- 1Department of Brain and Cognitive Sciences, College of Natural Sciences, Seoul National University, Seoul, South Korea
- 2Department of Neuropsychiatry, Seoul National University Hospital, Seoul, South Korea
- 3Institute of Human Behavioral Medicine, Medical Research Center, Seoul National University, Seoul, South Korea
- 4Department of Psychiatry, College of Medicine, Seoul National University, Seoul, South Korea
Introduction: The fundamental role of the cerebellum in higher cognitive processing has recently been highlighted. However, inconsistent findings exist in schizophrenia with respect to the exact nature of cerebellar structural abnormalities and their associations with cognitive and clinical features.
Materials and Methods: We undertook a detailed investigation of cerebellar lobular volumes in 40 patients with first-episode psychosis (FEP) and 40 healthy controls (HCs) using the spatially unbiased atlas template of the cerebellum (SUIT). We examined the functional significance of cerebellar structural abnormalities in relation to cognitive and clinical outcomes in patients.
Results: We found that left cerebellar lobules VI and X volumes were lower in FEP patients, compared to HCs. Smaller left lobules VI and X volumes were associated with fewer number of categories completed on the Wisconsin Card Sorting Test (WCST) in patients. In addition, smaller left lobule X volume was related to performance delay on the Trail Making Test (TMT) Part B in patients.
Conclusion: Our results demonstrate that cerebellar structural abnormalities are present at the early stage of schizophrenia. We suggest functional associations of cerebellar structural changes with non-verbal executive dysfunctions in FEP.
Introduction
Increasing evidence suggests that the cerebellum is associated with cerebral networks involved in cognitive and emotional processes, as well as motor control (1–3). Neuropsychological studies have shown that the cerebellum is associated with major neurocognitive domains such as processing speed, working memory, verbal fluency, and executive function (4–7). Neuroimaging studies have further shown that the lateral hemispheres of the posterior cerebellum (lobules VI–IX) and flocculonodular lobe (lobule X) were associated with cognitive functions, while the anterior lobe (lobules I-V) was primarily responsible for motor functions (2, 8–10). Although the aforementioned neurocognitive domains are known to be impaired in schizophrenia (11), it is still not fully understood how these neurocognitive domains are associated with specific cerebellar volumetric abnormalities in schizophrenia.
To date, the majority of neuroimaging studies in schizophrenia have reported some aspects of cerebellar abnormalities, although they are mostly inconsistent. A smaller whole cerebellar volume has been reported (12–14), but not replicated (15–17). In fact, Wilke and colleagues have even reported larger cerebellar volume in patients (18). Of studies seeking specific cerebellar regional volume deficits in schizophrenia, smaller volumes in the posterior superior lobe, such as lobule VI and Crus I/II, have been reported (19–22). Other studies have also reported smaller volume in the anterior or posterior inferior lobe, including lobule X (20, 23–25). Yet again, some studies found larger or no different volume in such regions (26–29). Relatively few studies have investigated relationships between cerebellar volumetric differences and neurocognitive deficits in schizophrenia with varying cerebellar foci (21, 30–32). Hence, it is yet to be determined whether cerebellar structure-function relationships are present in schizophrenia.
Potential reasons for this inconsistency in cerebellar structural findings in schizophrenia include the use of different methodological procedures to analyze cerebellar volumes. One of three methodologies has been used to extract cerebellar volumes: manual delineation, automated segmentation based on image normalization to whole-brain template, or automated segmentation using an isolated cerebellar image. In the absence of automated segmentation methods, the manual delineation method was used to measure cerebellar volumes (23, 25, 33). Later, researchers segmented cerebellar tissues after registering brain images into a whole-brain standard reference such as the Montreal Neurological Institute (MNI) template (34). This was widely adopted into the whole-brain voxel-based morphometry (VBM) method (35) and FreeSurfer pipeline (36). However, it had an innate issue of poor alignment of cerebellar structures across individuals, which limited anatomical details of cerebellar lobules (37). Thus, it was suggested to use isolated cerebellar images and a cerebellum-specific template such as the spatially unbiased atlas template of the cerebellum (SUIT) to improve the overlap of cerebellar structures across participants (37–39). Hence, the cerebellum-specific methods are expected to produce more consistent findings for cerebellar structural abnormalities in schizophrenia.
One of the other reasons for these inconsistent findings includes the illness chronicity of patients (40–42). It is critical to understand whether cerebellar abnormalities are present at the early phase of this illness, or whether they are associated with illness progression. However, most previous studies used a chronic sample of schizophrenia patients, producing confounding effects due to relatively varying degrees of illness duration, age, and medication. Like studies of chronic patients, studies of first-episode psychosis (FEP) patients have reported that the overall cerebellar gray matter (GM) volume was smaller compared to healthy controls (HCs) (14, 22). Furthermore, longitudinal studies have shown a reduced GM volume in the right cerebellum in high-risk individuals who subsequently developed schizophrenia (43, 44). Although these studies support the hypothesis that cerebellar abnormalities are not a result of the adverse effects from chronic deteriorating course of the illness or medications, relationships between neurocognitive functions and cerebellar lobular abnormalities have not been investigated in FEP.
In the present study, we conducted a detailed investigation of cerebellar lobules in 40 FEP patients and 40 HCs using SUIT that retained anatomical details within infra-tentorial structures. We also wished to examine the functional significance of cerebellar structural abnormalities in relation to cognitive and clinical outcomes in patients compared to HCs. Using FEP subjects, instead of chronic patients, minimizes the confounding effects resulting from both illness chronicity and long-term antipsychotic medications on cerebellar structural abnormalities. We hypothesized that FEP patients would exhibit smaller volumes in cerebellar lobules, especially in the posterior lobe. In addition, these structural abnormalities would have correlations with clinical and neurocognitive function measures in patients.
Materials and Methods
Participants
Forty patients with FEP were recruited between April 2010 and June 2016 from a prospective cohort study in the Seoul Youth Clinic (www.youthclinic.org), a center for early detection and intervention of individuals at high-risk for psychosis (45). The diagnosis of FEP was confirmed using the Structured Clinical Interview for DSM-IV Axis I Disorders, Patient Edition [SCID-I/P; (46)] by experienced psychiatrists. All patients had a history of less than 1 year since their first psychotic episode, which was defined as suffering from a brief psychotic disorder (N = 1), schizophreniform disorder (N = 11), schizoaffective disorder (N = 3), schizophrenia (N = 24), or psychotic disorder, not otherwise specified (N = 1), according to the DSM-IV-TR criteria. Twenty-nine patients were treated with antipsychotic medications, while others were not being medicated. We administered the Positive and Negative Syndrome Scale (PANSS) (47) and the Global Assessment of Functioning (GAF) (48) to assess symptom severity and overall functioning of patients. Forty HCs were recruited through internet advertisements (Table 1) and screened with SCID-I, Non-Patient Version [SCID-I/NP; (49)]. Exclusion criteria for all participants were brain injury or neurological disorders, major psychiatric disorders other than psychotic disorders, substance abuse, or Intelligence Quotient (IQ) below 70. After a complete description of the study was provided to participants, written informed consent was obtained. The Institutional Review Board of Seoul National University Hospital approved this study.
Neurocognitive Function Tests
Estimated IQ was obtained using the short form of the Korean version of the Wechsler Adult Intelligence Scale (WAIS) (50). A battery of neurocognitive function tests was used to assess the following neurocognitive domains in all participants: for cognitive processing speed, the Trail Making Test (TMT) Part A was used (51); visuospatial memory was assessed using the Rey-Osterrieth Complex Figure Test (RCFT), consisting of immediate and delayed recall stages (52); verbal executive function was assessed in letter and category fluency tasks using the Korean version of the Controlled Oral Word Association (COWA) test (53, 54); and the TMT Part B and the Wisconsin Card Sorting Test (WCST) were administered to assess non-verbal executive function (51, 55).
Image Acquisition and Processing
All participants were scanned with a 3T Trio magnetic resonance imaging (MRI) scanner (Siemens Magnetom Trio, Erlangen, Germany) using a 12-channel head coil at Seoul National University Hospital. The T1-weighted anatomical image was acquired using magnetization-prepared rapid gradient echo (MPRAGE) imaging (echo time [TE]/repetition time [TR] = 1.89/1670 ms, field of view [FOV] = 250 mm, flip angle = 9°, matrix = 256 × 256, voxel size = 1.0 × 1.0 × 1.0 mm3, 208 slices). The time required to acquire the T1 image was 234 s.
Structural T1 images were preprocessed using the SUIT toolbox (37) implemented in the Statistical Parametric Mapping toolbox version 12 (SPM12; http://www.fil.ion.ucl.ac.uk/spm/). The images were segmented into GM, white matter (WM), and cerebrospinal fluid (CSF) using the unified segmentation algorithm (56). The cerebellum and brainstem were then isolated and normalized into a study-specific template using the Dartel algorithm that applied tissue segmentation maps (57, 58). Lastly, the images were resliced into the SUIT space using flow field and affine transformation matrix.
Based on the structural images registered into the SUIT space, we calculated volumes of all 28 cerebellar lobules. In addition to the SUIT processing, T1-weighted images were processed using FreeSurfer v.5.3.0 to obtain cerebellar hemispheric GM/WM volumes and total intracranial volume (TIV) (59).
Statistical Analyses
All statistical analyses were performed using SPSS, version 23 (IBM, Armonk, N.Y.). Demographics and neurocognitive function scores were compared between FEP and HCs using two-tailed independent samples t-test. To assess influence of IQ on neurocognitive function, we additionally conducted ANCOVA with IQ as a covariate for tests of neurocognitive differences.
One-way analysis of covariance (ANCOVA) with TIV as a covariate was conducted between FEP and HCs on cerebellar hemispheric and each of the 28 cerebellar lobular volumes. As previous studies suggested a possibility of sex differences in cerebellum abnormalities in schizophrenia (26, 60), we also conducted subgroup analyses of cerebellar lobular volume differences between FEP and HCs by sex. We addressed the problem of false positives during multiple comparisons based on the weighted multiple testing correction, which approximates multivariate normal distribution using correlation matrix (61, 62). The method of multiple comparisons correction assumes (1) that test statistics are asymptotically distributed as multivariate normal with known correlation matrix and (2) that the statistical power depends on the assigned weights and the extent of correlation among endpoints (62).
In each of the groups, we conducted partial correlation analyses between cerebellar volumes and neurocognitive functions, using TIV as a covariate. In the patient group, the partial correlations between cerebellar volumes and clinical outcomes were also analyzed with TIV as a covariate. We further conducted linear regression analyses with each neurocognitive function as a dependent variable, with group, cerebellar volume, TIV, and group-by-volume interaction term as predictors. The results of the analyses were not adjusted for multiple comparisons.
Results
Demographic and Clinical Characteristics
Demographic and clinical variables are shown in Table 1. There were no significant differences between patients and HCs for sex, age, or handedness. However, FEP patients had lower estimated IQ than HCs [t(77) = 6.39, p < 0.001]. The two groups did not differ significantly in TIV.
Neurocognitive Functions
As shown in Table 2, FEP patients showed delayed reaction time (RT) in the TMT Part A [t(64) = −4.54, p < 0.001] and Part B [t(47) = −4.10, p < 0.001]. The accuracy of both immediate [t(72) = 2.69, p < 0.01] and delayed recall [t(69) = 3.44, p < 0.01] of the RCFT were lower in FEP patients. Both letter [t(72) = 4.81, p < 0.001] and category [t(72) = 6.02, p < 0.001] verbal fluency scores of the COWA were significantly lower in FEP patients. In addition, patients also had more perseverative errors [t(49) = −2.49, p < 0.05] and less categories completed [t(57) = 2.13, p < 0.05] on the WCST compared to HCs. All the neurocognitive functions, other than category fluency of the COWA [F(1, 71) = 10.49, p = .002], were no longer significantly different when we adjusted IQ as a covariate.
Cerebellar Hemispheric and Lobular Volumetric Comparisons
We found no group differences in left and right cerebellar hemispheric GM/WM volumes. As can be seen in Figure 1, we observed significantly smaller GM volumes in FEP patients in left lobule VI [F(1, 77) = 6.66, corrected p < 0.05] and left lobule X [F(1, 77) = 6.77, corrected p < 0.05], when controlling for TIV. Full details on between-group differences including trend level findings are presented in Table 3. There was a significantly smaller volume in vermis lobule VI [F(1, 34) = 6.90, corrected p < 0.05] when comparing male subjects between patients and HCs, while none of the cerebellar lobules were different in female subjects.
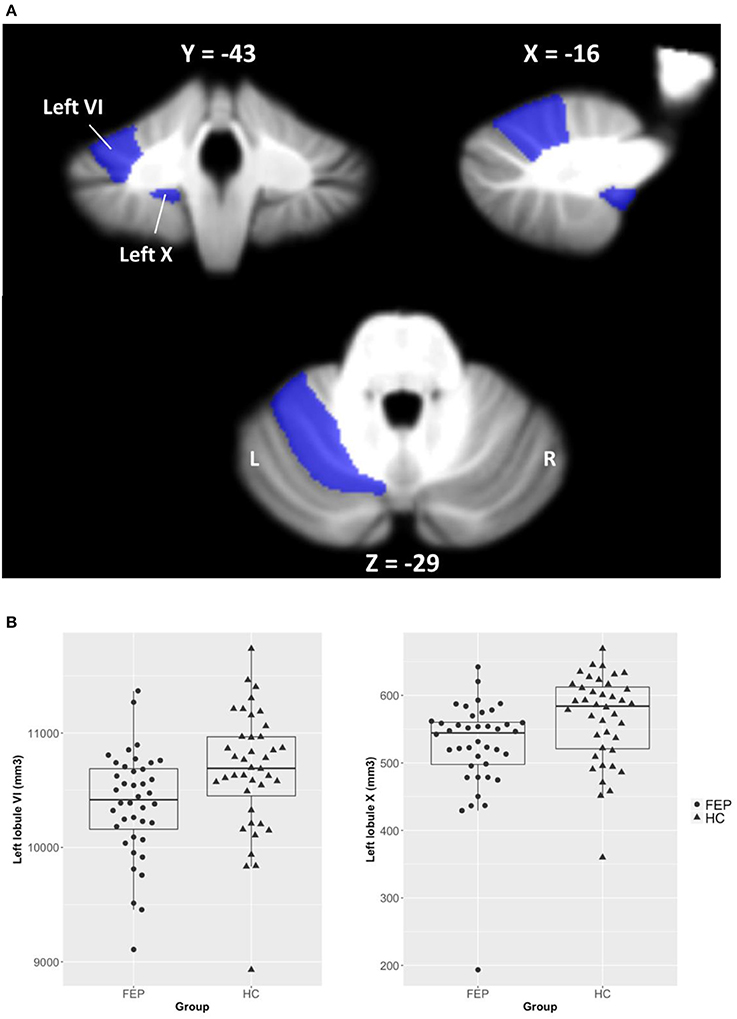
Figure 1. Volumetric differences of cerebellar lobules between FEP patients and HCs. (A) Regions of interest (ROIs) were selected from 28 cerebellar lobules using the SUIT cerebellum atlas, and ROIs of left lobules VI and X that significantly differed between groups are shown in the figure. (B) Cerebellar volumes of left lobules VI and X are smaller in FEP patients compared to HCs.
Correlation and Regression Analyses
Symptom severity was not associated with left lobe VI or X volume in patients. Smaller left lobule VI volume was associated with lower categories completed scores on the WCST in patients (r = 0.38, p < 0.05). Smaller left lobule X volume showed associations with delayed RT on the TMT Part B (r = −0.51, p < 0.01) and less categories completed on the WCST (r = 0.39, p < 0.05) within FEP patients. All the correlations observed in FEP patients were not found in HCs. Nonetheless, smaller left lobule VI volume was associated with delayed RT on the TMT Part A in HCs (r = −0.40, p < 0.05). Additional results of correlations between volumes of cerebellar regions (that produced non-significant between-group differences) and neurocognitive functions are presented in Tables S1, S2.
The results of regression analyses with HCs serving as a reference group are as follow. We found effects of group (Beta = −6.26, p < 0.01) and group-by-volume interaction of left lobule VI (Beta = 6.02, p < 0.05) on categories completed scores on the WCST, in which the interaction result indicated that FEP patients with larger left lobule VI volume had less impaired function in the WCST categories completed. The categories completed scores were also significantly predicted by group (Beta = −2.05, p < 0.05) and group-by-volume interaction of left lobule X (Beta = 1.84, p < 0.05) effects. In addition, group (Beta = 3.01, p < 0.001) and group-by-volume interaction of left lobule X (Beta = −2.61, p < 0.001) effects were variables that significantly predicted TMT Part B RT. The results of interaction terms of left lobule X indicated that FEP patients with larger volume of the region had less impaired functions in the WCST categories completed and TMT Part B. Full results of group-by-volume interaction effects of cerebellar regions (that produced non-significant between-group differences) on neurocognitive functions are presented in Table S3.
Within the patient group, volumes of left lobule VI and X were not significantly correlated with medication (chlorpromazine equivalent dose) (ρ = −0.28, p = 0.086; ρ = 0.03, p = 0.852), duration of illness (r = 0.04, p = 0.835; r = −0.22, p = 0.19), or IQ (r = −0.21, p = 0.214; r = 0.26, p = 0.115). However, age and duration of antipsychotic treatment had a significant negative correlation with left lobule VI (r = −0.36, p < 0.05; r = −0.34, p < 0.05), but not with left lobule X (r = −0.03, p = 0.876; r = −0.27, p = 0.103) volume.
Discussion
The present study examined the volume of each cerebellar lobule using SUIT in FEP patients and HCs. Our findings confirmed cerebellar volumetric abnormalities in FEP patients. FEP patients had specific volume deficits in the left lobule VI of the posterior superior lobe and left lobule X of the flocculonodular lobe. Furthermore, we observed that smaller volumes in these cerebellar regions were significantly associated with non-verbal executive functions, measured by both the TMT and WCST. Thus, this study provides evidence for altered cerebellar morphology and its associations with neurocognitive deficits in the early phase of schizophrenia.
We found smaller cerebellar GM volume in left lobule VI of patients, which was consistent with previous findings in both FEP patients (20) and chronic schizophrenia (3). Our finding of smaller left lobule VI volume together with executive dysfunction in FEP is important because it is line with previous functional neuroimaging studies showing an association between left lobule VI and executive function (63). Furthermore, recent functional connectivity studies have indicated that lobule VI is part of the ventral attention network (VAN) implicated in stimulus-driven attentional control (1, 64, 65). The association between smaller left lobule VI volume and neurological soft signs (NSS), which are subtle neurological abnormalities associated with cognitive impairments (66), in schizophrenia also supports the role of left lobule VI in neurocognitive deficits in the patients (22, 67, 68). We suggest that smaller left lobule VI volume could lead to executive dysfunction due to a disruption of VAN and contribute to the generation of NSS in schizophrenia.
We observed smaller left lobule X volume, which was consistent with previous cerebellar structural studies with FEP (20) as well as with chronic schizophrenia patients (3). Lobule X (i.e., flocculonodular lobe) is phylogenetically the oldest portion of the cerebellum and coordinates balance and movement via the vestibular system (69–71). Contrary to the traditional concept of the vestibular system, recent studies have shown evidence that vestibular dysfunction is related to impairments in executive functions, such as attentional set-shifting and cognitive inhibition (72–74). For example, Bigelow et al. showed an association between vestibular function and performance on TMT Part B, which assesses attentional set-shifting (73). Performance on the Stroop Task, measuring cognitive flexibility and cognitive inhibition (75), was also associated with vestibular dysfunction (74). Furthermore, at the whole-brain level, lobule X is part of the default mode network (DMN) involved in attention and cognitive shifting (1, 3, 64, 76, 77). Consistent with this, Kansal and colleagues reported an involvement of lobule X in attention and cognitive shifting, in cerebellar disorders (10). Our results of the associations between smaller lobule X volume and non-verbal executive dysfunctions in FEP patients are consistent with the studies mentioned above.
As reviewed in the introduction, and by others (78), inconsistent results have been reported in terms of cerebellar regional alterations in schizophrenia. Nonetheless, when considering only studies that employed cerebellar specific methods, smaller volume in the posterior superior lobe (VI - VIIB) and/or flocculonodular lobe (X) have consistently emerged (19–22). Obviously, clinical heterogeneity could remain a major factor in determining cerebellar structural abnormalities in schizophrenia (25). Nonetheless, we emphasize the importance of avoiding poor alignment of cerebellar structures by employing a cerebellar specific atlas such as SUIT (37), to minimize variance associated with methodology.
Cerebellar structural alterations have been reported mainly from chronic schizophrenia patients (3). However, we suggest that these cerebellar abnormalities are not a result of the adverse effects from a chronic deteriorating course of the illness or antipsychotic medications in patients. Instead, these abnormalities might be present at the early phase of the illness. In the present study, we showed that FEP patients also had cerebellar structural abnormalities, and that these cerebellar changes were not associated with antipsychotic medication effects or illness duration. This was consistent with previous studies that examined FEP and individuals at high risk for psychosis (43, 44). Therefore, we suggest that cerebellar structural alterations are more directly associated with the illness process itself rather than illness chronicity or antipsychotic medication effects in schizophrenia. A longitudinal study should be followed to investigate whether the cerebellar abnormalities are present from very young age in individuals who have a genetic or clinical risk for psychosis.
Our study has some limitations. First, it would be preferable to employ drug-naïve patients to study cerebellar volumes because the cerebellum could be affected by antipsychotic medication (79, 80). Nonetheless, the effect of antipsychotic medication might not be pronounced in our study, because our significant findings remained the same after controlling for medication effects. Second, in each of our participant group, approximately half were males. It is well known that cerebellar structural abnormalities are more pronounced in male than female patients with schizophrenia (26, 60). Hence, it is possible that cerebellar volume deficits in FEP in our study could have been diluted by inclusion of female participants. Further studies with a large sample could investigate cerebellar structural alterations in males and females separately. Finally, we did not strictly control for alcohol consumption in our study. Nonetheless, participants who had a diagnosis of alcohol use disorder were not included in our sample. Given that alcohol abuse is associated with cerebellar atrophy (81), future studies might want to investigate the effect of alcohol on cerebellar volumes even at sub-clinical level.
In conclusion, we provide evidence for smaller volumes in left cerebellar hemispheric lobules VI and X and their association with executive dysfunction in FEP patients. As these regional alterations were also reported in chronic schizophrenia patients using a cerebellar specific template, we conclude that these cerebellar structural abnormalities are related to illness itself, and not to illness progression and/or medication effects in schizophrenia. Whether these cerebellar alterations are associated with a phenotypic marker for emergence of symptoms (e.g., attenuated psychotic symptoms in high-risk individuals) or they are associated with a genetic predisposition to psychosis would need to be determined in future studies.
Author Contributions
TK, K-HL, TL, and JK contributed in drafting and revising the manuscript. JL and JK contributed in collecting the clinical data. TK and KC contributed in acquisition and analysis of MRI data. HO performed neurocognitive functioning tests. All the authors contributed in revising the manuscript.
Conflict of Interest Statement
The authors declare that the research was conducted in the absence of any commercial or financial relationships that could be construed as a potential conflict of interest.
Acknowledgments
The Brain Research Program through the National Research Foundation of Korea (NRF) funded by the Ministry of Science, ICT and Future Planning (Grant no. 2017M3C7A1029610) supported this research.
Supplementary Material
The Supplementary Material for this article can be found online at: https://www.frontiersin.org/articles/10.3389/fpsyt.2018.00286/full#supplementary-material
References
1. Buckner RL, Krienen FM, Castellanos A, Diaz JC, Yeo BTT. The organization of the human cerebellum estimated by intrinsic functional connectivity. J Neurophysiol (2011) 106:2322–45. doi: 10.1152/jn.00339.2011
2. Stoodley CJ. The cerebellum and cognition: evidence from functional imaging studies. Cerebellum (2012) 11:352–65. doi: 10.1007/s12311-011-0260-7
3. Moberget T, Doan NT, Alnaes D, Kaufmann T, Cordova-Palomera A, Lagerberg TV, et al. Cerebellar volume and cerebellocerebral structural covariance in schizophrenia: a multisite mega-analysis of 983 patients and 1349 healthy controls. Mol Psychiatry (2017) doi: 10.1038/mp.2017.106. [Epub ahead of print].
4. Szeszko PR, Gunning-Dixon F, Goldman RS, Bates J, Ashtari M, Snyder PJ, et al. Lack of normal association between cerebellar volume and neuropsychological functions in first-episode schizophrenia. Am J Psychiatry (2003) 160:1884–87. doi: 10.1176/appi.ajp.160.10.1884
5. Stoodley CJ, Valera EM, Schmahmann JD. Functional topography of the cerebellum for motor and cognitive tasks: an fMRI study. Neuroimage (2012) 59:1560–70. doi: 10.1016/j.neuroimage.2011.08.065
6. Baier B, Muller NG, Dieterich M. What part of the cerebellum contributes to a visuospatial working memory task? Ann Neurol. (2014) 76:754–7. doi: 10.1002/ana.24272.
7. Yoon YB, Shin WG, Lee TY, Hur JW, Cho KIK, Sohn WS, et al. Brain structural networks associated with intelligence and visuomotor ability. Sci Rep. (2017) 7:2177. doi: 10.1038/s41598-017-02304-z
8. Schmahmann JD, Caplan D. Cognition, emotion and the cerebellum. Brain (2006) 129:290–292. doi: 10.1093/brain/awh729
9. Stoodley CJ, Schmahmann JD. Evidence for topographic organization in the cerebellum of motor control versus cognitive and affective processing. Cortex (2010) 46:831–44. doi: 10.1016/j.cortex.2009.11.008
10. Kansal K, Yang Z, Fishman AM, Sair HI, Ying SH, Jedynak BM, et al. Structural cerebellar correlates of cognitive and motor dysfunctions in cerebellar degeneration. Brain (2017) 140:707–20. doi: 10.1093/brain/aww327
11. Green MF, Kern RS, Braff DL, Mintz J. Neurocognitive deficits and functional outcome in schizophrenia: are we measuring the “right stuff”? Schizophr Bull. (2000) 26:119–36. doi: 10.1093/oxfordjournals.schbul.a033430
12. Gaser C, Volz HP, Kiebel S, Riehemann S, Sauer H. Detecting structural changes in whole brain based on nonlinear deformations-application to schizophrenia research. Neuroimage (1999) 10:107–13. doi: 10.1006/nimg.1999.0458
13. Wassink TH,reasen NC, Nopoulos P, Flaum M. Cerebellar morphology as a predictor of symptom and psychosocial outcome in schizophrenia. Biol Psychiatry (1999) 45:41–48.
14. Bottmer C, Bachmann S, Pantel J, Essig M, Amann M, Schad LR, et al. Reduced cerebellar volume and neurological soft signs in first-episode schizophrenia. Psychiatry Res. (2005) 140:239–50. doi: 10.1016/j.pscychresns.2005.02.011
15. Cahn W, Hulshoff Pol HE, Lems EB, van Haren NE, Schnack HG, van der Linden JA, et al. Brain volume changes in first-episode schizophrenia: a 1-year follow-up study. Arch Gen Psychiatry (2002) 59:1002–10. doi: 10.1001/archpsyc.59.11.1002
16. Hulshoff Pol HE, Schnack HG, Bertens MG, van Haren NE, van der Tweel I, Staal WG, et al. Volume changes in gray matter in patients with schizophrenia. Am J Psychiatry (2002) 159:244–50. doi: 10.1176/appi.ajp.159.2.244
17. McDonald C, Grech A, Toulopoulou T, Schulze K, Chapple B, Sham P, et al. Brain volumes in familial and non-familial schizophrenic probands and their unaffected relatives. Am J Med Genet. (2002) 114:616–25. doi: 10.1002/ajmg.10604
18. Wilke M, Kaufmann C, Grabner A, Putz B, Wetter TC, Auer DP. Gray matter-changes and correlates of disease severity in schizophrenia: a statistical parametric mapping study. Neuroimage (2001) 13:814–24. doi: 10.1006/nimg.2001.0751
19. Okugawa G, Sedvall G, Nordstrom M,reasen N, Pierson R, Magnotta V, et al. Selective reduction of the posterior superior vermis in men with chronic schizophrenia. Schizophr Res. (2002) 55:61–7. doi: 10.1016/S0920-9964(01)00248-1
20. Rasser PE, Schall U, Peck G, Cohen M, Johnston P, Khoo K, et al. Cerebellar grey matter deficits in first-episode schizophrenia mapped using cortical pattern matching. Neuroimage (2010) 53:1175–80. doi: 10.1016/j.neuroimage.2010.07.018
21. Kuhn S, Romanowski A, Schubert F, Gallinat J. Reduction of cerebellar grey matter in crus I and II in schizophrenia. Brain Struct Funct. (2012) 217:523–9. doi: 10.1007/s00429-011-0365-2
22. Hirjak D, Wolf RC, Kubera KM, Stieltjes B, Maier-Hein KH, Thomann PA. Neurological soft signs in recent-onset schizophrenia: focus on the cerebellum. Prog Neuropsychopharmacol Biol Psychiatry (2015) 60:18–25. doi: 10.1016/j.pnpbp.2015.01.011
23. Jacobsen LK, Giedd JN, Berquin PC, Krain AL, Hamburger SD, Kumra S, et al. Quantitative morphology of the cerebellum and fourth ventricle in childhood-onset schizophrenia. Am J Psychiatry (1997) 154:1663–9. doi: 10.1176/ajp.154.12.1663
24. Nopoulos PC, Ceilley JW, Gailis EA, Andreasen NC. An MRI study of cerebellar vermis morphology in patients with schizophrenia: evidence in support of the cognitive dysmetria concept. Biol Psychiatry (1999) 46:703–11.
25. Loeber RT, Cintron CM, Yurgelun-Todd DA. Morphometry of individual cerebellar lobules in schizophrenia. Am J Psychiatry (2001) 158:952–4. doi: 10.1176/appi.ajp.158.6.952
26. Joyal CC, Pennanen C, Tiihonen E, Laakso MP, Tiihonen J, Aronen HJ. MRI volumetry of the vermis and the cerebellar hemispheres in men with schizophrenia. Psychiatry Res. (2004) 131:115–24. doi: 10.1016/j.pscychresns.2003.09.003
27. Lee KH, Farrow TF, Parks RW, Newton LD, Mir NU, Egleston PN, et al. Increased cerebellar vermis white-matter volume in men with schizophrenia. J Psychiatr Res. (2007) 41:645–51. doi: 10.1016/j.jpsychires.2006.03.001
28. Yuksel C, McCarthy J, Shinn A, Pfaff DL, Baker JT, Heckers S, et al. Gray matter volume in schizophrenia and bipolar disorder with psychotic features. Schizophr Res. (2012) 138:177–82. doi: 10.1016/j.schres.2012.03.003
29. Sheng J, Zhu Y, Lu Z, Liu N, Huang N, Zhang Z, et al. Altered volume and lateralization of language-related regions in first-episode schizophrenia. Schizophr Res. (2013) 148:168–74. doi: 10.1016/j.schres.2013.05.021
30. Toulopoulou T, Grech A, Morris RG, Schulze K, McDonald C, Chapple B, et al. The relationship between volumetric brain changes and cognitive function: a family study on schizophrenia. Biol Psychiatry (2004) 56:447–53. doi: 10.1016/j.biopsych.2004.06.026
31. Segarra N, Bernardo M, Valdes M, Caldu X, Falcon C, Rami L, et al. Cerebellar deficits in schizophrenia are associated with executive dysfunction. Neuroreport (2008) 19:1513–17. doi: 10.1097/WNR.0b013e3283108bd8
32. Schretlen DJ, Vannorsdall TD, Winicki JM, Mushtaq Y, Hikida T, Sawa A, et al. Neuroanatomic and cognitive abnormalities related to herpes simplex virus type 1 in schizophrenia. Schizophr Res. (2010) 118:224–31. doi: 10.1016/j.schres.2010.01.008
33. Levitt JJ, McCarley RW, Nestor PG, Petrescu C, Donnino R, Hirayasu Y, et al. Quantitative volumetric MRI study of the cerebellum and vermis in schizophrenia: clinical and cognitive correlates. Am J Psychiatry (1999) 156:1105–7. doi: 10.1176/ajp.156.7.1105
34. Evans AC, Cobia DL, Mills S, Brown ED, Kelly RL, Peters TM. 3D statistical neuroanatomical models from 305 MRI volumes. In: Proceedings IEEE Nuclear Science Symposium and Medical Imaging Conference. (1993) San Francisco, CA. p. 1813–7.
35. Ashburner J, Friston KJ. Voxel-based morphometry—the methods. Neuroimage (2000) 11:805–21. doi: 10.1006/nimg.2000.0582
36. Fischl B, Sereno MI, Tootell RBH, and Dale AM. High-resolution intersubject averaging and a coordinate system for the cortical surface. Hum Brain Mapp. (1999) 8:272–84. doi: 10.1002/(SICI)1097-0193(1999)8:4<272::AID-HBM10>3.0.CO;2-4
37. Diedrichsen J. A spatially unbiased atlas template of the human cerebellum. Neuroimage (2006) 33:127–38. doi: 10.1016/j.neuroimage.2006.05.056
38. Diedrichsen J, Balsters JH, Flavell J, Cussans E, Ramnani N. A probabilistic MR atlas of the human cerebellum. Neuroimage (2009) 46:39–46. doi: 10.1016/j.neuroimage.2009.01.045
39. Diedrichsen J, Maderwald S, Kuper M, Thurling M, Rabe K, Gizewski ER, et al. Imaging the deep cerebellar nuclei: a probabilistic atlas and normalization procedure. Neuroimage (2011) 54:1786–94. doi: 10.1016/j.neuroimage.2010.10.035
40. Steen RG, Mull C, McClure R, Hamer RM, Lieberman JA. Brain volume in first-episode schizophrenia: systematic review and meta-analysis of magnetic resonance imaging studies. Br J Psychiatry (2006) 188:510–8. doi: 10.1192/bjp.188.6.510.
41. Chan RC, Di X, McAlonan GM, Gong QY. Brain anatomical abnormalities in high-risk individuals, first-episode, and chronic schizophrenia: an activation likelihood estimation meta-analysis of illness progression. Schizophr Bull. (2011) 37:177–88. doi: 10.1093/schbul/sbp073
42. Torres US, Duran FL, Schaufelberger MS, Crippa JA, Louza MR, Sallet PC, et al. Patterns of regional gray matter loss at different stages of schizophrenia: a multisite, cross-sectional VBM study in first-episode and chronic illness. Neuroimage Clin. (2016) 12:1–15. doi: 10.1016/j.nicl.2016.06.002
43. Job DE, Whalley HC, Johnstone EC, Lawrie SM. Grey matter changes over time in high risk subjects developing schizophrenia. Neuroimage (2005) 25:1023–30. doi: 10.1016/j.neuroimage.2005.01.006
44. Borgwardt SJ, Riecher-Rossler A, Dazzan P, Chitnis X, Aston J, Drewe M, et al. Regional gray matter volume abnormalities in the at risk mental state. Biol Psychiatry (2007) 61:1148–56. doi: 10.1016/j.biopsych.2006.08.009
45. Kwon JS, Byun MS, Lee TY, An SK. Early intervention in psychosis: insights from Korea. Asian J Psychiatry (2012) 5:98–105. doi: 10.1016/j.ajp.2012.02.007
46. First MB, Spitzer RL, Gibbon M, Williams JBW. (2002b). Structured Clinical Interview for DSM-IV-TR Axis I Disorders, Research Version, Patient Edition, SCID-I/P. New York, NY: Biometrics Research, New York State Psychiatric Institute.
47. Kay SR, Fiszbein A, Opler LA. The positive and negative syndrome scale (PANSS) for schizophrenia. Schizophr Bull. (1987) 13:261–76.
48. Endicott J, Spitzer RL, Fleiss JL, Cohen J. The global assessment scale: a procedure for measuring overall severity of psychiatric disturbance. Arch Gen Psychiatry (1976) 33:766–71.
49. First MB, Spitzer RL, Gibbon M, Williams JBW. (2002a). Structured Clinical Interview for DSM-IV-TR Axis I Disorders, Research Version, Non-Patient Edition, SCID-I/NP. New York, NY: Biometrics Research, New York State Psychiatric Institute.
50. Wechsler D. (1997). WAIS-III, Wechsler Adult Intelligence Scale: Administration and Scoring Manual. San Antonio, TX: Psychological Corporation.
51. Tombaugh TN. Trail making test A and B: normative data stratified by age and education. Arch Clin Neuropsychol. (2004) 19:203–14. doi: 10.1016/S0887-6177(03)00039-8.
52. Meyers JE, Meyers KR. (1995). Rey Complex Figure Test and Recognition Trial Professional Manual. Odessa, FL: Psychological Assessment Resources.
53. Benton AL. Development of a multilingual aphasia battery: progress and problems. J Neurol Sci. (1969) 9:39–48.
54. Kang Y, Chin JH, Na DL, Lee J, Park JS. A normative study of the Korean version of controlled oral word association test (COWAT) in the elderly. Kor J Clin Psychol. (2000) 19:385–92.
55. Schretlen DJ. (2010). Modified Wisconsin Card Sorting Test: M-WCST; Professional Manual. Lutz, FL: PAR.
56. Ashburner J, Friston KJ. Unified segmentation. Neuroimage (2005) 26:839–51. doi: 10.1016/j.neuroimage.2005.02.018
57. Beg MF, Miller MI, Trouve A, Younes L. Computing large deformation metric mappings via geodesic flows of diffeomorphisms. Int J Comput Vis. (2005) 61:139–57. doi: 10.1023/B:VISI.0000043755.93987.aa
58. Ashburner J. A fast diffeomorphic image registration algorithm. Neuroimage (2007) 38:95–13. doi: 10.1016/j.neuroimage.2007.07.007
59. Fischl B, Salat DH, Busa E, Albert M, Dieterich M, Haselgrove C, et al. Whole brain segmentation: automated labeling of neuroanatomical structures in the human brain. Neuron (2002) 33:341–55. doi: 10.1016/S0896-6273(02)00569-X
60. Rossi A, Stratta P, Mancini F, de Cataldo S, Casacchia M. Cerebellar vermal size in schizophrenia: a male effect. Biol Psychiatry (1993) 33:354–7.
61. James S. Approximate multinormal probabilities applied to correlated multiple endpoints in clinical trials. Stat Med. (1991) 10:1123–35.
62. Xie C. Weighted multiple testing correction for correlated tests. Stat Med. (2012) 31:341–2. doi: 10.1002/sim.4434
63. Stoodley CJ, Schmahmann JD. Functional topography in the human cerebellum: a meta-analysis of neuroimaging studies. Neuroimage (2009) 44:489–501. doi: 10.1016/j.neuroimage.2008.08.039
64. Shinn AK, Baker JT, Lewandowski KE, Ongur D, Cohen BM. Aberrant cerebellar connectivity in motor and association networks in schizophrenia. Front Hum Neurosci. (2015) 9:134. doi: 10.3389/fnhum.2015.00134
65. Dong D, Wang Y, Chang X, Luo C, Yao D. Dysfunction of large-scale brain networks in schizophrenia: a meta-analysis of resting-state functional connectivity. Schizophr Bull. (2017) 44:168–81. doi: 10.1093/schbul/sbx034.
66. Bombin I, Arango C, Buchanan RW. Significance and meaning of neurological signs in schizophrenia: two decades later. Schizophr Bull. (2005) 31:962–77. doi: 10.1093/schbul/sbi028
67. Mohr F, Hubmann W, Cohen R, Bender W, Haslacher C, Honicke S, et al. Neurological soft signs in schizophrenia: assessment and correlates. Eur Arch Psychiatry Clin Neurosci. (1996) 246:240–8.
68. Smith RC, Kadewari RP. Neurological soft signs and response to risperidone in chronic schizophrenia. Biol Psychiatry (1996) 40:1056–9. doi: 10.1016/S0006-3223(96)00251-X.
70. Rubenstein JLR, Rakic P. (2013). Comprehensive Developmental Neuroscience. Amsterdam: Academic Press.
71. Meng H, Blazquez PM, Dickman JD, Angelaki DE. Diversity of vestibular nuclei neurons targeted by cerebellar nodulus inhibition. J Physiol. (2014) 592:171–88. doi: 10.1113/jphysiol.2013.259614.
72. Bigelow RT, Agrawal Y. Vestibular involvement in cognition: visuospatial ability, attention, executive function, and memory. J Vestib Res. (2015) 25:73–89. doi: 10.3233/VES-150544.
73. Bigelow RT, Semenov YR, Trevino C, Ferrucci L, Resnick SM, Simonsick EM, et al. Association between visuospatial ability and vestibular function in the baltimore longitudinal study of aging. J Am Geriatr Soc. (2015) 63:1837–44. doi: 10.1111/jgs.13609
74. Popp P, Wulff M, Finke K, Ruhl M, Brandt T, and Dieterich M. Cognitive deficits in patients with a chronic vestibular failure. J Neurol. (2017) 264:554–63. doi: 10.1007/s00415-016-8386-7
75. Scarpina F, Tagini S. The Stroop Color and Word Test. Front Psychol. (2017) 8:557. doi: 10.3389/fpsyg.2017.00557
76. Crittenden BM, Mitchell DJ, Duncan J. Recruitment of the default mode network during a demanding act of executive control. Elife (2015) 4:e06481. doi: 10.7554/eLife.06481
77. Arsenault JT, Caspari N, Vandenberghe R, Vanduffel W. Attention shifts recruit the monkey default mode network. J Neurosci. (2018) 38:1202–17. doi: 10.1523/JNEUROSCI.1111-17.2017
78. Lawyer G, Nesvag R, Varnas K, Okugawa G, Agartz I. Grey and white matter proportional relationships in the cerebellar vermis altered in schizophrenia. Cerebellum (2009) 8:52–60. doi: 10.1007/s12311-008-0071-7
79. Premkumar P, Fannon D, Kuipers E, Cooke MA, Simmons A, Kumari V. Association between a longer duration of illness, age and lower frontal lobe grey matter volume in schizophrenia. Behav Brain Res. (2008) 193:132–9. doi: 10.1016/j.bbr.2008.05.012
80. Abbott CC, Jaramillo A, Wilcox CE, Hamilton DA. Antipsychotic drug effects in schizophrenia: a review of longitudinal fMRI investigations and neural interpretations. Curr Med Chem. (2013) 20:428–37. doi: 10.2174/0929867311320030014
Keywords: schizophrenia, FEP, cerebellum, neurocognition, executive function, neuroimaging, SUIT
Citation: Kim T, Lee K-H, Oh H, Lee TY, Cho KIK, Lee J and Kwon JS (2018) Cerebellar Structural Abnormalities Associated With Cognitive Function in Patients With First-Episode Psychosis. Front. Psychiatry 9:286. doi: 10.3389/fpsyt.2018.00286
Received: 26 March 2018; Accepted: 12 June 2018;
Published: 03 July 2018.
Edited by:
Christian Huber, Universitäre Psychiatrische Kliniken Basel, SwitzerlandReviewed by:
Vincent Koppelmans, University of Utah, United StatesKrystal Lynn Parker, University of Iowa, United States
Uner Tan, Çukurova University, Turkey
Copyright © 2018 Kim, Lee, Oh, Lee, Cho, Lee and Kwon. This is an open-access article distributed under the terms of the Creative Commons Attribution License (CC BY). The use, distribution or reproduction in other forums is permitted, provided the original author(s) and the copyright owner(s) are credited and that the original publication in this journal is cited, in accordance with accepted academic practice. No use, distribution or reproduction is permitted which does not comply with these terms.
*Correspondence: Jun Soo Kwon, a3dvbmpzQHNudS5hYy5rcg==