- 1Plant Biology Department, Southern Illinois University, Carbondale, IL, United States
- 2International Institute of Tropical Forestry, USDA Forest Service, San Juan, PR, United States
Because stomata in bryophytes are uniquely located on sporangia, the physiological and evolutionary constraints placed on bryophyte stomata are fundamentally different from those on leaves of tracheophytes. Although losses of stomata have been documented in mosses, the extent to which this evolutionary process occurred remains relatively unexplored. We initiated this study by plotting the known occurrences of stomata loss and numbers per capsule on the most recent moss phylogeny. From this, we identified 40 families and 74 genera that lack stomata, of which at least 63 are independent losses. No trends in stomata losses or numbers are evident in any direction across moss diversity. Extant taxa in early divergent moss lineages either lack stomata or produce pseudostomata that do not form pores. The earliest land plant macrofossils from 400 ma exhibit similar sporangial morphologies and stomatal distribution to extant mosses, suggesting that the earliest mosses may have possessed and lost stomata as is common in the group. To understand why stomata are expendable in mosses, we conducted comparative anatomical studies on a range of mosses with and without stomata. We compared the anatomy of stomate and astomate taxa and the development of intercellular spaces, including substomatal cavities, across mosses. Two types of intercellular spaces that develop differently are seen in peristomate mosses, those associated with stomata and those that surround the spore sac. Capsule architecture in astomate mosses ranges from solid in the taxa in early divergent lineages to containing an internal space that is directly connected to the conducing tissue and is involved in capsule expansion and the nourishment, hydration and development of spores. This anatomy reveals there are different architectural arrangements of tissues within moss capsules that are equally effective in accomplishing the essential processes of sporogenesis and spore dispersal. Stomata are not foundational to these processes.
Introduction
Stomata in bryophytes are located on sporangia and are restricted in their occurrence across phylogeny. Liverworts are the only extant land plants that lack stomata entirely, while stomata are widespread but not ubiquitous in hornworts and mosses. With contemporary phylogenies pointing to hornworts as the earliest divergent bryophyte group (Puttick et al., 2018; Renzaglia et al., 2018), stomata are best interpreted as plesiomorphic in land plants, especially given that Leiosporoceros, the sister taxon to other hornworts, possesses stomata. Within the small hornwort clade of 10–12 genera there are two well-documented losses of stomata in derived taxa (Renzaglia et al., 2017). In comparison, early diversification of the moss assemblage apparently was not dependent on the existence of stomata as Takakiales and Andreaeopsida, two of the oldest moss clades, are stomata free. Moreover, there are multiple moss orders and families that include taxa with and without stomata. Clearly stomata are not vital to the survival and were not required for the initial radiation of bryophytes.
The sporadic occurrence of stomata in bryophytes calls into question the role stomata play in the physiology and growth of bryophyte sporophytes. Recent studies reveal that diurnal cycles of opening and closing, and responses to ABA and desiccation, which are key to water relations in tracheophytes, do not occur in hornworts (Pressel et al., 2018). However, substomatal cavities and intercellular spaces that are necessary for functional stomata are always present in mosses and hornworts with stomata, while species without stomata do not have substomatal spaces (Goffinet et al., 2009; Merced and Renzaglia, 2017). Intercellular spaces are common in different tissues of land plants, and in some bryophytes are present in both gametophyte and sporophyte generations, suggesting that spaces originated multiple times in the evolution of plants (Duckett and Pressel, 2018). In tracheophytes, intercellular spaces in the form of spongy tissue are coordinated with the presence of functional stomata to facilitate gas exchange (Dow et al., 2017; Lundgren et al., 2019).
In order to better understand the evolution of stomata within mosses, we traced the number per capsule, and known absence of stomata across the range of moss diversity. Due to the lack of stomata in early divergent moss lineages, we examined the fossil record on early land plants for clues to the origin of the moss capsule with and without stomata. We tested the hypothesis that stomata were lost repeatedly throughout the history of mosses and not restricted to derived taxa. We further speculated that stomatal losses were accompanied by anatomical and developmental modification within the sporophyte. Accordingly, we identified architectural features that characterize sporophytes with and without stomata and documented the development of intercellular spaces, including substomatal cavities. Anatomical and developmental analyses identify two distinct types of internal spaces in mosses and document the loss of peripheral spaces strictly associated with guard cells and the retention of internal spaces in taxa without stomata. Our anatomical studies point to modified architectural features that accompanied stomata loss and led to fundamentally different, but equally effective, internal hydration and capsule maturation.
Materials and Methods
Plants and Specimens Examined
Moss capsules were collected locally in Southern Illinois over the growing season to ensure observations of early and late stages of development. Prepared blocks of capsules from species not found in Illinois were sectioned and examined. Species examined include the following, with the seven taxa lacking stomata denoted by asterisks: Takakia ceratophylla∗, Andreaea rothii∗, Sphagnum angustifolium∗, Polytrichastrum ohiensis, Atrichum angustatum∗, Tetraphis pellucida∗, Diphysium foliosum, Buxbaumia viridis, Physcomitrium pomiform, Physcomitrium (Physcomitrella) patens, Funaria hygrometrica, Dicranum scoparium, Orthotrichum pusillum, Plagiomnium cuspidatum, Ephemerum spinosum, Leucobryum glaucum∗, Bartramia pomiforme, Hypnum curvifolium, Brachythecium rutabulum, Thuidium delicatulum, and Neckeropsis undulata.∗ A KNOX mutant of P. patens that lacks stomata was acquired from Dr. Neil Ashton.
Published records of fossils of the earliest land plants with sporangia and stomata were examined for comparisons with the morphology and anatomy of the extant members of early divergent moss lineages. Fossil stomata were reproduced from Edwards (1979) and Edwards et al. (1998) with permission.
Stomata Presence in the Phylogeny of Mosses
We assessed the presence and absence of stomata by mapping their occurrence across the most recent phylogeny of mosses (Liu et al., 2019). An extensive literature review (Table 1) identified genera and species that lack stomata, and confirmed the number of stomata reported for members of each moss family, if known. To determine the minimum number of losses in moss orders, we counted the number of families that have genera that lack stomata and assessed independent origin based on phylogenetic relationships. If a genus has species with both states (present and absent stomata) these were counted as independent losses. Losses within different families were each scored as independent. From these analyses, we estimate the minimum number and, in some cases, maximum number (in parenthesis) of losses for each order (Figure 1).
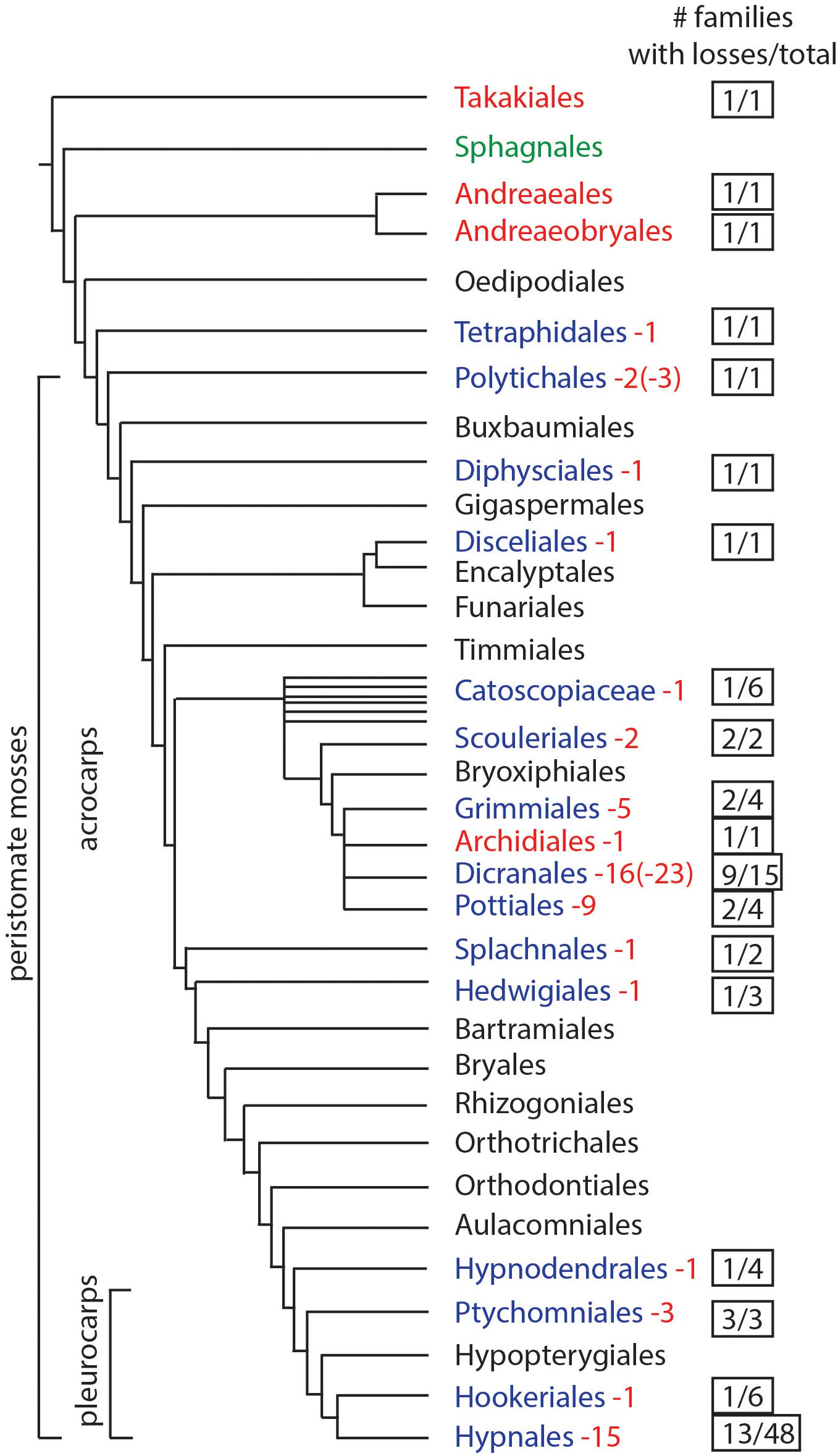
Figure 1. Phylogenetic of moss orders based on Liu et al. (2019). Orders in red lack stomata, green have pseudostomata, black have stomata (no records of losses), and blue have documented losses of stomata. Numbers in red represent the minimum times stomata were lost and numbers in parentheses indicate the maximum possible number of losses. The right column indicates the number of families that include taxa without stomata, over the total number of families in the order.
Light Microscopy, Electron Microscopy, and Immunogold Labeling
Protocols are described in detail in Merced and Renzaglia (2013, 2014). Sporophytes of mosses were fixed in 2% glutaraldehyde in 0.05M NaPO4 buffer, washed three times in 0.05M NaPO4 buffer and post-fixed for 20 min in 1% OsO4 in 0.05M NaPO4 buffer. Specimens were rinsed in distilled water and dehydrated in a graded ethanol series ending with 100% ethanol. For scanning electron microscopy (SEM), fixed capsules were critical point dried and mounted on stubs, then sputter-coated for 230 s with palladium-gold. Specimens were observed using a Hitachi S570 scanning electron microscope. For light microscopy and transmission electron microscopy (TEM), specimens were infiltrated with Spurr’s resin (Electron Microscopy Sciences, Hatfield, PA, United States) or LR White resin (London Resin Company, Berkshire, United Kingdom) and cured at 65°C. For light microscopy, semi-thin sections (250–750 nm) were mounted on glass slides and stained with 1.5% toluidine blue in distilled water. Slides were observed on a Leica DM5000 B compound microscope and images captured digitally. Thin sections (60–90 nm) were collected on nickel grids, incubated with 2% BSA in 0.02M PBS for immunogold labeling. Grids were transferred to the LM19 primary antibody (diluted 1: 20 in 2% BSA/PBS) for 3 h and controls (one grid each treatment) were left in buffer during that time. All grids were rinsed in 2% BSA/PBS, then incubated in goat anti-rat IgG secondary antibody (Sigma- Aldrich, St. Louis, MO, United States) diluted 1: 20 in 2% BSA/PBS for 30 min. Grids were rinsed with PBS followed by distilled/deionized autoclaved filtered water, and dried at room temperature. Grids were observed unstained with a Hitachi H7650 transmission electron microscope at 60 kV.
Results
Stomata Presence and Number in the Phylogeny of Mosses
Based on data mining from published literature, stomata are absent in 74 genera and 40 families of mosses, accounting for at least 63 independent losses in the phylogeny of mosses (Figure 1 and Supplementary Data). Nearly 60% (16 of 28) of the orders of peristomate mosses have recorded losses of stomata. These losses are equally present in acrocarps and pleurocarps with high numbers in the Dicranales, Pottiales, and Hypnales (Figure 1). As the sister taxon to peristomate mosses, Oedipodium represents the earliest divergent moss lineage to possess stomata. Numbers of stomata per capsule range from 0 to 250 (Figure 2 and Table 1), with the vast majority of counts (40 of 54 = 74%) ranging from 3 to 30 (Figures 2C,D). Numbers above 200 are rare and recorded only for three families, Polytrichaceae, Funariaceae (Figure 2B) and Bartramiaceae, although many members of these family have less than 70 stomata (Figures 2C,F) (Table 1). There are no evident trends in numbers in either direction with divergence time. For example, numbers vary in the first moss lineages with stomata: in Oedipodium the 60 or so stomata are scattered along the highly elongated neck and within the Tetraphidaceae, Tetraphis lacks stomata and Tetradontium contains only five per capsule. Members of the Polytrichales exhibit the extremes in stomata numbers per capsule, with 200 and 250 in Polytrichum and zero in three genera, Atrichum, Pogonatum, and Itatalia.
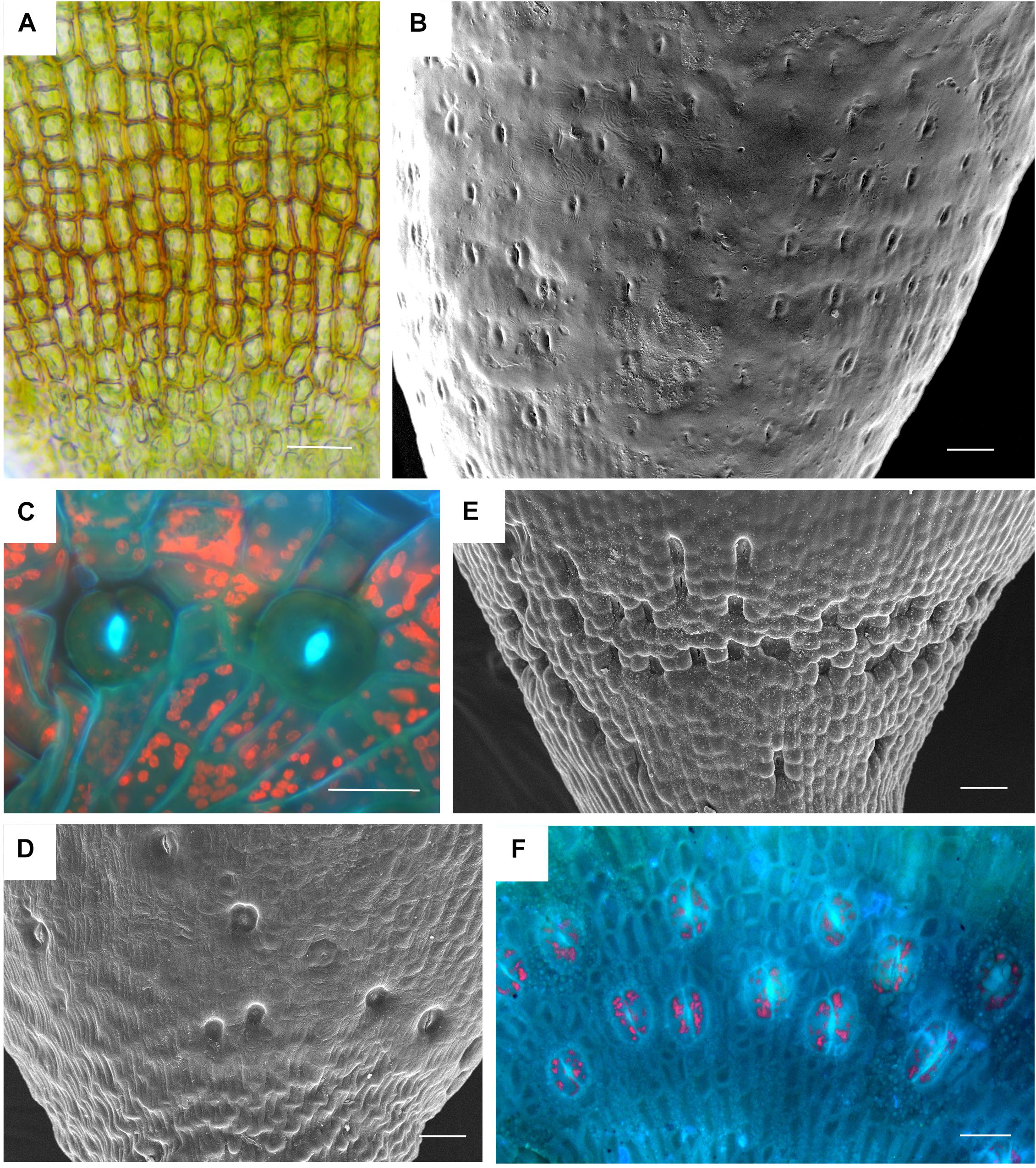
Figure 2. Stomata diversity in mosses. (A) Atrichum angustatum light micrograph of stomata free epidermis. (B) Funaria hygrometrica SEM of apophysis covered with ∼200 stomata. (C) Physcomitrium (Physcomitrella) patens 2 of 10 stomata in fluorescence. (D) Brachythecium rutabulum SEM of sparse scattered stomata. Image credit: Jeffrey J. Duckett. (E) Plagiomnium cuspidatum SEM showing numerous sunken stomata on the apophysis. 60 stomata estimated in the capsule. Image credit: Jeffrey J. Duckett. (F) Bartramia pomiforme group of stomata in fluorescence. 70 stomata estimated in the capsule. Bars: (A,C,F) = 20 μm, (B,D,E) = 50 μm.
Structure of Early Divergent Moss Capsules and Comparisons With Early Fossil Plants
Capsules of extant mosses in early divergent lineages (Takakia and Andreaea) lack stomata or contain over 100 pseudostomata that do not form pores and are evenly dispersed across the capsule epidermis (Sphagnales). These capsules lack apophyses, have prominent central columellae and have solid tissue throughout without air spaces (Figures 3A–C). Sporophyes of Andreaea and Sphagnum have short setae, and are embedded in an elongated pseudopodium of the gametophyte (Figures 3B,C). Comparisons with the oldest fossil plants reveal similar capsule morphology and stomatal arrangement/anatomy as in each of these extant early divergent mosses (Figures 3D–J). Sporangia of Tortilicaulis from the Silurian are spiraled and similar in shape to Takakia (Figures 3D,E). Early Devonian sporangia approximately 400 million years old demonstrate the occurrence of stomata scattered cross sporangia (Figure 3G), resembling the arrangement of pseudostomata in Sphagnum (Figure 3F), and restricted to the base similar to extant mosses (Figure 3H). In section, the stomatal complex of the earliest fossils have guard cells with ledges and substomatal cavities much like those of Oedipodium, the first moss group to possess stomata (Figures 3I,J).
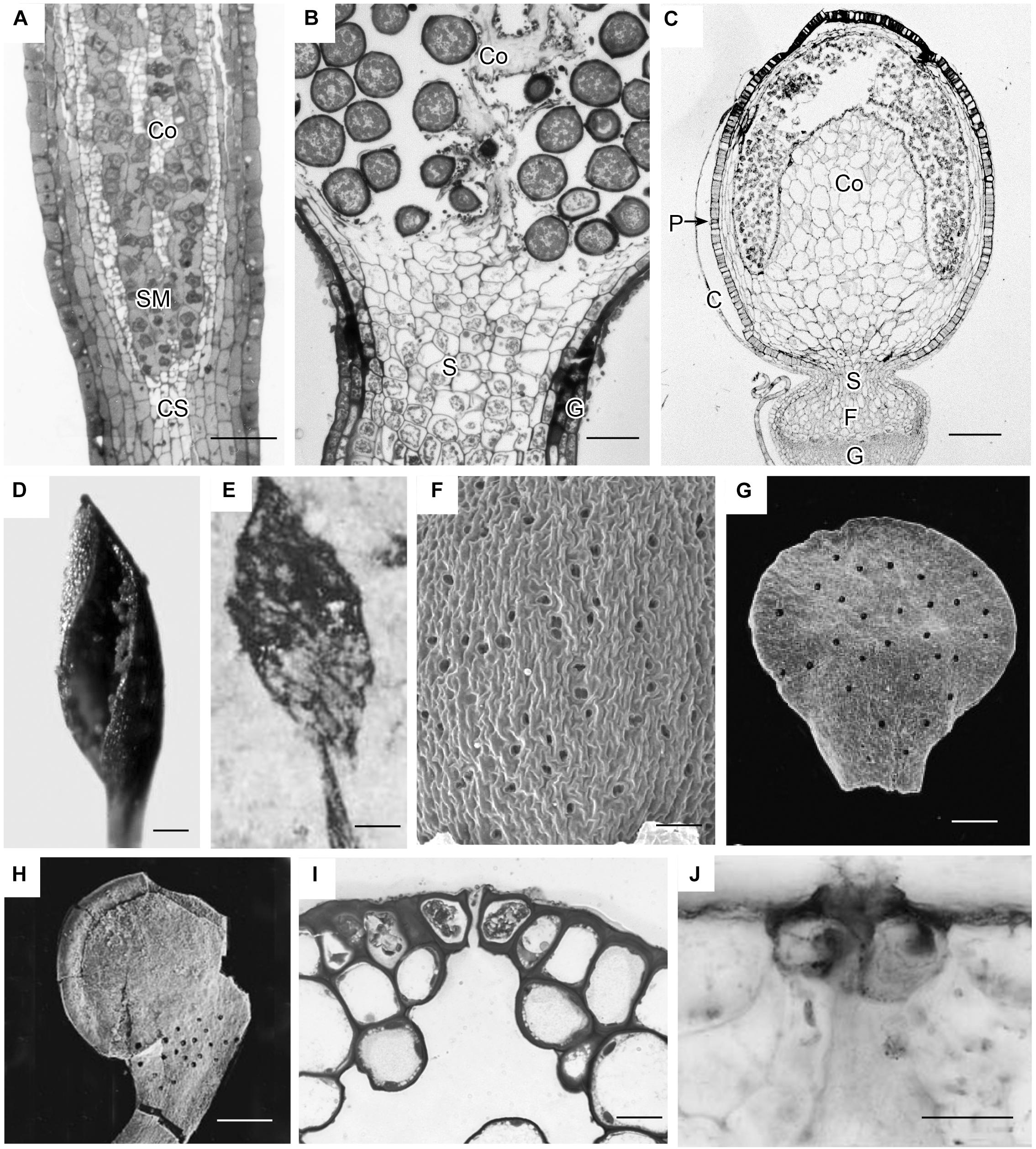
Figure 3. Capsule anatomy, pseudostomata and stomata in extant members of early divergent moss lineages, and sporangia and stomata of the first fossil land plants. (A) Takakia ceratophylla. Light micrograph (LM) longitudinal section of solid cylindrical capsule with spore mother cells (SM), columella (Co) and conducting strand (CS) in seta. (B) Andreaea rothii. LM longitudinal section of solid capsule with spores, columella (Co) and short seta (S) surrounded by gametophyte (G) tissue of the pseudopodium. (C) Sphagnum tenellum. LM longitudinal section of solid capsule, covered by calyptra (C), with pseudostomata (P) in the epidermis, massive columella (Co) covered by the spore sac, and highly reduced seta (S) embedded by foot (F) into gametophyte (G) pseudopodium. (D) Takakia ceratophylla capsule with single spiraled suture and spores. (E) Tortilicaulis transwalliensis capsule from the Silurian resembles Takakia in (D). (F) Sphagnum tenellum SEM showing scattered pseudostomata on dried capsule. (G) Early Devonian bivalved sporangium with scattered stomata (spots). (H) Early Devonian sporangium with band of stomata (spots) at base. (I) Oedipodium LM cross section of neck with guard cells with ledges over substomatal cavity. (J) Aglaophyton major from Rhynie Chert. Cross section of mature axis with stoma showing guard cells with ledges over substomatal cavity. Fossil images reproduced with permission from Journal of Experimental Botany (Edwards et al., 1998) and Paleontology (Edwards, 1979). Bars: (A,E,H) = 100 μm; (B,G,J) = 50 μm; (C,F) = 500 μm; (D)= 200 μm, (I) = 20 μm.
Anatomy of Peristomate Moss Capsules
Members of the Polytrichaceae have well-developed capsule regardless of whether they lack stomata (represented by Atrichum) or contain stomata (represented by Polytrichastrum with 100+ stomata) (Figure 4). In Atrichum the capsule is brown (reddish) when mature and cylindrical, and the short calyptra is situated at the apex (Figure 4A). From the urn down, the neck tapers toward the seta and there is no distinct apophysis. In Polytrichastrum, the capsule is swollen throughout with extensive internal spaces (Figures 4D,E). The distinct apophysis is green with a constriction at the base where the stomata are located. The calyptra covers the capsule up to the constriction throughout development. Side-by-side sections illustrate the arrangement of tissues, including air spaces, in these closely related genera. Atrichum lacks peripheral spaces including substomatal cavities (Figure 4B) that are abundant in Polytrichastrum (Figure 4E). Chloroplasts line cells associated with substomatal cavities (Figure 4G). A large internal air space occurs in Atrichum at the base of the capsule and around the entire spore sac (Figure 4F). This circumsporangial space forms in the young capsule just interior to the solid capsule wall in a zone between the amphithecium and endothecium, the two primary embryonic regions (Figure 4B). In both genera, a well-developed conducting strand of hydroids and leptoids extends in the seta to the spore sac where it ends abruptly and presumably fills the internal space with water and nutrients (Figures 4F,I). Unlike Polytrichastrum that has stomata to draw water toward the outside, the apophysis of Atrichum is covered in a thick cuticle, which retards water loss through the epidermis (Figure 4H). Capsule dehiscence through detachment of the operculum follows drying of liquid in the circumsporangial space and the constriction of the neck at the capsule base (Figure 4C).
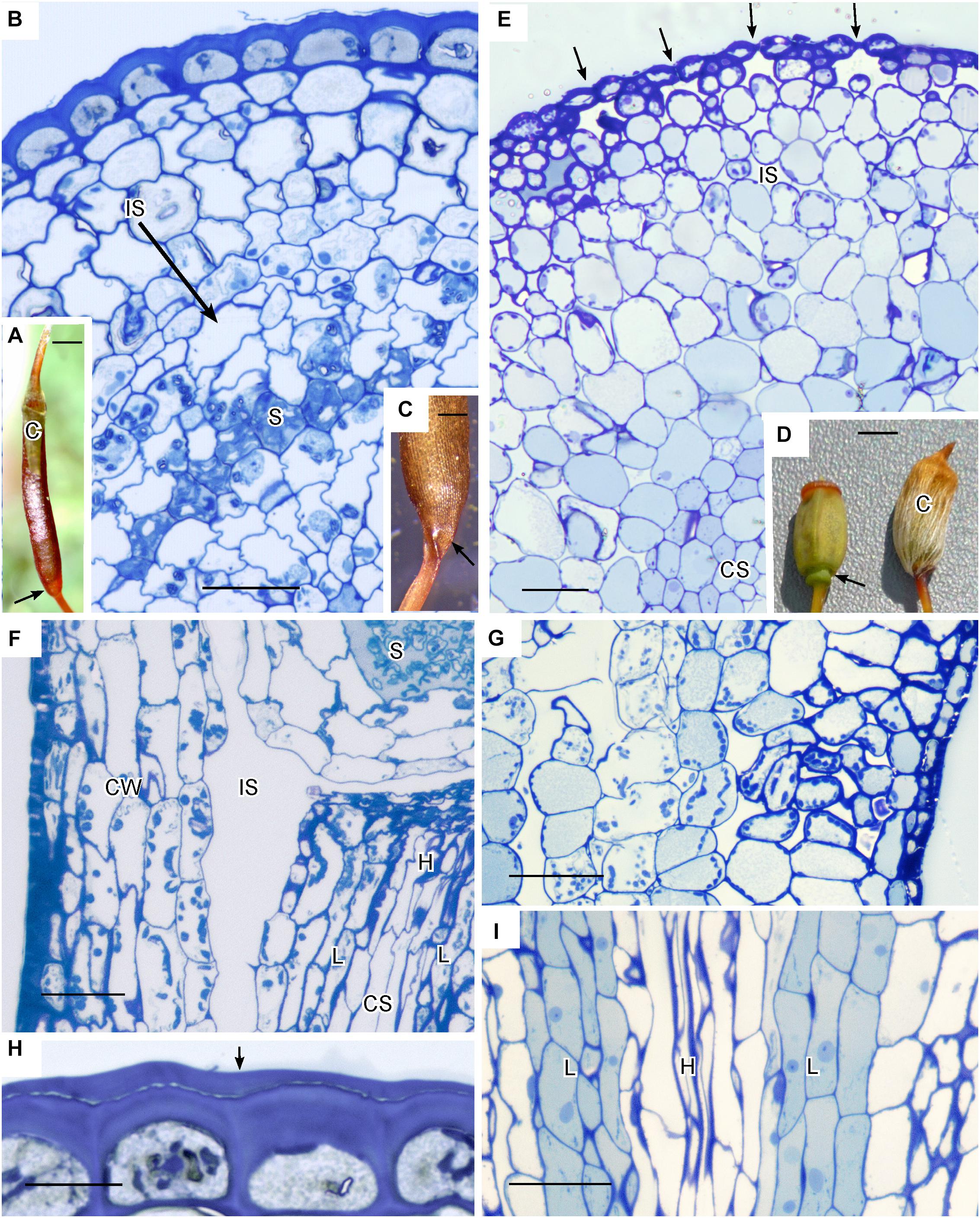
Figure 4. Structure of Polytrichaceae capsule. (A–C,F,H) Atrichum angustatum that lacks stomata in left hand column. (D,E,G,I) Polytrichastrum ohiensis with approximately 100 stomata in right hand column. (A) Long cylindrical red-brown mature Atrichum capsule with inconspicuous calyptra (C) on the top and tapering neck region (arrow) connecting to seta. (B) LM cross section at the capsule urn showing solid capsule wall, developing sporogenous region (S) and circumsporangial space (IS) forming between the capsule wall and spore sac. (C) Base of recently opened Atrichum capsule showing constriction of neck region (arrow) due to drying in circumsporangial cavity and connecting space. (D) Two mature Polytrichastrum capsules, left without calyptra and right covered by calyptra (C). The capsule is wide and green at the base where the calyptra ends and the narrowly constricted area of the apophysis houses stomata (arrow). (E) LM cross section at the constriction with multiple stomata (arrows), subtended by substomatal cavities and associated intercellular spaces (IS), and central conducting strand (CS). (F) LM longitudinal section at the junction between spore sac with spores (S) and neck. A large circumsporangial space (IS) extends just inside the solid capsule wall (CW), along the length of the spore sac and downward into the neck. The conducting strand (CS) of hydroids (H) and leptoids (L) ends abruptly at the circumsporangial space and spore sac. (G) LM longitudinal section at the constriction showing chloroplast rich cells next to spaces associated with substomatal region on the right and the circumsporangial space to the far left. (H) Epidermis with thick walls and cuticle (arrow). (I) Prominent conducting strand in the apophysis with leptoids (L) around hydroids (H). Bars: (A) = 0.5 mm, (B,E–G,I) = 50 μm, (C) = 0.2 mm, (D) = 1.0 mm, (H) = 20 μm.
Types and Development of Intercellular Spaces
Anatomy of capsules with and without stomata reveals two types of intercellular spaces: (1) the substomatal cavity and connected spaces associated with stomata and (2) the circumsporangial cavity that surrounds the spore sac and may extend into the capsule neck and seta (Figures 4, 5, 6). No stomata-lacking capsules have substomatal cavities and associated spaces but all capsules of peristomate mosses examined in this study possess circumsporangial cavities, regardless of whether they have stomata or not (Figures 4F, 5, 6). As illustrated in Atrichum (Figure 4F), Ephemerum (Figure 6A) and Brachythecium (Figure 6B), circumsporangial cavities surround the developing sporogenous tissue and are intimately associated with conducting tissue (when present), which delivers water and food to the developing spores. In some mosses that lack stomata, like Leucobryum, this circumsporangial space is found only during capsule development (Figure 5). The circumsporangial space forms between the embryonic endothecium and amphithecium, prior to the proliferation of sporogenous tissue, and extends the length of the spore sac when the archesporium is a single cell layer (Figures 4A, 5A). When the capsule is fully developed in taxa like Leucobryum, no space is discernible due to capsule expansion and spore differentiation (Figure 5B).
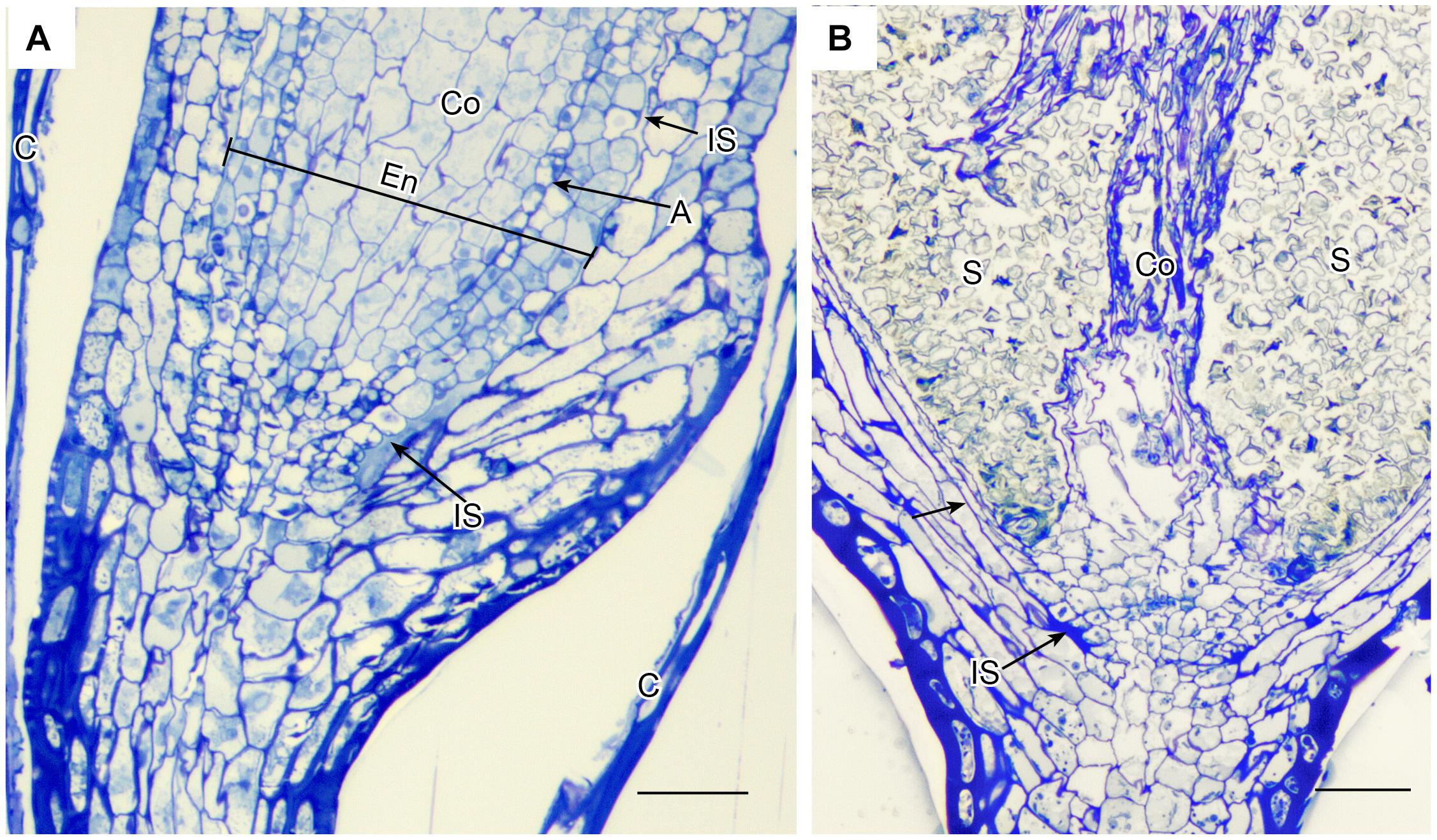
Figure 5. Leucobryum glaucum. LM longitudinal sections of astomate capsule. (A) Base of immature capsule where seta meets the neck covered by calyptra (C). An inconspicuous fluid-filled intercellular space (IS) extends the entire length of the region between the amphithecium that forms the capsule wall, and the endothecium (En) that consists of a prominent columella (Co) and developing spore sac with one layer of archesporium (A) (sporogeneous tissue). (B) Fully expanded capsule. With development of the spore sac that contains 100s of spores (S), the columella (Co) has partially degenerated and the intercellular spaces are closed (arrow) or residual (IS). Bars = 25 μm.
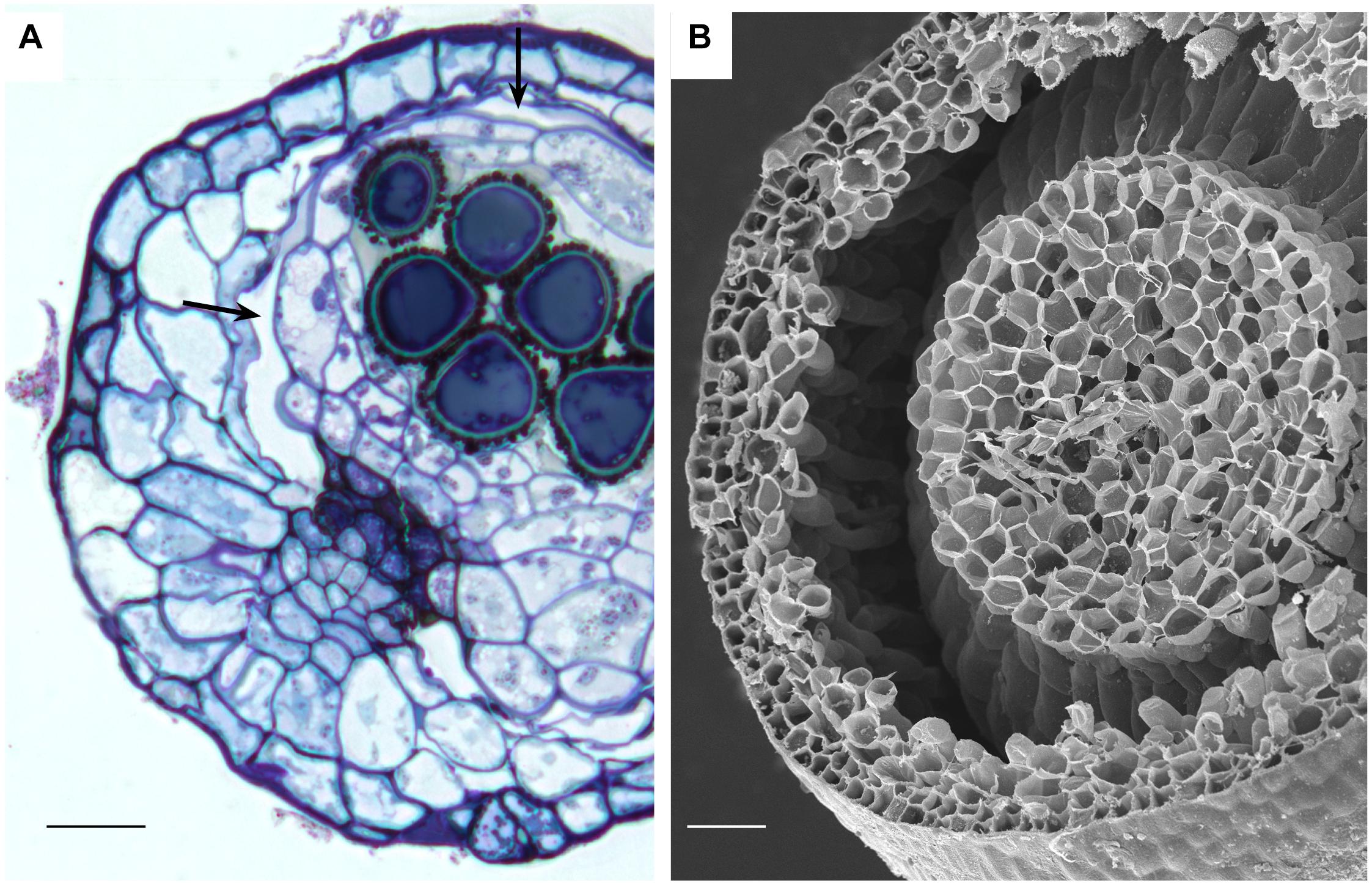
Figure 6. Stomata-containing capsules showing internal circumsporangial space (arrows) that forms between the embryonic endothecium and amphithecium, extends into the neck, and is involved in hydrating and nourishing the spore sac during development. (A) LM Ephemerum. (B) SEM Plagiomnium. Image credit: Jeffrey J. Duckett. Bars: (A) = 35 μm; (B) = 50 μm.
Both types of spaces, substomatal cavities and circumsporangial spaces form in the spear stage just before capsule expansion in mosses with stomata. In taxa with stomata, stomata and liquid-filled substomatal cavities form in the expanding neck or apophysis before the sporogenous tissue develops (Figure 7A). Circumsporangial spaces are not associated with stomata and are found in all mosses during development. They begin with the deposition of an electron-dense fibrillar material (Figure 7B) that abundantly localizes with the monoclonal antibody LM19, which recognizes unesterified homogalacturonas pectin (Figure 7C). When the capsule begins to expand and spaces become larger, the fluid inside the space lacks substructure and no longer localizes with this antibody (Merced and Renzaglia, 2016). Substomatal cavities, in contrast, do not form in the absence of stomata and do not label with LM19 early in development (not shown). Their development is coordinated with differentiation of the guard mother cell and before the division of guard cells and pore opening (Figure 7D). The doughnut shaped guard cell of P. patens has a small round pore (Figure 7E) and a very reduced substomatal cavity (Figure 7F). P. patens sporophytes without stomata have no substomatal cavities but the more internal liquid-filled intercellular spaces are connected to the circumsporangial space and remain throughout development (Figure 7G).
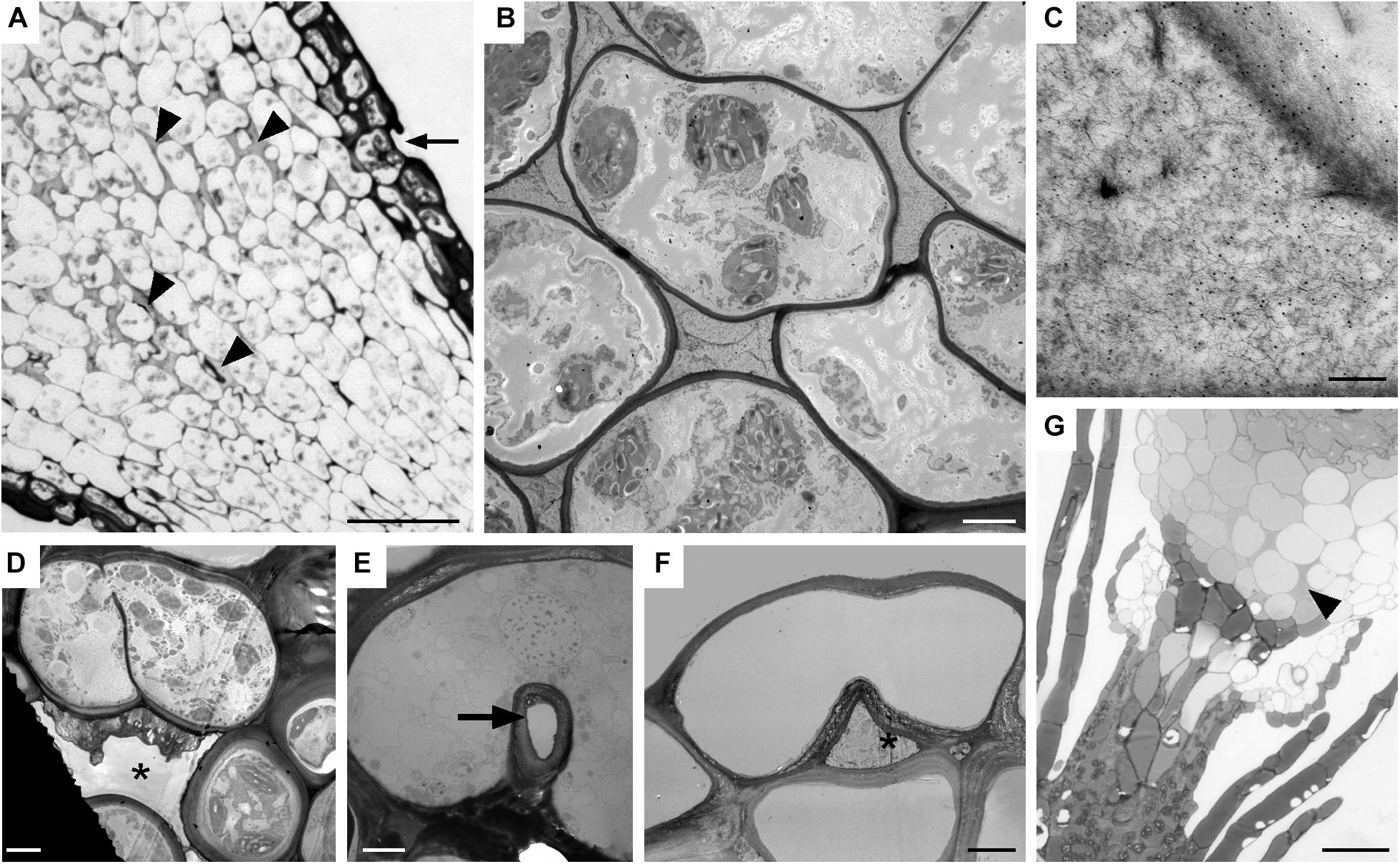
Figure 7. Substomatal cavities and intercellular spaces. (A–D) Dicranum scoparium. (A) LM tangential section of expanding capsule showing stomata (arrow) and associated intercellular spaces are liquid-filled (arrow heads). (B) TEM of circumsporangial space filled with dense filamentous material. (C) Immunogold labeling TEM shows the liquid in the developing circumsporangial space is positive for the LM19 antibody that recognize homogalacturonan pectin (small black dots). (D) Substomatal cavity begins to form before pore opening. (E,F) TEM micrographs of Physcomtrium patens. (E) Small round pore (arrow) of the single-celled stoma. (F) Reduced substomatal cavity∗. (G) LM of liquid-filled intercellular spaces (arrowhead) that are part of the circumsporangial space and not associated with the epidermis of a P. patens class 1 KNOX mutant that lacks stomata. Bars: (A) = 25 μm; (B,D–F) = 4 μm; (C) = 200 nm; (G) = 20 μm.
Discussion
Extant members of early divergent moss lineages entirely lack stomata (Takakiales and Andreaeales) or contain pseudostomata as in Sphagnales. Pseudostomata are pairs of specialized epidermal cells that lack cell wall ledges, do not completely separate to form pores and do not have underlying cavities. They collapse when mature, facilitating drying, capsule dehiscence and spore dispersal, and have been interpreted as either independent from stomata in origin (Duckett et al., 2009) or as modified stomata (Renzaglia et al., 2007; Merced, 2015; Merced and Renzaglia, 2017). Capsule anatomy in these three ancient lineages reflects the absence of pores as intercellular spaces are lacking and the capsule wall and columella are solid throughout. All of these distinctive capsules are erect, lack peristomes, do not contain a distinctive neck or swollen capsule base (apophysis) where stomata are housed, and disperse spores simultaneously with capsule dehiscence through sutures. The early divergent mosses universally lack pore-producing stomata. This includes the Sphagnales that produce high numbers of pseudostomata (100–200 per capsule) that have been interpreted as either independent from stomata in origin (Duckett et al., 2009) or modified stomata (Merced, 2015; Merced and Renzaglia, 2017). Capsule anatomy reflects the absence or pores as intercellular spaces are lacking in Takakia, Andreaea, and Sphagnum and the capsule wall and columella are solid throughout. All of these distinctive capsules are erect, lack peristomes, do not contain a swollen capsule base (apophysis) or distinctive neck where stomata are housed, and disperse spores simultaneously with capsule dehiscence through sutures. Across mosses, the capsules of Sphagnum and Andreaea (and Andreaeobryum not studied here) are uniquely positioned on a gametophytic extension or pseudopodium, not a sporophytic seta, and both generations lack conducting tissues. Takakia resembles other mosses in that gradual seta elongation elevates the capsule and there is a strand of water conducting cells that ends at the capsule base, albeit the cells in the strand are fundamentally different in development, and structure from those of moss hydroids (Renzaglia et al., 1997, 2000, 2007). These unique architectural features preclude comparisons with more derived peristomate mosses and suggest that true stomata evolved after mosses diversified (Duckett and Pressel, 2018). Consequently, we turned to the fossil record for clues as to when in moss evolution stomata evolved.
Because bryophytes exclusively bear stomata on sporangia, we surveyed the literature on the oldest fossil land plants with reference to sporangia and the occurrence, structure and anatomy of stomata. Fossil plants from the Silurian and early Devonian demonstrate that the range of variability in sporangia seen in extant mosses existed approximately 400 million years ago. These earliest fossil sporangia both bore stomata and lacked stomata, e.g., Tortilicaulis, which has a twisted sporangium that is remarkable similar to Takakia (Renzaglia et al., 1997, 2017; Edwards et al., 1998). The oldest fossil sporangia were valvate and contained stomata evenly dispersed on the surface similar to pseudostomata of Sphagnum, or aggregated at the base in a location that is reminiscent of those on moss necks and apophyses. Details of fossil stomata reveal guard cells and internal anatomy similar to that in Oedipodium, the first moss lineage with stomata. Based on the existence of stomata on sporangia in the first plant macrofossils and the similarities with architectural features of early mosses, it is quite possible/likely that stomata existed on moss capsules prior to the diversification of peristomate mosses, which occurred over 100 million years after mosses originated (Newton et al., 2009). Indeed, the estimated median stem age of Takakia and Sphagnum based on the oldest fossil land plants is 465 Ma, while those for Tetraphis and Oedipodium are 309 and 298 Ma, respectively (Laenen et al., 2014). This line of evidence identifies stomata on sporangia that resemble moss capsules when stomata first appeared in the fossil record. Early plant fossils and the high incidence of stomata loss in extant mosses are consistent with the hypothesis that stomata evolved once in bryophytes and were lost repeatedly during diversification, including in early divergent lineages and along the entire moss phylogeny.
Losses of stomata in peristomate mosses are numerous and widespread throughout acrocarps and pleurocarps (Figure 1). Minimally we identify 40 families and 74 genera in which stomata are absent. Of these, 63 are estimated to be independent losses based on phylogenetic relationships. This is a low estimate given the scant record of descriptions and counts of stomata in mosses. Stomata are first seen in the Oedipodiaceae, Tetraphidaceae and Polytrichaceae. The first family includes the single genus Oedipodium, which has the most elongated neck found in any moss and contains approximately 60 stomata (Shaw and Renzaglia, 2004). Both genera in the Tetraphidaceae have erect cylindrical capsules with simple anatomy and minimal neck. Tetrodontium contains five stomata while Tetraphis has none and has an anatomy at the short neck that is devoid of air spaces.
With 3–30 stomata in 74% of moss families (40 of the 54 families based on published counts), stomatal numbers per capsule are relatively low in most mosses. Only 9% of families with counts have more than 100 stomata per capsule. Four families include no members with stomata. Even in the groups with high numbers of stomata there are species with single digit to zero stomata. In general, higher numbers of stomata are found in sporophytes with larger capsules, but capsules devoid of stomata are variable in size (Paton and Pearce, 1957). In the Polytrichaceae, for example, stomata-free capsules of Atrichum and Pogonatum are similar in length to those of Polytrichum, which has up to 250 stomata per capsule (Smith Merrill, 2007). Ecological factors do not explain the absence of stomata either as these taxa often occur side by side along forest floors. In some cases, losses of stomata appear to be associated with capsule reduction. For example, in the Pottiaceae, a transformational series of capsule and seta reduction is associated with high incidences of stomatal losses that have been reported in eight genera (Zander and Eckel, 1993). In other instances, stomatal numbers are relatively low but no known instances of loss have been documented. In the Orthotrichaceae, for example, capsules that are immersed in protective leaves still possess stomata (Merced and Renzaglia, 2017) and cleistocarpic capsules of Ephemerum and P. patens also have stomata (Merced and Renzaglia, 2013). A clear trend is the absence of stomata in aquatic bryophytes, e.g., Fissidens subg. Octodiceras and Fontinalis (Supplementary Data) or semi aquatic taxa when submerged.
Anatomy and development are foundational for understanding plant structure/function relationships and evolution. Our examinations of the internal organization of tissues and their development in capsules confirm that the mosses in early divergent lineages, Takakia, Andreaea and Sphagnaceae, lack any type of intercellular space in the sterile tissue of the capsule, and that peristomate mosses possess intercellular spaces some time in development even if stomata are absent (Duckett and Pressel, 2018). Although these spaces in mosses begin development with the secretion of a fluid-filled matrix, we demonstrate the existence of two distinct types of intercellular spaces in moss capsules. The first is the substomatal cavity associated only with stomata. The second is a circumsporangial space that extends between the spore sac and capsule wall and is involved in capsule expansion during sporogenesis. In many capsules with stomata such as Funaria, circumsporangial spaces extend into the apophysis and eventually connect with substomatal cavities, forming an elaborate system of internal spaces (Merced and Renzaglia, 2016).
We identify different origins for the two types of intercellular spaces in moss capsules. Substomatal cavities begin to develop at the spear stage in concert with guard cell differentiation before sporogenesis. The formation of substomatal cavities involves deposition of a fluid in the cavity that does not localize for pectins, suggesting it is not mucilaginous in nature (Merced and Renzaglia, 2014, 2016). These cavities are necessary for guard cells to separate, develop their unique walls, and for the pore to form. The extent of the system of substomatal cavites and circumsporangial space is related to the size of the capsule or apophysis where stomata are present. This is exemplified in the large capsules of Oedipodium, Funaria, and Polytrichum with extensive interconnected systems of substomatal cavities and underlying intercellular spaces versus the reduced capsules of Ephemerum and P. patens that have small substomatal cavities and a reduced circumsporangial space (Merced and Renzaglia, 2013, 2014, 2016). No mosses without stomata, including stomata free mutants of P. patens, form cavities directly beneath the epidermis that compare with substomatal cavities. Similarly, the absence of substomatal cavities in Sphagnales coincides with the absence of pores in pseudostomata.
In tracheophytes, stomata and intercellular spaces are coordinated throughout development to maximize gas exchange and minimize water lost. The molecular mechanisms controlling air spaces and stomata placement are now being elucidated, and it is hypothesized that feedback signaling between stomata and air spaces influences mesophyll arrangement (Baillie and Fleming, 2020). In mutant wheat plants with arrested stomata, when guard cells fail to divide and do not form a pore, no substomatal cavity is formed (Lundgren et al., 2019). This is similar to what we observed in mosses without stomata, i.e., that substomatal cavities fail to form. The loss of pore formation in Sphagnum and lack of intercellular spaces is consistent with an interpretation that pseudostomata are modified stomata (Merced, 2015).
Unlike substomatal cavities, circumsporangial spaces form in all capsules of peristomate mosses regardless of whether they have stomata or not. This internal space develops with the deposition of fluid that results in an expanding schism between capsule wall and spore sac. As illustrated in the immature Leucobryum and mature Atrichum, Ephemerum and Plagiomnium capsules, the circumsporangial space extends around the entire spore sac, providing a protective and nutritive matrix during spore differentiation. Unlike substomatal cavities, the fluid in this internal space contains pectins as labeled by the LM19 antibody, suggestive of mucilage, and evidence that the two types of spaces are developmentally and genetically independent. The antibody LM19 recognize epitopes of unesterified homogalacturonan, pectin, a polymer found in cell walls of all land plants that is an important component of guard cell walls and mucilage of bryophytes and angiosperms (Merced and Renzaglia, 2014, 2019; Renzaglia et al., 2017).
The separation zone that forms the circumsporangial space is determined in the formative stage of embryogenesis at the time of delineation of the endothecium, which develops into the spore sac plus columella, and amphithecium that forms the capsule wall (Smith, 1955). In comparison, intercellular spaces in hornwort sporophytes are associated with stomata only and are therefore lacking in the two hornwort clades that have lost stomata (Renzaglia et al., 2017). That no circumsporangial space occurs in hornworts is easily explained by the continued and gradual maturation of the cylindrical sporophyte from a basal meristem upward. Unlike in mosses, there is no massive capsule expansion in width in hornworts. Moreover, formative divisions in hornworts do not mimic those in mosses as the amphithecium gives rise to the sporogenous tissue and endothecium, while the endothecium produces only the columella (Renzaglia, 1978). Developmentally there are few similarities between moss and hornwort sporophytes, thus stomata loss is associated with different anatomical modifications in the two bryophyte clades.
The astomate capsule of Atrichum provides abundant clues to the potential role of the internal spaces in moss capsules. In this plant, large spaces remain around and below the spore sac throughout development. These are fluid-filled from their origin and dry following capsule expansion and spore maturation. The circumsporangial spaces are strategically positioned around and above the sporogenous tissue at the region where the central strand of conducting tissue abruptly ends in the neck. The neck in turn consists of tightly packed cells with an epidermis covered by a thick cuticle. Based on this architecture, it is reasonable to deduce that water and dissolved photosynthate that is drawn up to the top of the neck fills the space around the spore sac. In this arrangement, sporogenous tissue is hydrated and provided with a constant source of nutrients. Unlike the neck or apophysis of stomata-containing mosses, there is no potential for a transpirational pull of water up and out of the capsule. Rather, water and solutes are sequestered around the developing spores, and resources are utilized and replenished as needed. This path from source to sink is unidirectional and draws nutrients and water from the gametophyte through the placenta and into the capsule throughout differentiation. The greater loss of water in astomate Atrichum capsules than in stomata bearing taxa as reported by Duckett and Pressel (2018) can be explained by the directed and constant use of water and nutrients in this closed systems. In the final stages of capsule differentiation, the fluid dries in the circumsporangial space, compressing the capsule urn and neck, and resulting in the detachment of the operculum and progressive spore release throughout the season.
A dearth in developmental and structural studies of moss capsules has limited comparisons across the group, making the role of specific anatomical structures in capsule function difficult to interpret. For example, there are many genera for which stomata occurrence and counts are not recorded. The existence and arrangement of key tissues such as conducting tissue are not adequately documented. Consequently, it is not verified but only speculated that hydroids occur in most moss setae (Hébant, 1977). There are mosses such as Orthotrichum that possess stomata but do not have conducting tissue in the sporophyte. Grimmia, in contrast, has been reported to have conducting tissue in the gametophyte but none in the sporophyte, while Buxbaumia has hydroids but no leptoids solely in the sporophyte. How these anatomical differences impact nutrient movement and capsule function are in need of further studied.
Coupled with our morphological and anatomical observations, recent studies on physiology and genetics are providing a comprehensive picture of function and evolution of stomata in bryophytes (Chater et al., 2017). Moss and hornwort stomata do not respond to environmental and endogenous cues including light intensity, water status, abscisic acid, plasmolysis, and physical damage as do angiosperm stomata (Pressel et al., 2018). In bryophytes there are no mechanisms for stomatal pores to open and close and ion changes are the same in all epidermal cells (Sussmilch et al., 2019). The preponderance of recent evidence suggests that stomata play a strategic role in capsule maturation, drying, and dehiscence without any active regulation of water loss.
The function of moss capsules in nourishing, hydrating, protecting, and dispersing spores occurs regardless of whether stomata are present. Stomata have been eliminated in over 60 moss genera/lineages in capsules that are highly modified in anatomy compared with their stomata-bearing relatives. The repeated and numerous evolutionary events that reduced and eliminated stomata on moss capsules point to the fact that unlike in tracheophytes where stomata loss is rare and restricted in occurrence (Keeley et al., 1984; Woodward, 1998), stomata are not necessary for mosses. The loss of stomata has no major consequences for the physiology of the sporophyte but results in delayed maturation and dispersion of spores in stomata-less mutants of P. patens (Chater et al., 2016, 2017). Capsule architecture in mosses without stomata ranges from solid in taxa in early divergent lineages to containing an internal circumsporangial space that is directly connected to the conducing tissue and is involved in capsule expansion and the nourishment, hydration and development of spores. This anatomy reveals there are different architectural arrangements of tissues within moss capsules that are equally effective in accomplishing the essential processes of sporogenesis and spore dispersal. Stomata are not foundational to these processes.
Data Availability Statement
All datasets generated for this study are included in the article/Supplementary Material.
Author Contributions
KR designed the study, conducted anatomical studies, prepared the figures, analyzed the data, and wrote the manuscript. AM conducted ultrastructural studies/immunogold labeling, generated the phylogenetic tree and assisted in preparing the figures and writing the manuscript. WB assisted with generating the phylogenetic tree, conducted literature searches, compiled data tables, and assisted in anatomical studies. All authors read and approved the manuscript.
Funding
This work was supported by grants from the National Science Foundation (NSF 1758497) and the National Institutes of Health (NIH 5R25GM107760-07).
Conflict of Interest
The authors declare that the research was conducted in the absence of any commercial or financial relationships that could be construed as a potential conflict of interest.
Acknowledgments
We thank Juan Larraín, Heinjo During, Richard Zander, Ida Bruggeman, Brent Mishler, Bernard Goffinet, and Jeffrey Duckett for providing information, publications and micrographs on stomata in obscure and diverse moss taxa.
Supplementary Material
The Supplementary Material for this article can be found online at: https://www.frontiersin.org/articles/10.3389/fpls.2020.00567/full#supplementary-material
References
Abella, L., Alcalde, M., Estébanez, B., Cortella, A., Alfayate, C., and Ron, E. (1999). Observations on the stomatal complex in ten species of mosses (Pottiaceae, Bryopsida). J. Hattori Bot. Lab. 86, 179–185. doi: 10.18968/jhbl.86.0_179
Allen, B. (2015). “Fontinalaceae,” in Flora of North America North of Mexico, Vol. 28, ed. Flora of North America Editorial Committee (New York, NY: Oxford University Press), 489–494.
Andreas, B. K. (2013). A revision of Blindia (Seligeriaceae) from Southern South America. Bryol. 116, 263–280. doi: 10.1639/0007-2745-116.3.263
Arzeni, C. B. (1954). The Pterobryaceae of the Southern United States, Mexico, Central America, and the West Indies. Am. Midl. Nat. 52, 1–67.
Baillie, A. L., and Fleming, A. J. (2020). The developmental relationship between stomata and mesophyll airspace. New Phytol. 225, 1120–1126. doi: 10.1111/nph.16341
Bartlett, J. K., and Vitt, D. H. (1986). A survey of species in the genus Blindia (Bryopsida, Seligeriaceae). N. Z. J. Bot. 24, 203–246. doi: 10.1080/0028825X.1986.10412674
Bryan, V. S. (2007). “Ephemeraceae,” in Flora of North America North of Mexico, Vol. 2, ed. Flora of North America Editorial Committee (New York, NY: Oxford University Press), 646–649.
Buck, W. R. (1981). A re-interpretation of the Fabroniaceae, III: anacamptodon and Fabronidium revisited, Mamillariella, Helicodontiadelphus and Bryobartlettia gen. nov. Brittonia 33, 473–481.
Chater, C. C., Caine, R. S., Fleming, A. J., and Gray, J. E. (2017). Origins and evolution of stomatal development. Plant Phys. 174, 624–638.
Chater, C. C., Caine, R. S., Tomek, M., Wallace, S., Kamisugi, Y., Cuming, A. C., et al. (2016). Origin and function of stomata in the moss Physcomitrella patens. Nat. Plants 2, 1–7.
Cheney, L. S. (1897). North American species of Amblystegium. Bot. Gazette 24, 236–291. doi: 10.1086/327591
Crum, H. A., and Anderson, L. E. (1981). Mosses of Eastern North America. New York, NY: Columbia University Press.
Dow, G. J., Berry, J. A., and Bergmann, D. C. (2017). Disruption of stomatal lineage signaling or transcriptional regulators has differential effects on mesophyll development, but maintains coordination of gas exchange. New Phytol. 216, 69–75. doi: 10.1111/nph.14746
Duckett, J. G., and Pressel, S. (2018). The evolution of the stomatal apparatus: intercellular spaces and sporophyte water relations in bryophytes—two ignored dimensions. Philos. Trans. R. Soc. Lond. B Biol. Sci. 373:20160498.
Duckett, J. G., Pressel, S., P’ng, K. M., and Renzaglia, K. S. (2009). Exploding a myth: the capsule dehiscence mechanism and the function of pseudostomata in Sphagnum. New Phytol. 183, 1053–1063. doi: 10.1111/j.1469-8137.2009.02905.x
During, H. J. (1977). A taxonomical Revision of the Garovaglioideae (Pterobryaceae, Musci). thesis, Bryophytorum Bibliotheca12, J. Cramer Verlag, Vaduz.
Edwards, D. (1979). A late Silurian flora from the lower old red sandstone of south-west dyfed. Palaeontology 22, 23–52.
Edwards, D., Kerp, H., and Hass, H. (1998). Stomata in early land plants: an anatomical and ecophysiological approach. J. Exp. Bot. 49, 255–278.
Fife, A. J. (2012). New taxa of Sematophyllum and Wijkia (Musci:Sematophyllaceae), with a key to New Zealand Sematophyllaceae. N. Z. J. Bot. 50, 435–447. doi: 10.1080/0028825X.2012.728993
Fife, A. J., and Dalton, P. J. (2005). A reconsideration of Pleurophascum (Musci: Pleurophascaceae) and specific status for a New Zealand endemic, Pleurophascum ovalifolium stat. et nom. nov. N. Z. J. Bot. 43, 871–884. doi: 10.1080/0028825X.2005.9512997
Goffinet, B. (2012). Australian Mosses Online. 53. Splachnaceae. Avaliable online at: http://www.anbg.gov.au/abrs/Mosses_online/Splachnaceae.pdf
Goffinet, B., Buck, W. R., and Shaw, A. J. (2009). “Morphology, anatomy and classification of the Bryophyta,” in Bryophyte Biology, eds B. Goffinet and A. J. Shaw, (Cambridge, MA: Cambridge University Press), 55–138.
Gradstein, S. R., Churchill, S. P., and Salazar-Allen, N. (2001). Guide to the Bryophytes of Tropical America. The Bronx, NY: Memoirs New York Botanical Garden.
Hastings, R. I., and Grevens, H. C. (2007). “Grimmia,” in Flora of North America North of Mexico, Vol. 27, ed. Flora of North America Editorial Committee (New York, NY: Oxford University Press), 225–257.
Hattaway, R. A. (1984). A Monograph of the Ptychomniaceae (Bryopsida). Ph.D. dissertation, Pennsylvania State University, University Park, PA.
Hedenäs, L. (2005). Bryophyte flora of Uganda. 4. Rhytidiaceae, Hylocomiaceae and Hypnaceae (Part 1). J. Bryol. 27, 55–66. doi: 10.1179/174328205x40734
Ireland, R. (2015). “Plagiotheciaceae,” in Flora of North America North of Mexico, Vol. 28, ed. Flora of North America Editorial Committee (New York, NY: Oxford University Press), 483–488.
Jennings, O. E. (1913). A Manual of the Mosses of Western Pennsylvania. Mosses, PA: Press of the City Mission Pub. Co., doi: 10.5962/bhl.title.54494
Keeley, J. E., Osmond, C. B., and Raven, J. A. (1984). Stylites, a vascular land plant without stomata absorbs CO2 via its roots. Nature 310, 694–695.
Klazenga, N. (2012a). Australian Mosses Online. 28. Orthorrhynchiaceae. Avaliable online at: http://www.anbg.gov.au/abrs/Mosses_online/Orthorrhynchiaceae.pdf
Klazenga, N. (2012b). Australian Mosses Online. 29. Pterigynandraceae. Avaliable online at: http://www.anbg.gov.au/abrs/Mosses_online/Pterigynandraceae.pdf
Laenen, B., Shaw, B., Schneider, H., Goffinet, B., Paradis, E., Désamoré, A., et al. (2014). Extant diversity of bryophytes emerged from successive post-Mesozoic diversification bursts. Nat. Commun. 5:5134.
Liu, Y., Johnson, M. G., Cox, C. J., Medina, R., Devos, N., Vanderpoorten, A., et al. (2019). Resolution of the ordinal phylogeny of mosses using targeted exons from organellar and nuclear genomes. Nat. Commun. 10:1485. doi: 10.1038/s41467-019-09454-w
Lundgren, M. R., Mathers, A., Baillie, A. L., Dunn, J., Wilson, M. J., Hunt, L., et al. (2019). Mesophyll porosity is modulated by the presence of functional stomata. Nat. Commun. 10:2825.
McIntosh, T. T. (2007). “Schistidium,” in Flora of North America North of Mexico, Vol. 27, ed. Flora of North America Editorial Committee (New York, NY: Oxford University Press), 207–224.
Merced, A. (2015). Novel insights on the structure and composition of pseudostomata of Sphagnum. Am. J. Bot. 102, 329–335.
Merced, A., and Renzaglia, K. (2014). Developmental changes in guard cell wall structure and pectin composition in the moss Funaria: implications for function and evolution of stomata. Ann. Bot. 114, 1001–1010. doi: 10.1093/aob/mcu165
Merced, A., and Renzaglia, K. S. (2013). Moss stomata in highly elaborated Oedipodium (Oedipodiaceae) and highly reduced Ephemerum (Pottiaceae) sporophytes are remarkably similar. Am. J. Bot. 100, 2318–2327. doi: 10.3732/ajb.1300214
Merced, A., and Renzaglia, K. S. (2016). Patterning of stomata in the moss Funaria: a simple way to space guard cells. Ann. Bot. 117, 985–994. doi: 10.1093/aob/mcw029
Merced, A., and Renzaglia, K. S. (2017). Structure, function and evolution of stomata from a bryological perspective. Bryophys. Divers. Evol. 39, 7–20.
Merced, A., and Renzaglia, K. S. (2019). Contrasting pectin polymers in guard cell walls of Arabidopsis and the hornwort Phaeoceros reflect physiological differences. Ann. Bot. 123, 579–585.
Milne, J., and Klazenga, N. (2012). Australian Mosses Online. 24. Entodontaceae. Avaliable online at: http://www.anbg.gov.au/abrs/Mosses_Online/Entodontaceae.pdf
Newton, A. E., Wikström, N., Shaw, A. J., Hedges, S. B., and Kumar, S. (2009). Mosses (Bryophyta). The Time Tree of Life. New York: Oxford University Press, 138–145.
Paton, J. A., and Pearce, J. V. (1957). The occurrence, structure and functions of the stomata in British bryophytes. Trans. Br. Bryol. Soc. 3, 228–259. doi: 10.1179/006813857804829560
Pressel, S., Renzaglia, K. S., Clymo, R. S., and Duckett, J. G. (2018). Hornwort stomata do not respond actively to exogenous and environmental cues. Ann. Bot. 122, 45–57. doi: 10.1093/aob/mcy045
Puttick, M. N., Morris, J. L., Williams, T. A., Cox, C. J., Edwards, D., Kenrick, P., et al. (2018). The interrelationships of land plants and the nature of the ancestral embryophyte. Curr. Biol. 28, 733–745. doi: 10.1016/j.cub.2018.01.063
Pursell, R. A. (1987). A taxonomic revision of Fissidens subgenus Octodiceras (Fissidentaceae). Mem N. Y. Bot. Gard. 45, 639–660.
Pursell, R. A. (2007). “Fissidentaceae,” in Flora of North America North of Mexico, Vol. 27, ed. Flora of North America Editorial Committee (New York, NY: Oxford University Press), 331–357.
Pursell, R. A., Bruggeman-Nannenga, M. A., and Allen, B. H. (1988). A taxonomic revision of Fissidens subgenus Sarawakia (Bryopsidae: Fissidentacaea). Bryologist 91, 202–213.
Renzaglia, K. S. (1978). A comparative morphology and developmental anatomy of the Anthocerotophyta. J. Hattori Bot. Lab. 44, 31–90.
Renzaglia, K. S., Duff, R. J., Nickrent, D. L., and Garbary, D. J. (2000). Vegetative and reproductive innovations of early land plants: implications for a unified phylogeny. Philos. Trans. R. Soc. Lond. B Biol. Sci. 355, 769–793.
Renzaglia, K. S., McFarland, K. D., and Smith, D. K. (1997). Anatomy and ultrastructure of the sporophyte of Takakia ceratophylla (Bryophyta). Am. J. of Bot. 84, 1337–1350.
Renzaglia, K. S., Schuette, S., Duff, R. J., Ligrone, R., Shaw, A. J., Mishler, B. D., et al. (2007). Bryophyte phylogeny: advancing the molecular and morphological frontiers. Bryologist 110, 179–213.
Renzaglia, K. S., Villareal Aguilar, J. C., and Garbary, D. J. (2018). Morphology supports the setaphyte hypothesis: mosses plus liverworts form a natural group. Bryophys. Divers. Evol. 40, 11–17.
Renzaglia, K. S., Villarreal, J. C., Piatkowski, B. T., Lucas, J. R., and Merced, A. (2017). Hornwort stomata: architecture and fate shared with 400-million-year-old fossil plants without leaves. Plant Physiol. 174, 788–797. doi: 10.1104/pp.17.00156
Schofield, W. B. (2007). “Diphysciaceae,” in Flora of North America North of Mexico, Vol. 27, ed. Flora of North America Editorial Committee (New York, NY: Oxford University Press), 162–164.
Shaw, A. J., and Renzaglia, K. S. (2004). Phylogeny and diversification of bryophytes. Am. J. Bot. 91, 1557–1581. doi: 10.3732/ajb.91.10.1557
Smith, G. M. (1955). Cryptogamic Botany: Vol. II Bryophytes and Pteridophytes. New York, NY: McGraw-Hill Book Company.
Smith Merrill, G. L. (2007). “Polytrichaceae,” in Flora of North America North of Mexico, ed. Flora of North America Editorial Committee, (New York, NY: Oxford University Press), 121–160.
Spence, J. R. (2015). “Leskeaceae,” in Flora of North America North of Mexico, Vol. 28, ed. Flora of North America Editorial Committee (New York, NY: Oxford University Press), 340–361.
Stark, L. (2015). “Leptodontaceae,” in Flora of North America North of Mexico, Vol. 28, ed. Flora of North America Editorial Committee (New York, NY: Oxford University Press), 623–627.
Sussmilch, F. C., Roelfsema, M. R. G., and Hedrich, R. (2019). On the origins of osmotically driven stomatal movements. New Phyt. 222, 84–90.
Tong, C., and He, S. (2002). “Ditrichaceae,” in Moss Flora of China, Vol. 1, eds Peng-cheng, W. Crosby and S. He (St. Louis, MO: Missouri Botanical Garden Press), 58–80.
Vitt, D. H. (2007). “Drummondia,” in Flora of North America North of Mexico, Vol. 27, ed. Flora of North America Editorial Committee (New York, NY: Oxford University Press), 40–41.
Vitt, D. H., and Buck, W. R. (1984). The familial placement of Bryowijkia (Musci: Trachypodaceae). Brittonia 36, 300–306. doi: 10.2307/2806531
Yu, N.-N., and Jia, Y. (2012). The taxonomic status of two species of Calyptothecium Mitt. (Pterobryaceae, Bryopsida). J. Bryol. 34, 63–65. doi: 10.1179/1743282011Y.0000000044
Keywords: stomata, mosses, guard cells, intercellular space, capsule, land plant evolution
Citation: Renzaglia KS, Browning WB and Merced A (2020) With Over 60 Independent Losses, Stomata Are Expendable in Mosses. Front. Plant Sci. 11:567. doi: 10.3389/fpls.2020.00567
Received: 20 February 2020; Accepted: 16 April 2020;
Published: 28 May 2020.
Edited by:
Michael T. Raissig, Heidelberg University, GermanyReviewed by:
Paula Rudall, Royal Botanic Gardens, Kew, United KingdomSilvia Pressel, Natural History Museum, United Kingdom
Copyright © 2020 Renzaglia, Browning and Merced. This is an open-access article distributed under the terms of the Creative Commons Attribution License (CC BY). The use, distribution or reproduction in other forums is permitted, provided the original author(s) and the copyright owner(s) are credited and that the original publication in this journal is cited, in accordance with accepted academic practice. No use, distribution or reproduction is permitted which does not comply with these terms.
*Correspondence: Karen S. Renzaglia, renzaglia@siu.edu