- 1Department of Plant Pathology, Washington State University, Pullman, WA, United States
- 2Wheat Health, Genetics and Quality Research Unit, USDA-ARS, Pullman, WA, United States
The purinoceptor P2K1/DORN1 recognizes extracellular ATP, a damage-associated molecular pattern (DAMP) released upon cellular disruption by wounding and necrosis, which in turn, boost plant innate immunity. P2K1 is known to confer plant resistance to foliar biotrophic, hemi-biotrophic, and necrotrophic pathogens. However, until now, no information was available on its function in defense against root pathogens. In this report, we describe the contribution of P2K1 to resistance in Arabidopsis against Rhizoctonia solani, a broad host range, necrotrophic soilborne fungal pathogen. In pot assays, the Arabidopsis P2K1 overexpression line OxP2K1 showed longer root length and a greater rosette surface area than wild type in the presence of the pathogen. In contrast, the knockout mutant dorn1-3 and the double mutant rbohd/f, defective in two subunits of the respiratory burst complex NADPH oxidase, exhibited significant reductions in shoot and root lengths and rosette surface area compared to wild type when the pathogen was present. Expression of PR1, PDF1.2, and JAZ5 in the roots was reduced in dorn1-3 and rbohd/f and elevated in OxP2K1 relative to wild type, indicating that the salicylate and jasmonate defense signaling pathways functioned in resistance. These results indicated that a DAMP-mediated defense system confers basal resistance against an important root necrotrophic fungal pathogen.
Introduction
The necrotrophic soilborne pathogen Rhizoctonia solani infects more than 250 plant species, including corn, potato, soybean, pulses, brassicas, and small-grain cereals. Rhizoctonia solani primarily infects the below-ground organs of the plant (Weinhold and Sinclair, 1996; Keijer et al., 1997), resulting in damping-off, root rot and bare patch of cereals, brown patch of turfgrass, black scurf of potato, and sheath blight of rice (Sneh et al., 1991; DeShields et al., 2018). For example, R. solani anastomosis group 8 (AG-8), causes chronic and acute yield losses from 10-30% in dryland cereal production systems of the Pacific Northwest, USA and up to 100% in parts of the world, amounting to billions of dollars of annual losses to agriculture worldwide (Okubara et al., 2014; Okubara et al., 2019). It is a broad host range pathogen, causing disease on a variety of crop plants, including canola and other brassicas. Rhizoctonia solani AG2-1 is the premier causal agent of Rhizoctonia damping-off of brassicas and pulses (Paulitz et al., 2006; Sturrock et al., 2015). It is frequently associated with wheat roots but generally is less pathogenic relative to R. solani AG-8 on both wheat and brassicas (Sturrock et al., 2015). Management of Rhizoctonia root diseases has been under development for decades. Genetic resistance to R. solani AG-8 has been reported for wheat (Okubara et al., 2009; Mahoney et al., 2016; Mahoney et al., 2017) but is not readily deployable in wheat breeding programs due to the multigenic nature of resistance and absence of molecular markers (Okubara et al., 2019). However, a broad-spectrum resistance gene from rice that confers R. solani resistance in Arabidopsis has been recently described (Maeda et al., 2019) and would be more readily deployable than multigenic resistance. Foliar plant defense activators such as probenazole are not effective for crop protection from R. solani infection (Kouzai et al., 2018). Crop rotation can have limited utility due to the exceptionally broad host range of R. solani. Biological control has not been practiced in large-scale production systems, but one study demonstrated that the use of hypovirulent Rhizoctonia isolates was effective in protecting against virulent isolates (Sharon et al., 2011). Current management practices include direct seed or minimum tillage, applications of nitrogen at the time of planting, and reduction of weeds and volunteers using herbicides (Mahoney et al., 2016).
Molecular genetic approaches have been successful in boosting plant defense to R. solani infections. For example, rice plants with increased jasmonate (JA)-mediated defense responses and ethylene (ET) production showed enhanced resistance to R. solani (Peng et al., 2012; Helliwell et al., 2013). Using Arabidopsis, researchers have demonstrated that the heterologous expression of a germin-like protein from sugar beet enhanced R. solani resistance (Knecht et al., 2010). Interestingly, NADPH oxidase components, such as RBOHD and RBOHF, were also suggested to play an important role in plant resistance to R. solani (Foley et al., 2013). Introduced defense genes provided about 50% protection against Rhizoctonia and Pythium in wheat (Okubara et al., 2014). Although the genes described above have provided plant resistance to R. solani, more genetic information is needed to effectively control this difficult disease.
Accumulating evidence suggests that extracellular ATP is released, triggering plant responses to various biotic and abiotic stresses (Dark et al., 2011; Ramachandran et al., 2019). Extracellular ATP is a damage-associated molecular pattern or DAMP (Tanaka et al., 2014). In response to cellular disruption by wounding, necrotizing invasion or predation, ATP is released into the apoplast and perceived by the plant cell surface purinoceptor P2K1 (also known as DORN1) (Choi et al., 2014). Upon ATP binding to the extracellular lectin domain of the purinoceptor (Nguyen et al., 2016), its intracellular kinase domain is activated (Chen et al., 2017), which subsequently results in the activation of a number of intracellular signaling pathways for the reprogramming of many plant defense-related genes (Jewell et al., 2019). Overexpression of P2K1 enhances plant resistance against various foliar pathogens, such as Phytophthora brassicae (biotrophic oomycete), Pseudomonas syringae (hemibiotrophic bacterium), and Botrytis cinerea (necrotrophic fungus) (Bouwmeester et al., 2011; Balagué et al., 2017; Chen et al., 2017; Tripathi et al., 2018), suggesting that extracellular ATP plays an important role in plant defense against a broad range of pathogens. However, there is no report on whether extracellular ATP enhances plant resistance to root necrotrophic pathogens such as R. solani.
We hypothesized that extracellular ATP acts as a DAMP to enhance root resistance to the necrotizing root pathogen R. solani. Here, we used the Arabidopsis-R. solani pathosystem to assess plant defense responses mediated by extracellular ATP signaling. The model plant Arabidopsis can be infected with two different R. solani anastomosis groups, AG2-1 and AG-8 (Foley et al., 2013). Using these fungal strains, we evaluated the susceptibility in Arabidopsis wild-type plants (WT), DAMP-related knockout mutants and plants overexpressing the P2K1 receptor. Our results demonstrated that P2K1 mediates a root defense response against both R. solani AG-8 and AG2-1. This is the first report of the role of extracellular ATP for resistance to the root pathogen R. solani.
Materials and Methods
Plant Materials
All Arabidopsis genotypes used in the studies were in the ecotype Col-0 background. The knockout mutant dorn1-3, the overexpression line OxP2K1, and rbohd/f (a double knockout mutant of two NADPH oxidase subunits) were previously reported (Torres et al., 2002; Choi et al., 2014). Seeds were surface sterilized and plated onto half-strength Murashige & Skoog (MS) medium containing 2.2 g/L MS salt with vitamins (Caisson Labs Inc., Smithfield, UT), 1% (w/v) sucrose, 1% (w/v) agar, and 0.05% (w/v) MES (pH 5.7) in a square petri dish. Seeds were kept in the dark at 4°C for 3 days to synchronize germination and transferred to a growth chamber (Conviron Inc., Winnipeg, Canada) at 22°C under a 16-h light/8-h dark cycle (100 µmol m-2 s-1 light intensity). The petri dishes were oriented vertically during plant growth.
Preparation and Enumeration of Rhizoctonia solani AG-8 Inoculum
Rhizoctonia solani AG-8 isolate C1 was cultured on potato dextrose agar (PDA) for up to 5 days (Okubara et al., 2009). Substrate for the inoculum was prepared by autoclaving feed-quality oats for 60 min per day on two consecutive days, with a 20- to 24-h period of cooling in between (Okubara et al., 2009). Approximately 15–20 agar cubes (~5 mm3) from the leading edge of the fungal colony were distributed among the cooled oats by gentle shaking. Inoculated oats were incubated in the dark for 3 weeks at 23°C. The oats were shaken gently every seven days for 2 weeks to redistribute the fungi. In the third week, the inoculum was air dried in a laminar hood on clean Kraft paper for 24–48 h. Dried inoculated oats were homogenized using a coffee grinder and passed through 1,000 µm and 250 µm sieves (Okubara et al., 2009). The ground inoculum was stored at -20°C for up to 3 weeks. Rhizoctonia colonization was quantified immediately before use from triplicate suspensions of 100 mg inoculum in 5 ml of water and 1:10 dilutions of each suspension. Two-hundred microliters of each suspension were plated on water agar containing 100 µg mL-1 of chloramphenicol. Colonies were counted for 3 successive days under a dissecting scope. Colony forming units (CFU) per gram of oat was the average of the cumulative colony counts for each dilution.
Comparison of Rhizoctonia solani AG-8 and AG2-1 Pathogenicity
Surface-sterilized seeds of Arabidopsis (WT of Col-0) were germinated for 11 days on 0.8% M9 agar and then transferred to 4” pots containing pasteurized Sunshine Potting Mix #4 moistened with Miracle Gro solution (0.9 g/L). Soil was infested immediately prior to sowing with 0 or 50 CFU g-1 soil of R. solani AG2-1 or AG-8 isolate C1. Plants were grown at 22°C in 16-h light/8-h dark in an environmental growth chamber, with Miracle Gro supplements. Seedling weight and root morphometric analysis (total root length) were obtained after 14 days of infection. Non-infested soil served as the control.
Rhizoctonia solani AG-8 Infection of Soil-Grown Plants
Eleven-day-old Arabidopsis seedlings were used for fungal infection in pots. Seedlings were transferred to a soil/sand mixture with a 1:1 (v:v) ratio containing R. solani AG-8 C1 inoculum in ground oats at a final population density of 150 CFU g-1 soil. No-pathogen controls were included for each genotype. Plants were grown for 2 weeks in a growth chamber at 22°C with 70% humidity under a 12-h light/12-h dark cycle (150 µmol m-2 s-1 light intensity), with 50 mL water per pot (1.50” × 3.38” × 2.27”) added on alternate days. At the time of harvest, water was added to the pot to loosen the soil and minimize root breakage. Eight replicates of the control and pathogen-challenged samples were evaluated in each experiment. The experiment was repeated three times. To evaluate the fungal growth in roots, total DNA was extracted and subjected to PCR-based quantification using primers for the AG-8 ITS region: AG-8_F (AGTTGGTTGTAGCTGGTCCATTAAT) and AG-8_R (AGTAGACAGAGGGGTCCAATAAATGA) (Budge et al., 2009). The data were normalized to an Arabidopsis reference gene, AtSAND (At2g28390).
Morphometric Analysis of Soil-Grown Plants
Rosette areas and total root length of all the plants were measured from digital images after 14 days of growth in infested soil. Rosette area was quantified in situ using ImageJ (https://imagej.nih.gov/ij/). The roots were washed to remove soil and debris. Individual root systems were stored at 4°C between wet paper towels in polythene bags for about 24 h. Roots were transferred to a glass tray (25 × 25 × 2 cm), and water was added to just submerge the roots. The roots were carefully spread to minimize tangles and crossovers. Digital images were acquired using the Epson Twain Pro scanner at 600 dpi resolution. Total root length (cm) was determined for each root system using WinRHIZO Regular software (ver. 2016b, Regent Instruments, Inc., Quebec, Canada).
Total RNA Extraction and Real-Time PCR
Total RNA in roots was extracted using a Quick-RNA Microprep kit (Zymo Research, Irvine, CA). Frozen tissues were powdered using ceramic beads for 60 s in a Mini-bead beater (Biospec, Bartlesville, OK). One microgram of total RNA was used to synthesize the first strand of cDNA using the iScript cDNA synthesis kit (Bio-Rad, Hercules, CA). Real-time PCR quantification of PR1, PDF1.2 and JAZ5 transcripts was performed using the SsoAdvanced SYBR Green Supermix kit on the CFX96 Touch Real-Time System (Bio-Rad, Hercules, CA). Thermal cycling conditions were composed of initial denaturation for 3 min at 95°C, followed by 40 cycles of denaturation for 10 s at 95°C and annealing and extension for 30 s at 60°C. The melting protocol was 65 to 95°C (increments 0.5°C/5 s) to ensure a unique PCR product was produced. AtUBQ10 (At4g05320) or AtSAND genes were used to normalize the expression data. The Cq values of three biological replicates were used to calculate the expression of genes using the 2^-(ΔΔCq) equation (Schmittgen and Livak, 2008).
Rhizoctonia solani AG2-1 Infection of Young Seedlings in the Presence of ATP
R. solani AG2-1 was grown on PDA for 2–3 days. A single agar plug (5-mm diameter) with actively growing fungus was placed in the middle of an MS plate amended with 100 µM ATP (Sigma-Aldrich, St. Louis, MO). Control plates did not contain exogenously added ATP. Ten to twelve 10-day-old Arabidopsis seedlings were placed around the fungal plug with the root tips facing the plug. After incubation for 2 days, the seedlings were washed three times with 3% (v/v) H2O2 and sterile water and then subjected to PCR-based quantification using primers for the AG2-1 ITS region. Primer sequences used are as follows: Rs2.1/8F (GTTGTAGCTGGCCCATTCATTTG) and Rs2.1/8R (GAGCAGGTGTGAAGCTGCAAAAG) (Okubara et al., 2008). The data were normalized to an Arabidopsis reference gene, AtSAND. The experiment was repeated at least three times.
Statistical Analysis
All experiments were performed independently at least three times or more. The results were shown as the mean ± SE. The significant differences among the means were analyzed using Student’s t-test or an ANOVA model and a post hoc analysis using a t-test (P-value < 0.05).
Results
Pathogenicity of R. solani AG-8 and AG2-1 on Arabidopsis
First, a pathogenicity comparison was carried out to determine which AG caused more disease on Arabidopsis and would be more likely to distinguish the responses of the mutants. Arabidopsis Col-0 plants showed differential susceptibility to R. solani AG2-1 compared to R. solani AG-8 in the pathogenicity assays (Figure 1). Reductions in seedling weight and total root length were less than twofold in the soil infested with 50 CFU g-1 to R. solani AG2-1 but approached fivefold for R. solani AG-8 relative to the non-infested control. The findings supported observations that the latter pathogen produced more severe disease symptoms than the former for Arabidopsis Col-0 plants. Therefore, subsequent experiments were focused on R. solani AG-8.
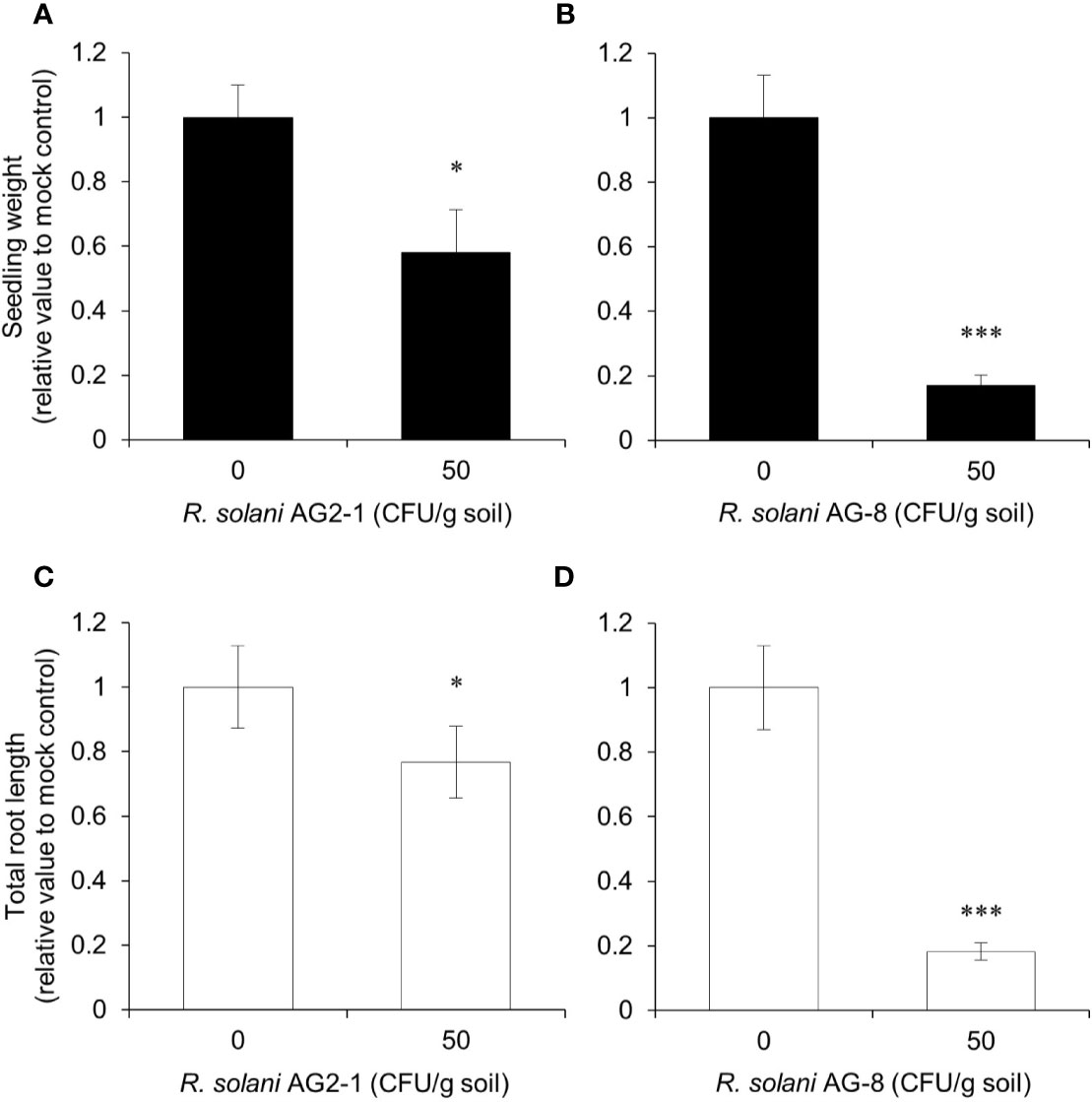
Figure 1 Pathogenicity assay for Rhizoctonia solani AG2-1 and AG-8 against Arabidopsis Col-0 WT. Eleven-day-old seedlings were planted in pasteurized soil infested with 0 and 50 CFU g-1 of R. solani AG2-1 (A, C) or AG-8 (B, D). At 14 days post-infection, total seedling weight (A, B) and total root length (C, D) were quantified. Means ± S.E. are the average of 8 to 16 plants. Data are presented as the relative value to mock control (0 CFU g-1 soil). Asterisks indicate statistically significant differences compared with the control: Student’s t test, *P < 0.05 and ***P < 0.001.
P2K1 Confers Plant Resistance Against R. solani
To measure the contribution of the purinoceptor P2K1 to plant resistance to the soilborne pathogen R. solani AG-8, we performed a pot-based fungal pathogenicity assay using four genotypes of Arabidopsis grown in soil infested with the pathogen. The NADPH oxidase double mutant rbohd/f was used as a positive control since it was reported to show complete loss of resistance to R. solani (Foley et al., 2013). After only one week of growth in soil containing 150 CFU g-1 of pathogen, severe stunting of aerial growth was observed. Reduction in rosette area was an indicator of underground-derived stress, usually due to root loss, in plants infected with soilborne pathogens (Mahoney et al., 2016; Julkowska et al., 2016). Figure 2A shows the rosette leaves of all four genotypes grown in noninfested and infested soils for 14 days. Both dorn1-3 and rbohd/f showed a very high reduction in total rosette area (Figure 2C and Supplementary Figure S1A). Based on a visual observation, dorn1-3 and rbohd/f also showed a yellow to tan chlorosis on their leaves (Figure 2A). In contrast, OxP2K1 showed the least reduction compared to wild type (WT) plants although the difference between them are not statistically significant (Figure 2C). Similar results were obtained with infested field soil locally collected from the Spillman Agronomy Farm, Pullman, WA (Supplementary Figures S2A, C).
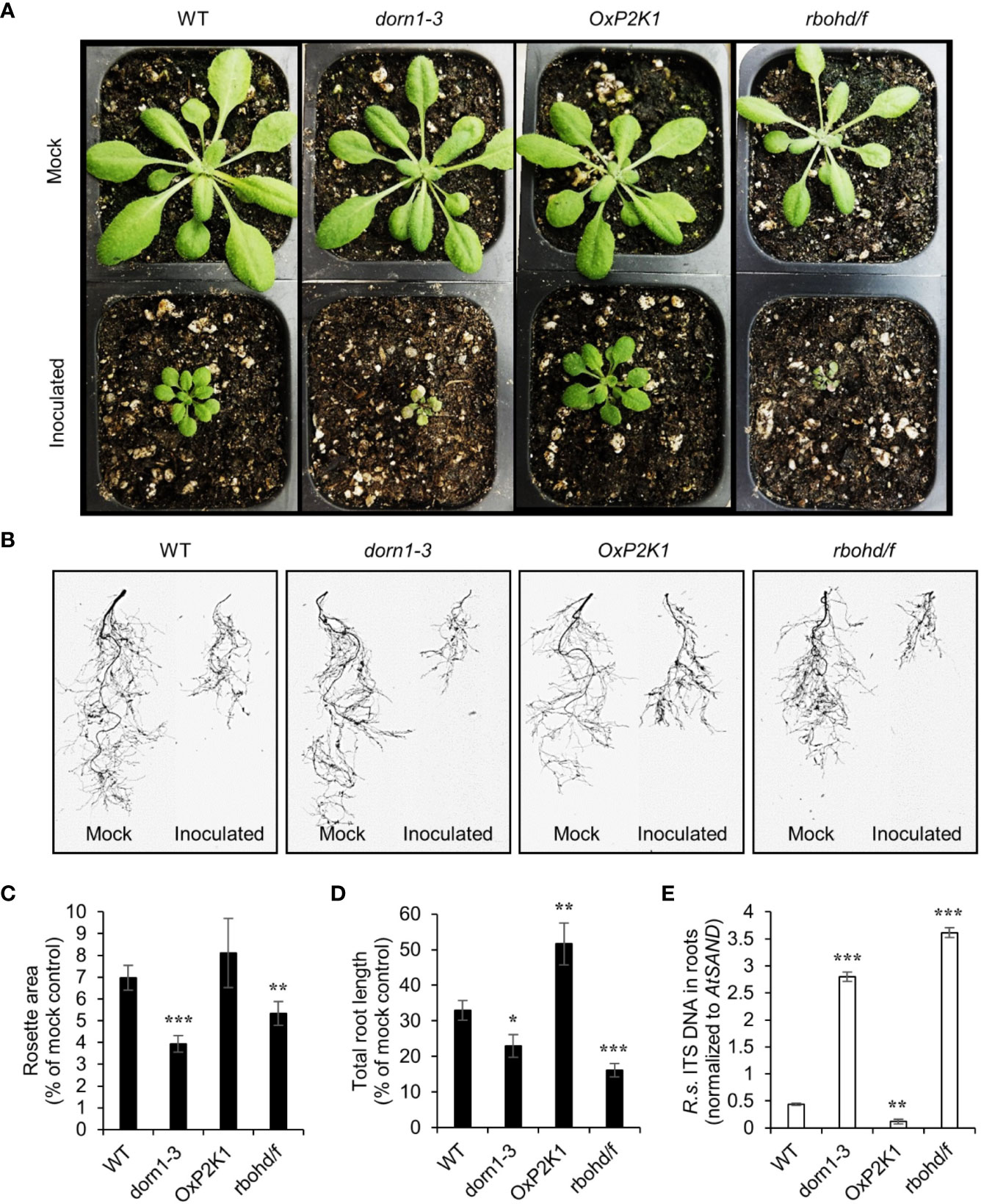
Figure 2 Effect of Rhizoctonia solani AG-8 infection on the aboveground rosette growth and the root growth of Arabidopsis. Eleven-day-old Arabidopsis seedlings were transferred to noninfested soil (mock) or soil infested with R. solani AG-8 C1 (150 CFU/g soil). Pictures of aboveground (A) and scan images of the root systems (B) were taken at 14 days of growth in the soil. The rosette areas of all four genotypes were measured as the smallest circle area enclosed by the convex hull of the rosette (C). The total root length was quantified using the WinRHIZO (D). Data are presented as the mean percentage ± S.E. (n = 8) relative to mock control. The level of infection in the roots quantified by real-time PCR based (E). Abundance is expressed as the ratios of the fungal-specific ITS region relative to the Arabidopsis reference gene AtSAND. The values are the means ± S.E. of three biological replicates. Asterisks indicate statistically significant differences compared with the WT control: Student’s t test; *P < 0.05; **0.001 < P < 0.01; ***P < 0.001.
Total root length is an informative phenotype for assessing plant resistance against soilborne pathogens (Okubara et al., 2009). After 14 days of growth in infested soil, significant reduction (>50%) in total root length was observed in the infected plants compared to the noninfected control plants (Figure 2B). Similar to the data in the rosette area, the total root length values in both dorn1-3 and rbohd/f were severely reduced by fungal infection. The OxP2K1 and WT lines exhibited lesser degrees of reduction (Figure 2D and Supplementary Figure S1B). The OxP2K1 line sustained the least reduction compared to all other genotypes. We also measured the infection level of R. solani in the root tissues by real-time PCR (Figure 2E), showing a similar trend as seen in the fungal biomass result in the infected roots. Similar results were obtained when field soil was infested (Supplementary Figures S2B, D). Altogether, our observations showed that dorn1-3 and rbohd/f were highly susceptible, while OxP2K1 was more resistant than the WT. The data suggested that P2K1 is involved in a basal plant resistance to Rhizoctonia infection, and that defects in DAMP signaling through P2K1 and reactive oxygen species (ROS) generation by the NADPH oxidases resulted in enhanced susceptibility.
P2K1 Is Involved in the Upregulation of Defense-Related Genes During R. solani Infection
The expression of a salicylic acid (SA)-induced gene, pathogenesis-related PR1 (Wildermuth et al., 2001), and two JA/ET-induced genes, the defensin PDF1.2 and the JA-ZIM-domain protein JAZ5 (Penninckx et al., 1998; Smirnova et al., 2017), were measured in the root tissues of the WT, dorn1-3, OxP2K1, and rbohd/f plants after growth for 14 days in noninfested and infested soil conditions. Consistent with a previous report (Foley et al., 2013), all these defense-related genes were upregulated after fungal infection in all genotypes tested, but highly upregulated in OxP2K1 compared to the WT, and weakly upregulated in dorn1-3 and rbohd/f after fungal infection (Figure 3). Additionally, the expression level of the defense-related genes were comparable in mock-inoculated roots among all genotypes tested (Supplementary Figure S3). The result further demonstrated that the expression of the defense-related genes was dependent on the P2K1-mediated pathway only upon host damage. In other words, P2K1 plays an important role for the induction of defense-related genes during R. solani infection.
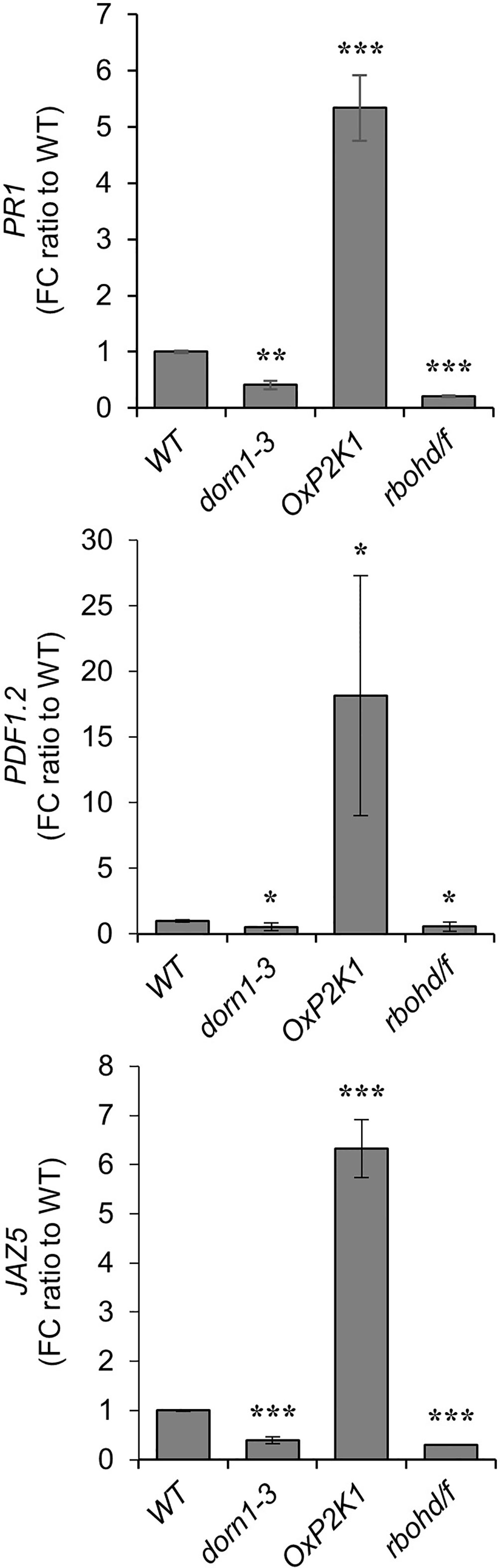
Figure 3 Expression of defense-related genes in the Rhizoctonia solani AG-8-infected roots. The expression of defense-related genes, PR1, PDF1.2, and JAZ5, were measured in the root tissues of the WT, dorn1-3, OxP2K1, and rbohd/f plants after growth for 14 days in noninfested and infested soils. Data are presented as the mean value of fold change (FC) ± S.E. (n = 5) relative to that of WT. Asterisks indicate statistically significant differences compared with the WT control: Student’s t test; *P < 0.05; **0.001 < P < 0.01; ***P < 0.001.
Application of ATP Reduces Infectionof R. solani and Alleviates Stunting of Root Growth
It was important to do one experiment with R. solani AG2-1, the causal agent of the main Rhizoctonia disease, post-emergence damping off in the brassicas. This AG group would be useful to see the effect of exogenously applied ATP on fungal colonization (biomass) at a high resolution, even in the susceptible mutant lines. To this end, colonization of roots by R. solani AG2-1 was tested in a petri plate assay in the presence or absence of ATP. Fungal growth was visible 2–3 days after inoculation (Figure 4), and the infection level was estimated by measuring the fungal growth on the plates and also by quantifying the fungus inside or closely associated with the root tissue. The root-associated fungal biomass varied according to the genotype and ATP treatment. Figure 4 shows that R. solani biomass was reduced in the OxP2K1 line, although it was no difference in dorn1-3, in comparison to that for WT (Figures 4A, B). Additionally, exogenous ATP significantly reduced fungal biomass in roots of the WT and OxP2K1 lines, whereas it failed to attenuate the fungal biomass in the dorn1-3 mutant (Figure 4B). We also measured the infection level of R. solani in the root tissues by real-time PCR (Figure 4C), showing a similar trend as seen in the fungal biomass result on the plates. These findings suggest that extracellular ATP mediates defense against R. solani through P2K1.
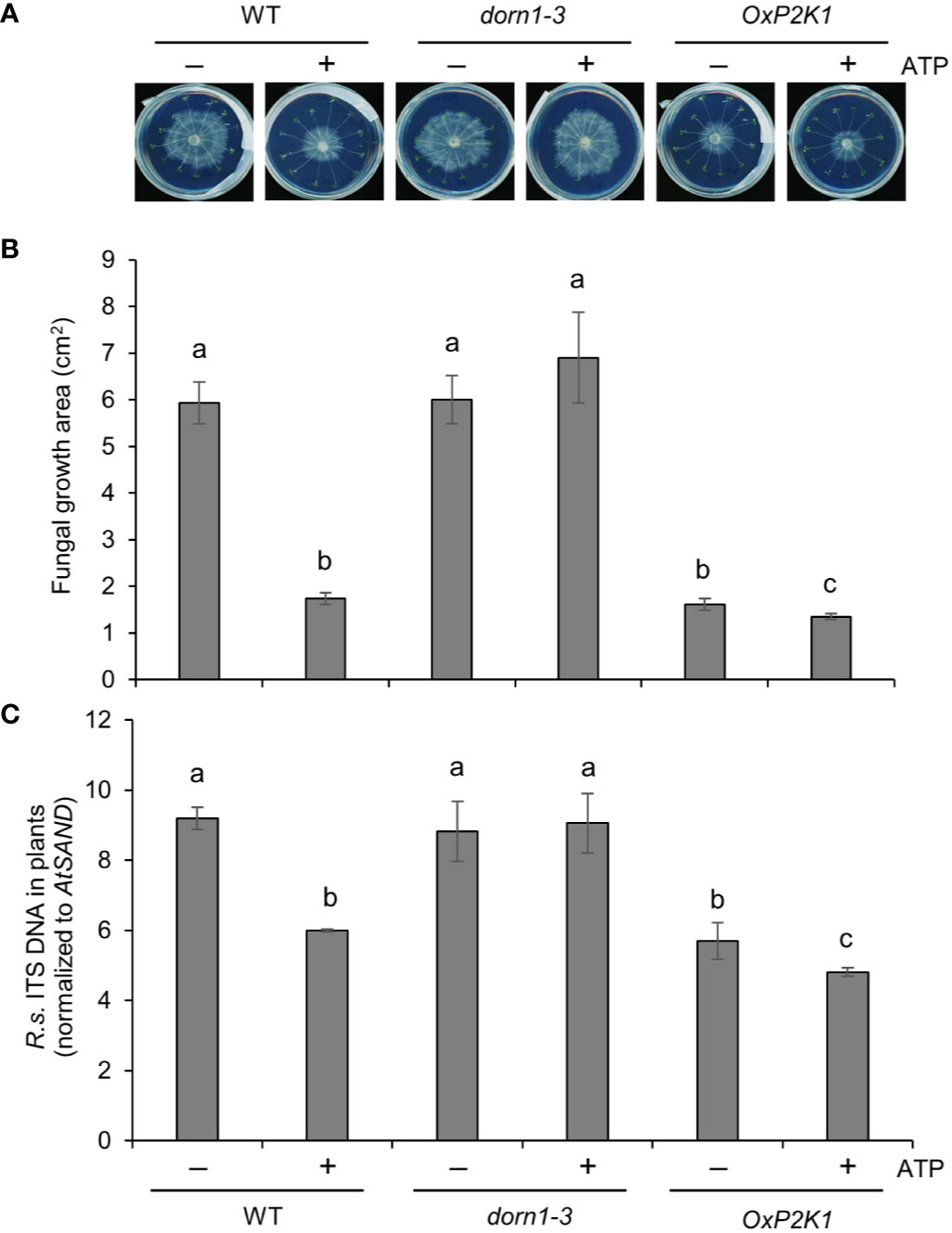
Figure 4 Extracellular ATP reduces Rhizoctonia solani AG2-1 infection in roots. (A) Representative pictures of R. solani infection in MS plates with wild type (WT), dorn1-3, and OxP2K1 seedlings at 2 days post infection. (B) The area of fungal growth on the MS plates in (A). (C) The level of infection in the seedlings quantified by real-time PCR. Abundance is expressed as the ratios of the fungal-specific ITS region relative to the Arabidopsis reference gene AtSAND. The values are the means ± SE of three biological replicates. Different letters indicate significant differences at P < 0.05.
Discussion
The soilborne fungus R. solani is an economically important pathogen (Sneh et al., 1996) causing diseases primarily in the roots or tubers and secondarily in the stems of plants (Keijer et al., 1997; Weinhold and Sinclair, 1996). Although R. solani AG2-1 is typically associated with disease in the brassicas and pulses, it primarily causes post-emergence damping off, and isolates can vary in pathogenicity. In contrast, R. solani AG-8 causes more severe disease symptoms in the field, including pre-emergence damping off and bare patch (Khangura et al., 1999; T. Paulitz, personal communication). Our findings indicated that it was also the stronger pathogen of Arabidopsis in our pathogenicity assay and therefore preferable for generating disease in the Arabidopsis mutants under controlled conditions. Arabidopsis was the host of choice, given that mutants in eATP signaling were not available for other crops.
Rhizoctonia solani is a necrotroph that typically causes cellular damage to acquire nutrients from the host. Necrosis-mediated cellular disruption is a direct mechanism for releasing intracellular ATP into the extracellular matrix (i.e., apoplast). In animals, ATP is released as a DAMP signal when endogenous physiological factors and foreign entities cause cell damage and death, e.g., apoptosis, pyroptosis, and necrosis (Dosch et al., 2018). Similarly, in plants, a high concentration of cytoplasmic ATP is released into the extracellular spaces following exposure to abiotic and biotic stresses (Dark et al., 2011; Ramachandran et al., 2019), suggesting a role of extracellular ATP in stress responses.
In the present study, we hypothesized that extracellular ATP, released upon R. solani-induced cellular damage (i.e., necrosis), activates the purinoceptor P2K1, which initiates a signaling cascade leading to the induction of defense genes. Our data showed that the purinoceptor P2K1 mediated defense against a necrotrophic root infection. The overexpression line of P2K1 was more resistant to both anastomosis groups (AG-8 and AG2-1) of R. solani than the WT. The knockout mutant dorn1-3 exhibited a high susceptibility to AG-8, but not to AG2-1 (this is probably due to different experiment systems used). In addition, ATP addition attenuated the fungal growth in roots of the WT and overexpression lines. This ATP-induced inhibition of fungal growth was abolished in the knockout mutant. These results demonstrated that extracellular ATP is involved in plant immune response to R. solani in roots, where the P2K1 receptor play an essential role to mediate ATP-induced defense. Given that there is no significant difference in the P2K1 expression upon R. solani inoculation (GSE26206) and ATP addition (GSE52610) based on previous transcriptomics (Foley et al., 2013; Choi et al., 2014; Jewell et al., 2019), the numbers of the purinoceptor is likely maintained same no matter when the plant cells get insulted by the fungal pathogen and exposed by extracellular ATP. This evidence further supports our hypothesis that extracellular ATP released upon fungal infection, not upregulation of the receptor, is critical for induction of defense responses to R. solani in the plant roots. To our knowledge, this study is the first demonstration of the role of extracellular ATP for basal resistance against a root pathogen, i.e., R. solani.
Reactive oxygen species produced by NADPH oxidases play a crucial role in resistance to several pathogens, such as Alternaria brassicicola, Magnaporthe oryzae, P. parasitica, B. cinerea, and R. solani (Segmueller et al., 2008; Pogany et al., 2009; Larroque et al., 2013; Foley et al., 2013; Nozaki et al., 2013; Li et al., 2015). Interestingly, our data with the knockout mutant of P2K1 showed a complete loss of resistance that was comparable to the ROS-deficient double mutant rbohd/f, suggesting that ROS generation was as important as extracellular ATP perception in the root defense response. Given that P2K1 directly interacts with and activates RBOHD by phosphorylation (Chen et al., 2017), it is possible that extracellular ATP released upon R. solani infection activates P2K1-RBOHD-mediated ROS signaling, thereby enhancing plant resistance to fungal infection.
Defense against R. solani has been linked to the overall effect produced by the signaling pathways of SA, ET, JA, abscisic acid, and auxin (Foley et al., 2013). In general, the plant defense response is a coordinated mechanism facilitated by the major defense hormones SA and JA/ET (Glazebrook, 2005; Spoel et al., 2007; Hillmer et al., 2017). Salicylic acid is a key modulator of local and systemic resistance. This molecule accumulates in tissues along with a coordinated expression of defense genes, including PR genes (Malamy et al., 1990; Métraux et al., 1990; Maleck et al., 2000). Jasmonic acid and ET are induced during necrotrophic pathogen infection, which induces the expression of several other defense genes, including defensins and proteinase inhibitors (Reymond and Farmer, 1998; Feys and Parker, 2000; Schenk et al., 2000; Devadas et al., 2002). A previous study showed that R. solani induces multiple defense hormone-related genes in potato sprouts (Lehtonen et al., 2008) and Arabidopsis (Foley et al., 2013). In this study, we confirmed that SA- and JA/ET-regulated genes were proportionally higher in OxP2K1 and lower in the dorn1 mutant during fungal infection, suggesting that the expression of these defense-related genes was dependent on the P2K1-mediated pathway upon host damage. Indeed, extracellular ATP induces various defense responses in parallel with plant defense hormones and through an independent mechanism (Jewell et al., 2019). Some of these responses are involved in enhancing resistance against the necrotroph B. cinerea mediated by extracellular ATP-JA-mediated synergistic signaling that required ROS as well as other second messengers, nitrous oxide, and calcium (Tripathi et al., 2018; Tripathi and Tanaka, 2018). Particularly, ROS and calcium signalings mutually interplay in plant immune response (Marcec et al., 2019). Additionally, the expression of many defense-related genes was further exaggerated in OxP2K1, in which ET-regulated genes were shown to be involved additional defense-related functions (Jewell and Tanaka, 2019).
Plants have basal resistance to necrotrophic pathogens, for which extracellular ATP reinforces plant defense responses via defense hormone pathways that may involve secondary metabolites, including tryptophan-derived compounds such as camalexin and glucosinolates, based on previous transcriptomic data (Jewell et al., 2019). To date, many efforts have been made to identify the resistance mechanism against R. solani in different crops. Genetic studies in wheat indicate that both single-gene and multigenic resistance are involved and that several mechanisms of resistance are likely, but until now, the molecular basis for basal resistance has been elusive. A better understanding of extracellular ATP signaling would contribute to breeding crop varieties with increased resistance to soilborne necrotrophic pathogens. Further studies are required to understand the complete mechanism by which extracellular ATP enhances defense against this and other soilborne pathogens, which might provide leads to new management strategies for growers. Very recently, putative orthologs of P2K1/DORN1 have been reported in banana, camelina, and wheat (Li et al., 2016; Okubara et al., 2019; Shan et al., 2020). Such functional studies using crops other than Arabidopsis would allow us to directly use the resistance mechanism against the necrotrophic pathogens.
Data Availability Statement
All datasets presented in this study are included in the article/Supplementary Material.
Author Contributions
PO and KT conceived and designed the study. SK, DT, PO, and KT performed research, analyzed data, and wrote the article.
Funding
This work was supported by the National Science Foundation (grant no. IOS-1557813) and USDA NIFA (Hatch project no. 1015621) to KT and CRIS project number 2090-22000-017-00D to PO.
Conflict of Interest
The authors declare that the research was conducted in the absence of any commercial or financial relationships that could be construed as a potential conflict of interest.
Acknowledgments
We thank Dr. Timothy Paulitz (Washington State University) for providing pathogen strains of R. solani.
Supplementary Material
The Supplementary Material for this article can be found online at: https://www.frontiersin.org/articles/10.3389/fpls.2020.572920/full#supplementary-material
References
Balagué, C., Gouget, A., Bouchez, O., Souriac, C., Haget, N., Boutet-Mercey, S., et al. (2017). The Arabidopsis thaliana lectin receptor kinase LecRK-I.9 is required for full resistance to Pseudomonas syringae and affects jasmonate signalling. Mol. Plant Pathol. 18, 937–948. doi: 10.1111/mpp.12457
Bouwmeester, K., de Sain, M., Weide, R., Gouget, A., Klamer, S., Canut, H., et al. (2011). The lectin receptor kinase LecRK-I.9 is a novel Phytophthora resistance component and a potential host target for a RXLR effector. PLoS Pathog. 7, e1001327. doi: 10.1371/journal.ppat.1001327
Budge, G. E., Shaw, M. W., Colyer, A., Pietravalle, S., Boonham, N. (2009). Molecular tools to investigate Rhizoctonia solani distribution in soil. Plant Pathol. 58, 1071–1080. doi: 10.1111/j.1365-3059.2009.02139.x
Chen, D., Cao, Y., Li, H., Kim, D., Ahsan, N., Thelen, J., et al. (2017). Extracellular ATP elicits DORN1-mediated RBOHD phosphorylation to regulate stomatal aperture. Nat. Commun. 8, 2265. doi: 10.1038/s41467-017-02340-3
Choi, J., Tanaka, K., Cao, Y., Qi, Y., Qiu, J., Liang, Y., et al. (2014). Identification of a plant receptor for extracellular ATP. Science 343, 290–294. doi: 10.1126/science.343.6168.290
Dark, A., Demidchik, V., Richards, S. L., Shabala, S., Davies, J. M. (2011). Release of extracellular purines from plant roots and effect on ion fluxes. Plant Signal Behav. 6, 1855–1857. doi: 10.4161/psb.6.11.17014
DeShields, J. B., Bomberger, R. A., Woodhall, J. W., Wheeler, D. L., Moroz, N., Johnson, D. A., et al. (2018). On-site molecular detection of soil-borne phytopathogens using a portable real-time PCR system. J. Vis. Exp. 132, e56891. doi: 10.3791/56891
Devadas, S. K., Enyedi, A., Raina, R. (2002). The Arabidopsis hrl1 mutation reveals novel overlapping roles for salicylic acid jasmonic acid and ethylene signaling in cell death and defence against pathogens. Plant J. 30, 467–480. doi: 10.1046/j.1365-313X.2002.01300.x
Dosch, M., Gerber, J., Jebbawi, F., Beldi, G. (2018). Mechanisms of ATP Release by Inflammatory Cells. Int. J. Mol. Sci. 19, 1222. doi: 10.3390/ijms19041222
Feys, B. J., Parker, J. E. (2000). Interplay of signaling pathways in plant disease resistance. Trends Genet. 16, 449–455. doi: 10.1016/S0168-9525(00)02107-7
Foley, R. C., Gleason, C. A., Anderson, J. P., Hamann, T., Singh, K. B. (2013). Genetic and Genomic Analysis of Rhizoctonia solani Interactions with Arabidopsis; Evidence of Resistance Mediated through NADPH Oxidases. PLoS One 8, e56814. doi: 10.1371/journal.pone.0056814
Glazebrook, J. (2005). Contrasting mechanisms of defense against biotrophic and necrotrophic pathogens. Annu. Rev. Phytopathol. 43, 205–227. doi: 10.1146/annurev.phyto.43.040204.135923
Helliwell, E., Wang, Q., Yang, Y. (2013). Transgenic rice with inducible ethylene production exhibits broad-spectrum disease resistance to the fungal pathogens Magnaporthe oryzae and Rhizoctonia solani. Plant Biotechnol. J. 11, 33–42. doi: 10.1111/pbi.12004
Hillmer, R. A., Tsuda, K., Rallapalli, G., Asai, S., Truman, W., Papke, M. D., et al. (2017). The highly buffered Arabidopsis immune signaling network conceals the functions of its components. PLoS Genet. 13, e1006639. doi: 10.1371/journal.pgen.1006639
Jewell, J. B., Tanaka, K. (2019). Transcriptomic perspective on extracellular ATP signaling: a few curious trifles. Plant Signal Behav. 14, e1659079. doi: 10.1080/15592324.2019.1659079
Jewell, J. B., Sowders, J. M., He, R., Willis, M. A., Gang, D. R., Tanaka, K. (2019). Extracellular ATP shapes a defense-related transcriptome both independently and along with other defense signaling pathways. Plant Physiol. 179, 1144–1158. doi: 10.1104/pp.18.01301
Julkowska, M. M., Klei, K., Fokkens, L., Haring, M. A., Schranz, M. E., C. Testerink, C. (2016). Natural variation in rosette size under salt stress conditions corresponds to developmental differences between Arabidopsis accessions and allelic variation in the LRR-KISS gene. J. Exp Bot. 67, 2127–2138. doi: 10.1093/jxb/erw015
Keijer, J., Korsman, M. G., Dullemans, A. M., Houterman, P. M., de Bree, J., van Silfhout, C. H. (1997). In vitro analysis of host plant specificity in Rhizoctonia solani. Plant Pathol. 46, 659–669. doi: 10.1046/j.1365-3059.1997.d01-61.x
Khangura, R. K., Barbetti, M. J., Sweetingham, M. W. (1999). Characterization and pathogenicity of Rhizoctonia species on canola. Plant Dis. 83, 714–721. doi: 10.1094/PDIS.1999.83.8.714
Knecht, K., Seyffarth, M., Desel, C., Thurau, T., Sherameti, I., Lou, B., et al. (2010). Expression of BvGLP-1 Encoding a Germin-Like Protein from Sugar Beet in Arabidopsis thaliana Leads to Resistance Against Phytopathogenic Fungi. Mol. Plant-Microbe Int. 23, 446–457. doi: 10.1094/MPMI-23-4-0446
Kouzai, Y., Kimura, M., Watanabe, M., Kusunoki, K., Osaka, D., Suzuki, T., et al. (2018). Salicylic acid-dependent immunity contributes to resistance against Rhizoctonia solani, a necrotrophic fungal agent of sheath blight, in rice and Brachypodium distachyon. New Phytol. 217, 771–783. doi: 10.1111/nph.14849
Larroque, M., Belmas, E., Martinez, T., Vergnes, S., Ladouce, N., Lafitte, C., et al. (2013). Pathogen-associated molecular pattern-triggered immunity and resistance to the root pathogen Phytophthora parasitica in Arabidopsis. J. Exp. Bot. 64, 3615–3625. doi: 10.1093/jxb/ert195
Lehtonen, M., Somervuo, P., Valkonen, J. (2008). Infection with Rhizoctonia solani induces defense genes and systemic resistance in potato sprouts grown without light. Phytopathology 98, 1190–1198. doi: 10.1094/PHYTO-98-11-1190
Li, X. H., Zhang, H. J., Tian, L. M., Huang, L., Liu, S. X., Li, D. Y., et al. (2015). Tomato SIRbohB, a member of the NADPH oxidase family, is required for disease resistance against Botrytis cinerea and tolerance to drought stress. Front. Plant Sci. 6, 14. doi: 10.1016/j.plantsci.2015.02.014
Li, Z., Chakraborty, S., Xu, G. (2016). X-ray crystallographic studies of the extracellular domain of the first plant ATP receptor, DORN1, and the orthologous protein from Camelina sativa. Acta Crist. F72, 782–787. doi: 10.1107/S2053230X16014278
Maeda, S., Dubouzet, J. G., Kondou, Y., Jikumaru, Y., Seo, S., Oda, K., et al. (2019). The rice CYP78A gene BSR2 confers resistance to Rhizoctonia solani and affects seed size and growth. Sci. Rep. 9, 587. doi: 10.1038/s41598-018-37365-1
Mahoney, A., Babiker, E., Paulitz, T., See, D., Okubara, P., Hulbert, S. (2016). Characterizing and mapping resistance in synthetic-derived wheat to Rhizoctonia root rot in a green bridge environment. Phytopathology 106, 1170–1176. doi: 10.1094/PHYTO-02-16-0055-FI
Mahoney, A. K., Babiker, E. M., See, D. R., Paulitz, T. C., Okubara, P. A., Hulbert, S. H. (2017). Analysis and mapping of Rhizoctonia root rot resistance traits from the synthetic wheat (Triticum aestivum L.) line SYN-172. Mol. Breed. 37, 130. doi: 10.1007/s11032-017-0730-9
Malamy, J., Carr, J. P., Klessig, D. F., Raskin, I. (1990). Salicylic acid: a likely endogenous signal in the resistance response of tobacco to viral infection. Science 250, 1002–1004. doi: 10.1126/science.250.4983.1002
Maleck, K., Levine, A., Eulgem, T., Morgan, A., Schmid, J., Lawton, K., et al. (2000). The transcriptome of Arabidopsis thaliana during systemic acquired resistance. Nat. Genet. 26, 403–409. doi: 10.1038/82521
Marcec, M. J., Gilroy, S., Poovaiah, B. W., Tanaka, K. (2019). Mutual interplay of Ca2+ and ROS signaling in plant immune response. Plant Sci. 283, 343–354. doi: 10.1016/j.plantsci.2019.03.004
Métraux, J. P., Signer, H., Ryals, J., Ward, E., Wyss-Benz, M., Gaudin, J., et al. (1990). Increase in salicylic acid at the onset of systemic acquired resistance in cucumber. Science 250, 1004–1006. doi: 10.1126/science.250.4983.1004
Nguyen, C. T., Tanaka, K., Cao, Y., Cho, S. H., Xu, D., Stacey, G. (2016). Computational Analysis of the Ligand Binding Site of the Extracellular ATP Receptor, DORN1. PLoS One 11, e0161894. doi: 10.1371/journal.pone.0161894
Nozaki, M., Kita, K., Kodaira, T., Ishikawa, A. (2013). AtRbohF Contributes to Non-Host Resistance to Magnaporthe oryzae in Arabidopsis. Biosci. Biotechnol. Biochem. 77, 1323–1325. doi: 10.1271/bbb.130092
Okubara, P. A., Schroeder, K. L., Paulitz, T. C. (2008). Identification and quantification of Rhizoctonia solani and R. oryzae using real-time polymerase chain reaction. Phytopathology 98, 837–847. doi: 10.1094/PHYTO-98-7-0837
Okubara, P., Steber, C., DeMacon, V., Walter, N., Paulitz, T., Kidwell, K. K. (2009). Scarlet-Rz1, an EMS-generated hexaploid wheat with tolerance to the soilborne necrotrophic pathogens Rhizoctonia solani AG-8 and R. oryzae. Theor. Appl. Genet. 119, 293–303. doi: 10.1007/s00122-009-1038-x
Okubara, P. A., Dickman, M. B., Blechl, A. E. (2014). Molecular and genetic aspects of controlling the soilborne necrotrophic pathogens Rhizoctonia and Pythium. Plant Sci. 228, 61–70. doi: 10.1016/j.plantsci.2014.02.001
Okubara, P. A., Peetz, A. B., Sharpe, R. M. (2019). Cereal root interactions with soilborne pathogens—from trait to gene and back. Agronomy 9, 188. doi: 10.3390/agronomy9040188
Paulitz, T. C., Okubara, P. A., Schillinger, W. F. (2006). First report of damping-off of canola caused by Rhizoctonia solani AG 2-1 in Washington State. Plant Dis. 90, 829. doi: 10.1094/PD-90-0829B
Peng, X., Hu, Y., Tang, X., Zhou, P., Deng, X., Wang, H., et al. (2012). Constitutive expression of rice WRKY30 gene increases the endogenous jasmonic acid accumulation, PR gene expression and resistance to fungal pathogens in rice. Planta 236, 1485–1498. doi: 10.1007/s00425-012-1698-7
Penninckx, I. A. M. A., Thomma, B. P. H. J., Buchala, A., Metraux, J.-P., Broekaert, W. F. (1998). Concomitant activation of jasmonate and ethylene response pathways is required for induction of a plant defensin gene in Arabidopsis. Plant Cell 10, 2103–2114. doi: 10.1105/tpc.10.12.2103
Pogany, M., von Rad, U., Grun, S., Dongo, A., Pintye, A., Simoneau, P., et al. (2009). Dual Roles of Reactive Oxygen Species and NADPH Oxidase RBOHD in an Arabidopsis-Alternaria Pathosystem. Plant Physiol. 151, 1459–1475. doi: 10.1104/pp.109.141994
Ramachandran, S. R., Kumar, S., Tanaka, K. (2019). Quantification of extracellular ATP in plant suspension cell cultures. Methods Mol. Biol. 1991, 43–54. doi: 10.1007/978-1-4939-9458-8_5
Reymond, P., Farmer, E. E. (1998). Jasmonate and salicylate as global signals for defence gene expression. Curr. Opin. Plant Biol. 1, 404–411. doi: 10.1016/S1369-5266(98)80264-1
Schenk, P. M., Kazan, K., Wilson, I., Anderson, J. P., Richmond, T., Somerville, S. C., et al. (2000). Coordinated plant defense responses in Arabidopsis revealed by cDNA microarray analysis. Proc. Natl. Acad. Sci. U. S. A. 97, 11655–11660. doi: 10.1073/pnas.97.21.11655
Schmittgen, T. D., Livak, K. J. (2008). Analyzing real-time PCR data by the comparative CT method. Nat. Protoc. 3, 1101–1108. doi: 10.1038/nprot.2008.73
Segmueller, N., Kokkelink, L., Giesbert, S., Odinius, D., van Kan, J., Tudzynski, P. (2008). NADPH Oxidases are involved in differentiation and pathogenicity in Botrytis cinerea. Mol. Plant-Microbe Interact. 21, 808–819. doi: 10.1094/MPMI-21-6-0808
Shan, Y., Huang, H., Lian, Q., Li, F., Zhang, J., Zhu, H., et al. (2020). Characterization and function of banana DORN1s during fruit ripening and cold storage. Postharvest Biol. Technol. 167, 111236. doi: 10.1016/j.postharvbio.2020.111236
Sharon, M., Freeman, S., Sneh, B. (2011). Assessment of Resistance Pathways Induced in Arabidopsis thaliana by Hypovirulent Rhizoctonia spp. Isolates Phytopathol. 101, 828–838. doi: 10.1094/PHYTO-09-10-0247
Smirnova, E., Marquis, V., Poirier, L., Aubert, Y., Zumsteg, J., Ménard, R., et al. (2017). Jasmonic Acid Oxidase 2 Hydroxylates Jasmonic Acid and Represses Basal Defense and Resistance Responses against Botrytis cinerea Infection. Mol. Plant 10, 1159–1173. doi: 10.1016/j.molp.2017.07.010
Sneh, B., Burpee, L., Ogoshi, A. (1991). Identification of Rhizoctonia species (St. Paul, MN: APS Press).
Sneh, B., Jabaji-Hare, S., Neate, S., Djist, G. (1996). Rhizoctonia species: taxonomy, molecular biology, ecology, pathology and disease control. Chapter V (p 247-404) and Chapter VIB (p. 445-559) (Dordrecht, The Nether- lands: Kluwer Academic Publishiers).
Spoel, S. H., Johnson, J. S., Dong, X. (2007). Regulation of tradeoffs between plant defenses against pathogens with different lifestyles. Proc. Natl. Acad. Sci. U. S. A. 104, 18842–18847. doi: 10.1073/pnas.0708139104
Sturrock, C. J., Woodhall, J., Brown, M., Walker, C., Mooney, S. J., Ray, R. V. (2015). Effects of damping-off caused by Rhizoctonia solani anastomosis group 2-1 on roots of wheat and oil seed rape quantified using X-ray Computed Tomography and real-time PCR. Front. Plant Sci. 6, 461. doi: 10.3389/fpls.2015.00461
Tanaka, K., Choi, J., Cao, Y., Stacey, G. (2014). Extracellular ATP acts as a damage-associated molecular pattern (DAMP) signal in plants. Front. Plant Sci. 5, 446. doi: 10.3389/fpls.2014.00446
Torres, M. A., Dangl, J. L., Jones, J. D. (2002). Arabidopsis gp91phox homologues AtrbohD and AtrbohF are required for accumulation of reactive oxygen intermediates in the plant defense response. Proc. Natl. Acad. Sci. U. S. A. 99, 517–522. doi: 10.1073/pnas.012452499
Tripathi, D., Tanaka, K. (2018). A crosstalk between extracellular ATP and jasmonate signaling pathways for plant defense. Plant Signal Behav. 13, e1432229. doi: 10.1080/15592324.2018.1432229
Tripathi, D., Zhang, T., Koo, A. J., Stacey, G., Tanaka, K. (2018). Extracellular ATP acts on jasmonate signaling to reinforce plant defense. Plant Physiol. 176, 511–523. doi: 10.1104/pp.17.01477
Weinhold, A. R., Sinclair, J. B. (1996). “Rhizoctonia solani: penetration, colonization and host response,” in Rhizoctonia species: taxonomy, molecular biology, ecology, pathology and disease control. Eds. Sneh, B., Jabaji-Hare, S., Neate, S., Dijst, G. (Dorrecht: Kluwer Academic Publishers), 163–174.
Keywords: extracellular ATP, damage-associated molecular patterns, purinoceptor P2K1, root disease, Rhizoctonia solani
Citation: Kumar S, Tripathi D, Okubara PA and Tanaka K (2020) Purinoceptor P2K1/DORN1 Enhances Plant Resistance Against a Soilborne Fungal Pathogen, Rhizoctonia solani. Front. Plant Sci. 11:572920. doi: 10.3389/fpls.2020.572920
Received: 15 June 2020; Accepted: 09 September 2020;
Published: 25 September 2020.
Edited by:
Massimo E. Maffei, University of Turin, ItalyReviewed by:
Suhua Bai, Qingdao Agricultural University, ChinaLucía Jordá, Polytechnic University of Madrid, Spain
Copyright © 2020 Kumar, Tripathi, Okubara and Tanaka. This is an open-access article distributed under the terms of the Creative Commons Attribution License (CC BY). The use, distribution or reproduction in other forums is permitted, provided the original author(s) and the copyright owner(s) are credited and that the original publication in this journal is cited, in accordance with accepted academic practice. No use, distribution or reproduction is permitted which does not comply with these terms.
*Correspondence: Kiwamu Tanaka, a2l3YW11LnRhbmFrYUB3c3UuZWR1
†Present address: Sonika Kumar, Department of Plant and Environmental Sciences, Clemson University, Clemson, SC, United States
Diwaker Tripathi, Department of Biology, University of Washington, Seattle, WA, United States
‡These authors have contributed equally to this work