S0925-4927(02)00088-4
19. Sekine Y, Minabe Y, Kawai M, Suzuki K, Iyo M, Isoda H, et al. Metabolite alterations in basal ganglia associated with methamphetamine-related psychiatric symptoms. A proton MRS study. Neuropsychopharmacology (2002)27:453–61. doi: 10.1016/S0893-133X(02)00321-4
20. O'Neill J, Tobias MC, Hudkins M, London ED. Glutamatergic neurometabolites during early abstinence from chronic methamphetamine abuse. Int J Neuropsychopharmacol. (2014) 18:pyu059. doi: 10.1093/ijnp/pyu059
21. Kim CK, Ye L, Jennings JH, Pichamoorthy N, Tang DD, Yoo AW, et al. Molecular and circuit-dynamical identification of top-down neural mechanisms for restraint of reward seeking. Cell (2017)170:1013–27.e14. doi: 10.1016/j.cell.2017.07.020
22. Ferenczi EA, Zalocusky KA, Liston C, Grosenick L, Warden MR, Amatya D, et al. Prefrontal cortical regulation of brain wide circuit dynamics and reward-related behavior. Science (2016) 351:aac9698. doi: 10.1126/science.aac9698
23. Steketee JD. Neurotransmitter systems of the medial prefrontal cortex: potential role in sensitization to psychostimulants. Brain Res Rev. (2003)41:203–228. doi: 10.1016/S0165-0173(02)00233-3
24. Wang S, Zhang Y, Lv L, Wu R, Fan X, Zhao J, et al. Abnormal regional homogeneity as a potential imaging biomarke for adolescent-onset schizophrenia:a resting-state fMRI study and support vector machine analysis. Schizophr Res. (2018)192:179–84. doi: 10.1016/j.schres.2017.05.038
25. Guo W, Cui X, Liu F, Chen J, Xie G, Wu R, et al. Increased anterior default-mode network homogeneity in first-episode, drug-naive major depressive disorder: a replication study. J Affect Disord. (2018)225:767–72. doi: 10.1016/j.jad.2017.08.089
26. Wu G, Wang Y, Mwansisya TE, Pu W, Zhang H, Liu C, et al. Effective connectivity of the posterior cingulate and medial prefrontal cortices relates to working memory impairment in schizophrenic and bipolar patients. Schizophr Res. (2014)158:85–90. doi: 10.1016/j.schres.2014.06.033
27. Gu H, Salmeron BJ, Ross TJ, Geng X, Zhan W, Stein EA, et al. Mesocorticolimbic circuits are impaired in chronic cocaine users as demonstrated by resting-state functional connectivity. Neuroimage (2010)53:593–601. doi: 10.1016/j.neuroimage.2010.06.066
28. Tang J, Fornito A, Liu T, Chen X, Chen H, et al. Alterations in regional homogeneity of resting-state brain activity in ketamine addicts. Neurosci Lett. (2012)522:36–40. doi: 10.1016/j.neulet.2012.06.009
29. Daumann J, Koester P, Becker B, Wagner D, Imperati D, Gouzoulis-Mayfrank E, et al. Medial prefrontal gray matter volume reductions in users of amphetamine-type stimulants revealed by combined tract-based spatial statistics and voxel-based morphometry. Neuroimage (2011)54:794–801. doi: 10.1016/j.neuroimage.2010.08.065
30. Becker B, Wagner D, Koester P, Tittgemeyer M, Mercer-Chalmers-Bender K, Hurlemann R, et al. Smaller amygdala and medial prefrontal cortex predict escalating stimulant use. Brain (2015) 138(Pt 7):2074–86. doi: 10.1093/brain/awv113
31. Aoki Y, Orikabe L, Takayanagi Y, Yahata N, Mozue Y, Sudo Y, et al. Volume reductions in frontopolar and left perisylvian cortices in methamphetamine induced psychosis. Schizophr Res. (2013)147:355–61. doi: 10.1016/j.schres.2013.04.029
32. Kim SJ, Lyoo IK, Hwang J, Chung A, Hoon Sung Y, Kim J et al. Prefrontal grey-matter changes in short-term and long-term abstinent methamphetamine abusers. Int J Neuropsychopharmacol. (2006)9:221–8. doi: 10.1017/S1461145705005699
33. Sesack SR, Deutch AY, Roth RH, Bunney BS. Topographical organization of the efferent projections of the medial prefrontal cortex in the rat: an anterograde tract-tracing study with Phaseolus vulgaris leucoagglutinin. J Comp Neurol. (1989)290:213–42.
34. Lu W, Chen H, Xue CJ, Wolf ME. Repeated amphetamine administration alters AMPA receptor subunits expression in rat nucleus accumbens and medial prefrontal cortex. Synapse (1997)26:269–80.
35. Spitzer RL, Williams JB, Gibbon M, First MB. The structured clinical interview for DSM-III-R (SCID). I: History, rationale, and description. Arch Gen Psychiatry. (1992)49:624–9.
36. American Psychiatric Association. Diagnostic and Statistical Manual for Mental Disorders, 4th Edn. (DSM-IV). Washington, DC: American Psychiatric Association (2000). doi: 10.1176/appi.books.9780890423349
37. Provencher SW. Estimation of metabolite concentrations from localized in vivo proton NMR spectra. Magn Reson Med. (1993)30:672–9.
38. Yan CG, Wang XD, Zuo XN, Zang YF. DPABI: Data Processing & Analysis for (Resting-State) Brain Imaging. Neuroinformatics (2016)14:339–51. doi: 10.1007/s12021-016-9299-4
39. Crocker CE, Bernier DC, Hanstock CC, Lakusta B, Purdon SE, Seres P, et al. Prefrontal glutamate levels differentiate early phase schizophrenia and methamphetamine addiction: a (1)H MRS study at 3 Tesla. Schizophr Res. (2014)157:231–7. doi: 10.1016/j.schres.2014.05.004
40. Burger A, Brooks SJ, Stein DJ, Howells FM. The impact of acute and short-term methamphetamine abstinence on brain metabolites: a proton magnetic resonance spectroscopy chemical shift imaging study. Drug Alcohol Depend. (2018)185:226–37. doi: 10.1016/j.drugalcdep.2017.11.029
41. Zong X, Hu M, Li Z, Cao H, He Y, Liao Y, et al. N-acetylaspartate reduction in the medial prefrontal cortex following 8 weeks of risperidone treatment in first-episode drug-naïve schizophrenia patients. Sci Rep. (2015)5:9109. doi: 10.1038/srep09109
42. Moffett JR, Ross B, Arun P, Madhavarao CN, Namboodiri AM. N-Acetylaspartate in the CNS: from neurodiagnostics to neurobiology. Prog Neurobiol. (2007)81:89–131. doi: 10.1016/j.pneurobio.2006.12.003
43. Paslakis G, Träber F, Roberz J, Block W, Jessen F. N-acetyl-aspartate (NAA) as a correlate of pharmacological treatment in psychiatric disorders: a systematic review. Eur Neuropsychopharmacol. (2014)24:1659–75. doi: 10.1016/j.euroneuro.2014.06.004
44. Chang L, Ernst T, Speck O, Grob CS. Additive effects of HIV and chronic methamphetamine use on brain metabolite abnormalities. Am J Psychiatry (2005)162:361–9. doi: 10.1176/appi.ajp.162.2.361
45. Brand A, Richter-Landsberg C, Leibfritz D. Multinuclear NMR studies on the energy metabolism of glial and neuronal cells. Dev Neurosci. (1993)15:289. doi: 10.1159/000111347
46. Nakanishi T, Turner RJ, Burg MB. Osmoregulatory changes in myo-inositol transport by renal cells. Proc Natl Acad Sci USA. (1989)86:6002–6.
47. Ross BD. Biochemical considerations in 1H spectroscopy. Glutamate and glutamine; myo-inositol and related metabolites. NMR Biomed. (1991)4:59–63.
48. Hebert MA, O'Callaghan JP. Protein phosphorylation cascades associated with methamphetamine-induced glial activation. Ann NY Acad Sci. (2000)914:238–62. doi: 10.1111/j.1749-6632.2000.tb05200.x
49. Harveya DC, Lacana G, Taniousa SP, Melega WP. Recovery from methamphetamine induced long-term nigrostriatal dopaminergic deficits without substantia nigra cell loss. Brain Res. (2000)871:259–70. doi: 10.1016/S0006-8993(00)02439-2
50. Ernst T, Chang L. Adaptation of brain glutamate plus glutamine during abstinence from chronic methamphetamine use. J Neuroimmune Pharmacol. (2008)3:165–72. doi: 10.1007/s11481-008-9108-4
51. Abekawa T, Ohmori T, Koyama T. Effects of repeated administration of a high dose of methamphetamine on dopamine and glutamate release in rat striatum and nucleus accumbens. Brain Res. (1994)643:276–81. doi: 10.1016/0006-8993(94)90033-7
52. Stephans SE, Yamamoto BY. Effect of repeated methamphetamine administrations on dopamine and glutamate efflux in rat prefrontal cortex. Brain Res. (1995)700:99–106. doi: 10.1016/0006-8993(95)00938-M
53. Parsegian A, See RE. Dysregulation of dopamine and glutamate release in the prefrontal cortex and nucleus accumbens following methamphetamine self-administration and during reinstatement in rats. Neuropsychopharmacology (2014)39:811–22. doi: 10.1038/npp.2013.231
54. Danbolt NC. Glutamate uptake. Prog Neurobiol. (2001)65:1–105. doi: 10.1016/S0301-0082(00)00067-8
55. Sailasuta N, Abulseoud O, Harris KC, Ross BD. Glial dysfunction inabstinent methamphetamine abusers. J Cereb Blood Flow Metab. (2010)30:950–60. doi: 10.1038/jcbfm.2009.261
56. Nakagawa T, Fujio M, Ozawa T, Minami M, Satoh M. Effect of MS-153, a glutamate transporter activator, on the conditioned rewarding effects of morphine, methamphetamine and cocaine in mice. Behav Brain Res. (2005)156:233–9. doi: 10.1016/j.bbr.2004.05.029
57. Fujio M, Nakagawa T, Sekiya Y, Ozawa T, Suzuki Y, Minami M, et al. Gene transfer of GLT-1, a glutamate transporter, into the nucleus accumbens shell attenuates methamphetamine- and orphine-induced conditioned place preference in rats. Eur J Neurosci. (2005)22:2744–54. doi: 10.1111/j.1460-9568.2005.04467.x
58. Chiang CY, Cherng CG, Lai YT, Fan HY, Chuang JY, Kao GS, et al. Medial prefrontal cortex and nucleus accumbens core are involved in retrieval of the methamphetamine-associated memory. Behav Brain Res. (2009)197:24–30. doi: 10.1016/j.bbr.2008.07.030
59. Krystal JH, D'Souza DC, Mathalon D, Perry E, Belger A, Hoffman R. NMDA receptor antagonist effects, cortical glutamatergic function, and schizophrenia:toward a paradigm shift in medication development. Psychopharmacology(Berl). (2003)169:215–33. doi: 10.1007/s00213-003-1582-z
60. Willuhn I, Wanat MJ, Clark JJ, Phillips PE. Dopamine signaling in the nucleus accumbens of animals self-administering drugs of abuse. Curr Top Behav Neurosci. (2010)3:29–71. doi: 10.1007/7854_2009_27
Keywords: methamphetamine, mPF, NAA/PCr+Cr, Glu/PCr+Cr, mI/PCr+Cr, 1H MRS
Citation: Wu Q, Qi C, Long J, Liao Y, Wang X, Xie A, Liu J, Hao W, Tang Y, Yang B, Liu T and Tang J (2018) Metabolites Alterations in the Medial Prefrontal Cortex of Methamphetamine Users in Abstinence: A 1H MRS Study. Front. Psychiatry 9:478. doi: 10.3389/fpsyt.2018.00478
Received: 12 May 2018; Accepted: 12 September 2018;
Published: 15 October 2018.
Edited by:
Feng Liu, Tianjin Medical University General Hospital, ChinaReviewed by:
Gabriele Ende, Zentralinstitut für Seelische Gesundheit (ZI), GermanyJeffrey A. Stanley, Wayne State University School of Medicine, United States
Copyright © 2018 Wu, Qi, Long, Liao, Wang, Xie, Liu, Hao, Tang, Yang, Liu and Tang. This is an open-access article distributed under the terms of the Creative Commons Attribution License (CC BY). The use, distribution or reproduction in other forums is permitted, provided the original author(s) and the copyright owner(s) are credited and that the original publication in this journal is cited, in accordance with accepted academic practice. No use, distribution or reproduction is permitted which does not comply with these terms.
*Correspondence: Tieqiao Liu, bGl1dGllcWlhbzEyM0Bjc3UuZWR1LmNu