- 1Key Laboratory of Plant Resources Conservation and Sustainable Utilization, South China Botanical Garden, Chinese Academy of Sciences, Guangzhou, China
- 2Department of Botany, National Museum of Natural History, MRC 166, Smithsonian Institution, Washington, DC, United States
- 3College of Life Science, Northwest A&F University, Yangling, China
- 4Germplasm Bank of Wild Species in Southwest China, Kunming Institution of Botany, Chinese Academy of Sciences, Kunming, China
- 5Department of Biology, Faculty of Science, Selçuk University, Konya, Turkey
- 6Department of Botany and HNU, Faculty of Biology, VNU Hanoi University of Science (HUS), Hanoi, Vietnam
- 7Laboratory of Natural Products Chemistry, College of Pharmaceutical Sciences, Ritsumeikan University, Kyoto, Japan
The liquorice genus, Glycyrrhiza L. (Leguminosae), is a medicinal herb with great economic importance and an intriguing intercontinental disjunct distribution in Eurasia, North Africa, the Americas, and Australia. Glycyrrhiza, along with Glycyrrhizopsis Boiss. and Meristotropis Fisch. & C.A.Mey., comprise Glycyrrhiza s.l. Here we reconstructed the phylogenetic relationships and biogeographic history in Glycyrrhiza s.l. using sequence data of whole chloroplast genomes. We found that Glycyrrhiza s.l. is sister to the tribe Wisterieae and is divided into four main clades. Clade I, corresponds to Glycyrrhizopsis and is sister to Glycyrrhiza sensu Meng. Meristotropis is embedded within Glycyrrhiza sensu Meng, and these two genera together form Clades II–IV. Based on biogeographic analyses and divergence time dating, Glycyrrhiza s.l. originated during the late Eocene and its most recent common ancestor (MRCA) was distributed in the interior of Eurasia and the circum-Mediterranean region. A vicariance event, which was possibly a response to the uplifting of the Turkish-Iranian Plateau, may have driven the divergence between Glycyrrhiza sensu Meng and Glycyrrhizopsis in the Middle Miocene. The third and fourth main uplift events of the Qinghai-Tibetan Plateau may have led to rapid evolutionary diversification within Glycyrrhiza sensu Meng. Subsequently, the MRCA of Clade II might have migrated to North America (G. lepidota) via the Bering land bridge during the early Pliocene, and reached temperate South America (G. astragalina) by long-distance dispersal (LDD). Within Clade III, the ancestor of G. acanthocarpa arrived at southern Australia through LDD after the late Pliocene, whereas all other species (the SPEY clade) migrated to the interior of Eurasia and the Mediterranean region in the early Pleistocene. The MRCA of Clade IV was restricted in the interior of Eurasia, but its descendants have become widespread in temperate regions of the Old World Northern Hemisphere during the last million years.
Introduction
The papilionoid legume genus Glycyrrhiza L. is commonly known as the liquorice genus (Polhill, 1981, 1994; LPWG, 2017). Glycyrrhiza is perennial herb adapting to mesophytic and xerophytic habitats; its flowering phase ranges from June to August (Li and Cui, 1998), and the seeds disperse by the spiny/hairy pods sticking to animal fur (Zhou and Jin, 2016). The liquorice genus is well-known by its morphologically diverse fruits (ovoid, oblong, or linear, rarely moniliform, according to Bao and Larsen, 2010), and long, strong roots with great economic importance. Traditionally, the roots have been widely used as an important medicinal herb in the Old World countries ranging from China and Japan to Turkey, Greece and Egypt, with the efficacy of relieving cough and phlegm (Bao and Larsen, 2010; Chinese Pharmacopoeia Commission, 2015; Dastagir and Rizvi, 2016; Zhou and Jin, 2016; Sharma et al., 2018) and have been used to make a popular candy in the West since at least the sixteenth-century (Richardson, 2008). The unique constituent of Glycyrrhiza, glycyrrhizin, is broadly used as a natural sweetener and a pharmaceutical agent because of its anti-inflammatory and hepatoprotective properties (Hayashi and Sudo, 2009) and extracts of Glycyrrhiza can also be used in the production of cosmetics, food additives, and tobacco flavors (Li and Cui, 1998; Zhou and Jin, 2016).
Despite its large economic and cultural importance, generic and species delimitation in Glycyrrhiza and related genera has had a torturous taxonomic history with the number of species varying from 13 (Kruganova, 1955; Meng, 2005) to 36 (Grankina, 2008). As for its two satellite genera, Glycyrrhizopsis Boiss. and Meristotropis Fisch. & C.A.Mey., Meng (2005) first merged the latter into Glycyrrhiza but retained the generic status of the former (referred to as “Glycyrrhiza sensu Meng” hereafter). Later, taxonomists treated both Meristotropis and Glycyrrhizopsis within Glycyrrhiza (Li, 1963; Li and Lu, 2015; Çetin, 2015), which formed “Glycyrrhiza s.l.” Previous molecular phylogenetic studies supported that Glycyrrhiza s.l. was an early branching clade of the inverted repeat-lacking clade (IRLC) of Papilionoideae (Wojciechowski et al., 2000, 2004; Lavin et al., 2005; Duan et al., 2015). With regard to the infra-generic phylogeny of Glycyrrhiza s.l., Yamazaki et al. (1994) resolved that G. glabra L. and G. uralensis Fisch. ex DC. are sisters according to RAPD and RFLP markers. Similarly, based on the chloroplast rbcL marker, two groups were recovered within Glycyrrhiza: one group included G. glabra, G. uralensis and G. inflata Batalin and the other contained G. echinata L. and G. pallidifolia Maxim. (Hayashi et al., 1998, 2000; Hu and Chang, 2003). Hayashi et al. (2005) and Meng (2005) discovered that G. lepidota Pursh diverged first in the genus, while other studies suggested G. flavescens Boiss. was sister to the rest of Glycyrrhiza s.l. (Erayman et al., 2014; Çetin, 2015; Altay et al., 2016). However, none of these prior studies showed Glycyrrhiza to be monophyletic, and all suffered from under-sampling (i.e., a maximum of seven species) and, therefore, did not represent strong molecular evidence. Thus, the infra-generic phylogeny of Glycyrrhiza and its relationship to Glycyrrhizopsis and Meristotropis require further investigation.
Glycyrrhiza has a widespread distribution, in every continent except for Antarctica (Kruganova, 1955; Lock and Schrire, 2005; Meng, 2005 also see the map in Figure 3), but it is widely distributed in north temperate zones globally, with only sparse distribution in the south temperate zone. On the other hand, most of the species of Glycyrrhiza are in the Old World, covering a vast area from the western Mediterranean region (Iberian peninsula in Europe and Algeria in North Africa), through the Russian Far East, Mongolia and northern China (as well as Sichuan and Yunnan of southwestern China) (Yeo, 1968; Chamberlain, 1970; Townsend, 1974; Ali, 1977; Rechinger, 1984; Lock, 1989; Podlech, 1991; Yakovlev, 1996; García, 1999; Bao and Larsen, 2010) to southern Australia (only G. acanthocarpa J.M.Black), including the states of Queensland, South New Wales, Victoria, South Australia, and Western Australia (Stanley and Ross, 1983; Weber, 1986; Gardner, 1991; Jeanes, 1996; Western Australian Herbarium, 1998). Only two species naturally occur in the New World: Glycyrrhiza lepidota in western temperate Canada and the United States (Britton and Brown, 1897; Scoggan, 1978) and G. astragalina Gillies in the temperate region of Argentina and Chile (ca. 40°S; Reiche, 1898; Gómer-Sosa, 1999). In contrast, the genera Glycyrrhizopsis and Meristotropis are restricted in southern Anatolia of Turkey (Chamberlain, 1970; Çetin, 2015) and Central Asia (Kruganova, 1955; Meng, 2005), respectively.
Notably, there are three classic types of intercontinental disjunction patterns represented by the distributional area of Glycyrrhiza: Eurasian-North American disjunction (i.e., north temperate disjunction, sensu Raven, 1972) North-South American amphitropical disjunction (trans-tropical disjunction, sensu Raven, 1972) and Asian-Australian disjunction (Southern Hemisphere temperate disjunction, sensu Kruganova, 1955; Raven, 1972; Meng, 2005). Compared to the other two types, the pattern of Asian-Australian disjunction is relatively unusual among angiosperm genera (for some examples see Gussarova et al., 2008; Nie et al., 2012; Temirbayeva and Zhang, 2015; Kramina et al., 2016; Slovák et al., 2018). On the other hand, many intercontinental diusjunctions comprise trans-tropical disjunctions sensu Raven (1972) or north and south temperate pattern sensu Thorne (1972). Wu et al. (2003, 2006) speculated that Glycyrrhiza may have originated in the Tethys region, and dispersed to eastern Gondwana, suggesting a fairly ancient series of events. In contrast, more recent inferences in other lineages (Apodanthaceae see Bellot and Renner, 2014; Nitraria see Temirbayeva and Zhang, 2015) based on molecular dating have shown much younger ages for similar patterns of disjunction (e.g., as predicted by Raven, 1972) compared to those presumed by Wu et al. (2006). Thus, the historical biogeography and the causes of the intercontinental disjunction of Glycyrrhiza needs further investigation using current analytical tools, and the subsequent findings may yield a greater understanding of evolutionary and biogeographic processes in other, similarly, widely distributed plant taxa.
In the present study, we inferred the phylogeny of Glycyrrhiza s.l. using dense taxonomic sampling and molecular sequence data from whole chloroplast (cp) genomes to (1) reveal the infra-generic relationships of Glycyrrhiza and test the phylogenetic positions of Glycyrrhizopsis and Meristotropis and (2) estimate the divergence times of lineages and reconstruct their biogeographic history. This study will provide a robust phylogenetic framework for resolving taxonomic problems in Glycyrrhiza as well as for sustainably using and developing consumable products from the genus.
Materials and Methods
Taxon Sampling
Sampling for molecular phylogenetic analyses consisted of 58 accessions representing 22 species of Glycyrrhiza s.l., including all the species, except for the rare Glycyrrhizopsis syriaca Turrill, accepted by Meng (2005) which is considered as the most thorough revision of Glycyrrhiza s.l. Outside of Glycyrrhiza s.l., within the IRLC clade, we sampled eight species representing five genera within tribe Wisterieae sensu Compton et al. (2019), and six species representing six other genera of the IRLC as in LPWG (2013). Four species representing three genera for the robinioids clade (sensu Wojciechowski et al., 2004) were sampled. We also included Hylodesmum podocarpum (Candolle) H.Ohashi & R.R.Mill as the outgroup. Most sequences for the study were obtained from field-collected or herbarium specimens and were new to this study (72 accessions, 38 species; see Supplementary Table S1 for detalis). DNA samples of Austrocallerya megasperma (F.Muell.) J.Compton & Schrire, Glycyrrhiza astragalina – 1 and Wisteria floribunda (Willd.) DC. were obtained from the DNA and Tissue Bank, Kew1. In addition, we downloaded sequences of Lotus japonicus (Regel) K.Larsen and Robinia pseudoacacia L. – 2 from GenBank (see Supplementary Table S1 for details).
DNA Extraction, Genome Assembly, Annotation, and Alignment
We extracted the total genomic DNA following a modified CTAB protocol (Doyle and Doyle, 1987). Yield and integrity (size distribution) of genomic DNA extracts were quantified by fluorometric quantification on a Qubit (Invitrogen, Carlsbad, California, United States) using a dsDNA HS kit, as well as by visual assessment on 1% agarose gels. Subsequently, we used all samples to build blunt-end DNA libraries using the NEBNext Ultra II DNA library Prep kit for Illumina (New England Bio-labs) following the protocol of the manufacturer. We pooled the final indexed libraries in equimolar ratios and sequenced them in a single lane of an Illumina XTen sequencing system (Illumina Inc.).
From the raw reads, we filtered out adaptors and low-quality reads in Trimmomatic v.0.33 (Bolger et al., 2014). We checked the quality of the remaining reads using FastQC2 and performed de novo assembly in SPAdes 3.11 (Bankevich et al., 2012) with the k-mer of 75, 85, 95, and 105. We employed a customized python script (Jin et al., 2018) with its default parameters to apply BLAST and a built-in library to connect verified contigs into plastomes in SPAdes. We annotated the assembly of the resulting complete chloroplast (cp) genomes using the Dual Organellar GenoMe Annotator (DOGMA) (Wyman et al., 2004) with Glycyrrhiza glabra (GenBank Accession #: NC_024038; Sabir et al., 2014) as a reference. Most of our samples belonged to the IRLC (Wojciechowski et al., 2004) which lack the inverted repeat (IR) regions (Lavin et al., 1990). Thus, to better align with the cp genomes of Glycyrrhiza s.l., IR regions of Hylodesmum podocarpum, Lotus japonicus, Robinia pseudoacacia, and Sesbania cannabina (Retz.) Poir. were removed. We also extracted 75 protein coding sequences (CDSs; as in Kang et al., 2018 except for rps12) from the annotated genome and concatenated them with Geneious Prime (Kearse et al., 2012).
Phylogenetic Analyses
We aligned whole cp genomes and CDSs independently with MAFFT v.7 (Katoh and Standley, 2013). We partitioned the alignment of CDSs but regarded the whole cp genomes without partitioning because intergenic spacers cannot be reliably modeled independently and based on many other recent studies (Wei et al., 2017; Wen et al., 2018; Yang et al., 2019). The best nucleotide substitution models of the whole cp genome and each CDS were detected using PartitionFinder 2 (Lanfear et al., 2016) under the default settings. GTR+G was selected as the best model for the whole cp genome and the best model for each CDS are shown in Supplementary Table S2.
We carried out separate phylogenetic analyses based on the whole cp genomes and the combined CDSs using Bayesian inference (BI; Rannala and Yang, 1996; Mau et al., 1999) implemented in the program MrBayes 3.2.5 (Ronquist and Huelsenbeck, 2003; Ronquist et al., 2012) by applying default prior settings. Each BI was performed using two independent runs of the Markov chain Monte Carlo (MCMC) for 10 million generations with sampling every 1,000 generations. We discarded the first 2,500 trees as burn-in and summarized the remaining posterior topologies as a maximum clade credibility (MCC) tree. We verified stationarity of the analyses with Tracer v1.63 by ensuring that all ESS values exceeded 200 and we confirmed convergence between independent runs. In addition to BI, we also performed maximum likelihood (ML) analyses using IQ-TREE v.1.6 (Nguyen et al., 2015) with the following settings: rapid bootstrap analysis with 1,000 replicates followed by a search for best-scoring ML tree starting with a random seed. We observed that both the BI and ML trees generated from the whole cp genomes had identical topologies but higher support values than those from CDSs (see Supplementary Trees 1, 2; see additional reasons in Caption and Notes in Supplementary File). Therefore, we used the whole cp genomes in subsequent phylogenetic and biogeographic analyses.
Divergence Time Estimation
We performed divergence time dating with a lognormal relaxed clock model in BEAST v.2.6.1 (Bouckaert et al., 2019) based on an alignment with no more than three accessions per species within Glycyrrhiza s.l. (see Alignment 2 and Caption and Notes in the Supplementary File). We used default parameters except that we applied the birth-death model for tree branching processes, the nucleotide substitution model of GTR+G based on PartitionFinder 2, and constrained the ages of two nodes. We constrained the root age at 50.6 Ma based on the results of Lavin et al. (2005). In addition, and set the stem age of Wisteria Nutt. according to the oldest reliable fossil with both fruits and leaves described by Wang et al. (2006). The fossil, Wisteria shanwangensis Wang, Dilcher, Zhu, Zhou et Lott, occurs in the Middle Miocene Shanwang flora, China, which has been recently dated to ca. 17 Ma on the basis of 40Ar/39Ar analysis (He et al., 2011). The prior distributions of the both calibration points were set as log normal, with a standard deviation of 1.0. Throughout, we follow the geological time scale according to the chronostratigraphic chart of International Commission on Stratigraphy (ICS)4 for the names of geological units and their time durations.
The parameters of BEAST were set using BEAUti v.2.5.0 (Bouckaert et al., 2019) with the abovementioned fossil calibrated nodes constrained as monophyletic according to Wojciechowski (2003) and Wojciechowski et al. (2004), and our present phylogenetic results. In BEAST, we performed the MCMC run for 700 million generations with sampling every 1,000 generations. Checking with the program of Tracer, the analysis reached convergence with burn-in of the first 170 million states. We performed the burn-in and then determined the MCC tree with mean heights in TreeAnnotator. The MCC tree was annotated as a chronogram with mean ages for the nodes and 95% highest posterior density (HPD) intervals.
Before conducting the analysis in BEAST, we determined the effective priors by performing an analysis with priors only (i.e., without sequence data). The analysis with priors only is important for revealing the actual marginal distributions of priors, which may differ from those set for the analysis due to complex interactions with other calibrations and the tree process, i.e., birth-death model, herein (Heled and Drummond, 2012; Warnock et al., 2015; Muellner-Riehl et al., 2016). In the case of the calibration of the two crown nodes, the marginal distribution of the priors reflected our intended settings, indicating limited prior interactions (see Prior-only.log in the Supplementary Material).
Ancestral Area Reconstruction
To infer the ancestral areas of Glycyrrhiza s.l., we used the Dispersal-Extinction-Cladogenesis model (DEC; Ree and Smith, 2008) implemented within a statistical framework in RASP v.4.0 (Yu et al., 2015). According to the distribution records of Glycyrrhiza s.l. in Kruganova (1955) and Meng (2005), and Li and Lu (2015) and taking endemism into consideration (Linder, 2001), we coded the extant taxa so that species fell into one or two geographic areas (see the map in Figure 3): (A) eastern Asia: northern China (except for Inner Mongolia and Xinjiang), southwestern China (Sichuan and Yunnan) and the Russian Far East; (B) interior of Eurasia (east of Black Sea): southern Siberia, Mongolia, Inner Mongolia and Xinjiang of China, Central Asia, western Himalayan region, northern Iran, western Asia (except for Turkey and Mediterranean east coast), Caucasus and European Russia; (C) circum-Mediterranean region: southern Europe, Turkey, Mediterranean east coast and northern Africa; (D) Australia: southern Queensland, South New Wales, Victoria, eastern South Australia and southeastern Western Australia; (E) temperate western North America: regions of west of the Great Lakes in United States and Canada; (F) temperate South America: around 40°S in Argentina and Chile. To infer the ancestral areas, we obtained 300,000 post-burnin trees and the corresponding MCC tree from the abovementioned BEAST analysis. One accession per species had remained within Glycyrrhiza s.l. (details see Caption and Notes in Supplementary File) and all the other taxa were pruned with Ape library in R (Paradis and Schliep, 2018) as including sparsely sampled related lineages is ill-advised and can lead to erroneous inferences at the root (Ronquist, 1997; Harris et al., 2013). All parameters were set as default in RASP except that the maximum number of areas was constrained to two based on the maximum number of areas in which extant species occur.
Results
All 72 newly sequenced plastomes were successfully assembled into complete circular configurations. The sizes of the plastid genomes ranged from 122,421 to 156,702 bp and the GC contents were between 33.9 and 36.0%, respectively (see Supplementary Table S1 for details). The lengths of alignments for the analyses in MrBayes, IQ-TREE, and BEAST (see Alignment 1 and 2 in the Supplementary File) were 241,311 and 167,779 bp, respectively. The structure and gene order of Glycyrrhiza s.l. were identical as those reported in previous studies on several cp genomes of Glycyrrhiza (Raveendar et al., 2017; Kang et al., 2018).
Phylogenetic Relationships
We present the phylogenetic tree with Bayesian posterior probabilities (PP) and ML bootstrap (LBS) values on the BI tree branches (Figure 1; BI tree with branch lengths see Supplementary Tree 3; ML tree see Supplementary Tree 4). The phylogenetic result indicated that Glycyrrhiza s.l. was monophyletic (PP = 1, LBS = 100%), forming a clade (PP = 1, LBS = 100%) with Wisterieae (PP = 1, LBS = 100%). This large clade was sister to all other sampled IRLC.
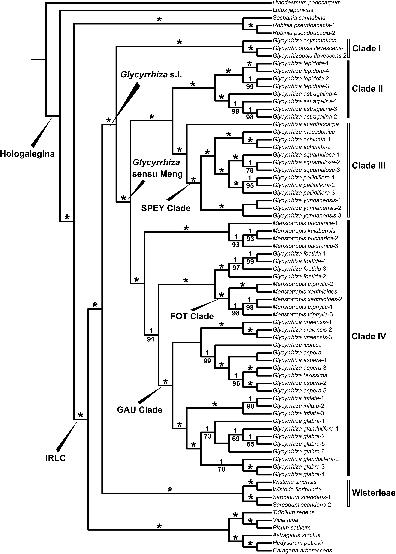
Figure 1. Maximum clade credibility tree resulting from Bayesian Inference of Glycyrrhiza s.l. based on whole chloroplast genome sequences. Bayesian posterior probabilities (PP ≥ 0.95) are given above branches, maximum likelihood bootstrap values (LBS ≥ 50%) below branches. Asterisks indicate both of PP = 1 and LBS = 100%. The Hologalegina and IRLC clades were labeled following Wojciechowski et al. (2004).
We recovered four main clades within Glycyrrhiza s.l. (clades I–IV), all of which were highly supported with PP = 1, LBS = 100%. Clade I contained Glycyrrhizopsis flavescens (PP = 1, LBS = 100%) and Glycyrrhiza asymmetrica Hub.-Mor., and was sister to all other Glycyrrhiza s.l., which correspond to Glycyrrhiza sensu Meng (Figure 1; PP = 1, LBS = 100%). Within Glycyrrhiza sensu Meng, Clade II was comprised of two well supported species, Glycyrrhiza astragalina (PP = 1, LBS = 100%) and G. lepidota (PP = 1, LBS = 100%). Within Clade III, G. acanthocarpa was sister to all other species, comprising G. echinata, G. macedonica Boiss. & Orph., G. pallidiflora Maxim., G. squamulosa Franch., and G. yunnanensis Cheng f. & L.K.Tai ex P.C.Li, which formed a clade (PP = 1, LBS = 100%) and was regarded as the “SPEY clade” hereafter. Clade IV consisted of 13 species and included a nested clade comprising Meristotropis bucharica (Regel) Kruganova and M. kulabensis Masl. (PP = 1, LBS = 100%), which were sister to the rest of Clade IV (PP = 1, LBS = 91%). The next diverging clade within Clade IV comprises G. foetida Desf., Meristotropis triphylla Fisch. & C.A.Mey, and M. xanthioides Vassilcz (PP = 1, LBS = 100%; as the “FOT clade”) and the remaining eight species constituted the “GAU clade” (PP = 1, LBS = 100%).
Divergence Times
All the PP values on our BEAST tree are 1.0, except for the Glycyrrhiza s.l.-Wisterieae clade (PP = 0.91; see Supplementary Tree 5). According to our molecular dating result (Figure 2), the ancestor of Glycyrrhiza s.l. originated at 37.02 Ma (95% HPD: 27.26–49.39 Ma) during the late Eocene, and Clade I and Glycyrrhiza sensu Meng diverged at 17.05 Ma (Node 1, 95% HPD: 7.64–27.83 Ma). The split of the ancestor of Clades II and III and that of Clade IV was dated to 5.36 Ma (Node 2, 95% HPD: 2.44–9.03 Ma) around the Miocene-Pliocene boundary, followed by the divergence of Clade II and Clade III at 3.53 Ma (Node 3, 95% HPD: 1.81–5.54 Ma). Within Clade II, the South American Glycyrrhiza astragalina and North American G. lepidota split at 2.55 Ma (Node 4, 95% HPD: 1.03–4.23 Ma). On the other hand, at around 2.82 Ma (Node 5, 95% HPD: 1.45–4.46 Ma), the most recent common ancestors (MRCA) of the Australian G. acanthocarpa separated from the SPEY clade within Clade III. The crown group of Clade IV began to diverge in the late Pliocene (Node 6, 3.26 Ma, 95% HPD: 1.36–5.58 Ma). Subsequently, the FOT clade and the GAU clade diverged at 2.83 Ma (Node 7, 95% HPD: 1.11–4.88 Ma). More recently, the FOT clade and the GAU clade started to split at 0.67 Ma (Node 8, 95% HPD: 0.19–1.34 Ma) and 0.93 Ma (Node 9, 95% HPD: 0.42–1.61 Ma), respectively.
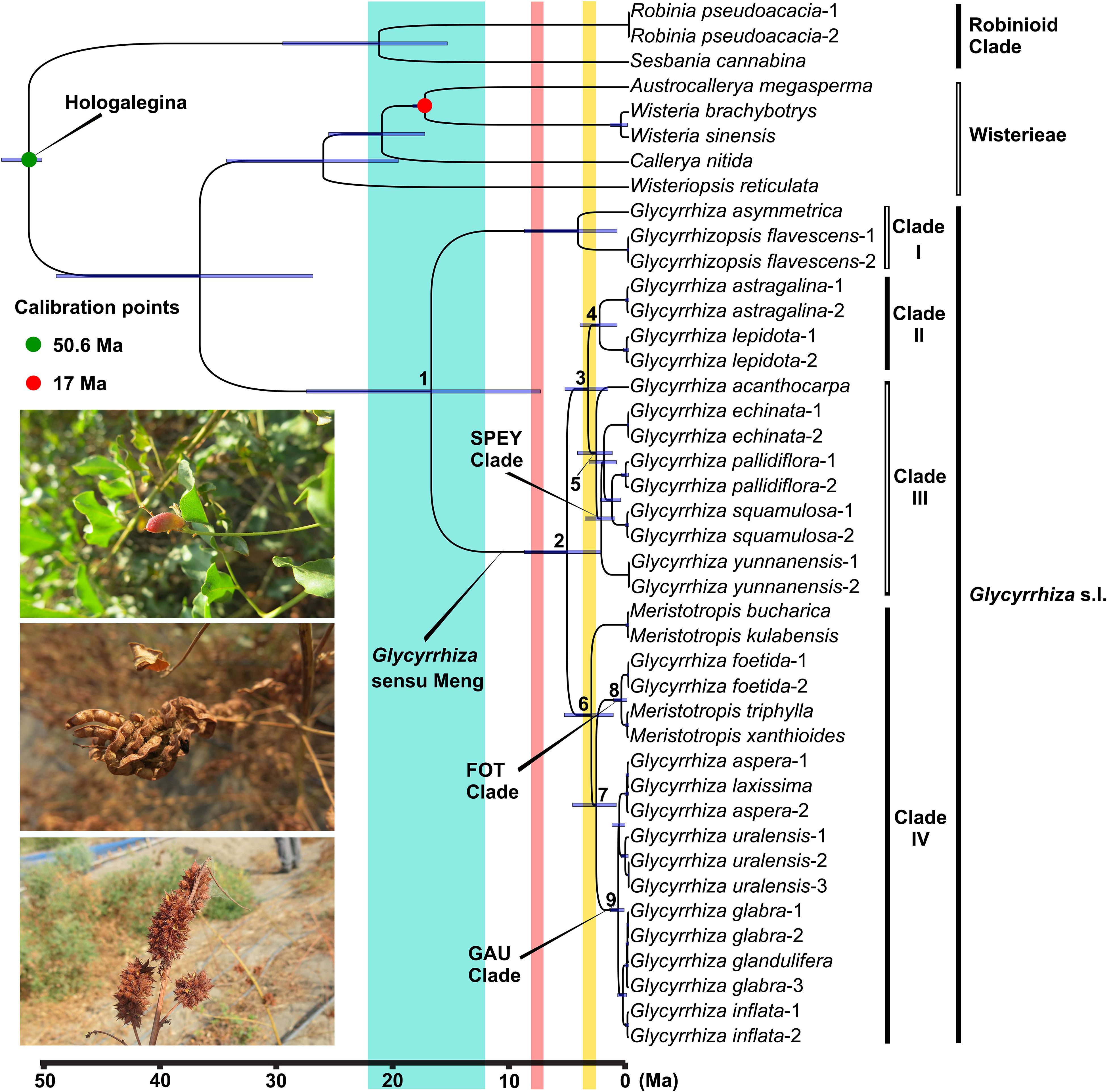
Figure 2. Ultrametric chronogram from BEAST of Glycyrrhiza s.l. and related groups based on chloroplast genome data. Blue bars represent the 95% highest posterior density credibility interval for node ages. Three paleogeological events are indicated with colored vertical rectangles: uplift of the Turkish-Iranian plateau (blue), the third rapid elevation of Qinghai-Tibetan Plateau (QTP; pink), and the fourth rapid upraising of QTP (yellow). The three photos show the fruits of Glycyrrhiza inflata (upper), G. uralensis (middle), and G. pallidiflora (lower), respectively.
Ancestral Area
Our DEC analysis (Figure 3; also see DEC result in the Supplementary File) indicated the interior of Eurasia and the circum-Mediterranean regions as the ancestral range of Glycyrrhiza s.l. (Node 1; coding: BC). The MRCA of was Clade I was restricted in circum-Mediterranean region (coding: C), and that of Glycyrrhiza sensu Meng spanned from Russian Far East and northern China to the Caucasus region (Node 2; coding: AB). Glycyrrhiza sensu Meng split into the MRCA of Clades II and III, spanning eastern Asia and North America (Node 3; coding: AE), and that of Clade IV (Node 6), which was distributed in the interior of Eurasia (coding: B). Subsequently, the MRCA of Clade II expanded to South America (Node 4; coding: EF), while the ancestor of Clade III remained in eastern Asia (Node 5; coding: A) and subsequently migrated to Australia (Glycyrrhiza acanthocarpa; coding: D). As for Clade IV, most of taxa were confined in the interior of Eurasia (coding: B), while a few groups, including the MRCA of the FOT clades (Node 8), and the MRCA of G. glabra and G. glandulifera Waldst. & Kit., spread back to the circum-Mediterranean region (coding: BC). Also within Clade IV, G. uralensis, dispersed to eastern Asia (coding: AB).
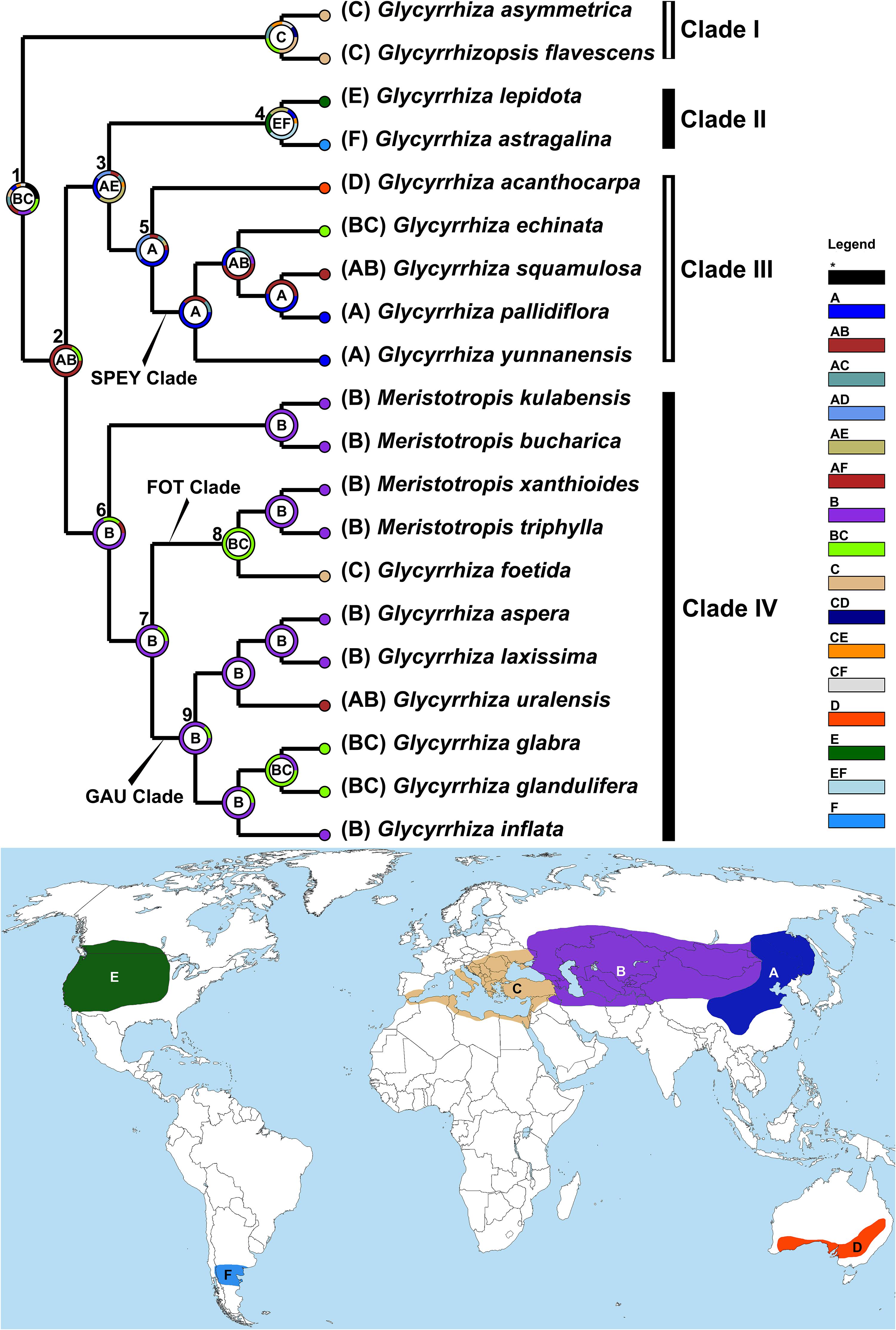
Figure 3. Ancestral area reconstruction of Glycyrrhiza s.l. estimated under the DEC model implemented in RASP v.4.0 based on the tree obtained from BEAST. The most likely ancestral distributions are labeled in the circle of nodes. The map shows the coding areas in different colors. For each species with multiple accessions, the “# 1” accessions were selected for this analysis (details see Supplementary Table S1).
Discussion
Phylogenetic Relationships of Glycyrrhiza s.l.
As shown in previous studies, Glycyrrhiza belongs to the IRLC (inverted repeat lacking clade; Lavin et al., 1990; Wojciechowski et al., 2000, 2004; Lock and Schrire, 2005) and more broadly to the Hologalegina, a larger group including IRLC and the robinioid clade (Wojciechowski, 2003; Lavin et al., 2005; Farruggia and Howard, 2011; Cardoso et al., 2012, 2013; also see Figure 1). Our analyses suggested that a large clade comprising Glycyrrhiza s.l. and Wisterieae sensu Compton et al. (2019) was sister to the rest of the IRLC (Figure 1). In previous taxonomic studies, Glycyrrhiza was treated within tribe Galegeae (Grigorev and Vasilchenko, 1948; Chamberlain, 1970; Bao and Larsen, 2010), however, our results show that Galegeae is non-monophyletic, with Glycyrrhiza and Astragalus strictus R.Grah. ex Benth distantly related, consistent with recent molecular phylogenetic studies (Wojciechowski et al., 2004; LPWG, 2013; Duan et al., 2015, 2016).
Within Glycyrrhiza s.l., we recognized four main clades (Figure 1) that are consistent with earlier studies having more limited sampling of the genus (Erayman et al., 2014; Çetin, 2015; Altay et al., 2016). Clade I includes Glycyrrhizopsis flavescens and Glycyrrhiza asymmetrica (Figure 1) and is sister to Glycyrrhiza sensu Meng. Therefore, it may be appropriate to treat G. asymmetrica in Glycyrrhizopsis. However, as our present study is based on a single accession of G. asymmetrica, it is unclear whether the species should be merged with Glycyrrhizopsis flavescens.
The monophyletic Glycyrrhiza sensu Meng contains Clades II, III, and IV and is sister to Clade I. The species of Clade II and III are distinguished on the basis of thorny, globular to ellipsoid fruits (except for G. squamulosa with prickless pods; see Grigorev and Vasilchenko, 1948; Jeanes, 1996; Li and Cui, 1998). Clade II includes Glycyrrhiza astragalina and G. lepidota (Figure 1), which are restricted in South and North America, respectively (Meng, 2005). This clade was resolved as sister to Clade III, and that result is new to this study.
The core taxa of Clade III are distributed in temperate Eurasia (Kruganova, 1955; Li and Lu, 2015), however, G. acanthocarpa ranges to southern Australia and diverged first in this clade (Figure 1). Within Clade III, G. pallidiflora is not sister to G. yunnanensis, which supports treating these as separate species (Grankina, 2008; Bao and Larsen, 2010; Li and Lu, 2015) rather than one species as was proposed by Meng (2005). On the other hand, Glycyrrhiza macedonica, represented by a single accession, and G. echinate, represented by two accessions, were sisters so that the controversial species status of the former (Grigorev and Vasilchenko, 1948; Kruganova, 1955; Li, 1963; Meng, 2005; Li and Lu, 2015; Çetin, 2015) cannot be determined by our study (Figure 1). Glycyrrhiza macedonica was circumscribed within G. echinata by Meng (2005) and Çetin (2015) while Grigorev and Vasilchenko (1948), Kruganova (1955), Li (1963), and Li and Lu (2015) kept it as a separate species. Their species boundary needs to be further delimited in the future study with additional evidence.
Clade IV was previously recovered by Hayashi et al. (2005) and Meng (2005). This clade, exhibits diverse morphology, is the largest clade in Glycyrrhiza s.l., and is widely distributed in northern temperate regions of the Old World (Kruganova, 1955; Li and Lu, 2015). Our result (Figure 1) explicitly resolved three monophyletic species: G. foetida, G. inflata and G. uralensis. As for other species, we discover that G. glandulifera was embedded within the accessions of G. glabra and G. iconica Hub.-Mor. was sister to a complex with G. laxissima Vassilcz. that was within G. aspera Pall. Additionally, Meristotropis kulabensis was embedded within M. bucharica, and M. xanthioides was nested within M. triphylla. In these respects, our results are congruent with Meng (2005) and agree with circumscribing G. glandulifera, G. laxissima, Meristotropis kulabensis, and M. xanthioides as synonyms of G. glabra, G. aspera, M. bucharica, and M. triphylla, respectively. Moreover, it is notable that the “three-leaflets group,” formerly recognized as the genus Meristotropis by Vasilchenko (1948) and Kruganova (1955), is non-monophyletic according to our cp genome tree (Figure 1) and can be merged into Glycyrrhiza as suggested by Li (1963), Meng (2005), and Li and Lu (2015). The formal generic and species delimitation will be included in our taxonomy study in the near future.
Historical Biogeography of Glycyrrhiza s.l.
According to our dating result (Figure 2) and ancestral area inferences (Figure 3), the ancestor of Glycyrrhiza s.l. originated during the late Eocene and its MRCA was likely distributed within the interior of Eurasia and circum-Mediterranean region. From the late Eocene to early Oligocene, the global temperature saw a dramatic decrease (Prothero, 1994; Zachos et al., 2001; Hansen et al., 2013) and the climate turned drier in Eurasia (Axelrod, 1975; Utescher and Mosbrugger, 2007; Sun et al., 2014), where the boreotropical flora, which had flourished in the early Cenozoic, was gradually replaced by sclerophyllous vegetation (Tiffney, 1985a, b; Mai, 1989). The climatic and vegetational change may lead to the separation between the MRCA of mesic/xeric, herbaceous Glycyrrhiza s.l. and Wisterieae, which are primarily thermophilic, woody lianas (Compton et al., 2019).
Our ancestral area reconstruction indicated that Clade I split from Glycyrrhiza sensu Meng in the Middle Miocene (Figure 2). The split was likely caused by a vicariance event that separated the MRCA of Clade I in the Mediterranean region and an ancestor of Glycyrrhiza sensu Meng in the interior of Eurasia. Thereafter, Glycyrrhiza sensu Meng was dispersed into eastern Asia before the clade diversified. In contrast, Clade I remained isolated in the Mediterranean region where extant species are endemic to the southern Anatolian plateau (Meng, 2005; Öztürk et al., 2017). The vicariance event leading to the separation of Clade I from Glycyrrhiza sensu Meng may have been the uplift of Turkish-Iranian Plateau (∼22–12 Ma; Mouthereau, 2011; Djamali et al., 2012) while the short period of warm climate in Middle Miocene (Middle Miocene Climatic Optimum; Böhme, 2003; You et al., 2009) may have facilitated the spread of Glycyrrhiza sensu Meng into eastern Asia (Figure 3).
Historical biogeography may help to elucidate the taxonomy of Glycyrrhizopsis. Most taxonomists treated Glycyrrhizopsis (corresponding to Clade I herein) within Glycyrrhiza (Li, 1963; Chamberlain, 1970; Li and Lu, 2015; Çetin, 2015), however, a few revisions raised Glycyrrhizopsis (Boissier, 1856; Meng, 2005) to generic status given the characters of yellow corolla, separated wings and dehiscent legumes. The geographic segregation of Glycyrrhizopsis from Glycyrrhiza in the Middle Miocene may have lent more support to the generic status of Glycyrrhizopsis as the two genera have seemingly developed in isolation for ca. 17 million years (Figure 2, Node 1).
The third rapid uplift of the Qinghai-Tibetan Plateau (QTP) occurred at 8–7 Ma, leading to a cooler climate and aridification of inland Asia (Harrison et al., 1992; Liu et al., 2001; Zheng and Yao, 2006; Wang et al., 2008) and subsequent psychrophytic and xerophytic adaptation of some temperate forest species, some of which developed shrub or herbaceous habits (Sun, 2002; Meng et al., 2015). Within Glycyrrhiza sensu Meng, two groups may have diverged within this paleoclimatic context around the Miocene-Pliocene boundary (Figure 2): the MRCA of Clades II and III, which was distributed in eastern Asia and North America, and the MRCA of Clade IV, which occurred in the interior of Eurasia (Figure 3).
Glycyrrhiza sensu Meng underwent rapid diversification and reached the New World and Australia within a short period during Pliocene. The ancestor of each of Clades II and III separated at ca. 3.53 Ma, and the crown groups of Clades II and III diverged at ca. 2.55 Ma and ca. 2.82 Ma, respectively (Figure 2). On the other hand, Clade IV, which contains more than half of the species of Glycyrrhiza sensu Meng, began divergence ca. 3.26 Ma (Figure 2). The initiation of the rapid diversification of Glycyrrhiza sensu Meng is possibly a response to the last (fourth) rapid uplift of the QTP. The uplift yielded aridification of the Asian interior and the formation of the Loess Plateau, which may have facilitated speciation of the Eurasian Glycyrrhiza sensu Meng (3.6–2.5 Ma; Chen et al., 1999; Li and Fang, 1999; Li et al., 2001; Tang and Liu, 2001; Zheng and Yao, 2006). The phenomenon of recent rapid diversification driven by climatic and habitat change is well-known by phytogeographers, and other examples can be seen within IRLC groups (Gueldenstaedtia and Tibetia in Xie et al., 2016; Oxytropis in Shahi Shavvon et al., 2017; Astragalus in Azani et al., 2019; Hedysarum in Nafisi et al., 2019) in papilionoid genera (Ammopiptanthus in Shi et al., 2017; Sesbania in Farruggia et al., 2018) and in angiosperm taxa (Allium and mint tribe Elsholtzieae in Li et al., 2016, 2017), respectively; Globularia and Campylanthus in Affenzeller et al. (2018), Coptis in Xiang et al. (2018), and Paris in Ji et al. (2019).
During the early Pliocene, species of Clade II migrated to temperate western North America (Glycyrrhiza lepidota; ca. 5.36–3.53 Ma; Figures 2, 3: Nodes 2–3), then arrived at temperate South America in the late Pliocene (G. astragalina; ca. 3.53–2.55 Ma; Figures 2, 3: Nodes 3–4) (Reiche, 1898; Gómer-Sosa, 1999; Meng, 2005). The ancestor of the Clade II was less likely to disperse directly from eastern Asia to South America (see map in Figure 3; but see Du et al., 2020), we suspect a migration route from eastern Asia to North America via the Bering land bridge (BLB). The timing of the migration may have coincided with BLB II (20–3 Ma), which is regarded as the main phase for trans-Beringian floristic exchanges (Wen et al., 2016; Duan et al., 2020). Some other papilionoid IRLC genera, such as Astragalus (Azani et al., 2019) and Hedysarum (Liu, 2017) were also found to have migrated through BLB from the early Pliocene to early Pleistocene. The disjunct pattern of Glycyrrhiza lepidota and G. astragalina comprises a temperate amphitropical disjunction (Raven, 1963; Wen and Ickert-Bond, 2009; Simpson et al., 2017) which may often result from bird-mediated long-distance dispersal (Popp et al., 2011). The rapid dispersal of G. astragalina from North America to South America (within one million years, see above) seems to favor an LDD hypothesis rather than one of gradual range expansion. This is especially true due to intervening habitats across the Americas that may have been unsuitable for the ancestor of this group and would have served a barrier to gene flow along with distance following an LDD, also supporting the rapid speciation event (Harris et al., 2018).
Within Clade III, the MRCA of Glycyrrhiza acanthocarpa and the SPEY clade diverged within the late Pliocene (Figure 2), and, subsequently, G. acanthocarpa reached southern Australia (Kruganova, 1955; Meng, 2005; see Figure 3). The pattern of Asian-Australian disjunction is relatively uncommon within angiosperms. Thorne (1972) who regarded the pattern as “North and South Temperate disjunction,” speculated its possible causes including “extinction of montane species formerly spanning the gaps” or due to bird migration. Although we cannot completely rule out the scenario that Glycyrrhiza arrived at Australia by a stepwise colonization directed from Southeast Asia via Indo-China region and Indonesia archipelago (Raven and Axelrod, 1974), the vast area of the tropical and subtropical regions between northern eastern China and southern Australia, which is uninhabitable to Glycyrrhiza, significantly decreases this potential (Heaney, 1991; Kershaw, 1994) over the relatively short time frame involved in the evolution of this genus. Thus, we are inclined to think that the colonization of Australia from an eastern Asian ancestor may result from a single or a few bird-mediated LDD events (Holzapfel, 1994; see Figure 3: A, Node 5). Notably, more Australian plant genera may have originated from other continents, i.e., from South America (Nie et al., 2012) and Africa-western Asia (Temirbayeva and Zhang, 2015; Kramina et al., 2016) compared to eastern Asia. Therefore, our study provides a case of this uncommon pattern of the Asia-originated Asian-Australian disjunct distribution, such as reported in Slovák et al. (2018) for Picris (Compositae). A more common pattern was observed for SPEY clade, for which the MRCA was located in eastern Asia (Figure 3) with subsequent spread back to the interior of Eurasia and the Mediterranean region (Figure 3) during the early Pleistocene (since 2.4 Ma; Figure 2).
Similar to the case of separation of Clades II and III (see Discussion above and Figures 2, 3), Central Asian aridification induced by the fourth rapid elevation of QTP may also have led to the split within Clade IV (Figure 2). The MRCA of Clade IV occurred in the interior of Eurasia and, while its two descended lineages, Meristotropis bucharica and the ancestor of the rest of Clade IV remained in the same area (Figure 3). As the second diverging group within Clade IV, the MRCA of the FOT clade migrated from the interior of Eurasia to the Mediterranean region (Figure 3). A vicariance event then occurred within the FOT clade during the Middle Pleistocene (ca. 0.67 Ma; Figure 2), so that Meristotropis triphylla and Glycyrrhiza foetida were confined in Central Asia and North Africa, respectively (Kruganova, 1955; Meng, 2005; Li and Lu, 2015). The emergence of the Central Asian glacier in Pleistocene (Abramowski et al., 2006) may have served as a climatic barrier between these two species leading to their speciation in allopatry.
Within the GAU clade, we noticed that Glycyrrhiza uralensis is sister to a clade formed by G. aspera and G, iconica with insignificant support (Figure 1). Species of the GAU clade have more or less overlapping distributions (Kruganova, 1955; Meng, 2005) and previous studies reported that natural hybridization frequently occurred among these taxa (Ashurmetov, 1996; Chen et al., 2017; Kang et al., 2018), which may cause the weakly supported topology. Thus, it might be premature to structure the whole biogeographic story for the GAU clade at this stage, but we can still uncover its dispersal directions from interior of Eurasia (Figure 3) eastwards to eastern Asia (G. uralensis) and westwards to Mediterranean region (G. glabra and G. glandulifera) within the last million years (since 0.93 Ma; Figure 2). Nearly all of the medicinal liquorice species belong to the GAU clade (Chinese Pharmacopoeia Commission, 2015; Öztürk et al., 2017) but its accurate phylogenetic relationships and divergence/hybridization history need further studies based on a dense populational sampling and reliable nuclear sequences.
Conclusion
The liquorice genus Glycyrrhiza has broad cultural and medicinal uses within Eurasia and the North America. This study presents the first comprehensive analysis of its evolutionary and biogeographic history using a phylogenomics approach with extensive sampling within Glycyrrhiza s.l. Our phylogenetic results support the monophyly of Glycyrrhiza s.l., inclusive of the satellite genera, Glycyrrhizopsis and Meristotropis. Glycyrrhizopsis appears phylogenetically distinct from Glycyrrhiza sensu Meng (2005) which includes Meristotropis. We suggest that Glycyrrhiza s.l. may have originated during the late Eocene, and its MRCA was distributed in the interior of Eurasia and the circum-Mediterranean region. Thereafter, the uplift of Turkish-Iranian and Qinghai-Tibetan Plateaus may be the main driver of rapid diversification of the major clades in Glycyrrhiza s.l. Since Pliocene, three liquorice species reached the temperate regions of North America, South America and Australia, respectively, via long-distance dispersal. Within Glycyrrhiza sensu Meng, the Asian-Australian disjunction is relatively rare in angiosperms, so that our study represents an important documentation of this pattern. Our results provide a robust theoretical foundation for sustainable utilization of genetic, pharmacological, and cultural resources of the economically significant liquorice genus and may be helpful elucidating evolutionary processes leading to broad intercontinental disjunctions in plants.
Data Availability Statement
The datasets presented in this study can be found in online repositories. The names of the repository/repositories and accession number(s) can be found in the article/Supplementary Table S1.
Author Contributions
LD and JW designed the research. H-FC financially supported this study. LD, EA, KE, PL, H-FC, and HH collected the samples. Z-RZ carried out the experiment. AH and CS performed the data analysis. LD, AH, and JW discussed the results and wrote the draft. All authors agreed on the contents of the final manuscript.
Funding
This study was supported by grants from the Natural Science Foundation of China (Grant No. 31600162), Science and Technology Key Research and Development project of Guangxi (Grant No. Guike AB18221054), Strategic Priority Research Program of Chinese Academy of Sciences (Grant No. XDA13020603), the Laboratories of Analytical Biology of the National Museum of Natural History (Smithsonian Institution), and the CAS Scholarship.
Conflict of Interest
The authors declare that the research was conducted in the absence of any commercial or financial relationships that could be construed as a potential conflict of interest.
Acknowledgments
We thank four reviewers for constructive suggestions on the manuscript. We are grateful to the curators of the following herbaria for providing specimen photos, leaf samples, seeds, or DNA samples: A, CSH, E, IBSC, K, KNYA, KUN, LE, MA, MO, P, PE, TASH, US, WUK, and XJBI. We thank Gabriel Johnson, Jing Liu, and Qin-Qin Li for assistance in the lab; Artyom Leostrin and Robin Everly for literature searches; and Cheng Du, Imalka Kahandawala, Jia-Hui Lu, Jing-Zhi Gong, Kathryn Richardson, Liang Zhao, Pei-Liang Liu, Shi-Min Duan, Virginia Valcárcel, Wei Shi, Zhao-Yang Chang, Zhen-Hai Wu, and Zhuo Zhou for sample collecting.
Supplementary Material
The Supplementary Material for this article can be found online at: https://www.frontiersin.org/articles/10.3389/fpls.2020.00793/full#supplementary-material
Footnotes
- ^ https://dnabank.science.kew.org
- ^ www.bioinformatics.babraham.ac.uk/projects/fastqc/
- ^ http://tree.bio.ed.ac.uk/software/tracer
- ^ http://www.stratigraphy.org/
References
Abramowski, U., Bergau, A., Seebach, D., Zech, R., Glaser, B., Sosin, P., et al. (2006). Pleistocene glaciations of Central Asia: results from 10Be surface exposure ages of erratic boulders from the Pamir (Tajikistan), and the Alay-Turkestan range (Kyrgyzstan). Quat. Sci. Rev. 25, 1080–1096. doi: 10.1016/j.quascirev.2005.10.003
Affenzeller, M., Kadereit, J. W., and Comes, H. P. (2018). Parallel bursts of recent and rapid radiation in the Mediterranean and Eritreo-Arabian biodiversity hotspots as revealed by Globularia and Campylanthus (Plantaginaceae). J. Biogeogr. 45, 552–566. doi: 10.1111/jbi.13155
Ali, S. I. (1977). “Glycyrrhiza,” in Flora of West Pakistan, NO. 100, eds E. Nasir and S. I. Ali (Karachi: Ferozsons Publishers), 94–97.
Altay, V., Karahan, F., Öztürk, M., Hakeem, K. R., Ilhan, E., and Erayman, M. (2016). Molecular and ecological investigations on the wild populations of Glycyrrhiza L. Taxa distributed in the East Mediterranean Area of Turkey. J. Plant Res. 129, 1021–1032. doi: 10.1007/s10265-016-0864-6
Ashurmetov, O. (1996). Selection of parental pairs for obtaining hybrids in the genera Glycyrrhiza L. and Meristotropis Fisch. et Mey. Genet. Resour. Crop Evol. 43, 167–171. doi: 10.1007/BF00126760
Axelrod, D. I. (1975). Evolution and biogeography of madrean-Tertiary sclerophyll vegetation. Ann. Mo. Bot. Gard. 62, 280–334. doi: 10.2307/2395199
Azani, N., Bruneau, A., Wojciechowski, M. F., and Zarre, S. (2019). Miocene climate change as a driving force for multiple origins of annual species in Astragalus (Fabaceae, Papilionoideae). Mol. Phylogenet. Evol. 137, 210–221. doi: 10.1016/j.ympev.2019.05.008
Bankevich, A., Nurk, S., Antipov, D., Gurevich, A. A., Dvorkin, M., Kulikov, A. S., et al. (2012). SPAdes: a new genome assembly algorithm and its applications to single-cell sequencing. J. Comput. Biol. 19, 455–477. doi: 10.1089/cmb.2012.0021
Bao, B. J., and Larsen, K. (2010). “Glycyrrhiza,” in Flora of China, Vol. 10, eds Z. Y. Wu, D. Y. Hong, and P. H. Raven (Beijing: Science Press), 509–511.
Bellot, S., and Renner, S. S. (2014). Exploring new dating approaches for parasites: the worldwide Apodanthaceae (Cucurbitales) as an example. Mol. Phylogenet. Evol. 80, 1–10. doi: 10.1016/j.ympev.2014.07.005
Böhme, M. (2003). The Miocene climatic optimum: evidence from ectothermic vertebrates of Central Europe. Palaeogeogr. Palaeoclimatol. Palaeoecol. 195, 389–401. doi: 10.1016/S0031-0182(03)00367-5
Boissier, P. E. (1856). “Glycyrrhizopsis,” in Diagnoses Plantarum Orientalium Novarum, Series II (5), ed. A. B. Herrmann (Geneva: Typis Ramboz et Schuchardt), 81–83.
Bolger, A. M., Lohse, M., and Usadel, B. (2014). Trimmomatic: a flexible trimmer for Illumina sequence data. Bioinformatics 30, 2114–2120. doi: 10.1093/bioinformatics/btu170
Bouckaert, R., Vaughan, T. G., Barido-Sottani, J., Duchêne, S., Fourment, M., Gavryushkina, A., et al. (2019). BEAST 2.5: an advanced software platform for Bayesian evolutionary analysis. PLoS Comput. Biol. 15:e1006650. doi: 10.1371/journal.pcbi.1006650
Britton, N. L., and Brown, H. A. (eds). (1897). “Glycyrrhiza,” in An Illustrated Flora of the Northern United States, Canada and the British Possessions, Vol. 2, (New York, NY: Charles Scribner’s sons), 310.
Cardoso, D., De Queiroz, L. P., Pennington, R. T., De Lima, H. C., Fonty, É., Wojciechowski, M. F., et al. (2012). Revisiting the phylogeny of papilionoid legumes: new insights from comprehensively sampled early-branching lineages. Am. J. Bot. 99, 1991–2013. doi: 10.3732/ajb.1200380
Cardoso, D., Pennington, R., De Queiroz, L., Boatwright, J., Van Wyk, B. E., Wojciechowski, M., et al. (2013). Reconstructing the deep-branching relationships of the papilionoid legumes. S. Afr. J. Bot. 89, 58–75. doi: 10.1016/j.sajb.2013.05.001
Çetin, Ö. (2015). Revision of the Genus Glycyrrhiza L. Distributed in Turkey. Doctoral dissertation, Selçuk University, Konya.
Chamberlain, D. F. (1970). “Glycyrrhiza,” in Flora of Turkey and East Aegean Islands, Vol. 3, ed. P. H. Davis (Edinburgh: Edinburgh University Press), 260–263.
Chen, C. N., Lu, J. H., Li, X. Y., Zhou, L. L., Xie, L. B., Li, X. L., et al. (2017). Inheritance analysis and discovery of chloroplast paternal inheritance in interspecific crossing of Glycyrrhiza. Guihaia 37, 162–168. doi: 10.11931/guihaia.gxzw201512021
Chen, L. X., Liu, J. P., Zhou, X. J., and Wang, P. X. (1999). Impact of uplift of Qinghai-Xizang Plateau and change of land-ocean distribution on climate over Asia. Quat. Sci. 4, 314–329.
Chinese Pharmacopoeia Commission (2015). Pharmacopoeia of the People’s Republic of China. 1. Beijing: China Medical Science Press, 86–87.
Compton, J. A., Schrire, B. D., Könyves, K., Forest, F., Malakasi, P., Mattapha, S., et al. (2019). The Callerya Group redefined and Tribe Wisterieae (Fabaceae) emended based on morphology and data from nuclear and chloroplast DNA sequences. Phytokeys 125, 1–112. doi: 10.3897/phytokeys.125.34877
Dastagir, G., and Rizvi, M. A. (2016). Glycyrrhiza glabra L.(Liquorice). Pak. J. Pharm. Sci. 29, 1727–1733.
Djamali, M., Baumel, A., Brewer, S., Jackson, S. T., Kadereit, J. W., López-Vinyallonga, S., et al. (2012). Ecological implications of Cousinia Cass. (Asteraceae) persistence through the last two glacial-interglacial cycles in the continental Middle East for the Irano-Turanian flora. Rev. Palaeobot. Palynol. 172, 10–20. doi: 10.1016/j.revpalbo.2012.01.005
Doyle, J. J., and Doyle, J. L. (1987). A rapid DNA isolation procedure for small quantities of fresh leaf tissue. Phytochem. Bull. 19, 11–15.
Du, Z. Y., Harris, A. J., and Xiang, Q. Y. (2020). Phylogenomics, co-evolution of ecological niche and morphology, and historical biogeography of buckeyes, horsechestnuts, and their relatives (Hippocastaneae, Sapindaceae) and the value of RAD-Seq for deep evolutionary inferences back to the Late Cretaceous. Mol. Phylogenet. Evol. 145:106726. doi: 10.1016/j.ympev.2019.106726
Duan, L., Harris, A. J., Su, C., Ye, W., Deng, S. W., Fu, L., et al. (2020). A fossil-calibrated phylogeny reveals the biogeographic history of the Cladrastis clade, an amphi-Pacific early-branching group in papilionoid legumes. Mol. Phylogenet. Evol. 143:106673. doi: 10.1016/j.ympev.2019.106673
Duan, L., Wen, J., Yang, X., Liu, P. L., Arslan, E., Ertuğrul, K., et al. (2015). Phylogeny of Hedysarum and tribe Hedysareae (Leguminosae: Papilionoideae) inferred from sequence data of ITS, matK, trnL-F and psbA-trnH. Taxon 64, 49–64. doi: 10.12705/641.26
Duan, L., Yang, X., Liu, P. L., Johnson, G., Wen, J., and Chang, Z. Y. (2016). A molecular phylogeny of Caraganeae (Leguminosae, Papilionoideae) reveals insights into new generic and infrageneric delimitations. Phytokeys 70, 111–137. doi: 10.3897/phytokeys.70.9641
Erayman, M., Ilhan, E., Güzel, Y., and Eren, A. H. (2014). Transferability of SSR markers from distantly related legumes to Glycyrrhiza species. Turk. J. Agric. For. 38, 32–38. doi: 10.3906/tar-1303-47
Farruggia, F. T., and Howard, J. H. (2011). Examination of five nuclear markers for phylogenetic study of Hologalegina (Leguminosae). Brittonia 63, 489–499. doi: 10.1007/s12228-011-9189-x
Farruggia, F. T., Lavin, M., and Wojciechowski, M. F. (2018). Phylogenetic systematics and biogeography of the pantropical genus Sesbania (Leguminosae). Syst. Bot. 43, 414–429. doi: 10.1600/036364418X697175
García, M. F. (1999). “Glycyrrhiza,” in Flora Iberica, Vol. 7, ed. S. Castroviejo (Madrid: Real Jardín Botánico), 350–353.
Gardner, C. (1991). “Glycyrrhiza,” in Flora of New South Wales, Vol. 2, ed. G. J. Harden (Kensington: New South Wales University Press), 442.
Gómer-Sosa, E. V. (1999). “Glycyrrhiza,” in Catálogo de las Plantas Vasculares de la República Argentina II, eds F. O. Zuloaga and O. M. Morrone (St. Louis, MO: Missouri Botanical Garden Press), 681.
Grankina, V. (2008). The system of the genus Glycyrrhiza L. (Fabaceae). Nov. Sist. Vyssh. Rast. 40, 89–108.
Grigorev, Y. S., and Vasilchenko, I. T. (1948). “Glycyrrhiza,” in Flora of the U.S.S.R. (English Version Published in 1972), Vol. 13, eds B. K. Shishkin and E. G. Bobrov (Moscow: Izdatel’stvo Akademii Nauk), 176–184.
Gussarova, G., Popp, M., Vitek, E., and Brochmann, C. (2008). Molecular phylogeny and biogeography of the bipolar Euphrasia (Orobanchaceae): recent radiations in an old genus. Mol. Phylogenet. Evol. 48, 444–460. doi: 10.1016/j.ympev.2008.05.002
Hansen, J., Sato, M., Russell, G., and Kharecha, P. (2013). Climate sensitivity, sea level and atmospheric carbon dioxide. Philos. Trans. R. Soc. A Math. 371:20120294. doi: 10.1098/rsta.2012.0294
Harris, A. J., Ickert-Bond, S., and Rodríguez, A. (2018). Long distance dispersal in the assembly of floras: a review of progress and prospects in North America. J. Syst. Evol. 56, 430–448. doi: 10.1111/jse.12422
Harris, A. J., Wen, J., and Xiang, Q. Y. (2013). Inferring the biogeographic origins of inter-continental disjunct endemics using a Bayes-DIVA approach. J. Syst. Evol. 51, 117–133. doi: 10.1111/jse.12007
Harrison, T. M., Copel, P., Kidd, W. S. F., and Yin, A. (1992). Raising tibet. Science 255, 1663–1670. doi: 10.1126/science.255.5052.1663
Hayashi, H., Hosono, N., Kondo, M., Hiraoka, N., and Ikeshiro, Y. (1998). Phylogenetic relationship of Glycyrrhiza plants based on rbcL sequences. Biol. Pharm. Bull. 21, 782–783. doi: 10.1248/bpb.21.782
Hayashi, H., Hosono, N., Kondo, M., Hiraoka, N., Ikeshiro, Y., Shibano, M., et al. (2000). Phylogenetic relationship of six Glycyrrhiza species based on rbcL sequences and chemical constituents. Biol. Pharm. Bull. 23, 602–606. doi: 10.1248/bpb.23.602
Hayashi, H., Miwa, E., and Inoue, K. (2005). Phylogenetic relationship of Glycyrrhiza lepidota, American licorice, in genus Glycyrrhiza based on rbcL sequences and chemical constituents. Biol. Pharm. Bull. 28, 161–164. doi: 10.1248/bpb.28.161
Hayashi, H., and Sudo, H. (2009). Economic importance of licorice. Plant Biotechnol. 26, 101–104. doi: 10.5511/plantbiotechnology.26.101
He, H., Deng, C., Pan, Y., Deng, T., Luo, Z., Sun, J., et al. (2011). New 40Ar/39Ar dating results from the Shanwang Basin, eastern China: constraints on the age of the Shanwang Formation and associated biota. Phys. Earth Planet. Inter. 187, 66–75. doi: 10.1016/j.pepi.2011.05.002
Heaney, L. R. (1991). “A synopsis of climatic and vegetational change in Southeast Asia,” in Tropical Forests and Climate, ed. N. Myers (Dordrecht: Springer), 53–61. doi: 10.1007/978-94-017-3608-4_6
Heled, J., and Drummond, A. J. (2012). Calibrated tree priors for relaxed phylogenetics and divergence time estimation. Syst. Biol. 61, 138–149. doi: 10.1093/sysbio/syr087
Holzapfel, S. (1994). A revision of the genus Picris (Asteraceae, Lactuceae) s.l. in Australia. Willdenowia 24, 97–218.
Hu, J. M., and Chang, S. P. (2003). Two new members of the Callerya group (Fabaceae) based on phylogenetic analysis of rbcL sequences: Endosamara racemosa (Roxb.) Geesink and Callerya vasta (Kosterm.) Schot. Taiwania 28, 118–128. doi: 10.6165/tai.2003.48(2).118
Jeanes, J. A. (1996). “Glycyrrhiza,” in Flora of Victoria, Vol. 3, eds N. G. Walsh and N. G. Entwisle (Melbourne: Inkata Press), 698–699.
Ji, Y., Yang, L., Chase, M. W., Liu, C., Yang, Z., Yang, J., et al. (2019). Plastome phylogenomics, biogeography, and clade diversification of Paris (Melanthiaceae). BMC Plant Biol. 19:543. doi: 10.1186/s12870-019-2147-6
Jin, J. J., Yu, W. B., Yang, J. B., Song, Y., Yi, T. S., and Li, D. Z. (2018). GetOrganelle: a simple and fast pipeline for de novo assembly of a complete circular chloroplast genome using genome skimming data. bioRxiv [Preprint] doi: 10.1101/256479
Kang, S. H., Lee, J. H., Lee, H. O., Ahn, B. O., Won, S. Y., Sohn, S. H., et al. (2018). Complete chloroplast genome and 45S nrDNA sequences of the medicinal plant species Glycyrrhiza glabra and Glycyrrhiza uralensis. Genes Genet. Syst. 93, 83–89. doi: 10.1266/ggs.17-00002
Katoh, K., and Standley, D. M. (2013). MAFFT multiple sequence alignment software version 7: improvements in performance and usability. Mol. Biol. Evol. 30, 772–780. doi: 10.1093/molbev/mst010
Kearse, M., Moir, R., Wilson, A., Stones-Havas, S., Cheung, M., Sturrock, S., et al. (2012). Geneious Basic: an integrated and extendable desktop software platform for the organization and analysis of sequence data. Bioinformatics 28, 1647–1649. doi: 10.1093/bioinformatics/bts199
Kershaw, A. P. (1994). Pleistocene vegetation of the humid tropics of northeastern Queensland, Australia. Palaeogeogr. Palaeoclimatol. Palaeoecol. 109, 399–412. doi: 10.1016/0031-0182(94)90188-0
Kramina, T. E., Degtjareva, G. V., Samigullin, T. H., Valiejo-Roman, C. M., Kirkbride, J. H., Volis, S., et al. (2016). Phylogeny of Lotus (Leguminosae: Loteae): partial incongruence between nrITS, nrETS and plastid markers and biogeographic implications. Taxon 65, 997–1018. doi: 10.12705/655.4
Kruganova, E. A. (1955). A review of species from the genera Glycyrrhiza L. and Meristotropis Fish. et C.A.Mey. Acta Inst. Bot. Acad. Sci. 11, 161–197.
Lanfear, R., Frandsen, P. B., Wright, A. M., Senfeld, T., and Calcott, B. (2016). PartitionFinder 2: new methods for selecting partitioned models of evolution for molecular and morphological phylogenetic analyses. Mol. Biol. Evol. 34, 772–773. doi: 10.1093/molbev/msw260
Lavin, M., Doyle, J. J., and Palmer, J. D. (1990). Evolutionary significance of the loss of the chloroplast-DNA inverted repeat in the Leguminosae subfamily Papilionoideae. Evolution 44, 390–402. doi: 10.2307/2409416
Lavin, M., Herendeen, P. S., and Wojciechowski, M. F. (2005). Evolutionary rates analysis of Leguminosae implicates a rapid diversification of lineages during the tertiary. Syst. Biol. 54, 575–594. doi: 10.1080/10635150590947131
Li, J., and Fang, X. (1999). Uplift of the Tibetan Plateau and environmental changes. Chin. Sci. Bull. 44, 2117–2124. doi: 10.1007/BF03182692
Li, J. J., Fang, X. M., Pan, B. T., Zhao, Z. J., and Song, Y. G. (2001). Late Cenozoic intensive uplift of Qinghai-Xizang Plateau and its impacts on environments in surrounding area. Quat. Sci. 21, 381–391.
Li, P., Qi, Z. C., Liu, L. X., Ohi-Toma, T., Lee, J., Hsieh, T. H., et al. (2017). Molecular phylogenetics and biogeography of the mint tribe Elsholtzieae (Nepetoideae, Lamiaceae), with an emphasis on its diversification in East Asia. Sci. Rep. 7:2057. doi: 10.1038/s41598-017-02157-6
Li, P. C. (1963). “Study of taxa of genus Glycyrrhiza Linn. (Leguminosae) in China,” in Proceedings of the of 30th Anniversary of Botanical Society of China, (Beijing: Institute of Scientific and Technical Intelligence of China), 90–91.
Li, P. C., and Cui, H. B. (1998). “Glycyrrhiza,” in Flora Reipublicae Popularis Sinicae, Vol. 42, ed. H. B. Cui (Beijing: Science Press), 167–176.
Li, Q. Q., Zhou, S. D., Huang, D. Q., He, X. J., and Wei, X. Q. (2016). Molecular phylogeny, divergence time estimates and historical biogeography within one of the world’s largest monocot genera. AoB Plants 8:lw041. doi: 10.1093/aobpla/plw041
Li, X. Y., and Lu, J. H. (2015). Revision of Glycyrrhiza L. In Taxonomy and Experimental Biology of the Genus Glycyrrhiza L. Shanghai: Fudan University Press, 3–51.
Linder, H. (2001). On areas of endemism, with an example from the African Restionaceae. Syst. Biol. 50, 892–912. doi: 10.1080/106351501753462867
Liu, P. L. (2017). Phylogeny and Biogeography of the Genus Hedysarum L. (Fabaceae). Doctoral dissertation, Northwest A&F University, Yangling.
Liu, S., Chi, X., Li, C., and Yang, R. (2001). The summarizing for the forming and uplifted mechanism of Qinghai-Tibet Plateau. World Geol. 20, 105–112.
Lock, J. M., and Schrire, B. D. (2005). “Galegeae,” in Legumes of the World, eds G. Lewis, B. Schrire, B. Mackinder, and M. Lock (Richmond, VA: Royal Botanic Gardens), 475–487.
LPWG (2013). The legume phylogeny working group, legume phylogeny and classification in the 21st century: progress, prospects and lessons for other species-rich clades. Taxon 62, 217–248. doi: 10.12705/622.8
LPWG (2017). The legume phylogeny working group, a new subfamily classification of the leguminosae based on a taxonomically comprehensive phylogeny. Taxon 66, 44–77. doi: 10.12705/661.3
Mai, D. H. (1989). “Development and regional differentiation of the European vegetation during the Tertiary,” in Woody Plants-Evolution and Distribution Since the Tertiary, ed. D. H. Mai (Vienna: Springer), 79–91. doi: 10.1007/978-3-7091-3972-1_4
Mau, B., Newton, M. A., and Larget, B. (1999). Bayesian phylogenetic inference via Markov chain Monte Carlo methods. Biometrics 55, 1–12. doi: 10.1111/j.0006-341X.1999.00001.x
Meng, H. H., Gao, X. Y., Huang, J. F., and Zhang, M. L. (2015). Plant phylogeography in arid North-west China: retrospectives and perspectives. J. Syst. Evol. 53, 33–46. doi: 10.1111/jse.12088
Meng, L. (2005). Systematics of Glycyrrhiza L. (Fabaceae) - with a Special Reference to its Relationship to Glycyrrhizopsis Boiss.&Bal. Doctoral dissertation, Institute of Botany, Chinese Academy of Sciences, Beijing.
Mouthereau, F. (2011). Timing of uplift in the Zagros belt/Iranian plateau and accommodation of late Cenozoic Arabia–Eurasia convergence. Geol. Mag. 148, 726–738. doi: 10.1017/S0016756811000306
Muellner-Riehl, A. N., Weeks, A., Clayton, J. W., Buerki, S., Nauheimer, L., Chiang, Y. C., et al. (2016). Molecular phylogenetics and molecular clock dating of Sapindales based on plastid rbcL, atpB and trnL-trnF DNA sequences. Taxon 65, 1019–1036. doi: 10.12705/655.5
Nafisi, H., Kazempour-Osaloo, S., Mozaffarian, V., and Schneeweiss, G. M. (2019). Molecular phylogeny and divergence times of the genus Hedysarum (Fabaceae) with special reference to section Multicaulia in Southwest Asia. Plant Syst. Evol. 305, 1001–1017. doi: 10.1007/s00606-019-01620-3
Nguyen, L. T., Schmidt, H. A., von Haeseler, A., and Minh, B. Q. (2015). IQ-TREE: a fast and effective stochastic algorithm for estimating maximum-likelihood phylogenies. Mol. Biol. Evol. 32, 268–274. doi: 10.1093/molbev/msu300
Nie, Z. L., Sun, H., Manchester, S. R., Meng, Y., Luke, Q., and Wen, J. (2012). Evolution of the intercontinental disjunctions in six continents in the Ampelopsis clade of the grape family (Vitaceae). BMC Evol. Biol. 12:17. doi: 10.1186/1471-2148-12-17
Öztürk, M., Altay, V., Rehman Hakeem, K., and Akçiçek, E. (2017). Liquorice: From Botany to Phytochemistry. Cham: Springer, 5–17. doi: 10.1007/978-3-319-74240-3
Paradis, E., and Schliep, K. (2018). ape 5.0: an environment for modern phylogenetics and evolutionary analyses in R. Bioinformatics 35, 526–528. doi: 10.1093/bioinformatics/bty633
Podlech, D. (1991). “Glycyrrhiza,” in Legumes of West Asia: A Check-List, eds J. M. Lock and K. Simpson (Richmond, VA: Royal Botanic Gardens), 136–137.
Polhill, R. M. (1981). “Galegeae,” in Advances in Legume Systematic, Part 1, eds R. M. Polhill and P. H. Raven (Richmond, VA: Royal Botanic Gardens, Kew), 357–363.
Polhill, R. M. (1994). “Classification of the Leguminosae,” in Phytochemical Dictionary of the Leguminosae, Vol. 1, eds I. W. Southon, F. A. Bisby, J. Buckingham, and J. B. Harborne (London: Chapman & Hall).
Popp, M., Mirré, V., and Brochmann, C. (2011). A single mid-pleistocene long-distance dispersal by a bird can explain the extreme bipolar disjunction in crowberries (Empetrum). Proc. Natl. Acad. Sci. U.S.A. 108, 6520–6525. doi: 10.1073/pnas.1012249108
Prothero, D. R. (1994). The Eocene-Oligocene Transition: Paradise Lost. New York, NY: Columbia University Press, 291.
Rannala, B., and Yang, Z. (1996). Probability distribution of molecular evolutionary trees: a new method of phylogenetic inference. J. Mol. Evol. 43, 304–311. doi: 10.1007/BF02338839
Raveendar, S., So, Y. S., Lee, K. J., Lee, D. J., Sung, J., and Chung, J. W. (2017). The complete chloroplast genome sequence of Glycyrrhiza lepidota (Nutt.) Pursh-An American wild licorice. J. Crop Sci. Biotechnol. 20, 295–303. doi: 10.1007/s12892-017-0137-0
Raven, P. H. (1963). Amphitropical relationships in the floras of North and South America. Q. Rev. Biol. 38, 151–177. doi: 10.1086/403797
Raven, P. H. (1972). Plant species disjunctions: a summary. Ann. Mo. Bot. Gard. 59, 234–246. doi: 10.2307/2394756
Raven, P. H., and Axelrod, D. I. (1974). Angiosperm biogeography and past continental movements. Ann. Mo. Bot. Gard. 61, 539–573. doi: 10.2307/2395021
Rechinger, K. H. (1984). “Glycyrrhiza & Meristotropis,” in Flora Iranica, NO. 157, ed. K. H. Rechinger (Graz: Akademische Druck- u. Verlagsanstalt), 164–171.
Ree, R. H., and Smith, S. A. (2008). Maximum likelihood inference of geographic range evolution by Dispersal, local Extinction, and Cladogenesis. Syst. Biol. 57, 4–14. doi: 10.1080/10635150701883881
Ronquist, F. (1997). Dispersal-vicariance analysis: a new approach to the quantification of historical biogeography. Syst. Biol. 46, 195–203. doi: 10.1093/sysbio/46.1.195
Ronquist, F., and Huelsenbeck, J. P. (2003). MrBayes 3: bayesian phylogenetic inference under mixed models. Bioinformatics 19, 1572–1574. doi: 10.1093/bioinformatics/btg180
Ronquist, F., Teslenko, M., van der Mark, P., Ayres, D. L., Darling, A., Höhna, S., et al. (2012). MrBayes 3.2: efficient Bayesian phylogenetic inference and model choice across a large model space. Syst. Biol. 61, 539–542. doi: 10.1093/sysbio/sys029
Sabir, J., Schwarz, E., Ellison, N., Zhang, J., Baeshen, N. A., Mutwakil, M., et al. (2014). Evolutionary and biotechnology implications of plastid genome variation in the inverted-repeat-lacking clade of legumes. Plant Biotechnol. J. 12, 743–754. doi: 10.1111/pbi.12179
Scoggan, H. J. (1978). Glycyrrhiza. In The Flora of Canada, Part 3. Ottawa: National Museum of Canada, 996.
Shahi Shavvon, R., Kazempour Osaloo, S., Maassoumii, A. A., Moharrek, F., Karaman Erkul, S., Lemmon, A. R., et al. (2017). Increasing phylogenetic support for explosively radiating taxa: the promise of high-throughput sequencing for Oxytropis (Fabaceae). J. Syst. Evol. 55, 385–404. doi: 10.1111/jse.12269
Sharma, V., Katiyar, A., and Agrawal, R. C. (2018). “Glycyrrhiza glabra: chemistry and pharmacological activity,” in Sweeteners: Pharmacology, Biotechnology, and Applications, eds J.-M. Mérillon and K. G. Ramawat (Cham: Springer International Publishing), 87–100. doi: 10.1007/978-3-319-27027-2_21
Shi, W., Liu, P. L., Duan, L., Pan, B. R., and Su, Z. H. (2017). Evolutionary response to the Qinghai-Tibetan Plateau uplift: phylogeny and biogeography of Ammopiptanthus and tribe Thermopsideae (Fabaceae). PeerJ 5:e3607. doi: 10.7717/peerj.3607
Simpson, M. G., Johnson, L. A., Villaverde, T., and Guilliams, C. M. (2017). American amphitropical disjuncts: perspectives from vascular plant analyses and prospects for future research. Am. J. Bot. 104, 1600–1650. doi: 10.3732/ajb.1700308
Slovák, M., Kučera, J., Lack, H. W., Ziffer-Berger, J., Melicharková, A., Záveská, E., et al. (2018). Diversification dynamics and transoceanic Eurasian-Australian disjunction in the genus Picris (Compositae) induced by the interplay of shifts in intrinsic/extrinsic traits and paleoclimatic oscillations. Mol. Phylogenet. Evol. 119, 182–195. doi: 10.1016/j.ympev.2017.11.006
Stanley, T. D., and Ross, E. M. (1983). Flora of South-Eastern Queensland, Vol. I. Brisbane: Queensland Deapartment of Primary Industries, 302.
Sun, H. (2002). Tethys retreat and Himalayas-Hengduanshan Mountains uplift and their significance on the origin and development of the Sino-Himalayan elements and alpine flora. Acta Bot. Yunnanica 24, 273–288.
Sun, J., Ni, X., Bi, S., Wu, W., Ye, J., Meng, J., et al. (2014). Synchronous turnover of flora, fauna, and climate at the Eocene–Oligocene Boundary in Asia. Sci. Rep. 4:7463. doi: 10.1038/srep07463
Tang, M., and Liu, Y. (2001). On causes and environmental consequences of the uplift of Qinghai-Xizang Plateau. Quat. Sci. 21, 500–507.
Temirbayeva, K., and Zhang, M. L. (2015). Molecular phylogenetic and biogeographical analysis of Nitraria based on nuclear and chloroplast DNA sequences. Plant Syst. Evol. 301, 1897–1906. doi: 10.1007/s00606-015-1202-5
Thorne, R. F. (1972). Major disjunctions in the geographic ranges of seed plants. Q. Rev. Biol. 47, 365–411. doi: 10.1086/407399
Tiffney, B. H. (1985a). Perspectives on the origin of the floristic similarity between eastern Asia and eastern North America. J. Arnold Arbor. 66, 73–94. doi: 10.5962/bhl.part.13179
Tiffney, B. H. (1985b). The Eocene North Atlantic land bridge: its importance in Tertiary and modern phytogeography of the Northern Hemisphere. J. Arnold Arbor. 66, 243–273. doi: 10.5962/bhl.part.13183
Townsend, C. C. (1974). “Glycyrrhiza,” in Flora of Iraq, Vol. 3, eds C. C. Townsend and E. Guest (Baghdad: Ministry of Agriculture and Agrarian Reform), 445–449.
Utescher, T., and Mosbrugger, V. (2007). Eocene vegetation patterns reconstructed from plant diversity-a global perspective. Palaeogeogr. Palaeoclimatol. Palaeoecol. 247, 243–271. doi: 10.1016/j.palaeo.2006.10.022
Vasilchenko, I. T. (1948). “Meristotropis,” in Flora of the U.S.S.R. vol. 13 (English version published in 1972), eds B. K. Shishkin and E. G. Bobrov (Moscow: Izdatel’stvo Akademii Nauk), 184–186.
Wang, C., Zhao, X., Liu, Z., Lippert, P. C., Graham, S. A., Coe, R. S., et al. (2008). Constraints on the early uplift history of the Tibetan Plateau. Proc. Natl. Acad. Sci. U.S.A. 105, 4987–4992. doi: 10.1073/pnas.0703595105
Wang, Q., Dilcher, D. L., Zhu, X. Y., Zhou, Y. L., and Lott, T. A. (2006). Fruits and leaflets of Wisteria (Leguminosae, Papilionoideae) from the Miocene of Shandong Province, eastern China. Int. J. Plant Sci. 167, 1061–1074. doi: 10.1086/502717
Warnock, R. C. M., Parham, J. F., Joyce, W. G., Lyson, T. R., and Donoghue, P. C. J. (2015). Calibration uncertainty in molecular dating analyses: there is no substitute for the prior evaluation of time priors. Proc. R. Soc. B Biol. Sci. 282:20141013. doi: 10.1098/rspb.2014.1013
Weber, J. Z. (1986). “Glycyrrhiza,” in Flora of South Australia Part II, 4th Edn, eds J. P. Jessop and H. R. Teolken (Adelaide: South Australian Government Printing Division), 603–604.
Wei, R., Yan, Y. H., Harris, A. J., Kang, J. S., Shen, H., Xiang, Q. P., et al. (2017). Plastid phylogenomics resolve deep relationships among eupolypod II ferns with rapid radiation and rate heterogeneity. Genome Biol. Evol. 9, 1646–1657. doi: 10.1093/gbe/evx107
Wen, J., Harris, A. J., Kalburgi, Y., Zhang, N., Xu, Y., Zheng, W., et al. (2018). Chloroplast phylogenomics of the New World grape species (Vitis, Vitaceae). J. Syst. Evol. 56, 297–308. doi: 10.1111/jse.12447
Wen, J., and Ickert-Bond, S. M. (2009). Evolution of the Madrean-Tethyan disjunctions and the North and South American amphitropical disjunctions in plants. J. Syst. Evol. 47, 331–348. doi: 10.1111/j.1759-6831.2009.00054.x
Wen, J., Nie, Z. L., and Ickert-Bond, S. M. (2016). Intercontinental disjunctions between eastern Asia and western North America in vascular plants highlight the biogeographic importance of the Bering land bridge from late Cretaceous to Neogene. J. Syst. Evol. 54, 469–490. doi: 10.1111/jse.12222
Western Australian Herbarium (1998). FloraBase-the Western Australian Flora. Kensington: Department of Biodiversity, Conservation and Attractions.
Wojciechowski, M. F. (2003). “Reconstructing the phylogeny of legumes (Leguminosae): an early 21st century perspective,” in Advances in Legume Systematics, Part 10, eds B. B. Klitgaard and A. Bruneau (Richmond, VA: Royal Botanic Gardens), 5–35.
Wojciechowski, M. F., Lavin, M., and Sanderson, M. J. (2004). A phylogeny of legumes (Leguminosae) based on analyses of the plastid matK gene resolves many well-supported subclades within the family. Am. J. Bot. 91, 1846–1862. doi: 10.3732/ajb.91.11.1846
Wojciechowski, M. F., Sanderson, M. J., Steele, K. P., and Liston, A. (2000). “Molecular phylogeny of the “temperate herbaceous tribes” of papilionoid legumes: a supertree approach,” in Advances in Legume Systematics, Part 9, eds P. S. Herendeen and A. Bruneau (Richmond, VA: Royal Botanic Garden), 277–298.
Wu, Z. Y., Lu, A. M., Tang, Y. C., Chen, Z. D., and Li, D. Z. (2003). The Families and Genera of Angiosperms in China: A Comprehensive Analysis. Beijing: Science Press, 728.
Wu, Z. Y., Zhou, Z. K., Sun, H., Li, D. Z., and Peng, H. (2006). The Areal-Types of Seed Plants and their Origin and Differentiation, Vol. 112. Kunming: Yunnan Science & Technology Press, 264.
Wyman, S. K., Jansen, R. K., and Boore, J. L. (2004). Automatic annotation of organellar genomes with DOGMA. Bioinformatics 20, 3252–3255. doi: 10.1093/bioinformatics/bth352
Xiang, K. L., Erst, A. S., Xiang, X. G., Jabbour, F., and Wang, W. (2018). Biogeography of Coptis Salisb. (Ranunculales, Ranunculaceae, Coptidoideae), an Eastern Asian and North American genus. BMC Evol. Biol. 18:74. doi: 10.1186/s12862-018-1195-0
Xie, Y. P., Meng, Y., Sun, H., and Nie, Z. L. (2016). Molecular phylogeny of Gueldenstaedtia and Tibetia (Fabaceae) and their biogeographic differentiation within Eastern Asia. PLoS One 11:e0162982. doi: 10.1371/journal.pone.0162982
Yakovlev, G. P. (1996). “Glycyrrhiza,” in Legumes of Northern Eurasia: A Check-List, eds G. P. Yakovlev, A. K. Sytin, and Y. R. Roskov (Richmond, VA: Royal Botanic Gardens), 289–292.
Yamazaki, M., Sato, A., Shimomura, K., Saito, K., and Murakoshi, I. (1994). Genetic relationships among Glycyrrhiza plants determined by RAPD and RPLP analyses. Biol. Pharm. Bull. 17, 1529–1531. doi: 10.1248/bpb.17.1529
Yang, L., Yang, Z., Liu, C., He, Z., Zhang, Z., Yang, J., et al. (2019). Chloroplast phylogenomic analysis provides insights into the evolution of the largest eukaryotic genome holder, Paris japonica (Melanthiaceae). BMC Plant Biol. 19:293. doi: 10.1186/s12870-019-1879-7
Yeo, P. F. (1968). “Glycyrrhiza,” in Flora Europaea, Vol. 2, eds T. G. Tutin, V. H. Heywood, N. A. Burges, D. M. Moore, D. H. Valentine, S. M. Walters, et al. (London: Cambridge University Press), 127.
You, Y., Huber, M., Müller, R. D., Poulsen, C. J., and Ribbe, J. (2009). Simulation of the middle Miocene climate optimum. Geophys. Res. Lett. 36:L0470. doi: 10.1029/2008GL036571
Yu, Y., Harris, A. J., Blair, C., and He, X. (2015). RASP (reconstruct ancestral state in phylogenies): a tool for historical biogeography. Mol. Phylogenet. Evol. 87, 46–49. doi: 10.1016/j.ympev.2015.03.008
Zachos, J., Pagani, M., Sloan, L., Thomas, E., and Billups, K. (2001). Trends, rhythms, and aberrations in global climate 65 Ma to present. Science 292, 686–693. doi: 10.1126/science.1059412
Zheng, D., and Yao, T. D. (2006). Uplifting of Tibetan Plateau with its environmental effects. Adv. Earth Sci. 21, 451–458.
Keywords: amphitropical disjunction, Asian-Australian disjunction, biogeography, Glycyrrhiza, Glycyrrhizopsis, long-distance dispersal, Meristotropis, Qinghai-Tibetan Plateau uplift
Citation: Duan L, Harris AJ, Su C, Zhang Z-R, Arslan E, Ertuğrul K, Loc PK, Hayashi H, Wen J and Chen H-F (2020) Chloroplast Phylogenomics Reveals the Intercontinental Biogeographic History of the Liquorice Genus (Leguminosae: Glycyrrhiza). Front. Plant Sci. 11:793. doi: 10.3389/fpls.2020.00793
Received: 29 December 2019; Accepted: 18 May 2020;
Published: 17 June 2020.
Edited by:
Carl J. Rothfels, University of California, Berkeley, United StatesReviewed by:
Martin F. Wojciechowski, Arizona State University, United StatesRan Wei, Institute of Botany, Chinese Academy of Sciences, China
Domingos Cardoso, Universidade Federal da Bahia, Brazil
Michael Ryan May, University of California, Berkeley, United States
Maryam Sedaghatpour, University of California, Berkeley, United States, in collaboration with reviewer MR
Copyright © 2020 Duan, Harris, Su, Zhang, Arslan, Ertuğrul, Loc, Hayashi, Wen and Chen. This is an open-access article distributed under the terms of the Creative Commons Attribution License (CC BY). The use, distribution or reproduction in other forums is permitted, provided the original author(s) and the copyright owner(s) are credited and that the original publication in this journal is cited, in accordance with accepted academic practice. No use, distribution or reproduction is permitted which does not comply with these terms.
*Correspondence: Lei Duan, duanlei@scbg.ac.cn; Jun Wen, wenj@si.edu; Hong-Feng Chen, h.f.chen@scbg.ac.cn
†These authors have contributed equally to this work