Corrigendum: The Sweet Taste of Adapting to the Desert: Fructan Metabolism in Agave Species
- Department of Genetic Engineering, Cinvestav Unidad Irapuato, Guanajuato, Mexico
Over 70% of Agave species, (159 of 206) are found in Mexico and are well adapted to survive under hot, arid conditions, often in marginal terrain, due to a unique combination of morphological and physiological attributes. In the pre-Columbian era agaves were also key to human adaptation to desert terrain. In contrast to other species such as cacti or resurrection plants, Agaves store carbohydrates in the form of fructan polymers rather than starch or sucrose, however, properties specific to fructans such as a strong hydration shell, the ability to be transported through phloem, variable composition throughout the Agave life-cycle and accumulation in succulent tissues and flowers suggest a potential for multiple functional roles. This mini-review summarizes current knowledge of molecular and biochemical aspects of fructan metabolism in Agave species.
Introduction
Fructan polymers, are synthesized by some bacteria and fungi and an estimated 15% of angiosperms including both monocotyledons and dicotyledons from different genera (Hendry, 1993; Figure 1). In plants, fructan polymers are described based on their structure and complexity (Versluys et al., 2018). Although neo type fructans have only been described in monocotyledons, no strong correlation exists between the type of fructan polymers and the genus or species in which they occur, supporting independent evolution of fructan metabolism.
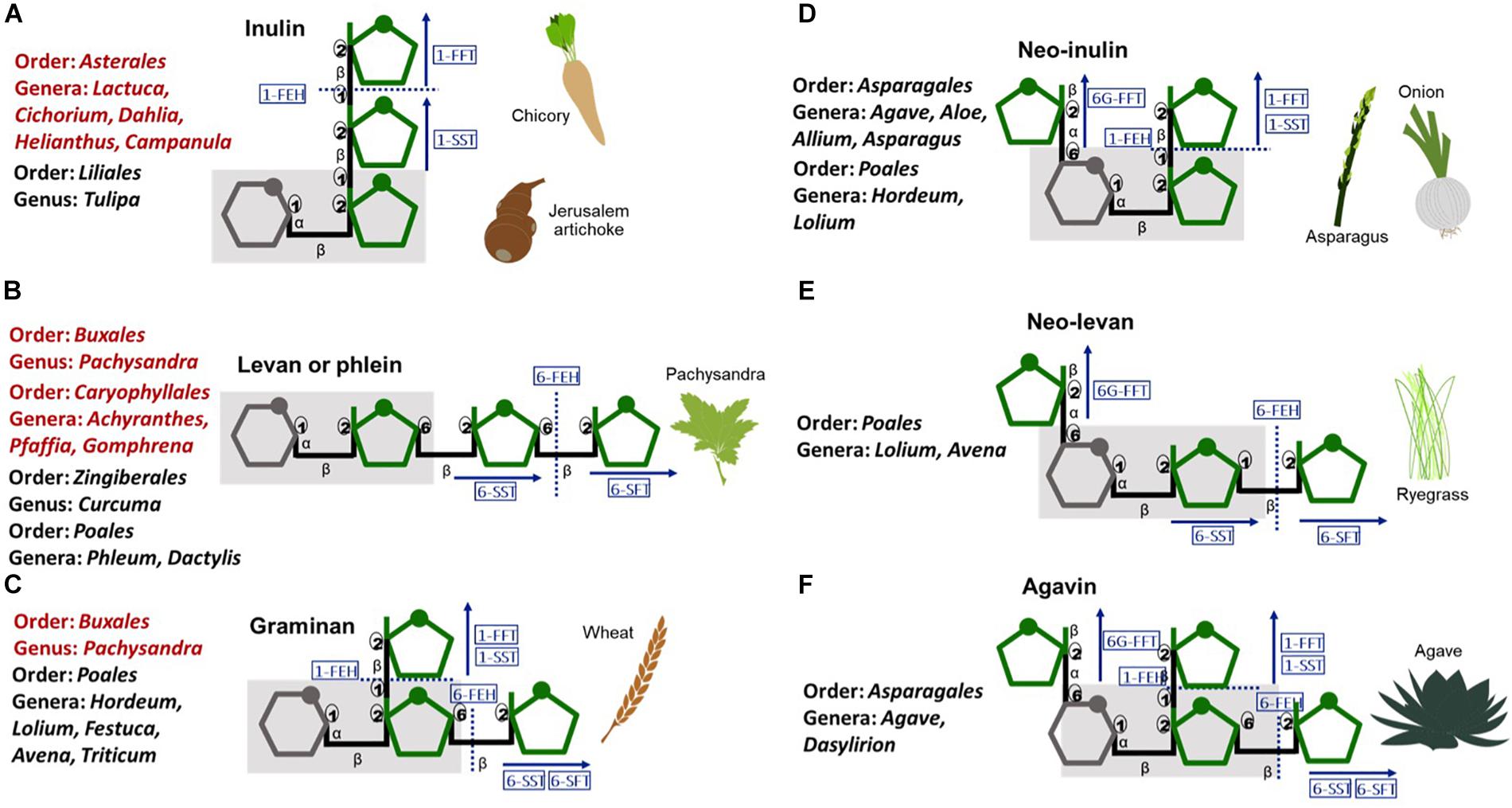
Figure 1. Schematic representation of plant fructans, their structural diversity and the enzymes involved in their metabolism. (A) linear inulin and (B) levan, (C) branched graminan, (D) neo-inulin, (E) neo-levan, and (F) highly branched agavin. Gray-glucose, green-fructose, gray shadow-sucrose moiety. Blue rectangles-enzymes:1-SST-sucrose:sucrose1-fructosyltransferase, 1-FFT-fructan:fructan1-fructosyltransferase, 6-SFT-sucrose:fructan 6-fructosyltransferase, 6G-FFT-fructan:fructan 6Gfructosyltransferase, FEH-fructan exohydrolase. Red text-dicotyledons, Black text-monocotyledons.
Fructans are water soluble, flexible fructose based polymers synthesized from sucrose and accumulating in the vacuole. They can act as a long-term reserve carbohydrate in some plant species, alone or in combination with starch.
Fructans are an alternative to starch for long-term carbohydrate storage. Starch, composed of linear amylose or branched amylopectin glucose (hexose) polymers, accumulates in chloroplasts, whereas fructans produced by adding fructose monomers to sucrose are stored in vacuoles. Fructans are structurally flexible, highly soluble, accumulate to high levels, and have the ability to associate with cell membranes (Van den Ende, 2013). These properties are intrinsic to their roles in response to stress (Versluys et al., 2018) or developmental signals (Bolouri Moghaddam and Van den Ende, 2013). Fructans are exploited commercially as a replacement for sugar or fats, as fiber or prebiotics (Vijn and Smeekens, 1999) and have useful properties for drug delivery and cryoprotection (Audouy et al., 2011; Gupta et al., 2019).
Agaves evolved during the Miocene period and synthesis and storage of fructans was an important factor in adaptation to drier environments (Arakaki et al., 2011). Agave species range from the Canadian/United States border to the Northern region of South America (Gentry, 1982; Garcia, 2007). Whereas some species such as A. deserti or A. americana are adapted to wide temperature ranges others such as A. tequilana will not thrive at temperatures below −4°C or above 36°C (Nobel et al., 1998), demonstrating that tolerance mechanisms are complex.
Artificial selection of Agaves mainly took place in Mexico where 58% of species are endemic (Gentry, 1982; Garcia, 2007). Pre-Columbian cultures exploited these plants for food, fiber, construction and beverages and they were essential elements of nomadic life styles. Agave fructans provide the raw material for production of tequila and mescal, are being developed as components of treatments for diabetes and obesity (Franco-Robles et al., 2019) and as a resource for low-cost, carbon neutral production of bioenergy (Niechayev et al., 2019).
Agave Fructans
The presence of fructans in Agave species was first recorded by Ekstrand and Johanson in 1888 as cited by Suzuki and Chatterton, 1993. In common with other members of the order Asparagales, Agave species synthesis inulin and neo series fructan polymers (Figure 1) and a new class of neofructans (subsequently known as “agavins”) was first identified in A. tequilana (Mancilla-Margalli and Lopez, 2006). Agavins are the most complex plant fructans described to date, comprising neoseries type fructans elongated at all three possible linkages (Figure 1). The composition of the fructan pool in A. tequilana varies as plants age, with agavins increasing in abundance in relation to inulins (Mellado-Mojica and Lopez, 2012).
In Agave leaves starch accumulation is largely limited to stomatal guard cells with minimal accumulation in other leaf cells (Zavala-Garcia et al., 2018). The presence of oligofructans containing 3–5 fructan residues (3–5 degrees of polymerization, D.P.) in Agave leaves indicates that sucrose produced by photosynthesis is metabolized to produce fructans rather than starch (Wang and Nobel, 1998) have shown that these oligofructans can accumulate in vascular tissue and are transported through the phloem. Although the transport mechanism is unknown, it is most plausibly by polymer trapping (Zhang and Turgeon, 2018). However, the presence of fructans in the extracellular space (apoplast) (Raveh et al., 1998) and putative roles in defense, signaling and membrane protection indicate that an apoplastic mechanism cannot be ruled out.
Oligofructans and/or sucrose transported from leaves are either metabolized to starch that accumulates in the peripheral meristem region between the leaf base and the stem (Zavala-Garcia et al., 2018; Figure 2) or converted to complex fructans for long-term storage in the vacuoles of stem tissue (Mellado-Mojica et al., 2017).
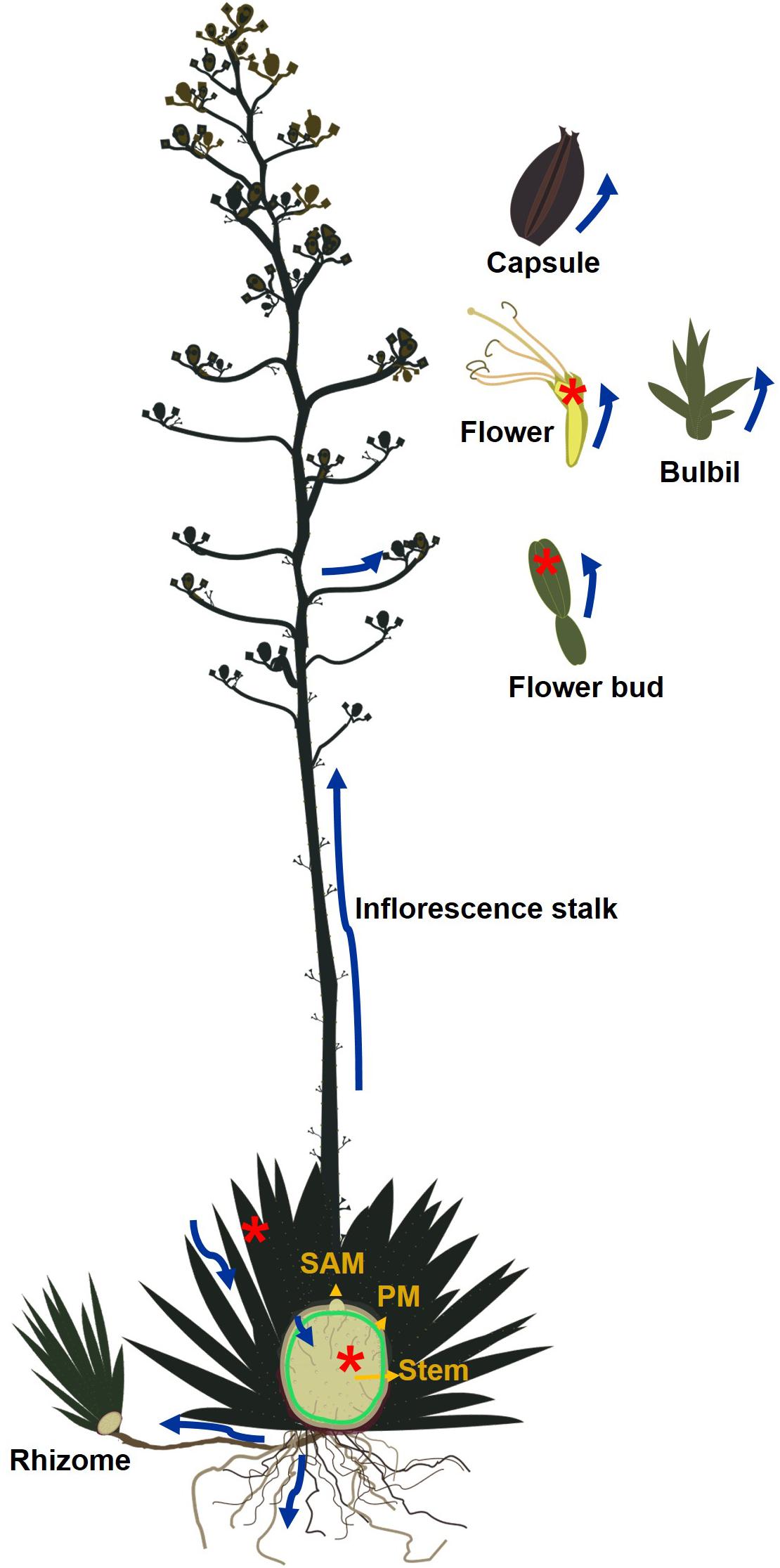
Figure 2. Fructan and/or sucrose mobility throughout an Agave plant. Blue arrows indicate fructan movement, green circle indicates the starch layer and peripheral meristem. Red asterisks indicate tissues where agavins are synthesized. SAM-shoot apical meristem. PM-Peripheral meristem.
Fructan Metabolism in Agave Species
To synthesize agavins and inulins 4 fructosyltransferase (FT) activities (1-SST, 1-FFT, 6-SFT, and 6G-FFT, Figure 1) are needed whereas degradation of fructans is carried out by fructan exohydrolases (FEH) that may be specific for β 1→2 or β 1→6 linkages or act on both. FT and FEH in common with vacuolar and cell wall invertases are members of Plant Glycoside Hydrolase Family 32 (PGHF32). The first Agave FT to be characterized was a 1-SST from A. tequilana (Avila-Fernandez et al., 2007) and by RNAseq 15 members of PGHF32 from A. tequilana, A. deserti, and A. victoriae-reginae were later identified (Avila De Dios et al., 2015). Sequence based predictions of enzyme activities have also been confirmed for some A. tequilana enzymes using the P. pastoris system (Cortes-Romero et al., 2012). cDNAs encoding 6-SFT or 1-FFT type enzymes have not yet been conclusively identified perhaps due to low or tissue specific expression. Alternatively, some Agave FT enzymes may have multiple activities as reported for a 6G-FFT from onion (Weyens et al., 2004). In silico modeling supports this hypothesis since (Huang et al., 2018) have shown that the predicted structure of an A. tequilana 6G-FFT differs from those identified in A. deserti and A. sisalana.
In silico expression patterns for genes encoding invertases and FEH across three different Agave species (A. tequilana, A. striata and A. victoriae-reginae) are consistent, whereas FT expression is highly variable (Avila De Dios et al., 2015). For example isoforms encoding 1-SST enzymes from A. tequilana and A. striata showed similar tissue specific patterns whereas those identified for A. victoriae-reginae varied widely and 6G-FFT encoding genes of A. victoriae-reginae and A. striata are strongly expressed in vegetative tissue in contrast to A. tequilana. Expression patterns for all three Agave species showed high levels of expression for both FT and FEH in floral tissue (Avila De Dios et al., 2015) suggesting that fructans are not only being degraded but are also being synthesized in these organs.
Transcriptome analysis of the vegetative to reproductive transition in A. tequilana revealed no differential expression for starch metabolism related genes (Zavala-Garcia et al., 2018) whereas fructan related genes are highly expressed in SAM tissue in comparison to leaf tissue. In particular a 6G-FFT isoform is specifically and strongly expressed at the initial stage of the reproductive phase (Avila De Dios et al., 2019).
Biological Functions of Agave Fructans
In Agave species fructans provide a source of carbohydrates for the vegetative to reproductive transition. Inflorescences can grow at a rate of 4–10 cms per day to reach 10 m or more (Valenzuela, 2003) and produce thousands of flowers, capsules, and seeds (Escobar-Guzman et al., 2008). Under cultivation, inflorescences are removed to avoid depletion of fructan reserves. Delgado Sandoval et al., 2012, showed that as the reproductive stage initiates, development of photosynthetically active leaves is suppressed and the SAM differentiates. Genes encoding FEH and invertases increase their expression during bolting (Avila De Dios et al., 2019) and leaves and stems senesce indicating that carbohydrate reserves are being harnessed for flowering.
Fructan reserves are also exploited during asexual reproduction since suckers produced from rhizomes or bulbils produced on inflorescences (Figure 2) also benefit from carbohydrates stored in the mother plant and may not survive if detached too early (Szarek et al., 1996). To accomplish these functions fructans must be mobilized over significant distances. Active fructan metabolism in floral tissue suggests carbohydrate availability could be limited by the rate of turnover or transport. Fructans may act as precursors to nectar production in floral tissue since A. palmeri produces 74 mg of nectar/flower composed mainly of glucose and fructose (Riffell et al., 2008). Alternatively fructans may be involved in generating osmolarity fluxes that lead to flower opening as described for Campanula rapunculoides (Vergauwen et al., 2000).
Agaves are perennial, monocarpic species with life cycles of 5 to over 50 years. They remain unresponsive to cues such as photoperiod or temperature, which induce flowering in annual or polycarpic species and probably respond to age-determined signals involving carbohydrate regulation. It could be speculated that accumulation of specific agavins produced by the 6G-FFT isoform described above may serve as age related molecular signals (Salinas et al., 2016) have also shown that neofructan levels increase in drought stressed A. barbadensis suggesting that neofructans play important functional roles.
The natural habitat of Agave species is in marginal desert terrain. Localization of fructans in hydrenchyma tissue in succulent A. victoria-reginae leaves (Singh et al., 2020) supports the evolution of fructan accumulation as an adaptation of Agavaceae to arid conditions. Consistent with these observations (Morales-Hernandez et al., 2019) showed that Agave fructans have a higher hydration shell in comparison to inulin and have predicted bioprotectant properties equivalent to trehalose. Suarez-Gonzalez et al., 2014 have shown that A. tequilana and A. inaequidens respond to cold and elicitors by increased FT expression and fructan production, consistent with roles in stress tolerance mechanisms.
Discussion and Perspectives
Biochemical analysis has shown the presence of fructans in all organs of different Agave species and the quantity and complexity of these polymers varies depending on specific tissue and plant age. The monocarpic, perennial life cycle, large genome size and lack of molecular tools for Agave species have hampered molecular/genetic analysis, however, transcriptome data has allowed preliminary classification, and characterization of cDNAs and enzymes involved in fructan metabolism. The failure to identify 2 key enzymes may reflect low or transient gene expression or multiple enzyme activities. Functional genetic analysis in Agave is inefficient but heterologous systems such as A. thaliana and P. pastoris are being exploited and development of a genome sequence will resolve questions regarding isoforms, gene structure and regulatory elements. Comparisons of fructan metabolism on an evolutionary level between related taxa such as Yucca and Aloe spp. and aspects of coevolution with nectar feeding pollinators will also be feasible. Sub-cellular localization of FT or FEH enzymes, detailed gene expression patterns and aspects of fructan mobility must also be addressed to provide insights to roles in signaling and stress tolerance.
Agaves represent an invaluable resource in relation to development of agricultural systems on marginal land with resilience to climate change. However, indiscriminate collection of wild plants leads to decimation of natural populations and their pollinators. Fructans are the basis for the commercial exploitation of Agaves, therefore, understanding Agave fructan metabolism, its multiple roles in the Agave life-cycle and in adaptation to different habitats will facilitate strategies for exploitation and conservation. The current challenge in Mexico is how to exploit Agave fructans under a profitable, sustainable and socially pertinent agricultural system.
Author Contributions
JS developed the outline of the manuscript. JS and AP-L wrote the manuscript and designed the figures. AP-L prepared the figures.
Funding
Funding for this research was provided by CONACyT grant CB 2013-220339 and SEP-CINVESTAV Grant# 131. AP-L received postgraduate fellowship 566173 from CONACyT.
Conflict of Interest
The authors declare that the research was conducted in the absence of any commercial or financial relationships that could be construed as a potential conflict of interest.
Acknowledgments
We are grateful to Katia Gil Vega for technical assistance.
References
Arakaki, M., Christin, P. A., Nyffeler, R., Lendel, A., Eggli, U., Ogburn, R. M., et al. (2011). Contemporaneous and recent radiations of the world’s major succulent plant lineages. Proc. Natl. Acad. Sci. U.S.A. 108, 8379–8384. doi: 10.1073/pnas.1100628108
Audouy, S. A., Van Der Schaaf, G., Hinrichs, W. L., Frijlink, H. W., Wilschut, J., and Huckriede, A. (2011). Development of a dried influenza whole inactivated virus vaccine for pulmonary immunization. Vaccine 29, 4345–4352. doi: 10.1016/j.vaccine.2011.04.029
Avila De Dios, E., Delaye, L., and Simpson, J. (2019). Transcriptome analysis of bolting in A. tequilana reveals roles for florigen. MADS, fructans and gibberellins. BMC Genomics 20:473. doi: 10.1186/s12864-019-5808-5809
Avila De Dios, E., Gomez Vargas, A. D., Damian Santos, M. L., and Simpson, J. (2015). New insights into plant glycoside hydrolase family 32 in Agave species. Front. Plant Sci. 6:594. doi: 10.3389/fpls.2015.00594
Avila-Fernandez, A., Olvera-Carranza, C., Rudino-Pinera, E., Cassab, G. I., Nieto-Sotelo, J., and Lopez-Munguia, A. (2007). Molecular characterization of sucrose: sucrose 1-fructosyltransferase (1-SST) from Agave tequilana Weber var. azul. Plant Sci. 173, 478–486. doi: 10.1016/j.plantsci.2007.07.009
Bolouri Moghaddam, M. R., and Van den Ende, W. (2013). Sugars, the clock and transition to flowering. Front. Plant Sci. 4:22. doi: 10.3389/fpls.2013.00022
Cortes-Romero, C., Martinez-Hernandez, A., Mellado-Mojica, E., Lopez, M. G., and Simpson, J. (2012). Molecular and functional characterization of novel fructosyltransferases and invertases from Agave tequilana. PLoS One 7:e35878. doi: 10.1371/journal.pone.0035878
Delgado Sandoval, S. C., Juárez, M. J. A., and Simpson, J. (2012). Agave tequilana MADS genes show novel expression patterns in meristems, developing bulbils and floral organs. Sex. Plant Reprod. 25, 11–26. doi: 10.1007/s00497-011-0176-x
Escobar-Guzman, R. E., Hernandez, F. Z., Vega, K. G., and Simpson, J. (2008). Seed production and gametophyte formation in Agave tequilana and Agave americana. Botany-Botanique 86, 1343–1353. doi: 10.1139/B08-099
Franco-Robles, E., Ramirez-Emiliano, J., and Lopez, M. G. (2019). Agave fructans and oligofructose decrease oxidative stress in brain regions involved in learning and memory of overweight mice. Nat. Prod. Res. 33, 1527–1530. doi: 10.1080/14786419.2017.1423297
Garcia, J. (2007). Los Agaves de México. Red de Revistas Científicas de América Latina Y El Caribe, España Y Portugal 87, 14–23.
Gupta, N., Jangid, A. K., Pooja, D., and Kulhari, H. (2019). Inulin: a novel and stretchy polysaccharide tool for biomedical and nutritional applications. Int. J. Biol. Macromol. 132, 852–863. doi: 10.1016/j.ijbiomac.2019.03.188
Hendry, G. A. F. (1993). Evolutionary origins and natural functions of fructans - a climatological, biogeographic and mechanistic appraisal. New Phytol. 123, 3–14.
Huang, X., Wang, B., Xi, J., Zhang, Y., He, C., Zheng, J., et al. (2018). Transcriptome comparison reveals distinct selection patterns in domesticated and wild Agave species, the important CAM plants. Int. J. Genomics 2018:5716518. doi: 10.1155/2018/5716518
Mancilla-Margalli, N. A., and Lopez, M. G. (2006). Water-soluble carbohydrates and fructan structure patterns from Agave and Dasylirion species. J. Agric. Food Chem. 54, 7832–7839. doi: 10.1021/jf060354v
Mellado-Mojica, E., Gonzalez De La Vara, L. E., and Lopez, M. G. (2017). Fructan active enzymes (FAZY) activities and biosynthesis of fructooligosaccharides in the vacuoles of Agave tequilana weber blue variety plants of different age. Planta 245, 265–281. doi: 10.1007/s00425-016-2602-2607
Mellado-Mojica, E., and Lopez, M. G. (2012). Fructan metabolism in A. tequilana Weber Blue variety along its developmental cycle in the field. J. Agric. Food Chem. 60, 11704–11713. doi: 10.1021/jf303332n
Morales-Hernandez, J. A., Singh, A. K., Villanueva-Rodriguez, S. J., and Castro-Camus, E. (2019). Hydration shells of carbohydrate polymers studied by calorimetry and terahertz spectroscopy. Food Chem. 291, 94–100. doi: 10.1016/j.foodchem.2019.03.132
Niechayev, N. A., Jones, A. M., Rosenthal, D. M., and Davis, S. C. (2019). A model of environmental limitations on production of Agave americana L. grown as a biofuel crop in semi-arid regions. J. Exp. Bot. 70, 6549–6559. doi: 10.1093/jxb/ery383
Nobel, P. S., Castaneda, M., North, G., Pimienta-Barrios, E., and Ruiz, A. (1998). Temperature influences on leaf CO2 exchange, cell viability and cultivation range for Agave tequilana. J. Arid Environ. 39, 1–9. doi: 10.1006/Jare.1998.0374
Raveh, E., Wang, N., and Nobel, P. S. (1998). Gas exchange and metabolite fluctuations in green and yellow bands of variegated leaves of the monocotyledonous CAM species Agave americana. Physiologia Plantarum 103, 99–106. doi: 10.1034/j.1399-3054.1998.1030112.x
Riffell, J. A., Alarcon, R., Abrell, L., Davidowitz, G., Bronstein, J. L., and Hildebrand, J. G. (2008). Behavioral consequences of innate preferences and olfactory learning in hawkmoth-flower interactions. Proc. Natl. Acad. Sci. U.S.A. 105, 3404–3409. doi: 10.1073/pnas.0709811105
Salinas, C., Handford, M., Pauly, M., Dupree, P., and Cardemil, L. (2016). Structural modifications of fructans in aloe barbadensis miller (Aloe Vera) grown under water stress. PLoS One 11:e0159819. doi: 10.1371/journal.pone.0159819
Singh, A. K., Pérez-López, A. V., Simpson, J., and Castro-Camus, E. (2020). Three-dimensional water mapping of succulent Agave victoriae-reginae leaves by terahertz imaging. Sci. Rep. 10:1404. doi: 10.1038/s41598-020-58277-z
Suarez-Gonzalez, E. M., Lopez, M. G., Delano-Frier, J. P., and Gomez-Leyva, J. F. (2014). Expression of the 1-SST and 1-FFT genes and consequent fructan accumulation in Agave tequilana and A. inaequidens is differentially induced by diverse (a)biotic-stress related elicitors. J. Plant Physiol. 171, 359–372. doi: 10.1016/j.jplph.2013.08.002
Suzuki, M., and Chatterton, N. J. (1993). Science and Technology of Fructans. Boca Raton: CRC Press.
Szarek, S. R., Driscoll, B., Shohet, C., and Priebe, S. (1996). Bulbil production in Agave (Agavaceeae) and related genera. Southwest. Nat. 41, 465–469.
Valenzuela, A. (2003). El agave Tequilero: Su Cultivo e Industria de México. México: Mundiprensa Editores.
Van den Ende, W. (2013). Multifunctional fructans and raffinose family oligosaccharides. Front. Plant Sci. 4:247. doi: 10.3389/fpls.2013.00247
Vergauwen, R., Van den Ende, W., and Van Laere, A. (2000). The role of fructan in flowering of Campanula rapunculoides. J. Exp. Bot. 51, 1261–1266. doi: 10.1093/Jexbot/51.348.1261
Versluys, M., Kirtel, O., Toksoy Oner, E., and Van den Ende, W. (2018). The fructan syndrome: evolutionary aspects and common themes among plants and microbes. Plant Cell Environ. 41, 16–38. doi: 10.1111/pce.13070
Vijn, I., and Smeekens, S. (1999). Fructan: more than a reserve carbohydrate? Plant Physiol. 120, 351–360.
Wang, N., and Nobel, P. S. (1998). Phloem transport of fructans in the crassulacean acid metabolism species Agave deserti. Plant Physiol. 116, 709–714.
Weyens, G., Ritsema, T., Van Dun, K., Meyer, D., Lommel, M., Lathouwers, J., et al. (2004). Production of tailor-made fructans in sugar beet by expression of onion fructosyltransferase genes. Plant Biotechnol. J. 2, 321–327. doi: 10.1111/j.1467-7652.2004.00074.x
Zavala-Garcia, L. E., Sanchez-Segura, L., Avila De Dios, E., Perez-Lopez, A., and Simpson, J. (2018). Starch accumulation is associated with active growth in A. tequilana. Plant Physiol. Biochem. 130, 623–632. doi: 10.1016/j.plaphy.2018.08.011
Keywords: Agavaceae, agavins, signaling, metabolism, adaptation
Citation: Pérez-López AV and Simpson J (2020) The Sweet Taste of Adapting to the Desert: Fructan Metabolism in Agave Species. Front. Plant Sci. 11:324. doi: 10.3389/fpls.2020.00324
Received: 17 December 2019; Accepted: 05 March 2020;
Published: 24 March 2020.
Edited by:
Luis Enrique Eguiarte, National Autonomous University of Mexico, MexicoReviewed by:
Wim Van den Ende, KU Leuven, BelgiumGretchen North, Occidental College, United States
Cristobal N. Aguilar, Universidad Autónoma de Coahuila, Mexico
Copyright © 2020 Pérez-López and Simpson. This is an open-access article distributed under the terms of the Creative Commons Attribution License (CC BY). The use, distribution or reproduction in other forums is permitted, provided the original author(s) and the copyright owner(s) are credited and that the original publication in this journal is cited, in accordance with accepted academic practice. No use, distribution or reproduction is permitted which does not comply with these terms.
*Correspondence: June Simpson, june.simpson@cinvestav.mx