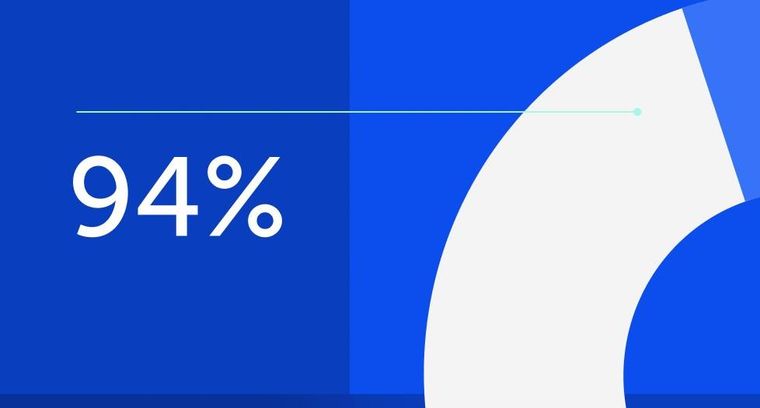
94% of researchers rate our articles as excellent or good
Learn more about the work of our research integrity team to safeguard the quality of each article we publish.
Find out more
REVIEW article
Front. Plant Sci., 15 July 2020
Sec. Crop and Product Physiology
Volume 11 - 2020 | https://doi.org/10.3389/fpls.2020.00988
Crops during their early growth stages are vulnerable to a wide range of environmental stressors; thus, earlier seed invigoration and seedling establishment are essential in crop production. As an alternative to synthetic chemical treatments, plasma technology could be one of the emerging technologies to enhance seed germination and seedling vigor by managing environmental stressors. Recent studies have shown its beneficial effects in various stress conditions, suggesting that plasma treatment can be used for early crop stress management. This paper reviewed the effects of different types of plasma treatments on plant responses in terms of the seed surface environment (seed scarification and pathogen inactivation) and physiological processes (an enhanced antioxidant system and activated defense response) during the early growth stages of plants. As a result, plasma treatment can enhance seed invigoration and seedling establishment by alleviating the adverse effects of environmental stressors such as drought, salinity, and pathogen infection. More information on plasma applications and their mechanisms against a broad range of stressors is required to establish a better plasma technology for early crop stress management.
Rapid seed invigoration and seedling establishment during the early growth stages of crops are necessary to prevent crop yield loss due to unfavorable environments. Seed germination and early seedling growth are the most sensitive growth stages for a crop to a wide range of environmental stressors (Ashraf and Foolad, 2005; Jisha et al., 2013; Sharma et al., 2015). Once the stressors affect the seeds or plants during the early growth stages, they can delay the onset, reduce the rate, and decrease the uniformity of germination and the emergence of seedlings. As a result, plant growth and final crop yield are reduced. Thus, seed invigoration is used to improve germination and seedling vigor (seedling size, health, and growth rate). Many efforts have been made to enhance seed germination and seedling vigor under both adverse and non-adverse conditions by applying various methods, including chemical treatments such as sulfuric acid, pesticides, and chlorine-based disinfectants (Ashraf and Foolad, 2005; Kimura and Islam, 2012; Jisha et al., 2013; Sharma et al., 2015) (see Figure 1). The synthetic chemical treatments are based on soaking seeds or spraying young plants using chemical substance-containing solutions. However, the use of synthetic chemical treatments can increase chemical pollutants in seeds or young plants and consequently can cause adverse effects on human health and the environment.
Figure 1 A comparison of plasma technology and conventional treatments with three factors affecting seed invigoration and seedling establishment: 1) seed scarification, 2) pathogen inactivation, and 3) enhanced cellular antioxidant systems. Seed scarification conventionally is done by acid treatment, heating, freeze-thawing, and mechanical scarifier. Plasma technology, as a particle bombardment or an oxidizing agent or both, can also be used for seed scarification at presowing. Additionally, plasma treatment, like other possible agents, can be applied at the early growth stage. Traditionally, synthetic chemicals have been commonly used for pathogen inactivation and antioxidant system activation.
Plasma technology has been widely designated as an advanced oxidation process (Misra et al., 2011; Ekezie et al., 2017; Fan and Song, 2020). It has advantages over conventional treatments based on synthetic chemical substances, although large-scale applications of plasma technology are still expensive. A significant benefit of plasma technology is associated with its synergistic effects on seed germination and seedling vigor without any synthetic chemical residues. In general, an apparatus for plasma treatment simply consists of electrodes for plasma generation, a treatment chamber that accommodates the electrodes, and electric power to supply current to the electrodes. When a high electric discharge is applied to air or an aqueous solution in a chamber, reactive oxygen species (ROS, e.g., superoxides, singlet oxygens, atomic oxygen, ozone, hydrogen peroxide, and hydroxyl radicals), reactive nitrogen species (RNS, e.g., nitric oxide, nitrogen dioxide, nitrate, nitrite, and peroxynitrite), and ultraviolet (UV) photons are mainly generated from the plasma discharge (Selwyn et al., 2001; Laroussi and Leipold, 2004; Song et al., 2020). ROS, RNS, and UV have been independently used to scarify seeds (a technique to soften the seed coat while keeping the seed viable), inactivate seed-borne pathogens, and enhance antioxidant defense systems in crop plants (Jisha et al., 2013; Araújo et al., 2016; Antoniou et al., 2016; Thomas and Puthur, 2017). The synergistic effects of plasma treatment have recently been reported for a wide range of crops and reviewed by Randeniya and de Groot (2015), Ito et al. (2018), and Adhikari et al. (2020).
However, no review has yet summarized plasma applications and their protective mechanisms against a broad range of stressors. The potential applications of plasma technology for early crop stress management are largely unknown. This review evaluated the effects of the different types of plasma treatments on plant responses in terms of the seed surface environment (seed scarification and pathogen inactivation) and physiological processes (an enhanced antioxidant system and activated defense response) during the early growth stages of plants. Recent information on plasma applications and their mechanisms against a broad range of stressors was reviewed from these two perspectives. Thus, this review proposes that plasma technology has potential uses for seed invigoration and seedling establishment under stressful conditions.
The seed coat regulates seed germination through its thickness and permeability. Therefore, seeds with thick coats are incapable of water uptake rapidly unless it is scarified (Noodén et al., 1985). In particular, legumes (e.g., wild soybeans) have highly thickened and impermeable seed coats. In soybeans, a seed coat/embryo weight ratio higher than 0.1 indicates an impermeable seed coat (Noodén et al., 1985; Yaklich et al., 1986; de Souza and Marcos-Filho, 2001; Zhou et al., 2010). Therefore, seed scarification may often be required for seed invigoration and seedling establishment. Table 1 summarizes the scarification effects of plasma treatments on seeds depending on the treatment conditions of the plasma sources, including radiofrequency (RF) discharge, dielectric barrier discharge (DBD), and other types of plasma sources. Power (W) and exposure time (minutes) are vital operating parameters to describe the beneficial effects among the individual elements of the plasma treatment (see Supplementary Table 1 for additional factors).
Table 1 The scarification effects of the plasma treatments on seeds from a wide range of crops, including cereals, legumes, and vegetables.
RF discharge is extensively used to confirm plasma effects on seed invigoration of crops in a temperature-controlled chamber depending on the crop species, feed gas, power, and exposure time. Air and helium have been commonly used as feed gases for plasma generation driven by the RF discharge. RF discharge in air increased the seed invigoration of wheat, maize, and mung bean (Bormashenko et al., 2012; Filatova et al., 2013; Filatova et al., 2014; Sadhu et al., 2017). In the case of the common bean, a discharge in air reduced the time to achieve 50% germination, but it showed no significant change in the final percentage of germination (Bormashenko et al., 2015). Moreover, an excessive exposure of 20 min to an air-based discharge with a high power intensity of 100 W decreased the final seed germination of wheat (Filatova et al., 2013). RF discharge in helium improved seed invigoration with a 9% and 7% increase in the germination of soybeans and oilseed rapes compared with that of the untreated control, respectively, when generated with a power intensity of 80 to 100 W for an exposure time of 0.25 min (Li et al., 2014; Li et al., 2015). However, Li et al. (2014) reported that the exposure of a helium-based RF discharge with a power intensity lower than 60 W had no significant effect on the seed germination of soybeans. This result might be associated with the thick and impermeable testa (outer layer) of the soybean seed coat (Ma et al., 2004; Moïse et al., 2005; Shao et al., 2007). RF discharge has shown contrasting effects depending on the feed gases used. Volin et al. (2000) observed that RF discharge reduced the germination time of maize and soybean when generated from aniline (C6H5NH2) or cyclohexane (C6H12). However, RF discharge delayed the germination time of maize, radish, and some legumes such as soybeans, peas, and common beans when generated from carbon tetrafluoride (CF4) or octadecafluorodecalin (ODFD) otherwise known as perfluorodecalin (Volin et al., 2000). Additionally, RF discharge sometimes showed no significant effect on the germination time of maize when generated from hydrazine (N2H4) (Volin et al., 2000).
Dielectric barrier discharge (DBD) has been proven to be effective for seed germination and seedling vigor when generated under optimal conditions. DBD in air induced faster germination, leading to a better germination rate and early seedling growth of wheat, barley, and peas under laboratory conditions (Dobrin et al., 2015; Stolárik et al., 2015; Li et al., 2017; Park et al., 2018). A DBD with a power intensity of 1.5 to 2.7 W for an exposure of 7 to 15 min improved the seed invigoration of wheat by a maximum value of 47% compared with that of the untreated control (Dobrin et al., 2015; Li et al., 2017). In the case of a higher power intensity (370 to 400 W), DBD in air improved the seed invigoration by a 31% and 51% increase in peas and barley after an exposure of 2 and 0.2 min compared with that of the untreated control, respectively (Stolárik et al., 2015; Park et al., 2018). Additionally, DBD in nitrogen enhanced the seed germination and early seedling growth of barley and spinach (Ji et al., 2016; Park et al., 2018).
Other discharge plasmas, including corona, arc, glow, and microwave discharges, also have shown stimulating effects on seed invigoration of some crops although their maximum values differ depending on the treatment conditions of the plasma sources (Šerá et al., 2010; Shao et al., 2013; Jiang et al., 2014a; Chen et al., 2016; Khamsen et al., 2016; Roy et al., 2018).
These results suggest that plasma treatment can enhance the water absorption of seeds, thus leading to a better invigoration of crops. The beneficial effect of plasma treatment on seed invigoration is mainly associated with its power (W) and exposure time (min) (Figure 2). For example, the plasma treatment of seeds, when generated with a power of 100 W and an exposure time ≤ 10 min, can enhance the seed invigoration of crops without plasma-induced damage. These parameters for plasma treatment must be further optimized based on the crop species.
Figure 2 Maximum seed invigoration (% of control) as a function of the power (W) and exposure time (min) of the plasma treatments for a wide range of crops, which were described in the literature presented in Table 1.
Pathogen inactivation by plasma treatment prevents plant diseases in crops (Table 2). In various studies, the power (W) and exposure time (min) are the most important determinants to inactivate seed-borne pathogens (see Supplementary Table 2 for additional factors). In general, seed germination and early seedling growth are susceptible to plant diseases that are caused by seed-borne bacteria and fungi during crop growing seasons.
Table 2 The inactivation effects of the plasma treatments on seed-borne bacteria and fungi to prevent plant diseases in crops such as some cereals, legumes, and vegetables.
Seed-borne pathogens cause plant diseases leading to reduced seed germination and seedling establishment of crops; however, RF discharge can inactivate seed-borne bacteria and fungi. The seed-borne fungi Alternaria and Fusarium species cause a range of economically significant diseases in a large variety of crops, including cereals, legumes, and vegetables (Thomma, 2003; Khan et al., 2006). In a vacuum chamber, an RF plasma treatment for 8 min with a power intensity of 77 W reduced fungal infection by the Alternaria and Fusarium species by a maximum value of 71% and 99% in maize and wheat, respectively (Filatova et al., 2014). With a similar power intensity of 80 W in a vacuum, an RF discharge inactivated the bacterial pathogen Ralstonia solanacearum after an exposure time of 0.25 min (Jiang et al., 2014b). Ralstonia solanacearum causes bacterial wilt symptoms on young tomato plants (Eljounaidi et al., 2016).
Other types of plasma sources such as DBD and arc discharge also have been shown to have inactivation effects on a wide range of filamentous fungi, thus resulting in a higher survival of crops. In the case of Gibberella fujikuroi (synonym, Fusarium fujikuroi), plasma treatments with DBD and arc discharge effectively inactivated its growth and thus reduced the fungal infection of rice by a maximum value of 51% under environmentally controlled conditions (Jo et al., 2014; Kang et al., 2015). Similarly, DBD in air effectively prevented the fungal infection of sweet basil in an in vitro seed culture from naturally established fungi including the Alternaria, Aspergillus, and Penicillium species by approximately 44% when generated with a power intensity of 6.5 W for an exposure time of 5 min (Ambrico et al., 2017). With a high power intensity of 400 W, DBD in air completely inhibited the growth of fungal pathogens, including Fusarium nivale, F. culmorum, Aspergillus flavus, and Trichothecium roseum, after an exposure of 4 min to wheat seeds in vitro (Zahoranová et al., 2016). Even in the case of a seed mixture, DBD treatment effectively reduced fungal contamination by the Aspergillus and Penicillium species (Selcuk et al., 2008). However, if no seed treatment is applied, fungal pathogens including the Fusarium and Aspergillus species and Trichothecium roseum are capable of inhibiting plant growth and the development of cereal and legume crops, thus resulting in severe crop loss (Tu, 1985; Parry et al., 1995; Goswami and Kistler, 2004; Khan et al., 2006; Klich, 2007; Palencia et al., 2010; Scherm et al., 2013). Additionally, the seed-borne Fusarium, Aspergillus, and Penicillium species produce plenty of mycotoxins that are potentially toxic to the health of both humans and animals (Kumar et al., 2008; Amaike and Keller, 2011).
Thus, plasma treatment of seeds can be used to prevent plant diseases in a wide range of crops, including cereals, legumes, and vegetables. According to Figure 3, plasma treatments might require a high power and long exposure to inactivate seed-borne pathogens effectively during seed germination. For example, a plasma exposure generated with a power of 100 W and an exposure time ≥ 10 min almost inactivates seed-borne pathogens in wheat. There are still other considerations when determining the standardized values for the power (W) and exposure time (min) of the plasma treatment because various parameters for pathogen inactivation can commonly occur depending on the environmental conditions.
Figure 3 Maximum infection control (% of control) as a function of the power (W) and exposure time (min) of the plasma treatments for a few crops, which were described in the literature presented in Table 2.
Plants possess effective antioxidant systems to scavenge intracellular ROS and to protect cells against oxidative damage. Antioxidant defense systems consist of non-enzymatic components, such as quercetin and polyphenols, and enzymatic components, such as superoxide dismutase (SOD), catalase (CAT), and peroxidase (POD), in plant cells (Gill and Tuteja, 2010; Agati et al., 2012). The antioxidant enzymes SOD, CAT, and POD respond together when cells are exposed to excess ROS, although they work in different subcellular compartments (Mittler, 2002; Mittler et al., 2004; Sharma et al., 2012). SODs in almost all cellular compartments catalyze the dismutation (or partitioning) of the superoxide (O2-) radical into hydrogen peroxide (H2O2) and an ordinary molecular oxygen (O2). Finally, CAT in peroxisomes and PODs in the cytosol detoxify the hydrogen peroxide (H2O2) by catalyzing its reduction to water (H2O). Together with these enzymes, non-enzymatic antioxidants can provide cells with highly efficient machinery to detoxify the molecular species of active oxygen. After plasma treatment, the enhancement of these antioxidant systems has recently been reported in a few crops (Table 3).
Table 3 The enhancement effect of the plasma treatments on cellular antioxidant systems in crops, including cereals, legumes, and vegetables.
Cellular antioxidant systems are effectively enhanced by plasma treatments with a low power (W) and short exposure (min) (see Supplementary Table 3 for additional factors). RF discharge, when generated with a power intensity of 80 to 100 W for a short exposure time of 0.25 min, rapidly increased the POD activity of tomato seedlings and the CAT and SOD activities of oil rapeseed seedlings when compared with the untreated control under environmentally controlled conditions (Jiang et al., 2014b; Li et al., 2015). Similarly, growing plants of wheat, maize, and soybeans had higher activities in the antioxidant enzymes POD, CAT, or SOD after DBD exposure to their seeds (Henselová et al., 2012; Guo et al., 2017; Iranbakhsh et al., 2017; Li et al., 2017; Zhang et al., 2017). In particular, DBD in air enhanced the POD and SOD activities of wheat seedlings after its exposure of up to 4 min with a power intensity of 1.5 W (Guo et al., 2017; Li et al., 2017). Wheat seedlings that were directly exposed to DBD for 1 min had a higher POD activity compared with the untreated control (Iranbakhsh et al., 2018). In the case of arc discharge, a short exposure enhanced the POD activity of tomato seedlings (Yin et al., 2005).
Dielectric barrier discharge has shown positive effects on the contents of the total phenolic compounds under laboratory conditions, although its effects depend on the types of feed gas. Ji et al. (2016) reported that an increase in the total phenolic compounds was observed in spinach seedlings after a nitrogen-based DBD treatment with an increasing duration of up to 5 min, while those treated with an air-based DBD and the same exposure time had a decrease in the total phenolic compounds. A similar study with micro DBD showed a higher content of total phenolic compounds in coriander seedlings after a nitrogen-based treatment up to 1 min (Ji et al., 2015). In contrast, a discharge in air increased the total phenolic compounds in the growing plants of wheat and rice when their seeds had a longer exposure time (Šerá et al., 2010; Chen et al., 2016).
These results indicate that plasma treatments generated with a low power and short exposure time (for example, a power of 10 W and an exposure time ≤ 10 min) can enhance cellular antioxidant systems (Figure 4). In particular, antioxidant enzyme activities are increased by 8% to 100% compared to the untreated control. More experimental evidence is required to support the enhancement of the cellular antioxidant systems after plasma treatment.
Figure 4 Enhanced enzyme activity (% of control) as a function of the power (W) and exposure time (min) of the plasma treatments in seeds (or seedlings), which were described in the literature presented in Table 3.
Recent studies have suggested the potential beneficial role of plasma treatment on crop stress tolerance under environmentally controlled conditions (Table 4 and Supplementary Table 4). The RF discharge treatment of seeds, generated with a power intensity of 40 to 100 W and an exposure time of 0.25 min, enhanced crop tolerance to drought stress in oilseed rape (Li et al., 2015) and alfalfa (Feng et al., 2018). Li et al. (2015) demonstrated that plasma treatment enhanced the water absorption of the seeds, increased the activities of antioxidant enzymes, and increased the accumulation of soluble sugars and proteins as osmolytes during stress. A similar study on RF discharge treatment reported plant disease resistance to Ralstonia solanacearum, which causes bacterial wilt in tomatoes. Jiang et al. (2014b) observed that plasma treatment induced a rapid increase in the H2O2 concentration, which sequentially increased the activities of antioxidant and defense-related enzymes in tomato leaves inoculated with R. solanacearum. In the case of DBD plasma, the optimal exposure also enhanced plant resistance to two seed-borne rice seedling diseases (bakanae disease and bacterial seedling blight) through H2O2-mediated signaling activating defense-related responses (Ochi et al., 2017). DBD treatment of wheat seeds increased the activities of antioxidant and defense-related enzymes, which suggests a potential role for plasma treatment in crop stress tolerance (Iranbakhsh et al., 2017). Guo et al. (2017) demonstrated that DBD treatment enhanced the water absorption of wheat seeds due to their surface modification. This modification alleviated drought-induced oxidative damage by regulating various biological processes (e.g., hormone-mediated signaling, drought-tolerant-related gene expression, antioxidant enzyme activation, and osmolyte accumulation). Interestingly, direct treatment of DBD on wheat seedlings also alleviated salinity-induced oxidative damage by inducing stress-related gene expression and activating antioxidant and defense-related enzymes (Iranbakhsh et al., 2018). Bußler et al. (2015) observed that repeated DBD treatment induced plant acclimation to other oxidative stresses (e.g., ROS, RNS, and UV) in peas in the early growth stage by increasing the concentration of flavonoid glycosides.
Thus, plasma treatments can enhance crop tolerance before stress events. The optimal plasma exposure can improve seed germination and seedling growth by modulating the seed surface environment (e.g., seed scarification and pathogen inactivation) and physiological processes (e.g., enhanced antioxidant systems and activated defense responses) in seeds under stressful conditions such as drought, salinity, and pathogen infection (Figure 5). In particular, only a plasma exposure with a low power and a short exposure time (for example, power of 10 W and an exposure time ≤ 10 min) can regulate plasma-induced crop tolerance (Figure 6).
Figure 5 Crop response as a function of power (W) and exposure time (min) of the plasma treatment. The optimal plasma exposure can enhance seed germination and seedling growth before stress events.
Figure 6 Enhanced stress tolerance (% of control) as a function of the power (W) and exposure time (min) of the plasma treatments on seeds (or seedlings), which were described in the literature presented in Table 4.
The plasma-induced stress tolerance might result from various interactions between the plasma (e.g., ROS, RNS, and UV) and the seed surface, seed-borne pathogens, and cellular homeostasis, respectively. Although the plasma-induced effects are still poorly understood, their potential mechanisms for crop stress tolerance can be suggested based on the review of the literature on plasma-induced plant responses (Section 2). The ROS, RNS, and UV of plasma can change the physical and chemical properties of the seed surface before exposure to environmental stressors, thus enabling seeds to be more hydrophilic and permeable to water. Scanning electron microscopy studies have shown partial degradation of cellulose and formation of cracks on the surface of plasma-treated seeds (Šerá et al., 2010; Mitra et al., 2014; Tong et al., 2014; Stolárik et al., 2015; Ji et al., 2016; Zhou et al., 2016; Li et al., 2017; Guo et al., 2018). Additionally, Fourier transform infrared spectroscopy and optical emission spectra studies have shown the formation of oxygen- and nitrogen-containing groups at the surface of treated samples (Filatova et al., 2013; Guo et al., 2017; Wang et al., 2017). Finally, plasma-treated seeds are hydrophilic and have cracks in the seed coat, which are beneficial for water uptake before exposure to drought stress (Li et al., 2015; Guo et al., 2017). Thus, the enhancement of water absorption can trigger faster germination and earlier seedling vigor by regulating endogenous hormones and hydrolytic enzymes during imbibition and sequentially supplying nutrients to an actively growing embryo (Li et al., 2014; Stolárik et al., 2015; Chen et al., 2016; Ji et al., 2016; Guo et al., 2017; Sadhu et al., 2017). Additionally, plasma-induced ROS, RNS, and UV can lead to better seed germination and seedling establishment under stressful conditions as follows: 1) reduce infection with pathogenic microorganisms in germinating seeds or growing plants (Selcuk et al., 2008; Filatova et al., 2014; Jiang et al., 2014b; Zahoranová et al., 2016; Ono et al., 2017; Lee et al., 2019), 2) regulate ROS homeostasis through the antioxidant machinery of plants (Yin et al., 2005; Šerá et al., 2010; Henselová et al., 2012; Bußler et al., 2015; Li et al., 2015; Chen et al., 2016; Ji et al., 2016; Guo et al., 2017; Li et al., 2017; Zhang et al., 2017), and 3) activate other defense-related responses in plant cells (Iranbakhsh et al., 2017; Iranbakhsh et al., 2018). Many studies have shown that the application of exogenous ROS, RNS, or UV radiation has similar effects on seeds, thus resulting in an enhanced stress tolerance before stress events (Antoniou et al., 2016; Thomas and Puthur, 2017). Altogether, plasma-induced ROS, RNS, and UV could promote crop stress tolerance by regulating surface hydrophilicity, infection with pathogenic microorganisms, and diverse cellular mechanisms (Figure 7).
Figure 7 The proposed mechanisms of the enhanced stress tolerance to environmental stressors in plasma-treated seeds (or seedlings). The plasma treatment (e.g., ROS, RNS, and UV) can enhance crop tolerance before a stress event by modulating the seed surface environment (e.g., hydrophilicity and pathogen inactivation) and physiological processes (e.g., enhanced antioxidant system and activated defense response) in the seed (or seedling).
Seed invigoration and seedling establishment under stressful conditions are highly variable depending on numerous factors, including biological (e.g., crop species, cultivar, and growth and developmental stages) and environmental factors (e.g., timing, duration, and intensity of the exposure to the stressor). There is little or no information for establishing crop stress management practices using plasma technology. To our knowledge, there are only a few studies under laboratory conditions on crop tolerance to disease (Jiang et al., 2014b; Ochi et al., 2017), drought (Li et al., 2015; Guo et al., 2017; Feng et al., 2018), salinity (Iranbakhsh et al., 2018), and oxidative stress (Bußler et al., 2015; Iranbakhsh et al., 2017) using plasma technology. Therefore, further studies are needed to optimize the effectiveness of plasma treatment on crop tolerance to a broad range of stressors. For example, flooding is highly detrimental to upland crops (Arduini et al., 2019; Arduini et al., 2020). Thus, plasma treatment could be useful to alleviate flooding stress, as demonstrated by a similar seed treatment using a magnetic field (Balakhnina et al., 2015). Future studies should extensively investigate plasma-induced stress tolerance under laboratory, greenhouse, and field conditions. Crop growth is greatly affected not only by biological and environmental factors but also by culture (e.g., irrigation, tillage, and fertilization) and plasma operating factors (e.g., plasma type, power, and exposure time). Further studies are necessary to determine the physiological, biochemical, and molecular mechanisms of stress tolerance in plasma-treated seeds or plants. More knowledge about the embryo (which is a young plant itself) within the seed is required to understand better the mechanisms of plant responses after plasma exposure. Specifically, a better understanding is necessary for the epigenetic changes and long-lasting plasma effects on the whole plant life cycle without any mutations.
Plasma applications have been widely used for seed scarification, pathogen inactivation, and antioxidant system activation with proven beneficial effects on seed invigoration and seedling establishment under laboratory and greenhouse conditions. Moreover, the optimal plasma exposure can enhance crop tolerance before stress events (e.g., drought stress, plant disease, and oxidative stress) by modulating the seed surface environment (e.g., seed scarification and pathogen inactivation) and physiological processes (e.g., enhanced antioxidant system and activated defense response) in seeds. This promising technology is potentially valuable for alleviating the adverse effects of environmental stressors on seed germination and seedling growth in crop production. However, information on plasma effects under stressful conditions is limited to a few crops under laboratory conditions. Further studies are necessary to fully understand the effectiveness of plasma applications to a broad range of stressors under field and greenhouse conditions. This review suggests that the power (W) and exposure time (min) are vital operating parameters of the plasma treatment that affect the phenomenon of the plasma. More experimental evidence is needed to adopt the use of the power (W) and the exposure time (min) as standardized values for the plasma treatment of various crops (Figure 8).
Figure 8 The most important parameters for plasma applications to enhance seed scarification, pathogen inactivation, cellular antioxidant systems, and stress tolerance in seeds or plants.
J-SS conceived the idea for the topic, drafted and revised the manuscript, and initially provided all the Tables. SK helped draft the outline of the manuscript and provide most of the Figures. SR helped revise the Tables. JO provided Figure 7. D-SK helped draft the outline of the manuscript. All the authors critically reviewed the manuscript. All authors contributed to the article and approved the submitted version.
This work was supported by the R&D Program of the “Plasma Advanced Technology for Agriculture and Food (Plasma Farming, Project No. EN2025)” through the National Fusion Research Institute of Korea (NFRI) funded by government funds.
The authors declare that the research was conducted in the absence of any commercial or financial relationships that could be construed as a potential conflict of interest.
The Supplementary Material for this article can be found online at: https://www.frontiersin.org/articles/10.3389/fpls.2020.00988/full#supplementary-material
RF, radiofrequency; DBD, dielectric barrier discharge; ROS, reactive oxygen species; RNS, reactive nitrogen species; SOD, superoxide dismutase; CAT, catalase; POD, peroxidase; PAL, phenylalanine ammonia lyase.
Adhikari, B., Pangomm, K., Veerana, M., Mitra, S., Park, G. (2020). Plant disease control by nonthermal atmospheric-pressure plasma. Front. Plant Sci. 11, 77. doi: 10.3389/fpls.2020.00077
Agati, G., Azzarello, E., Pollastri, S., Tattani, M. (2012). Flavonoids as antioxidants in plants: location and functional significance. Plant Sci. 196, 67–76. doi: 10.1016/j.plantsci.2012.07.014
Amaike, S., Keller, N. P. (2011). Aspergillus flavus. Annu. Rev. Phytopathol. 49, 107–133. doi: 10.1146/annurev-phyto-072910-095221
Ambrico, P. F., Šimek, M., Morano, M., De Miccolis Angelini, R. M., Minafra, A., Trotti, P., et al. (2017). Reduction of microbial contamination and improvement of germination of sweet basil (Ocimum basilicum L.) seeds via surface dielectric barrier discharge. J. Phys. D.: Appl. Phys. 50, 305401. doi: 10.1088/1361-6463/aa77c8
Antoniou, C., Savvides, A., Christou, A., Fotopoulos, V. (2016). Unravelling chemical priming machinery in plants: the role of reactive oxygen-nitrogen-sulfur species in abiotic stress tolerance enhancement. Curr. Opin. Plant Biol. 33, 101–107. doi: 10.1016/j.pbi.2016.06.020
Araújo, S. S., Paparella, S., Dondi, D., Bentivoglio, A., Carbonera, D., Balestrazzi, A. (2016). Physical methods for seed invigoration: advantages and challenges in seed technology. Front. Plant Sci. 7, 646. doi: 10.3389/fpls.2016.00646
Arduini, I., Baldanzi, M., Pampana, S. (2019). Reduced growth and nitrogen uptake during waterlogging at tillering permanently affect yield components in late sown oats. Front. Plant Sci. 10, 1087. doi: 10.3389/fpls.2019.01087
Arduini, I., Kokubun, M., Shao, G., Licausi, F. (Ed.) (2020). Crop response to waterlogging (Lausanne: Frontiers Media SA). doi: 10.3389/978-2-88963-366-1
Ashraf, M., Foolad, M. R. (2005). Pre-sowing seed treatment – A shotgun approach to improve germination, plant growth, and crop yield under saline and non-saline conditions. Adv. Agron. 88, 223–271. doi: 10.1016/S0065-2113(05)88006-X
Balakhnina, T., Bulak, P., Nosalewicz, M., Pietruszewski, S., Włodarczyk, T. (2015). The influence of wheat Triticum aestivum L. seed pre-sowing treatment with magnetic fields on germination, seedling growth, and antioxidant potential under optimal soil watering and flooding. Acta Physiol. Plant 37, 59. doi: 10.1007/s11738-015-1802-2
Bormashenko, E., Grynyov, R., Bormashenko, Y., Drori, E. (2012). Cold radiofrequency plasma treatment modifies wettability and germination speed of plant seeds. Sci. Rep. 2, 741–748. doi: 10.1038/srep00741
Bormashenko, E., Shapira, Y., Grynyov, R., Bormashenko, Y., Drori, E. (2015). Interaction of cold radiofrequency plasma with seeds of beans (Phaseolus vulgaris). J. Exp. Bot. 66, 4013–4021. doi: 10.1093/jxb/erv206
Bußler, S., Herppich, W. B., Neugart, S., Schreiner, M., Ehlbeck, J., Rohn, S., et al. (2015). Impact of cold atmospheric pressure plasma on physiology and flavonol glycoside profile of peas (Pisum sativum ‘Salamanca'). Food Res. Int. 76, 132–141. doi: 10.1016/j.foodres.2015.03.045
Chen, H. H., Chang, H. C., Chen, Y. K., Hung, C. L., Lin, S. Y., Chen, Y. S. (2016). An improved process for high nutrition of germinated brown rice production: Low-pressure plasma. Food Chem. 191, 120–127. doi: 10.1016/j.foodchem.2015.01.083
de Souza, F. D., Marcos-Filho, J. (2001). The seed coat as a modulator of seed-environment relationships in Fabaceae. Rev. Bras. Bot. 24, 365–375. doi: 10.1590/S0100-84042001000400002
Dobrin, D., Magureanu, M., Mandache, N. B., Ionita, M. D. (2015). The effect of nonthermal plasma treatment on wheat germination and early growth. Innov. Food Sci. Emerg. Technol. 29, 255–260. doi: 10.1016/j.ifset.2015.02.006
Ekezie, F. G. C., Sun, D. W., Cheng, J. H. (2017). A review on recent advances in cold plasma technology for the food industry: current applications and future trends. Trends Food Sci. Tech. 69, 46–58. doi: 10.1016/j.tifs.2017.08.007
Eljounaidi, K., Lee, S. K., Bae, H. (2016). Bacterial endophytes as potential biocontrol agents of vascular wilt diseases – Review and future prospects. Biol. Control 103, 62–68. doi: 10.1016/j.biocontrol.2016.07.013
Fan, X., Song, Y. (2020). Advanced oxidation process as a postharvest decontamination technology to improve microbial safety of fresh produce. J. Agric. Food Chem. doi: 10.1021/acs.jafc.0c01381
Feng, J., Wang, D., Shao, C., Zhang, L., Tang, X. (2018). Effects of cold plasma treatment on a seed growth under simulated drought stress. Plasma Sci. Technol. 20, 035505. doi: 10.1088/2058-6272/aa9b27
Filatova, I. I., Azharonok, V., Lushkevich, V., Zhukovsky, A., Gadzhieva, G., Spaisić, K., et al. (2013). “Plasma seeds treatment as a promising technique for seed germination improvement,” in: Proceedings of the 31st International Conference on Phenomena in Ionized Gases, (Granada, Spain: ICPIG (International Conference on Phenomena in Ionized Gases)). pp. 4–7.
Filatova, I. I., Azharonok, V. V., Goncharik, S. V., Lushkevich, V. A., Zhukovsky, A. G., Gadzhieva, G. I. (2014). Effect of RF plasma treatment on the germination and phytosanitary state of seeds. J. Appl. Spectr. 81, 250–256. doi: 10.1007/s10812-014-9918-5
Gill, S. S., Tuteja, N. (2010). Reactive oxygen species and antioxidant machinery in abiotic stress tolerance in crop plants. Plant Physiol. Biochem. 48, 909–930. doi: 10.1016/j.plaphy.2010.08.016
Goswami, R. S., Kistler, H. C. (2004). Heading for disaster: Fusarium graminearum on cereal crops. Mol. Plant Pathol. 5, 515–525. doi: 10.1111/J.1364-3703.2004.00252.X
Guo, Q., Wang, Y., Zhang, H., Qu, G., Wang, T., Sun, Q., et al. (2017). Alleviation of adverse effects of drought stress on wheat seed germination using atmospheric dielectric barrier discharge plasma treatment. Sci. Rep. 7, 16680. doi: 10.1038/s41598-017-16944-8
Guo, Q., Meng, Y., Qu, G., Wang, T., Yang, F., Liang, D., et al. (2018). Improvement of wheat seed vitality by dielectric barrier discharge plasma treatment. Bioelectromagnetics 39, 120–131. doi: 10.1002/bem.22088
Henselová, M., Slováková, Ľ., Martinka, M., Zahoranová, A. (2012). Growth, anatomy and enzyme activity changes in maize roots induced by treatment of seeds with low-temperature plasma. Biologia 67, 490–497. doi: 10.2478/s11756-012-0046-5
Iranbakhsh, A., Ghoranneviss, M., Ardebili, Z. O., Ardebili, N. O., Tackallou, S. H., Nikmaram, H. (2017). Nonthermal plasma modified growth and physiology in Triticum aestivum via generated signaling molecules and UV radiation. Biol. Plant 61, 702–708. doi: 10.1007/s10535-016-0699-y
Iranbakhsh, A., Ardebili, N. O., Ardebili, Z. O., Shafaati, M., Ghoranneviss, M. (2018). Nonthermal plasma induced expression of heat shock factor A4A and improved wheat (Triticum aestivum L.) growth and resistance against salt stress. Plasma Chem. Plasma Process 38, 29–44. doi: 10.1007/s11090-017-9861-3
Ito, M., Oh, J. S., Ohta, T., Shiratani, M., Hori, M. (2018). Current status and future projects of agricultural applications using atmospheric-pressure plasma technologies. Plasma Process Polym. 15, e1700073. doi: 10.1002/ppap.201700073
Ji, S. H., Kim, T., Panngom, K., Hong, Y. J., Pengkit, A., Park, D. H., et al. (2015). Assessment of the effects of nitrogen plasma and plasma-generated nitric oxide on early development of coriandum sativum. Plasma Process Polym. 12, 1164–1173. doi: 10.1002/ppap.201500021
Ji, S. H., Choi, K. H., Pengkit, A., Im, J. S., Kim, J. S., Kim, Y. H., et al. (2016). Effects of high voltage nanosecond pulsed plasma and micro DBD plasma on seed germination, growth development and physiological activities in spinach. Arch. Biochem. Biophys. 605, 117–128. doi: 10.1016/j.abb.2016.02.028
Jiang, J., He, X., Li, L., Li, J., Shao, H., Xu, Q., et al. (2014a). Effect of cold plasma treatment on seed germination and growth of wheat. Plasma Sci. Technol. 16, 54–58. doi: 10.1088/1009-0630/16/1/12
Jiang, J., Lu, Y., Li, J., Li, L., He, X., Shao, H., et al. (2014b). Effect of seed treatment by cold plasma on the resistance of tomato to Ralstonia solanacearum (bacterial wilt). PloS One 9, e97753. doi: 10.1371/journal.pone.0097753
Jisha, K. C., Vijayakumari, K., Puthur, J. T. (2013). Seed priming for abiotic stress tolerance: an overview. Acta Physiol. Plant 35, 1381–1396. doi: 10.1007/s11738-012-1186-5
Jo, Y. K., Cho, J., Tsai, T. C., Staack, D., Kang, M. H., Roh, J. H., et al. (2014). A nonthermal plasma seed treatment method for management of a seedborne fungal pathogen on rice seed. Crop Sci. 54, 796–803. doi: 10.2135/cropsci2013.05.0331
Kang, M. H., Pengkit, A., Choi, K., Jeon, S. S., Choi, H. W., Shin, D. B., et al. (2015). Differential inactivation of Fungal spores in water and on seeds by ozone and arc discharge plasma. PloS One 10, e0139263. doi: 10.1371/journal.pone.0139263
Khamsen, N., Onwimol, D., Teerakawanich, N., Dechanupaprittha, S., Kanokbannakorn, W., Hongesombut, K., et al. (2016). Rice (Oryza sativa L.) seed sterilization and germination enhancement via atmospheric hybrid nonthermal discharge plasma. ACS Appl. Mater. Interfaces 8, 19268–19275. doi: 10.1021/acsami.6b04555
Khan, M. R., Fischer, S., Egan, D., Doohan, F. M. (2006). Biological control of Fusarium seedling blight disease of wheat and barley. Phytopathology 96, 386–394. doi: 10.1094/PHYTO-96-0386
Kimura, E., Islam, M. A. (2012). Seed scarification methods and their use in forage legumes. Res. J. Seed Sci. 5, 38–50. doi: 10.3923/rjss.2012.38.50
Klich, M. A. (2007). Aspergillus flavus: the major producer of aflatoxin. Mol. Plant Pathol. 8, 713–722. doi: 10.1111/J.1364-3703.2007.00436.X
Kumar, V., Basu, M. S., Rajendran, T. P. (2008). Mycotoxin research and mycoflora in some commercially important agricultural commodities. Crop Prot. 27, 891–905. doi: 10.1016/j.cropro.2007.12.011
Laroussi, M., Leipold, F. (2004). Evaluation of the roles of reactive species, heat, and UV radiation in the inactivation of bacterial cells by air plasmas at atmospheric pressure. Int. J. Mass Spectrom. 233, 81–86. doi: 10.1016/j.ijms.2003.11.016
Lee, E. J., Khan, M. S. I., Shim, J., Kim, Y. J. (2019). Roles of oxides of nitrogen on quality enhancement of soybean sprout during hydroponic production using plasma discharged water recycling technology. Sci. Rep. 8, 16872. doi: 10.1038/s41598-018-35385-5
Li, L., Jiang, J., Li, J., Shen, M., He, X., Shao, H., et al. (2014). Effects of cold plasma treatment on seed germination and seedling growth of soybean. Sci. Rep. 4, 5859–5865. doi: 10.1038/srep05859
Li, L., Li, J., Shen, M., Zhang, C., Dong, Y. (2015). Cold plasma treatment enhances oilseed rape seed germination under drought stress. Sci. Rep. 5, 13033. doi: 10.1038/srep13033
Li, Y., Wang, T., Meng, Y., Qu, G., Sun, Q., Liang, D., et al. (2017). Air atmospheric dielectric barrier discharge plasma induced germination and growth enhancement of wheat seed. Plasma Chem. Plasma Process 37, 1621–1634. doi: 10.1007/s11090-017-9835-5
Ma, F., Cholewa, E., Mohamed, T., Peterson, C. A., Gijzen, M. (2004). Cracks in the palisade cuticle of soybean seed coats correlate with their permeability to water. Ann. Bot. 94, 213–228. doi: 10.1093/aob/mch133
Misra, N. N., Tiwari, B. K., Raghavarao, K. S. M. S., Cullen, P. J. (2011). Nonthermal plasma inactivation of food-borne pathogens. Food Eng. Rev. 3, 159–170. doi: 10.1007/s12393-011-9041-9
Mitra, A., Li, Y. F., Klämpfl, T. G., Shimizu, T., Jeon, J., Morfill, G. E., et al. (2014). Inactivation of surface-borne microorganisms and increased germination of seed specimen by cold atmospheric plasma. Food Bioprocess Technol. 7, 645–653. doi: 10.1007/s11947-013-1126-4
Mittler, R., Vanderauwera, S., Gollery, M., Van Breusegem, F. (2004). Reactive oxygen gene network of plants. Trends Plant Sci. 9, 490–498. doi: 10.1016/j.tplants.2004.08.009
Mittler, R. (2002). Oxidative stress, antioxidants, and stress tolerance. Trends Plant Sci. 7, 405–410. doi: 10.1016/S1360-1385(02)02312-9
Moïse, J. A., Han, S., Gudynaite-Savitch, L., Johnson, D. A., Miki, B. L. A. (2005). Seed coats: structure, development, composition, and biotechnology. Vitro Cell. Dev. Biol. Plant 41, 620–644. doi: 10.1079/IVP2005686
Noodén, L. D., Blakley, K. A., Grzybowski, J. M. (1985). Control of seed coat thickness and permeability in soybean: a possible adaptation to stress. Plant Physiol. 79, 543–545. doi: 10.1104/pp.79.2.543
Ochi, A., Konishi, H., Ando, S., Sato, K., Yokoyama, K., Tsushima, S., et al. (2017). Management of bakanae and bacterial seedling blight diseases in nurseries by irradiating rice seeds with atmospheric plasma. Plant Pathol. 66, 67–76. doi: 10.1111/ppa.12555
Ono, R., Uchida, S., Hayashi, N., Kosaka, R., Soeda, Y. (2017). Inactivation of bacteria on plant seed surface by low-pressure RF plasma using a vibrating stirring device. Vacuum 136, 214–220. doi: 10.1016/j.vacuum.2016.07.017
Palencia, E. R., Hinton, D. M., Bacon, C. W. (2010). The black Aspergillus species of maize and peanuts and their potential for mycotoxin production. Toxins 2, 399–416. doi: 10.3390/toxins2040399
Park, Y., Oh, K. S., Oh, J., Seok, D. C., Kim, S. B., Yoo, S. J., et al. (2018). The biological effects of surface dielectric barrier discharge on seed germination and plant growth with barley. Plasma Process Polym. 15, e1600056. doi: 10.1002/ppap.201600056
Parry, D. W., Jenkinson, P., McLeod, L. (1995). Fusarium ear blight (scab) in small grain cereals – a review. Plant Pathol. 44, 207–238. doi: 10.1111/j.1365-3059.1995.tb02773.x
Randeniya, L. K., de Groot, G. J. J. B. (2015). Nonthermal plasma treatment of agricultural seeds for stimulation of germination, removal of surface contamination and other benefits: A review. Plasma Process Polym. 12, 608–623. doi: 10.1002/ppap.201500042
Roy, N. C., Hasan, M. M., Talukder, M. R., Hossain, M. D., Chowdhury, A. N. (2018). Prospective applications of low frequency glow discharge plasmas on enhanced germination, growth and yield of wheat. Plasma Chem. Plasma Process 38, 13–28. doi: 10.1007/s11090-017-9855-1
Sadhu, S., Thirumdas, R., Deshmukh, R. R., Annapure, U. S. (2017). Influence of cold plasma on the enzymatic activity in germinating mung beans (Vigna radiate). LWT-Food Sci. Technol. 78, 97–104. doi: 10.1016/j.lwt.2016.12.026
Scherm, B., Balmas, V., Spanu, F., Pani, G., Delogu, G., Pasquali, M., et al. (2013). Fusarium culmorum: causal agent of foot and root rot and head blight on wheat. Mol. Plant Pathol. 14, 323–341. doi: 10.1111/mpp.12011
Selcuk, M., Oksuz, L., Basaran, P. (2008). Decontamination of grains and legumes infected with Aspergillus spp. and Penicillum spp. by cold plasma treatment. Biores. Technol. 99, 5104–5109. doi: 10.1016/j.biortech.2007.09.076
Selwyn, G. S., Herrmann, H. W., Park, J., Henins, I. (2001). Materials processing using an atmospheric pressure, RF-generated plasma source. Contrib. Plasma Phys. 6, 610–619. doi: 10.1002/1521-3986(200111)41:6<610::AID-CTPP610>3.0.CO;2-L
Šerá, B., Špatenka, P., Šerý, M., Vrchotová, N., Hrušková, I. (2010). Influence of plasma treatment on wheat and oat germination and early growth. IEEE Trans. Plasma Sci. 38, 2963–2968. doi: 10.1109/TPS.2010.2060728
Shao, S., Meyer, C. J., Ma, F., Peterson, C. A., Bernards, M. A. (2007). The outermost cuticle of soybean seeds: chemical composition and function during imbibition. J. Exp. Bot. 58, 1071–1082. doi: 10.1093/jxb/erl268
Shao, C. Y., Wang, D., Tang, X., Zhao, L., Li, Y. (2013). Stimulating effects of magnetized arc plasma of different intensities on the germination of old spinach seeds. Math. Comput. Model. 58, 814–818. doi: 10.1016/j.mcm.2012.12.022
Sharma, P., Jha, A. B., Dubey, R. S., Pessarakli, M. (2012). Reactive oxygen species, oxidative damage, and antioxidative defense mechanism in plants under stressful conditions. J. Bot. 217037. doi: 10.1155/2012/217037
Sharma, K. K., Singh, U. S., Sharma, P., Kumar, A., Sharma, L. (2015). Seed treatments for sustainable agriculture- A review. J. Appl. Nat. Sci. 7, 521–539. doi: 10.31018/jans.v7i1.641
Song, J. S., Lee, M. J., Ra, J. E., Lee, K. S., Eom, S., Ham, H. M., et al. (2020). Growth and bioactive phytochemicals in barley (Hordeum vulgare L.) sprouts affected by atmospheric pressure plasma during seed germination. J. Phys. D.: Appl. Phys. 53, 314002. doi: 10.1088/1361-6463/ab810d
Stolárik, T., Henselová, M., Martinka, M., Novák, O., Zahoranová, A., Cernák, M. (2015). Effect of low-temperature plasma on the structure of seeds, growth, and metabolism of endogenous phytohormones in pea (Pisum sativum L.). Plasma Chem. Plasma Process 35, 659–676. doi: 10.1007/s11090-015-9627-8
Thomas, T. T. D., Puthur, J. T. (2017). UV radiation priming: a means of amplifying the inherent potential for abiotic stress tolerance in crop plants. Environ. Exp. Bot. 138, 57–66. doi: 10.1016/j.envexpbot.2017.03.003
Thomma, B. P. H. J. (2003). Alternaria spp.: from general saprophyte to specific parasite. Mol. Plant Pathol. 4, 225–236. doi: 10.1046/j.1364-3703.2003.00173.x|
Tong, J., He, R., Zhang, X., Zhan, R., Chen, W., Yang, S. (2014). Effects of atmospheric pressure air plasma pretreatment on the seed germination and early growth of Andrographis paniculata. Plasma Sci. Technol. 16, 260–266. doi: 10.1088/1009-0630/16/3/16
Tu, J. C. (1985). Pink pod rot of bean caused by Trichothecium roseum. Can. J. Plant Pathol. 7, 55–57. doi: 10.1080/07060668509501515
Volin, J. C., Ferencz, S. D., Raymond, A. Y., Park, S. M. T. (2000). Modification of seed germination performance through cold plasma chemistry technology. Crop Sci. 40, 1706–1718. doi: 10.2135/cropsci2000.4061706x
Wang, X. Q., Zhou, R. W., de Groot, G., Bazaka, K., Murphy, A. B., Ostrikov, K. K. (2017). Spectral characteristics of cotton seeds treated by a dielectric barrier discharge plasma. Sci. Rep. 7, 5601. doi: 10.1038/s41598-017-04963-4
Yaklich, R. W., Vigil, E. L., Wergin, W. P. (1986). Pore development and seed coat permeability in soybean. Crop Sci. 26, 616–624. doi: 10.2135/cropsci1986.0011183X002600030041x
Yin, M., Huang, M., Ma, B., Ma, T. (2005). Stimulating effects of seed treatment by magnetized plasma on tomato growth and yield. Plasma Sci. Technol. 7, 3143–3147. doi: 10.1088/1009-0630/7/6/017
Zahoranová, A., Henselova´, M., Hudecova´, D., Kalinˇa´kova´, B., Kova´cˇik, D., Medvecka´, V., et al. (2016). Effect of cold atmospheric pressure plasma on the wheat seedlings vigor and on the inactivation of microorganisms on the seeds surface. Plasma Chem. Plasma Process 36, 397–414. doi: 10.1007/s11090-015-9684-z
Zhang, J. J., Jo, J. O., Huynh, D. L., Mongre, R. K., Ghosh, M., Singh, A. K., et al. (2017). Growth-inducing effects of argon plasma on soybean sprouts via the regulation of demethylation levels of energy metabolism-related genes. Sci. Rep. 7, 41917. doi: 10.1038/srep41917
Zhou, S., Sekizaki, H., Yang, Z., Sawa, S., Pan, J. (2010). Phenolics in the seed coat of wild soybean (Glycine soja) and their significance for seed hardness and seed germination. J. Agric. Food Chem. 58, 10972–10978. doi: 10.1021/jf102694k
Keywords: atmospheric pressure plasma, seed germination, seedling growth, microorganism inactivation, antioxidant system, stress
Citation: Song J-S, Kim SB, Ryu S, Oh J and Kim D-S (2020) Emerging Plasma Technology That Alleviates Crop Stress During the Early Growth Stages of Plants: A Review. Front. Plant Sci. 11:988. doi: 10.3389/fpls.2020.00988
Received: 24 March 2020; Accepted: 17 June 2020;
Published: 15 July 2020.
Edited by:
Bernard Grodzinski, University of Guelph, CanadaCopyright © 2020 Song, Kim, Ryu, Oh and Kim. This is an open-access article distributed under the terms of the Creative Commons Attribution License (CC BY). The use, distribution or reproduction in other forums is permitted, provided the original author(s) and the copyright owner(s) are credited and that the original publication in this journal is cited, in accordance with accepted academic practice. No use, distribution or reproduction is permitted which does not comply with these terms.
*Correspondence: Seong Bong Kim, c2JraW1AbmZyaS5yZS5rcg==
†ORCID: Jong-Seok Song, orcid.org/0000-0002-1900-7806
Seong Bong Kim, orcid.org/0000-0002-0472-8694
Do-Soon Kim, orcid.org/0000-0002-7388-4308
Disclaimer: All claims expressed in this article are solely those of the authors and do not necessarily represent those of their affiliated organizations, or those of the publisher, the editors and the reviewers. Any product that may be evaluated in this article or claim that may be made by its manufacturer is not guaranteed or endorsed by the publisher.
Research integrity at Frontiers
Learn more about the work of our research integrity team to safeguard the quality of each article we publish.