- 1Photosynthesis Laboratory, Department of Horticulture, Aburaihan Campus, University of Tehran, Tehran, Iran
- 2Faculty of Natural Sciences, Institute of Horticultural Production Systems, Floriculture, Leibniz University Hannover, Hannover, Germany
- 3Wageningen Food & Biobased Research, Wageningen, Netherlands
- 4Horticulture and Product Physiology, Wageningen University & Research, Wageningen, Netherlands
- 5Institute of Environment and Sustainable Development in Agriculture, Chinese Academy of Agricultural Sciences, Beijing, China
Improving marketability and extension of vase life of cut flowers has practical significance for the development of the cut flower industry. Although considerable efforts have been made over many years to improve the vase life of cut flowers through controlling the immediate environment and through post-harvest use of floral preservatives, the impact of lighting environment on vase life has been largely overlooked. In the current study, the effect of three LED light spectra [white (400–730 nm), blue (peak at 460 nm), and red (peak at 660 nm)] at 150 μmol m–2 s–1 on vase life and on physiological and biochemical characteristics of carnation cut flowers was investigated. Exposure to blue light (BL) considerably delayed senescence and improved vase life over that of flowers exposed to red light (RL) and white light (WL). H2O2 and malondialdehyde (MDA) contents in petals gradually increased during vase life; the increase was lowest in BL-exposed flowers. As a consequence, BL-exposed flowers maintained a higher membrane stability index (MSI) compared to RL- and WL-exposed flowers. A higher activity of antioxidant enzymes [superoxide dismutase (SOD), peroxidase (POD), catalase (CAT), and ascorbate peroxidase (APX)] was detected in petals of BL-exposed flowers, compared to their activities in RL- and WL-exposed flowers. In BL-exposed flowers, the decline in petal carotenoid contents was delayed in comparison to RL- and WL-exposed flowers. Maximum quantum efficiency of photosystem II (Fv/Fm) and a higher percentage of open stomata were observed in leaves of BL-exposed flowers. Sucrose and glucose contents accumulated in petals during vase life; sugar concentrations were higher in BL-exposed flowers than in RL- and WL-exposed flowers. It is concluded that BL exposure improves the vase life of carnation cut flowers through its effect on the antioxidant defense system in petals and on photosynthetic performance in the leaves.
Introduction
Ornamental plant production is an expanding industry worldwide and has great potential for continued future growth in international markets (Jerzy et al., 2011). However, cut flowers generally have a short vase life depending on genetic and environmental factors, and this often limits development of the industry (Kumar et al., 2014; Van Meeteren and Aliniaeifard, 2016). Carnation (Dianthus caryophyllus L.) is one of the most popular and important of cut flowers for the ornamental industry, also useful as an ornamental model plant, for which the genome has been sequenced (Karami et al., 2008; Yagi et al., 2013). Normally carnations have a short vase life of around 5–10 days depending on the cultivar.
Post-harvest senescence of cut flowers is an active process involving physiological and biochemical changes (Buchanan-Wollaston and Morris, 2000; Rubinstein, 2000; Battelli et al., 2011), and is regulated by a cell death program (Arora and Singh, 2006; Van Doorn and Woltering, 2008; Wagstaff et al., 2009; Van Doorn, 2011). Physiological and biochemical aspects of carnation senescence have previously been described (Sugawara et al., 2002; Shibuya and Ichimura, 2010; Satoh, 2011), and conditions during growth of mother plants, storage and handling, environment, and phyto-hormones all play roles in senescence regulation (Karimi et al., 2012; Asil et al., 2013; Hotta et al., 2016). Particularly since it is a model flower, the mechanisms involved in vase life determination have attracted much interest (Sugawara et al., 2002; Tanase et al., 2008; Satoh, 2011; Tanase et al., 2015).
In recent years considerable efforts have been made to improve vase life of cut flowers through controlling the post-harvest environment; however, the influence of lighting environment on vase life has largely been overlooked. Light is one of the most influential and versatile of environmental stimuli controlling plant life from seed germination to plant senescence (Rodrigues et al., 2014). Some studies have shown that light delays senescence during post-harvest storage of some horticultural products such as basil (Costa et al., 2013), broccoli (Zhan et al., 2012), spinach (Lester et al., 2010), mushrooms (Oms-Oliu et al., 2010), and cabbage (Perrin, 1982). However, both negative and positive effects of different light spectra on the post-harvest longevity of horticultural products such as tomato, asparagus, strawberry, broccoli, peach, lettuce, and pot Chrysanthemum have been reported (Jerzy et al., 2011; Dhakal and Baek, 2014; Woltering and Seifu, 2014; Xu et al., 2014; Gong et al., 2015; Hasperué et al., 2016a, b; Mastropasqua et al., 2016). To date, the effects of light spectra on post-harvest performance of cut flowers have not been studied in detail and the mechanisms underlying the responses to different spectra remain largely unknown.
Photosynthesis is the main process providing energy input for growth and development. Previous studies have reported that plants grown under blue light (BL) are characterized by higher photosynthetic electron transport and chlorophyll a/b ratios than plants grown under red light (RL) (Eskins et al., 1991). Zheng and Van Labeke (2017a,b), demonstrated that BL resulted in the highest maximum quantum efficiency (ΦPSII) and quantum yield (Fv/Fm) in Cordyline australis, Ficus benjamina, Sinningia speciosa, and Chrysanthemum morifolium. However, knowledge about the effects of the lighting environment on photosynthesis of leaves of cut flowers and the subsequent importance of photosynthetic performance on vase life is largely unknown.
Oxidative stress plays a key role in cut flower senescence. Several reports have shown that different light spectra strongly affect the activity of antioxidant systems in different plant species such as Solanum lycopersicum (Kim et al., 2013), Kalanchoe pinnata (Nascimento et al., 2013), Fragaria ananassa (Xu et al., 2014), Brassica oleracea (Deng et al., 2017), and Camptotheca acuminata (Yu et al., 2017). These studies revealed that an enhanced antioxidant system improves post-harvest longevity of these products (Xu et al., 2014; Deng et al., 2017). Therefore, studying the antioxidant defense system during post-harvest assessment of horticultural products will help advance our knowledge of the regulatory role of environmental cues on the vase life of cut flowers.
Only one report, Jerzy et al. (2011), studied the effects of different light spectra on post-harvest longevity of pot Chrysanthemum, and the literature concerning the effect of light spectra on cut flower vase life, and the underlying response mechanisms, are scarce. Therefore, the purpose of this study was to explore the effects of light spectra on the vase life of carnation flowers and to investigate the underlying mechanisms determining their vase life.
Materials and Methods
Plant Materials and Growth Conditions
Cut flowers of a standard type of carnation (Dianthus caryophyllus cv. ‘Moon light’) (40–45 cm in length) were obtained from a commercial greenhouse in the early morning. Healthy flowers were harvested at the commercial stage of flower development, described as ‘fully open,’ when the outer petals were reflected at right angles to the pedicel and the inner ones were relatively small and immature (Nichols and Ho, 1975). The stems were placed in tap water and the end of each stem was re-cut under water to 30 cm to allow for water uptake by the vessel elements of the stem. Thereafter, 12 replicates per treatment were randomly allocated to three spectral light treatments. Air temperature of the test room was set at 21 ± 2°C and flowers received a photoperiod of 12/12 h light/dark cycles.
Light Treatments
For applying light treatments, LED modules (Iran grow light, Tehran, Iran) were placed in the top of chambers (120 cm × 90 cm × 80 cm). Three light spectra consisting of red (R; peak at 660 nm), blue (B; peak at 460 nm), and white (W; 400–730 nm) were used in the chambers without any other illumination sources. Light intensity at the flower level was set at 150 μmol m–2 s–1 by adjusting the distance of the light source. Light spectral distribution was measured using a Sekonic C7000 SpectroMaster spectrometer (Sekonic, Corp., Tokyo, Japan) in the range of 300–800 nm (Figure 1) and uniformity was verified by measuring the light intensity at five points of each light treatment at the flower level.
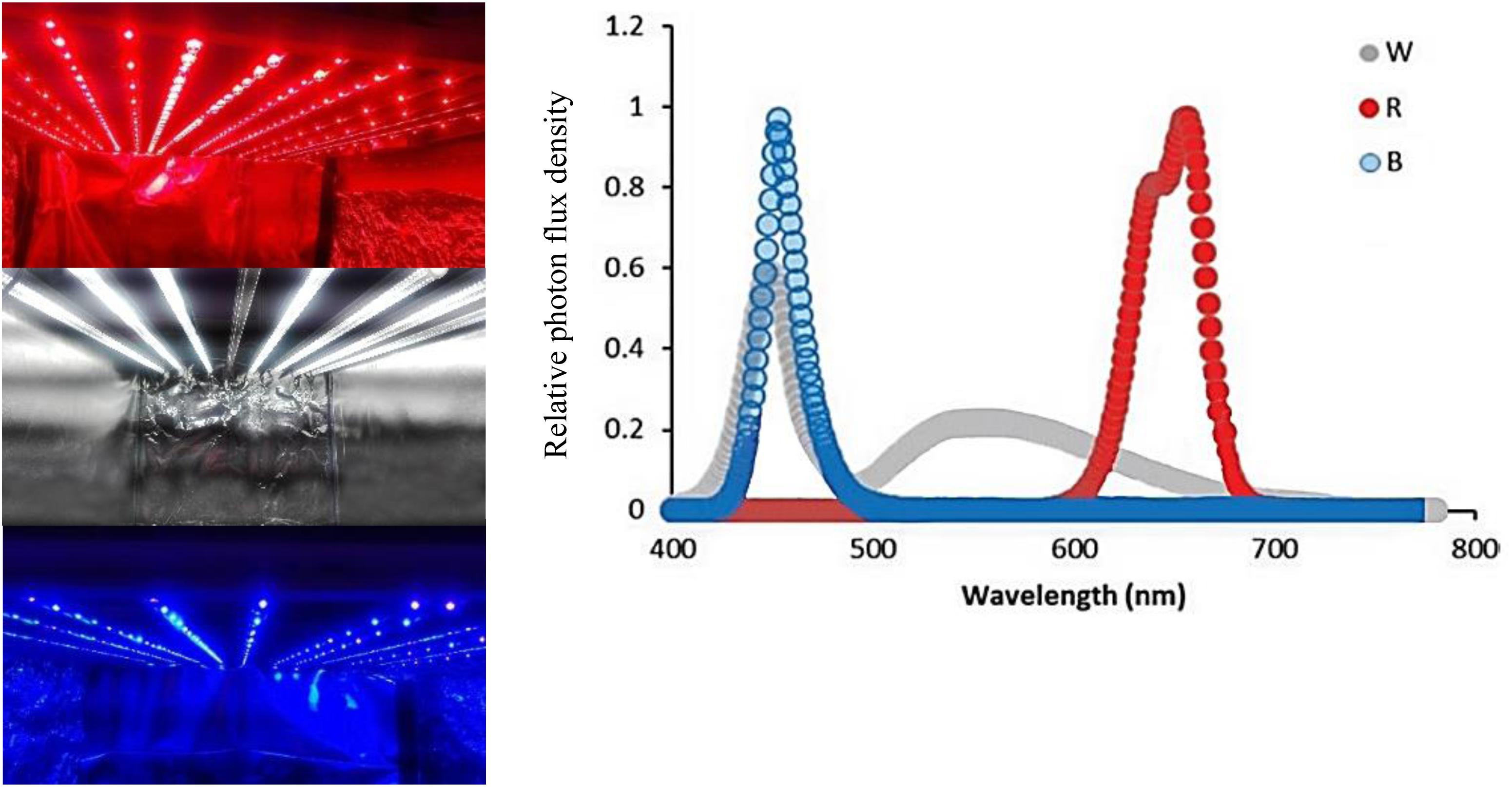
Figure 1. Light spectra of the white (W), red (R), and blue (B) lighting environments measured at the flower level in the cabinets.
Vase Life
The degree of flower wilting and vase life of flowers was determined according to the morphological stages (Paulin et al., 1986) and appearance of symptoms such as shrinkage, brown edges, wilting stems, and yellow/brown foliage (VBN, 2005). The petals were judged to have senesced when they wilted or became necrotic at the edges (Mor et al., 1980). Vase life of individual flowers was defined by the duration from the starting time to the occurrence of flower wilting (Yamamoto et al., 1992).
Relative Fresh Weight (RFW) and Water Uptake
Flower stems were daily weighed and the following formula was used to measure relative fresh weight:
Flower weight was determined at time 0 and at 2, 4, 6, 8, 10, 12, and 15 days following onset of the trial (Zeng et al., 2011). Flowers were placed in 300 mL of tap water. At the different time points, the amount of water (mL) taken up was measured and thereafter the level was brought back up again to 300 mL. At the end of the trial, cumulative water uptake was calculated.
Malondialdehyde (MDA) and Hydrogen Peroxide (H2O2) Contents
Malondialdehyde (MDA) and H2O2 were measured as indices of lipid peroxidation and oxidative stress. The petals of flowers were collected and ground in liquid nitrogen and then homogenized in 4% ice-cold trichloroacetic acid (TCA). The homogeneous samples were then centrifuged at 13,000 g for 15 min at 5°C. The supernatants were used for the measurement of MDA (Heath and Parker, 1968) and H2O2 (Guo et al., 2006) contents. Absorbance was spectrophotometrically (Lambda 25 UV/VIS) measured at 532 and 500 nm for H2O2 and MDA, respectively. The MDA and H2O2 were expressed as μmol g–1 on a fresh weight basis.
Petal Membrane Stability Index (MSI)
For determining petal membrane stability, two samples of plant material (15 disks of petals) were prepared and dipped in 10 mL double distilled water. One of them was kept at 40°C for 30 min and its electrical conductivity (EC) was measured (EC1). The second sample was kept in a boiling water bath (100°C) for 10 min, cooled to room temperature, and EC also recorded (EC2). MSI was determined according to Sairam et al. (1997) based on the following equation:
Measurement of Carbohydrate Levels
Samples from petals were collected during the vase life of flowers (0, 8, 10, and 15 days following start of the trial) and immediately frozen in liquid nitrogen then stored at −80°C. Sugars (sucrose and glucose) and starch were determined by enzymatic assays according to Trethewey et al. (1998) and Hajirezaei et al. (2000). Concentrations of sucrose and glucose were determined in microplates via NADH-specific extinction at 340 nm. The results were expressed as μmol g–1 on a fresh weight basis.
Pigment Analysis
Carotenoids were determined by extraction in 80% acetone as described by Lichtenthaler and Buschmann (2001). Their absorbance was recorded at 470 nm with a spectrophotometer (Lambda 25 UV/VIS) according to Lichtenthaler and Buschmann (2001). The entire process was conducted in low light conditions and placing the samples on ice, and the results expressed as mg g–1 on a fresh weight basis.
Determination of Antioxidant Enzyme Activity
Frozen petal samples (0.2 g for each replicate) were ground in 5 mL of 50 mM sodium phosphate-buffer (pH 7.8) at 4°C. Samples were centrifuged at 13,000 g for 20 min and the supernatant was used to measure the activity of antioxidant enzymes. The 3-mL reaction solution for SOD contained 63 μM ρ-nitro blue tetrazolium chloride, 1.3 μM riboflavin, 13 μM methionine, 50 mM phosphate buffer (pH 7.8), and enzyme extract. Absorbance was measured at 560 nm with a spectrophotometer (Lambda 25 UV/VIS). The 3-mL reaction solution for CAT contained 50 mM phosphate buffer (pH 7.0), 15 mM H2O2, and 50 μl of enzyme extract. The reaction was initiated by adding enzyme extract. The decrease of absorbance of H2O2 over 1 min at 240 nm was recorded. The 3-mL reaction solution for APX contained 0.5 mM AsA, 0.1 mM H2O2, 50 mM phosphate buffer (pH 7.0) and 0.1 mL enzyme extract. APX activity was evaluated by following the decrease in absorbance of AsA over 1 min at 290 nm. The 3-mL reaction solution for POX contained 0.2% (w/v) o-dianisidine, 0.1M potassium phosphate buffer (pH 7.8) and the enzyme extract. Absorbance was measured at 470 nm (Han et al., 2008; Chen et al., 2009). The results were expressed as μmol min–1 mg–1 of total protein for CAT, APX and POD and U mg–1 for SOD.
Stomatal Opening and Polyphasic Chlorophyll a Florescence (OJIP)
On the 8th day of vase life at 2 h after exposure to different light treatments, stomatal opening was determined using a nail polish replica method on the lower epidermis of the second lateral leaflets from the apex (abaxial side) as described by Aliniaeifard and Van Meeteren (2016). The nail polish layer was separated with a strip of transparent sticky tape and pasted on a glass slide and observed using a light microscope (BH-323, Olympus, Tokyo, Japan). Pore aperture of more than 6 μm was considered as open stomata, less than 3 μm as closed, and between 3 and 6 μm as semi-closed. The polyphasic chlorophyll a fluorescence (OJIP) transients were determined using a Fluorpen FP 100-MAX (Photon Systems Instruments, Drásov, Czechia) on young fully expanded carnation leaves after 20 min dark adaptation (Strasser, and Strasser, 1995) according to the JIP test (Strasser et al., 2000) at 1, 4, 8, and 12 days following onset of the trial. The measurement of transient fluorescence was induced by a saturating light of 3000 μmol m–2 s–1 PPFD. Three leaves of each cut carnation stem were used for each replicate. The parameters obtained from this protocol were calculated according to Kalhor et al. (2018).
Statistical Analysis
The data were statistically evaluated by the ‘completely randomized design’ method using SAS software (Statistical Analysis System, Version 9). Means were separated using the Duncan Multiple Range test at a significance level of 0.01. Three (for all measurements traits except vase life) or nine (vase life) replicates were considered for each treatment. A pooled sample of outer and inner petals from each cut carnation flowers was used for measurements of biochemical traits for each replicate. In Figures 3–8, the measurements were conducted up until the end of vase life.
Results
Blue Light Improves the Vase Life of Carnation Cut Flowers
The vase life of flowers was significantly (P > 0.01) prolonged by exposure to B spectral light. The vase life of BL-exposed flowers was 5 days longer than the vase life of WL-exposed flowers (Figures 2, 3). Exposure to RL shortened the vase life of flowers in comparison with WL exposure (Figures 2, 3). Exposure to BL approximately doubled the vase life compared to RL exposure (Figures 2, 3). These results are indicative of positive effects of B wavelength on the post-harvest performance of carnation flowers.
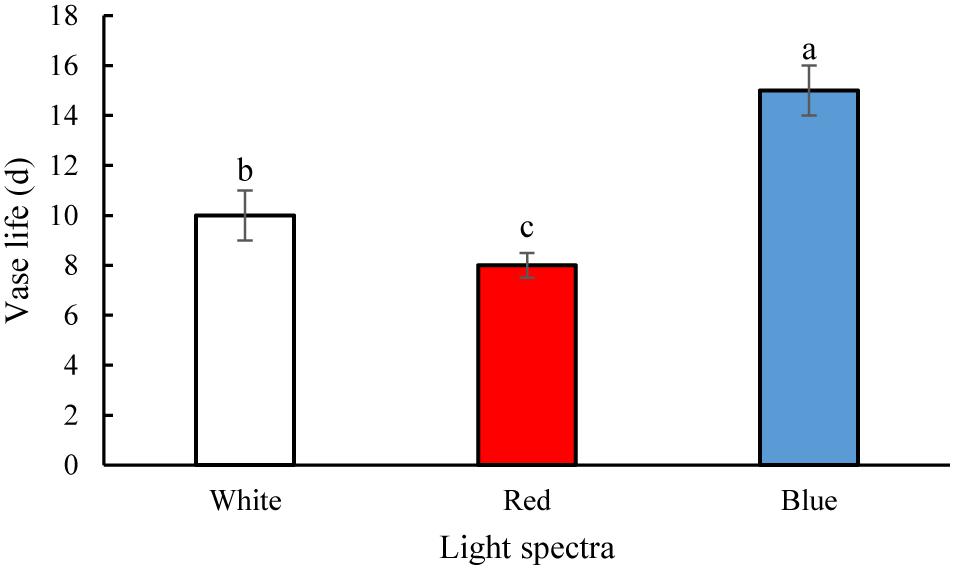
Figure 2. Effects of light spectra on the vase life of carnation cut flowers. Averages ± SEM (n = 9) are shown. Cut flowers were placed in cabinets (120 cm × 90 cm × 80 cm) with different light spectra (white, red, or blue light). Light intensity at the flower level was set at 150 μmol m–2 s–1. Air temperature of the test room was set at 21 ± 2°C and flowers received a photoperiod of 12/12 h light/dark cycles. Different letters indicate that values are significantly different at P < 0.01 according to Duncan’s multiple range tests.
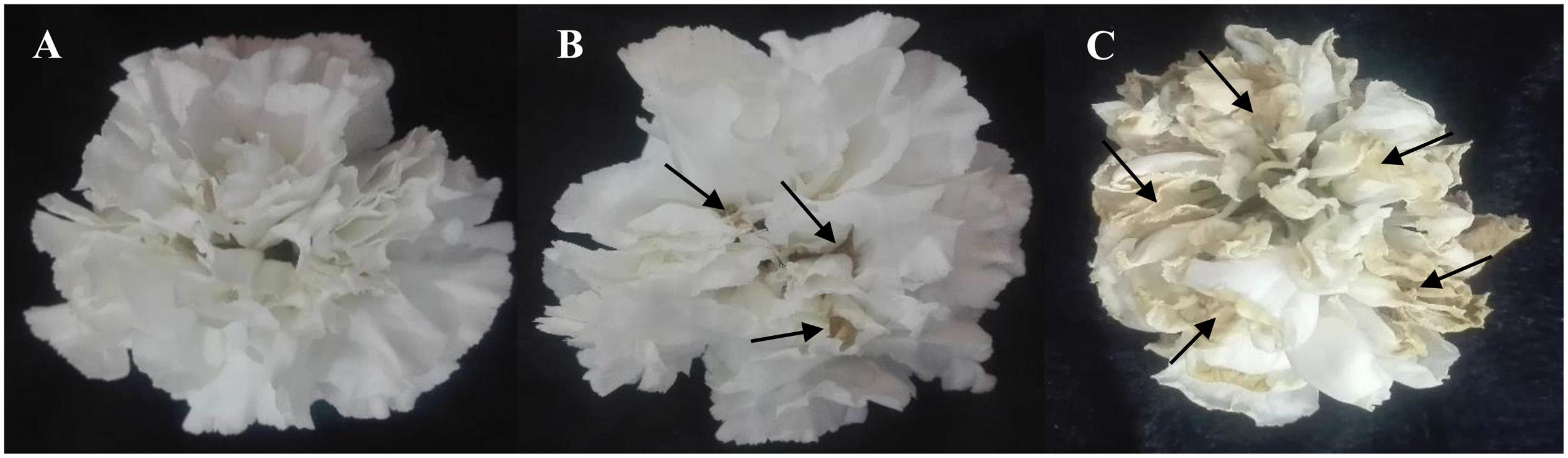
Figure 3. Effects of light spectra (A; blue light, B; white light, and C; red light) on vase life of carnation cut flowers on the 10th day of vase life. Cut flowers were placed in cabinets (120 cm × 90 cm × 80 cm) with different light spectra (white, red, or blue light). Light intensity at the flower level was set at 150 μmol m–2 s–1. Air temperature of the test room was set at 21 ± 2°C and flowers received a photoperiod of 12/12 h light/dark cycles. The arrows indicate areas that are turning yellow and brown.
Relative Fresh Weight (RFW) and Water Uptake of Cut Flowers
The RFW of flowers increased till day 4 of vase life in RL-exposed flowers and till day 6 of BL- and WL-exposed flowers, and decreased thereafter (Figure 4). There was no distinct difference in RFW among the light treatments during the first 4 days. On the 6th day of vase life, the RFW of RL-exposed flowers was three and four times lower than that of WL- and BL-exposed flowers. Following day 6 till the end of vase life assessment, RFW of BL-exposed flowers was significantly higher than the RFW of flowers exposed to other light spectra (Figure 4).
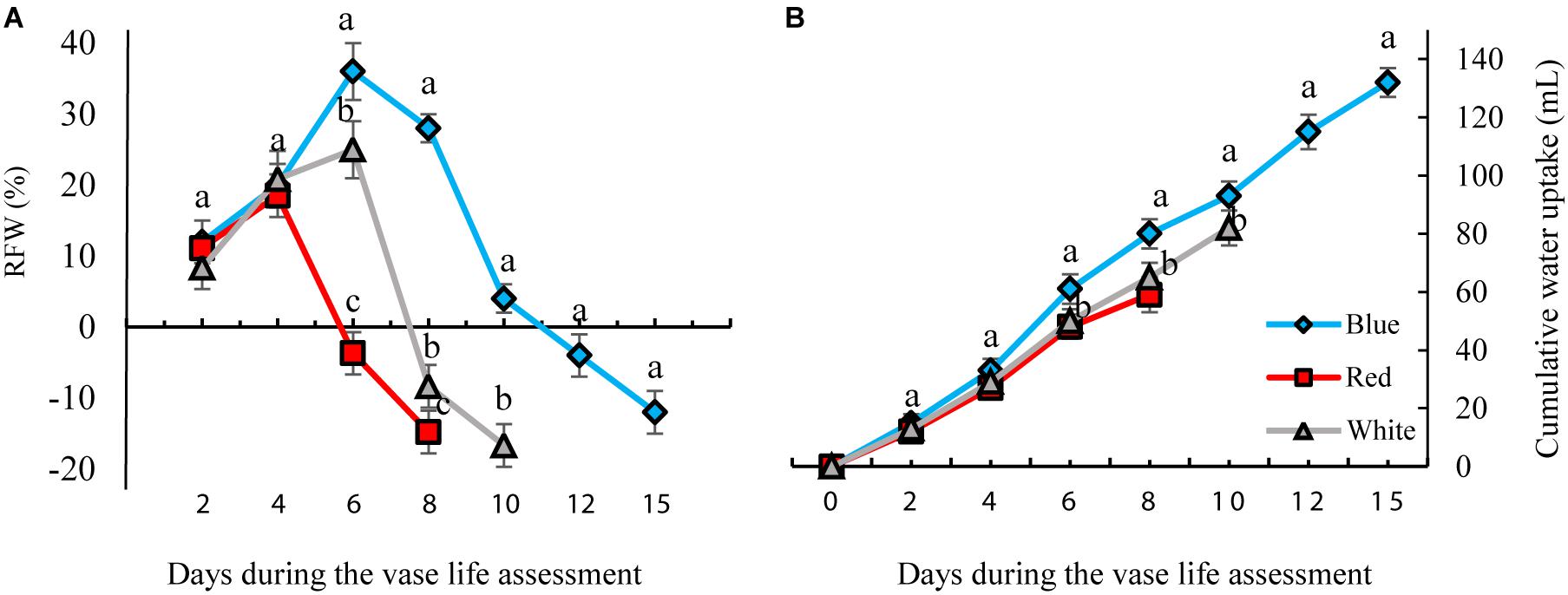
Figure 4. Effects of different light spectra (blue, red, and white) on the relative fresh weight [RFW, (A)] and cumulative water uptake (B) of carnation cut flowers during storage. Cut flowers were placed in cabinets (120 cm × 90 cm × 80 cm) with different light spectra (white, red, or blue light). Light intensity at the flower level was set at 150 μmol m–2 s–1. Air temperature of the test room was set at 21 ± 2°C and flowers received a photoperiod of 12/12 h light/dark cycles. Averages ± SEM (n = 3) are presented. Different letters indicate that values are significantly different at P < 0.01 according to Duncan’s multiple range tests.
The highest and lowest water uptake was found in BL- and RL-exposed flowers, respectively. At the end of these measurements, the cumulative water uptake of BL-exposed flowers was 30 and 18% more than that of RL- and WL-exposed flowers. These results indicate that exposure to BL significantly improves water uptake and postpones weight loss of flowers during their vase life.
Blue Light Delays and Reduces Severity of Oxidative Damage to Carnation Petals
H2O2 and MDA contents were measured to assess the oxidative damage to the petals. H2O2 gradually accumulated in the carnation petals during vase life. BL significantly reduced the concentration of H2O2 by 35, 25, and 42% on days 4, 8, and 10, respectively, compared to the concentration of H2O2 in WL-exposed flowers (Figure 5A). In petals of RL-exposed flowers, concentrations of H2O2 showed an earlier increase than with the other light spectra. On the 8th day of vase life, H2O2 content in RL-exposed flowers was four times higher than in BL-exposed flowers. Similar to H2O2, MDA content also gradually accumulated in the carnation petals during vase life. Exposure to BL caused reductions in MDA contents during the whole vase life assessment period compared to the MDA content of RL- and WL-exposed flowers. MDA content in RL-exposed flowers was approximately doubled in comparison with BL-exposed flowers during vase life (Figure 5B). These results indicate that post-harvest application of BL can decrease the severity of oxidative damage to carnation petals.
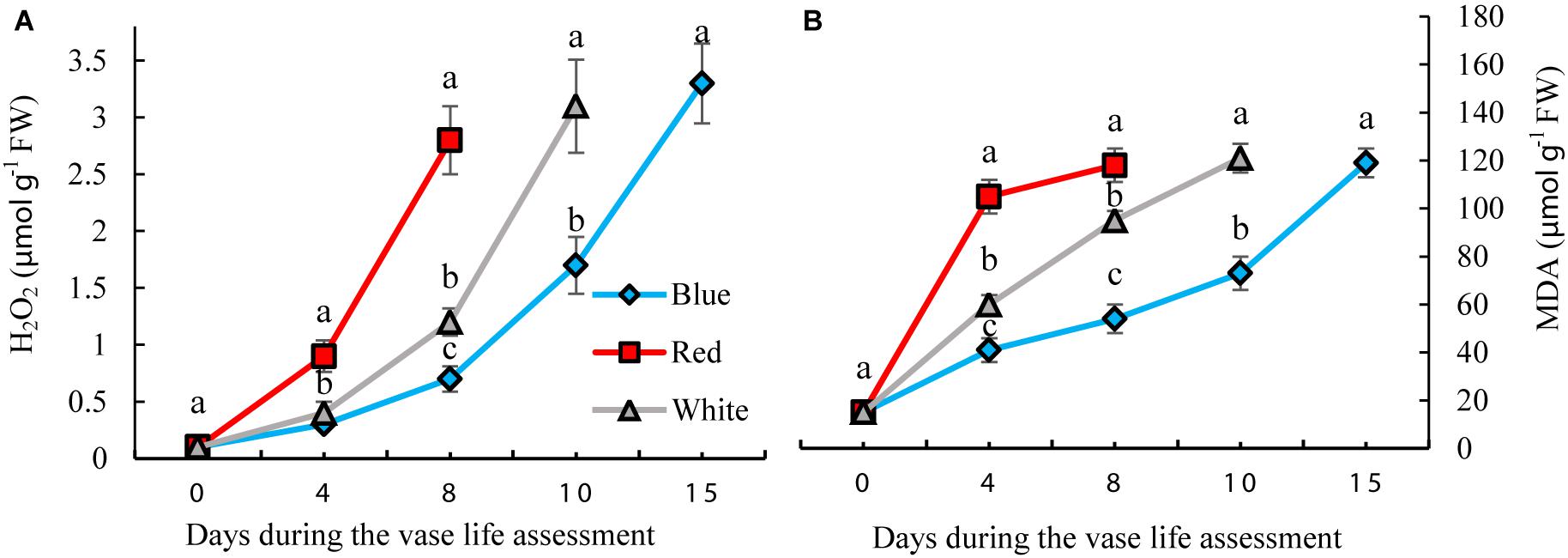
Figure 5. Effects of different light spectra on H2O2 (A) and Malondialdehyde (MDA) (B) contents in the petals of carnation cut flowers during vase life. Cut flowers were placed in cabinets (120 cm × 90 cm × 80 cm) with different light spectra (white, red, or blue light). Light intensity at the flower level was set at 150 μmol m–2 s–1. Air temperature of the test room was set at 21 ± 2°CC and flowers received a photoperiod of 12/12 h light/dark cycles. Means ± SEM are presented (n = 3). Different letters indicate that values are significantly different at P < 0.01 according to Duncan’s multiple range tests.
Blue Light Enhances Antioxidant Enzyme Activities in the Petals of Carnation Cut Flowers
Antioxidant enzyme activities in petals were significantly influenced by light spectra during vase life. Activity of antioxidant enzymes was divided into two phases for all the flowers that were exposed to different light spectra; in the first phase there was an induction in the activity of antioxidant enzymes, and in the second phase their activities declined (Figure 6). For the RL-exposed flowers, activities of antioxidant enzymes reached their maximum levels at an earlier time than the BL- and WL-exposed flowers. The magnitude of increase in antioxidant enzyme activities in BL-exposed flowers was significantly greater than the amplification of enzyme activity in RL- and WL-exposed flowers. In the case of SOD, its activity in BL-exposed flowers was always higher than its activity in RL- and WL-exposed flowers following the start of vase life (Figure 6A). After 4 days, POD activity in BL-exposed flowers was higher than in RL- and WL-exposed flowers (Figure 6B). APX and CAT activities in RL-exposed flowers at the last day of vase life were lower than initial activities, while the activity of these enzymes were more than doubled or tripled in their maximum activities in WL- and BL-exposed flowers, respectively (Figures 6C,D). These results indicate that BL is an environmental signal that results in augmentation of petal antioxidant enzyme activities.
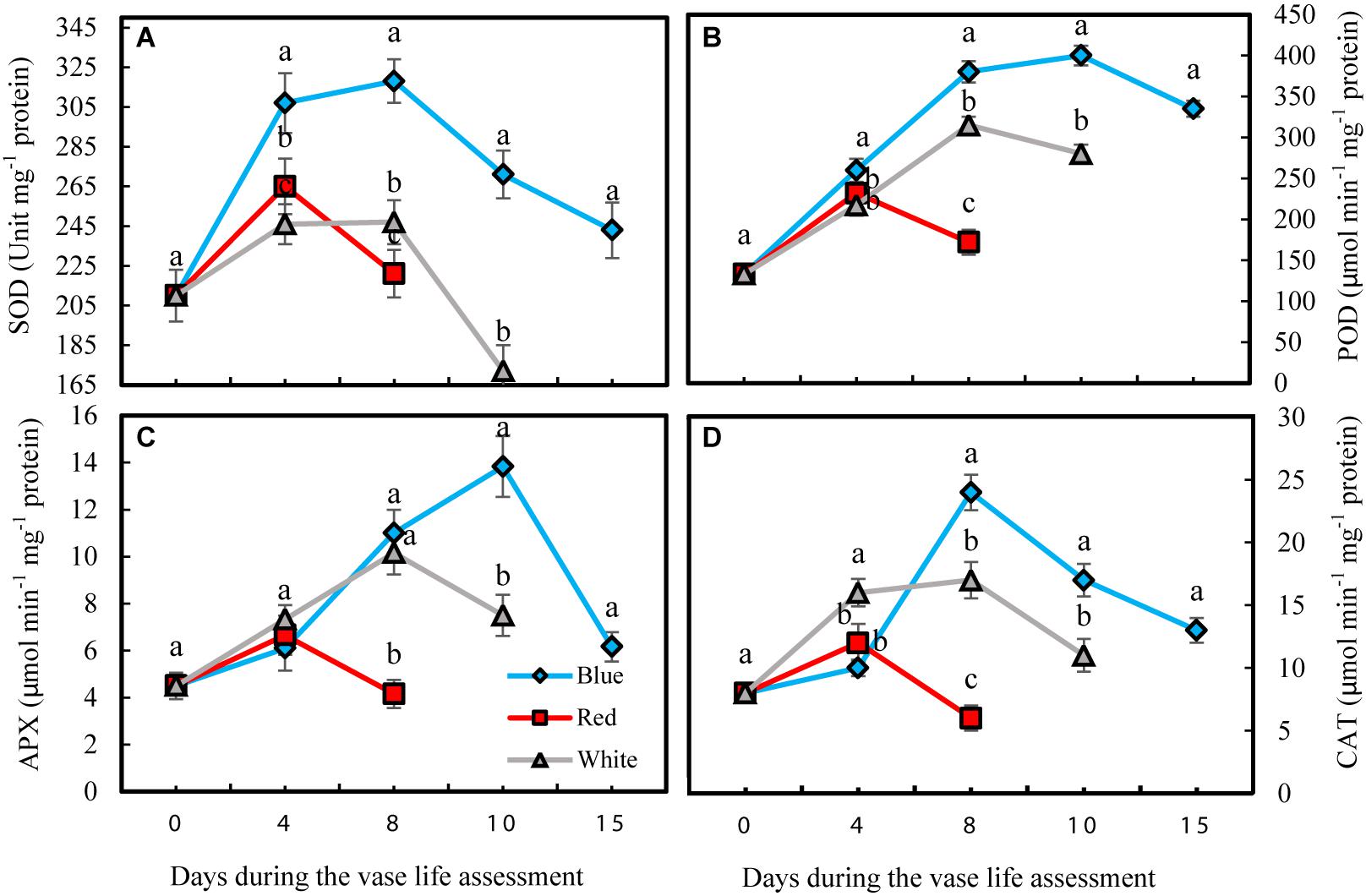
Figure 6. Effects of light spectra on the antioxidant enzymes activity during vase life in cut carnation flower petals. Cut flowers were placed in cabinets (120 cm × 90 cm × 80 cm) with different light spectra (white, red, or blue light). Light intensity at the flower level was set at 150 μmol m–2 s–1. Air temperature of the test room was set at 21 ± 2°C and flowers received a photoperiod of 12/12 h light/dark cycles. (A) Superoxide dismutase (SOD); (B) Peroxidase (POD); (C) Ascorbate peroxidase (APX), and (D) Catalase (CAT). Means ± SEM are presented (n = 3). Different letters indicate that values are significantly different at P < 0.01 according to Duncan’s multiple range tests.
Blue Light Maintains Membrane Stability Index (MSI) During Vase Life of Carnation
On the 8th day of vase life assessment, BL- and WL-exposed flowers had significantly (P < 0.01) higher petal MSI than in the petals of RL-exposed flowers (Figure 7). On the 10th day, the highest MSI (87%) was detected in BL-exposed flowers, significantly higher than the MSI of WL-exposed flowers (58%). On the 15th day when petals of RL- and WL-exposed flowers were wilted, BL-exposed flowers retained 61% MSI in their petals (Figure 7). These results indicate that BL maintains the stability of the petals during storage and delays senescence.
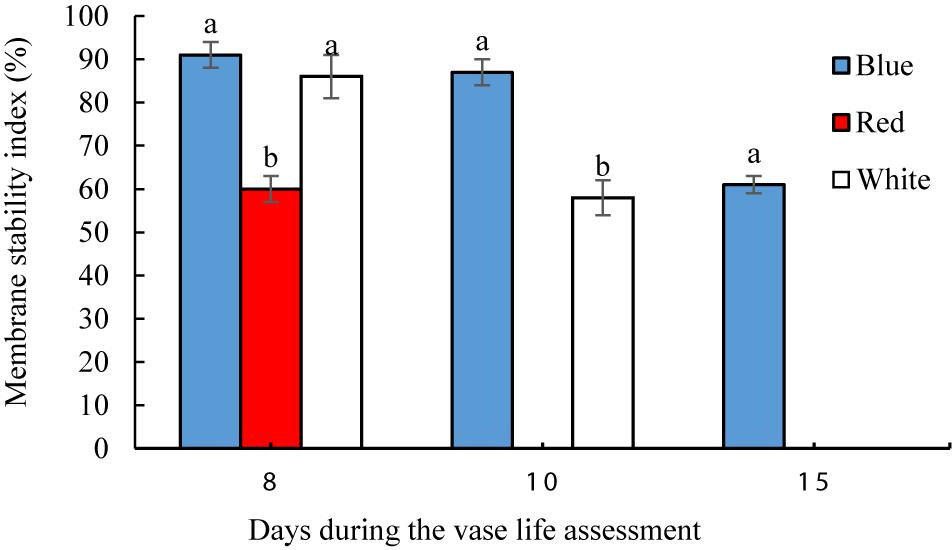
Figure 7. Effects of light spectra on petal membrane stability index (MSI) of carnation cut flowers during vase life. Cut flowers were placed in cabinets (120 cm × 90 cm × 80 cm) with different light spectra (white, red, or blue light). Light intensity at the flower level was set at 150 μmol m–2 s–1. Air temperature of the test room was set at 21 ± 2°C and flowers received a photoperiod of 12/12 h light/dark cycles. Means ± SEM are presented (n = 3). Different letters indicate that values are significantly different at P < 0.01 according to Duncan’s multiple range tests.
Blue Light and Duration of Storage Increase Petal Carbohydrate Content
Carbohydrate status of the petals was largely influenced by exposure of flowers to different light spectra. Concentrations of sucrose and glucose and starch were determined during the vase life in the petals of cut carnation flowers under different light spectra. The starch level in the petals invariably remained below the detection limit of 0.5 μmol g–1 FW (data not shown).
Petals had lower sucrose levels compared to glucose under all different light spectra (Figure 8). Concentrations of both glucose and sucrose increased over the whole vase life period in flowers under all light spectra (Figures 8A,B). Concentrations of both glucose and sucrose were higher in the petals of BL-exposed flowers in comparison with their concentrations in WL and RL-exposed flowers during all stages of vase life (Figures 8A,B). At day 15, sugar levels in petals of BL-exposed flowers were 2 mpared to their levels in RL-exposed flowers on the last day of their vase life (8 and 10 days from onset of experiment).
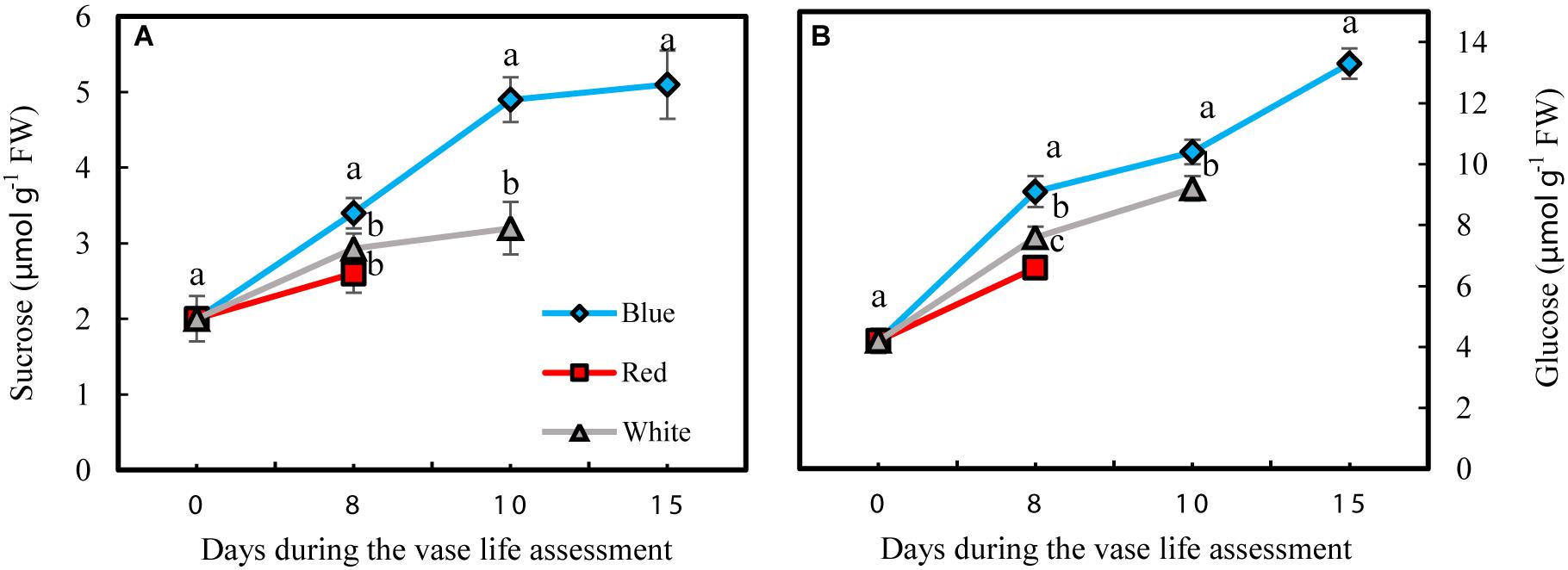
Figure 8. Effects of different light spectra on the content of sucrose (A) and glucose (B) in the petals of carnation cut flowers during vase life. Cut flowers were placed in cabinets (120 cm × 90 cm × 80 cm) with different light spectra (white, red, or blue light). Light intensity at the flower level was set at 150 μmol m–2 s–1. Air temperature of the test room was set at 21 ± 2°C and flowers received a photoperiod of 12/12 h light/dark cycles. Means ± SEM are presented (n = 3). Different letters indicate that values are significantly different at P < 0.01 according to Duncan’s multiple range tests.
Effect of Light Spectra on the Concentration of Carotenoids in Flowers
Significant differences were observed in concentrations of carotenoids during the vase life of flowers exposed to different light spectra. The concentrations gradually decreased during the vase life in all treatments; however the extent of this decline was highest in RL-exposed flowers (Figure 9). Under exposure to BL and WL, the decreases in carotenoid contents were less pronounced compared to their contents under RL (Figure 9). These results showed the negative role of RL in maintaining carotenoids of carnation petals.
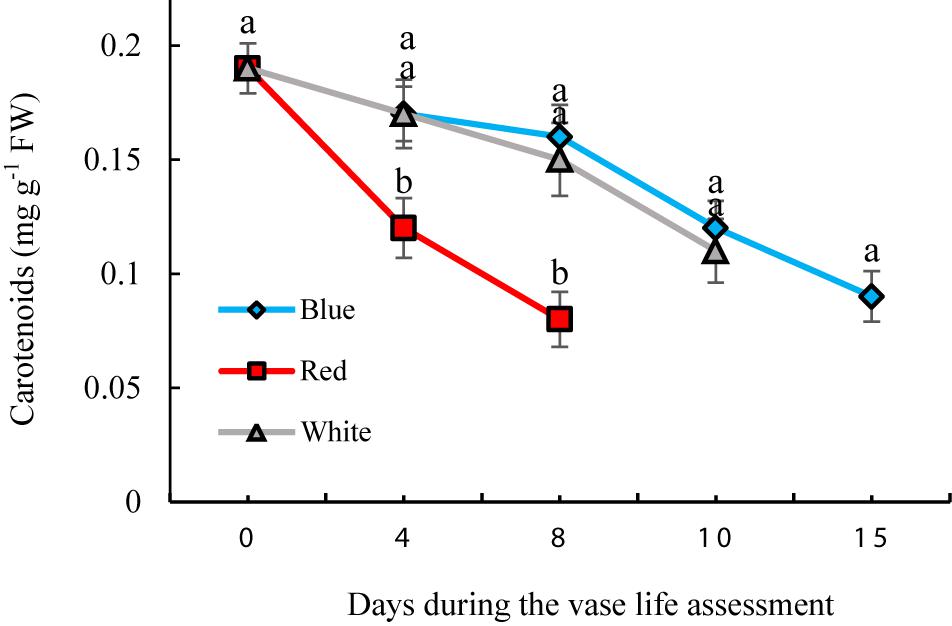
Figure 9. Effects of different light spectra on the content of carotenoids in cut carnation flower. Cut flowers were placed in cabinets (120 cm × 90 cm × 80 cm) with different light spectra (white, red, or blue light). Light intensity at the flower level was set at 150 μmol m–2 s–1. Air temperature of the test room was set at 21 ± 2°C and flowers received a photoperiod of 12/12 h light/dark cycles. Means ± SEM are presented (n = 3). Different letters indicate that values are significantly different at P < 0.01 according to Duncan’s multiple range tests.
Stomatal Status and Photosynthetic Biophysical Analysis in Leaves
Stomatal status in the leaves of carnation cut flowers was affected by light spectra (Table 1). A higher percentage of fully open stomata was observed on the leaves of BL-exposed flowers (95%) than in the RL-exposed flowers (0%) (Table 1). WL-exposed flowers showed a high percentage of semi-closed stomata (80%) (Table 1).
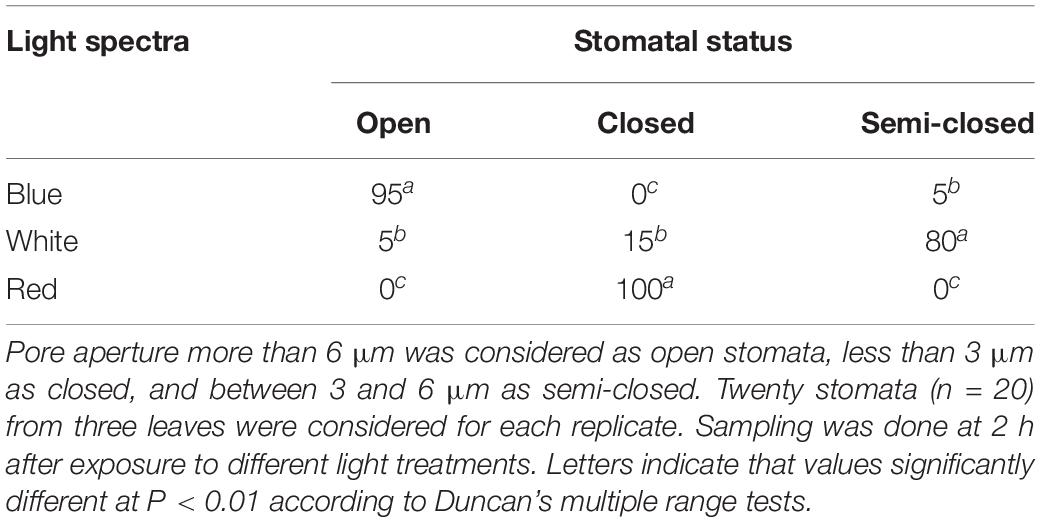
Table 1. Percentage of open, closed, and semi-closed stomata in the leaves of carnation cut flowers following 8 days of exposure to different light spectra.
At the 1st day of the vase life, there was no difference among Fv/Fm in leaves of flowers under different light spectra (Figure 10A). Fv/Fm of RL-exposed flowers significantly decreased during vase life, while no significant difference was observed for Fv/Fm of BL- and WL-exposed flowers. At day 12 of vase life, the highest and lowest Fv/Fm was detected in BL- and RL-exposed flowers, respectively (Figure 10A). The specific energy fluxes per reaction center (RC) for energy absorption (ABS/RC), dissipated energy flux (DI0/RC) and trapped energy flux (TR0/RC) increased but performance index on the absorption basis (PI/ABS) and electron transport flux (ET0/RC) decreased during vase life for flowers under all light spectra (Figure 10). At day 12, highest ABS/RC, DI0/RC and TR0/RC and lowest PI/ABS and ET0/RC were detected in RL-exposed flowers, while no significant difference was detected for these parameters between BL- and WL-exposed flowers (Figure 10). These results are indicative of the negative impact of RL on the photosynthetic performance of carnation leaves.
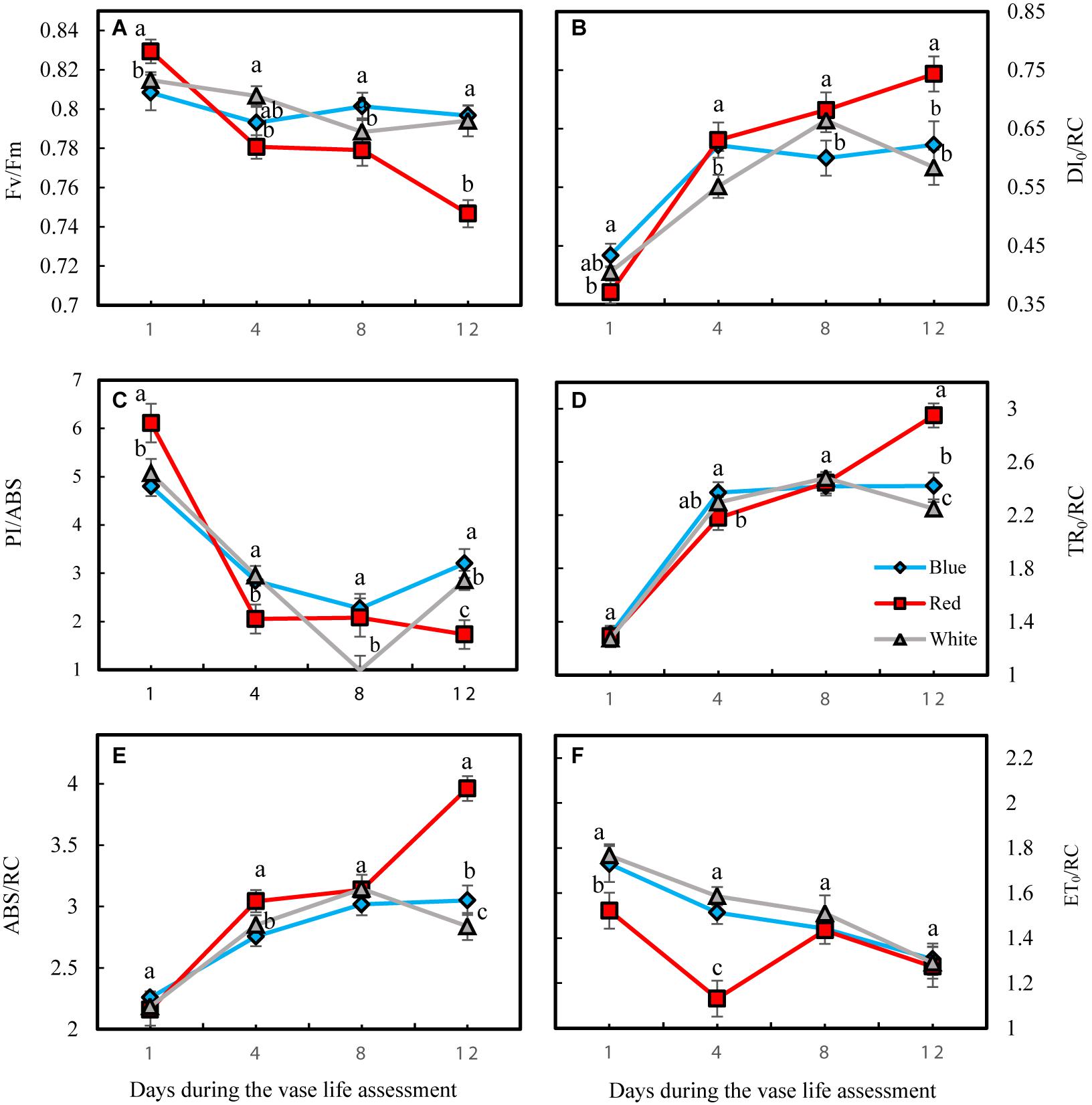
Figure 10. Parameters related to OJIP test including Fv/Fm (A), DI0/RC (B), PI/ABS (C), TR0/RC (D), ABS/RC (E), and ET0/RC (F) obtained from the transient florescence exhibited by leaves of carnation. Cut flowers were placed in cabinets (120 cm × 90 cm × 80 cm) with different light spectra (white, red, or blue light). Light intensity at the flower level was set at 150 μmol m–2 s–1. Air temperature of the test room was set at 21 ± 2°C and flowers received a photoperiod of 12/12 h light/dark cycles. Means ± SEM are presented (n = 3). Different letters indicate that values are significantly different at P < 0.01 according to Duncan’s multiple range tests.
Discussion
Petal senescence is a major physiological process that influences quality attributes and limits vase life of cut flowers (Kumar et al., 2014). Post-harvest application of light spectra on vegetable and fruit has attracted much attention in recent times (Dhakal and Baek, 2014; Gong et al., 2015; Hasperué et al., 2016a, b), with some studies showing beneficial effects of light on vase life of hyacinth (Krzymińska and Karasiewicz, 2017) and pot chrysanthemums (Jerzy et al., 2011). Information regarding the role of different light spectra on vase life of cut flowers is scarce however. In the present study, we exposed carnation cut flowers during vase life to 150 μmol m–2 s–1 of white, blue or red light derived from LEDs for 12 h per day. BL significantly delayed flower senescence and therefore extended vase life.
Senescence of petals in cut flowers is related to a series of highly regulated biochemical and physiological processes. The maintenance of water relations and good water uptake are among the most important factors for the vase life of a variety of cut flowers (Slootwet, 1995; Van Meeteren and Aliniaeifard, 2016). Adverse water relations can lead to problems in flower opening, premature petal wilting and bending of the pedicel, all resulting in shortened vase life (Yamada et al., 2007; Aliniaeifard and Van Meeteren, 2016). It is well-recognized that light spectra are involved in stomatal movement and that BL stimulates stomatal opening (Noichinda et al., 2007). Therefore BL might increase water transport efficiency by increasing transpiration rate and water uptake. Unlike vegetables and fruit (Xu et al., 2014; Hasperué et al., 2016b; Van Meeteren and Aliniaeifard, 2016), elevated water uptake, higher transpiration rates and water loss are beneficial for cut flowers placed in water (Elibox and Umaharan, 2010; Lu et al., 2010). Findings of the present study confirmed that the openness of stomata in the BL treatment coincided with an improved vase life of flowers, increased water uptake, transpiration rate and fresh weight.
Blue light improved carbohydrate levels in the petals during vase life (Figure 6). Sugars are the major source of energy and their deficiency results in senescence of cut flowers (Liao et al., 2000; Singh et al., 2008). Sucrose and glucose are involved in diverse plant processes including antioxidant metabolism, anthocyanin biosynthesis and storage and cell wall biosynthesis. It has been reported that higher amount of sugars in petals is associated with a delay in the senescence of cut flowers (Solfanelli et al., 2006; Agulló-Antón et al., 2011). The starch content in the carnation petals was very low (below the detection limit of 0.5 μmol g–1 FW); similar results have been obtained for the amount of starch in carnation by Agulló-Antón et al. (2011). Change in sugar levels as a result of exposure to different light spectra has been previously reported (Ohashi-Kaneko et al., 2007; Mastropasqua et al., 2016). Light is the driving force of photosynthesis for production of carbohydrates. Influence of light spectrum on the photosynthetic performance of flowers such as roses during cultivation has been recently reported (Bayat et al., 2018). Consistent with our results, Miao et al. (2019) reported that application of BL induced better photosynthetic performance than RL in cucumber plants during cultivation. Similar results were also reported for Chrysanthemum, Cordyline australis, Ficus benjamina, and Sinningia speciosa during cultivation (Zheng and Van Labeke, 2017a, b). In the current study in carnation, BL (and also WL) maintained a high performance of photosynthesis through limiting energy dissipation and elevating electron transport in the electron transport chain of the photosynthetic apparatus. This consequently resulted in an increase in sugar content from harvest to senescence. Application of 14C-sucrose showed that carnation petals act as a strong sink for absorption of sucrose (Ho and Nichols, 1975; Nichols and Ho, 1975). The significant increase in the sugar content in petals under BL might be correlated with the preserved photosynthetic ability in the leaves (Hogewoning et al., 2010; Muneer et al., 2014; Zheng and Van Labeke, 2017a, b) and translocation of sugars from the leaves toward the petals (Ho and Nichols, 1975), where a significant increase in the two sugars occurred. Nichols and Ho (1975) showed that the sucrose levels decrease during flower senescence in carnation. Decrease in soluble carbohydrate levels is related to lack of a carbohydrate source for cut flowers (Van Geest et al., 2016). It has been reported that carbohydrate levels decrease during vase life of different genotypes of chrysanthemum. However, when the flowers are fed by sucrose the levels of glucose and fructose increase during vase life (Van Geest et al., 2016). In the present experiment, due to presence of light intensities above the light compensation point, photosynthesis in leaves and calyx may have provided sugars for the flowers (as the main sink of carbohydrates). As a result it led to elevation in glucose and fructose levels during the vase life. The decrease in carbohydrate content in carnation reported by Ho and Nichols (1975) was probably due to light levels below the light compensation point during the vase life study. This indicates that the performance of the photosynthesis system plays an important role in extending vase life of carnation flowers. However, whether photosynthesis-derived carbohydrates directly influence the vase life of cut flowers is a matter for further research.
Oxidative stress was decreased and the antioxidant defense system was augmented in petals as a result of exposure to BL (Figures 4–6). The carotenoids are among the most important pigments that take part in antioxidative defense in higher plants (Yu et al., 2017). In the present study, the concentration of carotenoids decreased in flowers under all light spectra, but BL maintained higher carotenoids during vase life (Figure 9). Similar results, where application of BL increased the concentration of carotenoids, have been found in lettuce, pepper and broccoli (Li and Kubota, 2009; Gangadhar et al., 2012; Kopsell and Sams, 2013; Hasperué et al., 2016a). These results are similar to those reported by Hasperué et al. (2016a,b); who reported elevated carotenoid levels as a result of BL application as a post-harvest treatment on brussels sprouts and broccoli. It seems that stability and presence of appropriate levels of carotenoids is important to maintain the integrity of membranes, ROS detoxification and other associated physiological processes. Damage to membranes and H2O2 accumulation are considered as oxidative damage during senescence processes (Dhindsa et al., 1981; Bayat et al., 2018), and previous studies have shown that ROS accumulation is coincident with flower senescence (Rogers, 2013). Antioxidant enzymes such as SOD, CAT, APX and POD are the most important defense enzymes for detoxification of ROS in plant tissues (Ye et al., 2017). Increase in antioxidant enzymes activity usually helps neutralize the potential harmful effects of photo-oxidative damage to tissues (Lee et al., 2016; Hasan et al., 2017). Elevated antioxidant enzyme activity, and as a consequence, decrease in lipid peroxidation and H2O2 content, have been reported in response to BL in vegetables such as lettuce (Johkan et al., 2010), tomato (Kim et al., 2013), Chinese cabbage and kale (Lee et al., 2016). In the present study, SOD and CAT activities were elevated as a result of exposure to BL. Ye et al. (2017) reported higher activity of SOD and CAT in Anoectochilus roxburghii leaves with exposure to BL than with exposure to RL. Similar results were also reported for Rehmannia glutinosa (Manivannan et al., 2015) and tomato plants (Kim et al., 2013). APX and CAT in the AsA-GSH cycle and also POD enzyme are responsible for the degradation of H2O2 generated by SOD in plant cells. We showed that the activity of CAT, APX, and POD had similar patterns of change to that observed for SOD activity in BL-exposed flowers, with higher APX, SOD, POD, and CAT activities during vase life; as a result, they contained lower H2O2 in their petals in comparison with RL and WL treatments. This is in line with the findings of Manivannan et al. (2015) and Simlat et al. (2016) who also showed that BL induces activation of the antioxidant defense system in Rehmannia glutinosa and Stevia rebaudiana. These findings suggest that CAT, APX and POD work in a coordinated manner to scavenge H2O2 and that BL might induce their activities. MDA is the product of membrane lipid peroxidation, which is indicative of compromised integrity and structure of the membranes. In line with the other ameliorative effects of BL on oxidative damage, it delayed MDA accumulation in the petals and improved MSI compared to MDA and MSI under other light spectra. This resulted in a decrease in lipid degradation and peroxidation of cell membranes. These results are in accordance with those reported by Xu et al. (2014), who found a postponing effect of BL on MDA content of strawberry fruit during storage.
Of the parameters that were influenced by the light treatments the greatest difference was observed between BL and RL, WL being intermediate or similar to BL. This may be related to the relatively high proportion of B spectrum (41% in the range of 400–500 nm and 18% in the range of 600–700 nm) in the white LEDs. The observed beneficial effects of BL and to a lesser extent WL on, for example, vase life and the antioxidant enzymes, presumably are related to the percentage of BL. However, one could also argue that the lower proportion of RL caused the observed effects.
Carnation vase life is under the influence of ethylene. The longer vase life observed in our experiments under BL may have been due to lower ethylene production, although this was not measured. Light levels and quality can influence ethylene production, but the exact mechanism is still poorly understood. In addition, depending on the tissue under study and the other environmental conditions, light and different wavelengths may either stimulate or reduce ethylene production. There is currently no information available on the effect of light on ethylene production and its signaling pathway in cut flowers, and this would be an interesting topic for further experiments.
Conclusion
The most important finding of the present study is that BL has a positive effect on the post-harvest life of carnation at physiological and biochemical levels. Our report on physiological and biochemical changes during vase life of flowers under different light spectra provides valuable information regarding the mechanism underlying the beneficial effects of BL on the determination of vase life. Therefore, management of lighting during post-harvest storage is important for improving vase life of cut carnation flowers. Based on the obtained results, BL boosted the antioxidant defense system in carnation cut flowers. In our experiments, cut flowers were exposed during their vase life to light levels that are well above the levels applied in the consumer’s home. Therefore, application of the results would not likely be with the consumer. This intensity of light, however, can be applied during storage and in retail facilities. As well as lower temperatures, a novel display design including LED light of suitable wavelengths and intensity may significantly prolong the storage or display life of the flowers and may serve as an non-chemical flower preservative.
Data Availability Statement
The datasets generated for this study are available on request to the corresponding author.
Author Contributions
MAa, SA, MAr, and MM planned and designed the research, performed the experiments, analyzed the data, and wrote the final version of the manuscript. MS, SD, and EW critically revised the manuscript and edited it to present form. TL helped in providing the materials and critically revised the manuscript. All authors read and approved the final manuscript.
Funding
We would like to thank The National Natural Science Foundation of China (No. 31501808), Iran National Science Foundation (INSF) (grant number 96006991) and University of Tehran for their supports.
Conflict of Interest
The authors declare that the research was conducted in the absence of any commercial or financial relationships that could be construed as a potential conflict of interest.
Acknowledgments
The authors would like to thank Dr. Ian Ferguson for linguistic editing of the manuscript.
References
Agulló-Antón, M. Á., Sánchez-Bravo, J., Acosta, M., and Druege, U. (2011). Auxins or sugars: what makes the difference in the adventitious rooting of stored carnation cuttings? J. Plant Growth Regul. 30, 100–113. doi: 10.1007/s00344-010-9174-8
Aliniaeifard, S., and Van Meeteren, U. (2016). Stomatal characteristics and desiccation response of leaves of cut chrysanthemum (Chrysanthemum morifolium) flowers grown at high air humidity. Sci. Hortic. 205, 84–89. doi: 10.1016/j.scienta.2016.04.025
Arora, A., and Singh, V. P. (2006). Polyols regulate the flower senescence by delaying programmed cell death in Gladiolus. J. Plant Biochem. Biotechnol. 15, 139–142. doi: 10.1007/bf03321918
Asil, M. H., Karimi, M., and Zakizadeh, H. (2013). 1-MCP improves the postharvest quality of cut spray carnation (Dianthus caryophyllus L.)‘Optima’flowers. Hortic. Environ. Biotechnol. 54, 58–62. doi: 10.1007/s13580-013-0044-8
Battelli, R., Lomardi, L., Rogers, H. J., Picciarelli, P., Lorenzi, R., and Ceccarelli, N. (2011). Changes in the ultrastructure, protease and caspase-like activities during flower senescence in Lilium longiflorum. Plant Sci. 180, 716–725. doi: 10.1016/j.plantsci.2011.01.024
Bayat, L., Arab, M., Aliniaeifard, S., Seif, M., Lastochkina, O., and Li, T. (2018). Effects of growth under different light spectra on the subsequent high light tolerance in rose plants. AoB Plants. 10:ly052. doi: 10.1093/aobpla/ply052
Buchanan-Wollaston, V., and Morris, K. (2000). “Senescence and cell death in Brassica napus and Arabidopsis,” in Programmed Cell Death in Animals and Plants, eds J. A. Bryant, S. G. Hughes, and J. M. Garland (Oxford: Bios Scientific Publishers Ltd), 163–174.
Chen, C., Lu, S., Chen, Y., Wang, Z., Niu, Y., and Guo, Z. (2009). A gamma-ray induced dwarf mutant from seeded Bermuda grass and its physiological responses to drought stress. HortScience 134, 22–30. doi: 10.21273/jashs.134.1.22
Costa, L., Montano, Y. M., Carrión, C., Rolny, N., and Guiamet, J. J. (2013). Application of low intensity light pulses to delay postharvest senescence of Ocimum basilicum leaves. Postharvest Biol. Technol. 86, 181–191. doi: 10.1016/j.postharvbio.2013.06.017
Deng, M., Qian, H., Chen, L., Sun, B., Chang, J., Miao, H., et al. (2017). Influence of pre-harvest red light irradiation on main phytochemicals and antioxidant activity of Chinese kale sprouts. Food Chem. 222, 1–5. doi: 10.1016/j.foodchem.2016.11.157
Dhakal, R., and Baek, K. H. (2014). Short period irradiation of single blue wavelength light extends the storage period of mature green tomatoes. Postharvest Biol. Technol. 90, 73–77. doi: 10.1016/j.postharvbio.2013.12.007
Dhindsa, R., Plumb-Dhindsa, P., and Thorpe, T. (1981). Leaf senescence: correlated with increased levels of membrane permeability and lipid peroxidation, and decreased levels of superoxide dismutase and catalase. J. Exp. Bot. 32, 93–101. doi: 10.1093/jxb/32.1.93
Elibox, W., and Umaharan, P. (2010). Cultivar differences in the deterioration of vase-life in cut-flowers of Anthurium andraeanum is determined by mechanisms that regulate water uptake. Sci. Hortic. 124, 102–108. doi: 10.1016/j.scienta.2009.12.005
Eskins, K., Jiang, C. Z., and Shibles, R. (1991). Light-quality and irradiance effects on pigments, light-harvesting proteins and Rubisco activity in a chlorophyll- and light- harvesting-deficient soybean mutant. Physiol. Plant. 83, 47–53. doi: 10.1111/j.1399-3054.1991.tb01280.x
Gangadhar, B. H., Mishra, R. K., Pandian, G., and Park, S. W. (2012). Comparative study of color, pungency, and biochemical composition in chili pepper (Capsicum annuum) under different light-emitting diode treatments. HortScience 47, 1729–1735. doi: 10.21273/hortsci.47.12.1729
Gong, D., Cao, S., Sheng, T., Shao, J., Song, C., Wo, F., et al. (2015). Effect of blue light on ethylene biosynthesis, signalling and fruit ripening in postharvest peaches. Sci. Hortic. 197, 657–664. doi: 10.1016/j.scienta.2015.10.034
Guo, Z., Ou, W., Lu, S., and Zhong, Q. (2006). Differential responses of antioxidative system to chilling and drought in four rice cultivars differing in sensitivity. Plant Physiol. Biochem. 44, 828–836. doi: 10.1016/j.plaphy.2006.10.024
Hajirezaei, M. R., Takahata, Y., Trethewey, R. N., Willmitzer, L., and Sonnewald, U. (2000). Impact of elevated cytosolic and apoplastic invertase activity on carbon metabolism during potato tuber development. J. Exp. Bot. 51, 439–445. doi: 10.1093/jexbot/51.suppl_1.439
Han, L. B., Song, G. L., and Zhang, X. (2008). Preliminary observation of physiological responses of three turfgrass species to traffic stress. HortTechnology. 18, 139–143. doi: 10.21273/horttech.18.1.139
Hasan, M., Bashir, T., Ghosh, R., Lee, S. K., and Bae, H. (2017). An overview of LEDs’ effects on the production of bioactive compounds and crop quality. Molecules. 22:1420. doi: 10.3390/molecules22091420
Hasperué, J. H., Guardianelli, L., Rodoni, L. M., Chaves, A. R., and Martínez, G. A. (2016a). Continuous white–blue LED light exposition delays postharvest senescence of broccoli. Food Sci. Technol. 65, 495–502. doi: 10.1016/j.lwt.2015.08.041
Hasperué, J. H., Rodoni, L. M., Guardianelli, L. M., Chaves, A. R., and Martínez, G. A. (2016b). Use of LED light for Brussels sprouts postharvest conservation. Sci. Hortic. 213, 281–286. doi: 10.1016/j.scienta.2016.11.004
Heath, R. L., and Parker, L. (1968). Photoperoxidation in isolated chloroplasts kinetics and stoichiometry of fatty acid peroxidation. Arch. Biophys. 25, 189–198. doi: 10.1016/0003-9861(68)90654-1
Ho, L. C., and Nichols, R. (1975). The role of phloem transport in the translocation of sucrose along the stem of carnation cut flowers. Ann. Bot. 39, 439–446. doi: 10.1093/oxfordjournals.aob.a084958
Hogewoning, S. W., Trouwborst, G., Maljaars, H., Poorter, H., van Ieperen, W., and Harbinson, J. (2010). Blue light dose–responses of leaf photosynthesis, morphology, and chemical composition of Cucumis sativus grown under different combinations of red and blue light. J. Exp. Bot. 61, 3107–3117. doi: 10.1093/jxb/erq132
Hotta, M., Hattori, H., Hirano, T., Kume, T., Okumura, Y., et al., (2016). Breeding and characteristics of spray-type carnation’Kaneainou 1 go’with a long vase life. Res. Bull. Aichi Agric. Res. Ctr. 48, 63–71.
Jerzy, M., Zakrzewski, P., and Schroeter-Zakrzewska, A. (2011). Effect of colour of light on the opening of inflorescence buds and post-harvest longevity of pot chrysanthemums (Chrysanthemum × grandiflorum (Ramat.) Kitam). Acta Agrobot. 64, 13–18. doi: 10.5586/aa.2011.025
Johkan, M., Shoji, K., Goto, F., Hashida, S., and Yoshihara, T. (2010). Blue light-emitting diode light irradiation of seedlings improves seedling quality and growth after transplanting in red leaf lettuce. HortScience 45, 1809–1814. doi: 10.21273/hortsci.45.12.1809
Kalhor, M., Aliniaeifard, S., Seif, M., Asayesh, E., Bernard, F., Hassani, B., et al., (2018). Enhanced salt tolerance and photosynthetic performance: implication of γ-amino butyric acid application in salt-exposed lettuce (Lactuca sativa L.) plants. Plant Physiol. Biochem. 130, 157–172. doi: 10.1016/j.plaphy.2018.07.003
Karami, O., Deljou, A., and Kordestani, G. K. (2008). Secondary somatic embryogenesis of carnation (Dianthus caryophyllus L.). Plant Cell, Tissue Organ Cult. 92, 273–280. doi: 10.1007/s11240-007-9332-2
Karimi, M., Asil, M. H., and Zakizadeh, H. (2012). Increasing plant longevity and associated metabolic events in potted carnation (Dianthus caryophyllus L. Clove Pink). Braz. J. Plant Physiol. 24, 247–252. doi: 10.1590/s1677-04202012000400003
Kim, K., Kook, H. S., Jang, Y. J., Lee, W. H., Kamala-Kannan, S., Chae, J. C., et al., (2013). The effect of blue-light-emitting diodes on antioxidant properties and resistance to Botrytis cinerea in tomato. J. Plant Pathol. Microbiol. 4:203.
Kopsell, D. A., and Sams, C. E. (2013). Increases in shoot tissue pigments, glucosinolates, and mineral elements in sprouting broccoli after exposure to short-duration blue light from light emitting diodes. J. Amer. Soc. Hort. Sci. 138, 31–37. doi: 10.21273/jashs.138.1.31
Krzymińska, A., and Karasiewicz, M. (2017). Postharvest longevity of cut hyacinths depending on light colour and types of lamps. Nauka Przyr. Technol. 11, 55–64.
Kumar, M., Singh, V. P., Arora, A., and Singh, N. (2014). The role of abscisic acid (ABA) in ethylene insensitive Gladiolus (Gladiolus grandiflora Hort.) flower senescence. Acta Physiol. Plant. 36, 151–159. doi: 10.1007/s11738-013-1395-6
Lee, M. K., Arasu, M. V., Park, S., Byeon, D. H., Chung, S. O., Park, S. U., et al., (2016). LED lights enhance metabolites and antioxidants in chinese cabbage and kale. Braz. Arch. Biol. Technol. 59:e16150546.
Lester, G. E., Makus, D. J., and Hodges, D. M. (2010). Relationship between fresh-packaged spinach leaves exposed to continuous light or dark and bioactive contents: effects of cultivar, leaf size, and storage duration. J. Agric. Food Chem. 58, 2980–2987. doi: 10.1021/jf903596v
Li, Q., and Kubota, C. (2009). Effects of supplemental light quality on growth and phytochemicals of baby leaf lettuce. Environ. Expt. Bot. 67, 59–64. doi: 10.1002/jsfa.6173
Liao, L. J., Lin, Y. H., Huang, K. L., Chen, W. S., and Cheng, Y. M. (2000). Postharvest life of cut rose flowers as affected by silver thiosulfate and sucrose. Bot. Bull. Acad. Sin. 41, 299–303.
Lichtenthaler, H. K., and Buschmann, C. (2001). Chlorophylls and carotenoids: measurement and characterization by UV-VIS spectroscopy. Curr Protoc Food Anal Chem. 1, 1–8.
Lu, P., Cao, J., He, S., Liu, J., Li, H., Cheng, G., et al., (2010). Nano-silver pulse treatments improve water relations of cut rose cv. Movie Star flowers. Postharvest Biol. Technol. 57, 196–202. doi: 10.1016/j.postharvbio.2010.04.003
Manivannan, A., Soundararajan, P., Halimah, N., Ko, C. H., and Jeong, B. R. (2015). Blue LED light enhances growth, phytochemical contents, and antioxidant enzyme activities of Rehmannia glutinosa cultured in vitro. Hortic. Environ. Biotechnol. 56, 105–113. doi: 10.1007/s13580-015-0114-1
Mastropasqua, L., Tanzarella, P., and Paciolla, C. (2016). Effects of postharvest light spectra on quality and health-related parameters in green Asparagus officinalis L. Postharvest Biol. Technol. 112, 143–151. doi: 10.1016/j.postharvbio.2015.10.010
Miao, Y., Chen, Q., Qu, M., Gao, L., and Hou, L. (2019). Blue light alleviates ‘red light syndrome’ by regulating chloroplast ultrastructure, photosynthetic traits and nutrient accumulation in cucumber plants. Sci. Hortic. 257:108680. doi: 10.1016/j.scienta.2019.108680
Mor, Y., Reid, M. S., and Kofranek, A. M. (1980). Role of the ovary in carnation senescence. Sci. Hortic. 13, 377–383. doi: 10.1016/0304-4238(80)90096-5
Muneer, S., Kim, E., Park, J., and Lee, J. (2014). Influence of green, red and blue light emitting diodes on multiprotein complex proteins and photosynthetic activity under different light intensities in lettuce leaves (Lactuca sativa L.). Int. J. Mol. Sci. 15, 4657–4670. doi: 10.3390/ijms15034657
Nascimento, L. B. S., Leal-Costa, M. V., Coutinho, M. A. S., Moreira, N.D. S., Lage, C. L. S., Barbi, N. D. S., et al., (2013). Increased antioxidant activity and changes in phenolic profile of Kalanchoe pinnata (Lamarck) persoon (crassulaceae) specimens grown under supplemental blue light. Photochem. Photobiol. 89, 391–399. doi: 10.1111/php.12006
Nichols, R., and Ho, L. C. (1975). Effects of ethylene and sucrose on translocation of dry matter and 14C-sucrose in the cut flower of the glasshouse carnation (Dianthus caryophyllus) during senescence. Ann. Bot. 39, 287–296. doi: 10.1093/oxfordjournals.aob.a084942
Noichinda, S., Bodhipadma, K., Mahamontri, C., Narongruk, T., and Ketsa, S. (2007). Light during storage prevents loss of ascorbic acid, and increases glucose and fructose levels in Chinese kale (Brassica oleracea var. alboglabra). Postharvest Biol. Technol. 44, 312–315. doi: 10.1016/j.postharvbio.2006.12.006
Ohashi-Kaneko, K., Takase, M., Kon, N., Fujiwara, K., and Kurata, K. (2007). Effect of light quality on growth and vegetable quality in leaf lettuce, spinach and komatsuna. Environ. Control Biol. 45, 189–198. doi: 10.2525/ecb.45.189
Oms-Oliu, G., Aguiló-Aguayo, I., Martín-Belloso, O., and Soliva-Fortuny, R. (2010). Effects of pulsed light treatments on quality and antioxidant properties of fresh mushrooms (Agaricus bisporus). Postharvest Biol. Technol. 56, 216–222. doi: 10.1016/j.postharvbio.2009.12.011
Paulin, A., Droillard, M. J., and Bureau, J. M. (1986). Effect of a free radical scavenger, 3,4,5- trichlorophenol, on ethylene production and on changes in lipids and membrane integrity during senescence of petals of cut carnations (Dianthus caryophyllus). J. Plant Physiol. 67, 465–471. doi: 10.1111/j.1399-3054.1986.tb05764.x
Perrin, P. W. (1982). Post storage effect of light, temperature and nutrient spray treatments on chlorophyll development in cabbage. Can. J. Plant Sci. 62, 1023–1026. doi: 10.4141/cjps82-151
Rodrigues, M. A., Bianchetti, R. E., and Freschi, L. (2014). Shedding light on ethylene metabolism in higher plants. Front. Plant Sci. 5:665. doi: 10.3389/fpls.2014.00665
Rogers, H. J. (2013). From models to ornamentals: how is flower senescence regulated? Plant Mol. Biol. 82, 563–574. doi: 10.1007/s11103-012-9968-0
Sairam, R. K., Deshmukh, P. S., and Shukla, D. S. (1997). Tolerance of drought and temperature stress in relation to increased antioxidant enzyme activity in wheat. J Agron. Crop Sci. 178, 171–177.
Satoh, S. (2011). Ethylene production and petal wilting during senescence of cut carnation (Dianthus caryophyllus) flowers and prolonging their vase life by genetic transformation. J. Jpn. Soc. Hortic. Sci. 80, 127–135. doi: 10.2503/jjshs1.80.127
Shibuya, K., and Ichimura, K. (2010). Depression of autocatalytic ethylene production by high-temperature treatment in carnation flowers. J. Jpn. Soc. Hortic. Sci. 79, 97–102. doi: 10.2503/jjshs1.79.97
Simlat, M., Lêzak, P., Mo’s, M., Warcho, M., Skrzypek, E., and Ptak, A. (2016). The effect of light quality on seed germination, seedling growth and selected biochemical properties of Stevia rebaudiana Bertoni. Sci. Hortic. 211, 295–304. doi: 10.1016/j.scienta.2016.09.009
Singh, A., Kumar, J., and Kumar, P. (2008). Effects of plant growth regulators and sucrose on postharvest physiology, membrane stability and vase life of cut spikes of gladiolus. Plant Growth Regul. 55:221. doi: 10.1007/s10725-008-9278-3
Slootwet, G. (1995). Effect of water temperature on water uptake and vase life of different cut flowers. Acta Hort. 405, 67–74. doi: 10.17660/actahortic.1995.405.7
Solfanelli, C., Poggi, A., Loreti, E., Alpi, A., and Perata, P. (2006). Sucrose-specific induction of the anthocyanin biosynthetic pathway in Arabidopsis. Plant Physiol. 140, 637–646. doi: 10.1104/pp.105.072579
Strasser, R. J., Srivastava, A., and Tsimilli-Michael, M. G. (2000). The florescence transient as a tool to characterize and screen photosynthetic samples, in Probing Photosynthesis: Mechanisms, Regulation and Adaptation, eds M. Yunus, U. Pathre, and P. Mohanty (Boca Raton, FL: CRC press), 445–483.
Strasser B. J., and Strasser, R. J. (1995). “Measuring fast florescence transients to address environmental questions: the JIP test,” in Photosynthesis: From Light to Biosphere, Ed. P. Mathis (Amsterdam: Kluwer Academic Publishers).
Sugawara, H., Shibuya, K., Yoshioka, T., Hashiba, T., and Satoh, S. (2002). Is a cysteine proteinase inhibitor involved in the regulation of petal wilting in senescing carnation (Dianthus caryophyllus L.) flowers? J. Exp. Bot. 53, 407–413. doi: 10.1093/jexbot/53.368.407
Tanase, K., Onozaki, T., Satoh, S., Shibata, M., and Ichimura, K. (2008). Differential expression levels of ethylene biosynthetic pathway genes during senescence of long-lived carnation cultivars. Postharvest Biol. Technol. 47, 210–217. doi: 10.1016/j.postharvbio.2007.06.023
Tanase, K., Otsu, S., Satoh, S., and Onozaki, T. (2015). Expression levels of ethylene biosynthetic genes and senescence-related genes in carnation (Dianthus caryophyllus L.) with ultra-long-life flowers. Sci. Hortic. 183, 31–38. doi: 10.1016/j.scienta.2014.11.025
Trethewey, R. N., Geigenberger, P., Riedel, K., Hajirezaei, M. R., Sonnewald, U., Stitt, M., et al., (1998). Combined expression of glucokinase and invertase in potato tubers leads to a dramatic reduction in starch accumulation and a stimulation of glycolysis. Plant J. 15109–118. doi: 10.1046/j.1365-313x.1998.00190.x
Van Doorn, W. G. (2011). Classes of programmed cell death in pants, compared to those in animals. J. Exp. Bot. 62, 4749–4761. doi: 10.1093/jxb/err196
Van Doorn, W. G., and Woltering, E. J. (2008). Physiology and molecular biology of petal senescence. J. Exp. Bot. 59, 453–480. doi: 10.1093/jxb/erm356
Van Geest, G., Choi, Y. H., Arens, P., Post, A., Liu, Y., and van Meeteren, U. (2016). Genotypic differences in metabolomic changes during storage induced-degreening of chrysanthemum disk florets. Postharvest Biol. Technol. 115, 48–59. doi: 10.1016/j.postharvbio.2015.12.008
Van Meeteren, U., and Aliniaeifard, S. (2016). “Stomata and postharvest physiology,” in Postharvest Ripening. Physiology of Crops, Ed. S. Pareek (Boca Raton, FL: CRC Press), 157–216.
Wagstaff, C., Yang, T. J. W., Stead, A. D., Buchanan-Wollaston, V., and Roberts, J. A. (2009). A molecular and structural characterization of senescing petals and leaves. Plant J. 57, 690–705. doi: 10.1111/j.1365-313X.2008.03722.x
Woltering, E. J., and Seifu, Y. W. (2014). Low intensity monochromatic red, blue or green light increases the carbohydrate levels and substantially extends the shelf life of fresh-cut lettuce. Acta Hortic. 1079, 257–264. doi: 10.17660/actahortic.2015.1079.30
Xu, F., Shi, L., Chen, W., Cao, S., Su, X., and Yang, Z. (2014). Effect of blue light treatment on fruit quality, antioxidant enzymes and radical-scavenging activity in strawberry fruit. Sci. Hortic. 175, 181–186. doi: 10.1016/j.scienta.2014.06.012
Yagi, M., Kosugi, S., Hirakawa, H., Ohmiya, A., Tanase, K., Harada, T., et al., (2013). Sequence analysis of the genome of carnation (Dianthus caryophyllus L.). DNA Res. 21, 231–241.
Yamada, K., Ito, M., Oyama, T., Nakada, M., Maesaka, M., and Yamaki, S. (2007). Analysis of sucrose metabolism during petal growth of cut roses. Postharvest. Biol. Technol. 43, 174–177. doi: 10.1016/j.postharvbio.2006.08.009
Yamamoto, K., Saitoh, C., Yokoo, Y., Furukawa, T., and Oshima, K. (1992). Inhibition of wilting and autocatalytic ethylene production in cut carnation flowers by cispropenylphosphonic acid. Plant Growth Regul. 11, 405–409. doi: 10.1007/bf00130649
Ye, S., Shao, Q., Xu, M., Li, S., Wu, M., Tan, X., et al. (2017). Effects of light quality on morphology, enzyme activities, and bioactive compound contents in Anoectochilus roxburghii. Front. Plant Sci. 8:857. doi: 10.3389/fpls.2017.00857
Yu, W., Liu, Y., Song, L., Jacobs, D. F., Du, X., Ying, Y., et al., (2017). Effect of differential light quality on morphology, photosynthesis, and antioxidant enzyme activity in Camptotheca acuminata seedlings. J. Plant Growth Regul. 36, 148–160. doi: 10.1007/s00344-016-9625-y
Zeng, C. L., Liu, L., and Xu, G. Q. (2011). The physiological responses of carnation cut flowers to exogenous nitric oxide. Sci. Hortic. 127, 424–430. doi: 10.1016/j.scienta.2010.10.024
Zhan, L., Hu, J., Li, Y., and Panga, L. (2012). Combination of light exposure and low temperature in preserving quality and extending shelf-life offresh-cut broccoli (Brassica oleracea L.). Postharvest Biol. Technol. 72, 76–81. doi: 10.1016/j.postharvbio.2012.05.001
Zheng, L., and Van Labeke, M. C. (2017a). Chrysanthemum morphology, photosynthetic efficiency and antioxidant capacity are differentially modified by light quality. J. Plant Physiol. 213, 66–74. doi: 10.1016/j.jplph.2017.03.005
Keywords: antioxidant enzymes, carnation, light spectrum, oxidative stress, radiation, vase life
Citation: Aalifar M, Aliniaeifard S, Arab M, Zare Mehrjerdi M, Dianati Daylami S, Serek M, Woltering E and Li T (2020) Blue Light Improves Vase Life of Carnation Cut Flowers Through Its Effect on the Antioxidant Defense System. Front. Plant Sci. 11:511. doi: 10.3389/fpls.2020.00511
Received: 18 December 2019; Accepted: 06 April 2020;
Published: 26 May 2020.
Edited by:
Patrícia Duarte De Oliveira Paiva, Federal University of Lavras, BrazilReviewed by:
Chao Ma, China Agricultural University, ChinaRoberta Paradiso, University of Naples Federico II, Italy
Copyright © 2020 Aalifar, Aliniaeifard, Arab, Zare Mehrjerdi, Dianati Daylami, Serek, Woltering and Li. This is an open-access article distributed under the terms of the Creative Commons Attribution License (CC BY). The use, distribution or reproduction in other forums is permitted, provided the original author(s) and the copyright owner(s) are credited and that the original publication in this journal is cited, in accordance with accepted academic practice. No use, distribution or reproduction is permitted which does not comply with these terms.
*Correspondence: Sasan Aliniaeifard, aliniaeifard@ut.ac.ir; Tao Li, litao06@caas.cn