- 1School of Environment, State Key Joint Laboratory of Environmental Simulation and Pollution Control, Beijing Normal University, Beijing, China
- 2School of Life and Geographic Sciences, Qinghai Normal University, Xining, China
- 3Qinghai Academy of Animal Husbandry and Veterinary Science, Qinghai University, Xining, China
- 4Northwest Institute of Plateau Biology, Key Laboratory of Restoration Ecology of Cold Are in Qinghai Province, Chinese Academy of Science, Xining, China
- 5Research Department, Earth University, San José, Costa Rica
Warming and Nitrogen (N) deposition are key global changes that may affect eco-physiological process of territorial plants. In this paper, we examined the effects of warming, N deposition, and their combination effect on the physiological performances of Leymus secalinus. Four treatments were established in an alpine meadow of Qinghai-Tibetan plateau: control (CK), warming (W), N deposition (N), and warming plus N deposition (NW). Warming significantly decreased the photosynthetic rate (Anet), stomatal conductance (gs), intercellular CO2 concentration (Ci), and transpiration rate (Tr), while N deposition and warming plus N deposition significantly increased those parameters of L. secalinus. Warming significantly increased the VPD and Ls, while N deposition and warming plus N deposition had a significant positive effect. Warming negatively reduced the leaf N content, Chla, Chlb, and total Chl content, while N deposition significantly promoted these traits. Warming, N deposition, and their combination significantly increased the activity of SOD, POD, and CAT. Besides, warming and warming plus N deposition significantly increased the MDA content, while N deposition significantly decreased the MDA content. N deposition and warming plus N deposition significantly increased the Rubisco activity, while warming showed no significant effect on Rubisco activity. N deposition and warming plus N deposition significantly increased the Fv/Fm, ΦPSII, qP, and decreased NPQ, while warming significantly decreased the Fv/Fm, ΦPSII, qP, and increased NPQ. N deposition strengthened the relations between gs, Chl, Chla, Chlb, Rubisco activity, and Anet. Under warming, only gs showed a significantly positive relation with Anet. Our findings suggested that warming could impair the photosynthetic potential of L. secalinus enhanced by N deposition. Additionally, the combination of warming and N deposition still tend to lead positive effects on L. secalinus.
Introduction
Climate warming and Nitrogen (N) deposition, as two major factors that drive global change, are predicted to increase simultaneously in the future (Zong et al., 2018a). The global surface temperature has been significantly increased since the industrial revolution and predicted to increase by approximately 0.9°C-5.4°C in this century (IPCC, 2013). Grassland, the dominant ecosystem which cover over 60% of local territory (Dong and Sherman, 2015), is mainly distributed in semi-dry or dry areas in the QTP (Qin et al., 2014) and is very vulnerable to global changes (Chen et al., 2014).
Climate warming has been considered as one of the highly influential factors that pose a threat to ecological integrity and function (Hughes et al., 2018). It can influence carbon sequestration in terrestrial ecosystems, especially alpine ecosystems (Ganjurjav et al., 2018). Warming and drying over the QTP is becoming serious for decades (Chen et al., 2014), which may affect plant physiological processes such as photosynthesis and enzymatic antioxidant (Shi et al., 2010; Yu et al., 2019). Generally, photosynthetic process and the antioxidant enzyme are the most sensitive to climate warming (Atkin et al., 2006). A recent study has reported that warming can significantly decrease plant stomatal conductance on the QTP (Zhang et al., 2010), thus affecting plant photosynthesis. Until now, warming effects on plant photosynthesis remain controversial (Niu et al., 2008); e.g., positive (Shaw et al., 2000), neutral (Loik et al., 2000), and negative (He and Dong, 2003) effects have been reported by previous researchers. Warming can increase the temperature of air and soil, and reduce soil water content (Allison et al., 2018; Samaniego et al., 2018), finally leading to dramatic ecological effects on plants. Previous study found that warming-induced increase of soil temperature and decrease of soil moisture can lead to declined biomass accumulation in herbaceous perennial plants (Harte and Shaw, 1995). Besides, plant water potential may be impaired under warming by a reduction in stomatal conductance and photosynthetic CO2 assimilation, which leads to a lower photosynthetic rate and more non-photochemical quenching (Loik et al., 2000). Additionally, warming-induced water stress can lead to photoprotection of PSII, which is important for plant adaptation (Demmig-Adams and Adams, 1996), as downregulation of PSII reaction centers and non-photochemical quenching under environmental stress is of high significance (Valladares and Pearcy, 1997). Many efforts have been made to examine the effects of climate warming on growth and physiological process of temperate plants (Nakaji et al., 2001), yet little is known about the effects of climate warming on plant physiology of alpine grassland. Plant physiology is highly sensitive to temperature, a slight change in temperature may lead to a significant alteration of plant physiological process (Atkin et al., 2006). Therefore, a better understanding of ecosystem responses to warming from the perspective of plant physiological acclimation is critically important.
N deposition is another important component of global change. Though high latitude regions are usually N-limited (Marañón-Jiménez et al., 2019), the rate of N deposition on Qinghai-Tibetan plateau has significantly increased in recent decades (Zhang et al., 2018a). However, the influences of N deposition on plant performance are still unclear. Photosynthesis is strongly influenced by N availability (Wang et al., 2018), e.g., change of leaf N content can alter the ribulose-1, 5-bisphosphate carboxylase/oxygenase (Rubisco) activity and thus affect plant photosynthesis (Cheng and Fuchigami, 2000). N deposition could regulate plant responses to warming (Zong et al., 2018b), and change in temperature is also likely to interact with the effects of N deposition (Suddick et al., 2013). Up to now, little is known about the physiological responses of plants under warming and N deposition.
Leymus secalinus is one of the key grass species in alpine grasslands of the QTP (Mou et al., 2018). Through field investigation in our study site, we found that L. secalinus is the most dominant grass species accounting for nearly 50% of the total coverage under each treatment. Therefore, we choose this species as our model plant to investigate the effects of warming and N deposition on plants. We assumed that climate warming and N deposition can alter photosynthetic potential of L. secalinus through changing leaf nutrients, gas exchange, photosynthetic enzyme, antioxidant metabolism, and chlorophyll fluorescence. To test this hypothesis, we conducted this study with the objective of disclosing the possible adaptable mechanism in physiology of L. secalinus under warming and N deposition. Our objectives were to: 1) determine the physiological performances of L. secalinus response to warming and N deposition 2) explore main factors that determine the alteration of L. secalinus photosynthesis under warming and N deposition 3) disclose the direct and indirect path that lead to the variation of Anet under warming and N deposition. The study is helpful to better understand the latent mechanisms of adaptation of alpine grassland plants to N deposition and climate warming in the future.
Material and Methods
Study Sites
This study was conducted in Xihai Town, Haiyan County (100°57′E, 36°56′N, 3100 m ASL), which is located at the eastern side of Qinghai Lake with mean annual temperature about 1.4°C, mean annual precipitation about 330-370 mm, and annual evaporation capacity around 1400 mm. The soil was mostly loam-clay (Zhao et al., 2017) (Figure 1).
Experimental Design
A complete randomized block factorial experimental design was used. Four treatments: control treatment (CK), warming alone (W), N deposition alone (N), and warming plus N deposition (NW) were established for the experiment in this study. In early 2015, we fenced twelve 2 m × 5 m plots in an alpine meadow of Qinghai-Tibetan plateau. Three plots (replicates) were randomly selected for the control treatments; three plots (replicates) were randomly selected and treated with ammonium nitrate (NH4NO3) of 8 kg N ha−1 year−1, which is the annual N deposition of this area (Lü and Tian, 2007); and another three plots (replicates) were randomly selected for the warming treatment by placing the open top chambers (OTC), in which the mean temperature in July (growing season) was about 2°C higher than the outside (Table 1). The remaining three plots were randomly selected for warming plus N deposition by placing the open-top chambers (OTC) and fertilizing with 8 kg N ha−1 year−1 NH4NO3. The interval between plots was 1 m. All the plots were similar in the topographies and land use histories. The treatments of warming and nitrogen application treatments were initiated since early May of 2015, and the corresponding plots were fertilized with ammonium nitrate (NH4NO3) in early May and July every year. Soil temperature and moisture were measured using digital temperature sensors (Table 1).
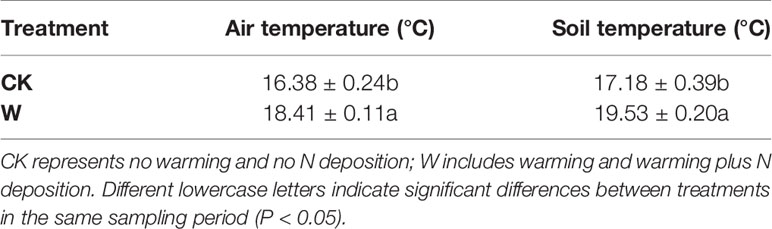
Table 1 Means ( ± SE) of air temperature (°C) and soil temperature (°C) in July 2018 in the study plots.
Sample Collection
Plant samples were collected in July 2018 (growing season). Nine L. secalinus with similar growth status were chosen, and the completely expanded and intact leaves of each plant were used for gas exchange and chlorophyll fluorescence measurement, then the leaves were wrapped in aluminum foil, immediately immersed in liquid nitrogen, and stored at -80°C for laboratory analysis.
MDA Content Measurement
The malondialdehyde (MDA) content was measured according to the method of thiobarbituric acid (TBA) (Heath and Packer, 1965) with some modifications. The liquid nitrogen-stored leaves of L. secalinus were stored thawed and 0.1 g of fresh leaves were ground in a ceramic mortar. Liquid nitrogen and 5 ml 10% trichloroacetic acid (TCA) were added into the mortar until the formation of a homogeneous powder. The homogenate was centrifuged at 4000 × g for 15 min at 4°C, then 2 ml supernatant was mixed with 5 ml of 0.6% TBA in 10% TCA and heated for 15 min, cooled in an ice bath to room temperature, and centrifuged once again. Finally, the absorbance was measured at 450, 532, and 600 nm. The MDA content was measured according to the below formula: MDA (μmol·L-1) = 6.45 (A532-A600) - 0.56A450, and then expressed as μmol·g−1 fresh weight.
Chlorophyll Extraction
The chlorophyll and carotenoid contents in the L. secalinus leaves of each treatment were extracted with 80% acetone according to Lichtenthaler (1987). The absorbance of chlorophyll a (Chla) and chlorophyll b (Chlb) was measured at 663 nm and 646 nm, respectively, and their concentrations (mg·g-1 FW) were calculated according to the following equations:
Gas Exchange Parameters Measurement
Fully expanded and exposed leaves of selected samples were measured between 9:00 and 11:00 (local time) in each plot on sunny days using a portable photosynthesis system (Li6400XT, Li-Cor, Inc., Lincoln, NE, USA). Three individual plants in a similar healthy state per replicate were selected for sampling. Three intact leaves of each individual were measured for statistical analysis. The photon flux density (PFD) was maintained at 1500 μmol·m-2·s-1 using the red-blue source of Li6400XT. In the chamber, the temperature was maintained at 25°C, with a relative humidity of 55%-70%. The CO2 concentration was maintained at 400 μmol·mol-1. The net photosynthetic rate (Anet), stomatal conductance (gs), intercellular CO2 concentration (Ci), transpiration rate (Tr), vapor pressure deficit (VPD), and stomatal limitation (Ls) were measured as Ls= 1-Ci/Ca by following Yin et al.’s (2006) methods.
Chlorophyll Fluorescence Measurement
After the measurements of gas exchange parameters, the marked leaves were wrapped in tin foil paper for dark adaptation for 30 minutes before measurement (ensuring total darkness) in order to standardize chlorophyll activity of all leaves. Then, it was measured with a portable LI-6400-40 pulse-amplitude-modulation fluorometer (Li-cor, USA). The measured fluorescence variables included the following: maximum photochemical efficiency of PSII (Fv/Fm), actual photochemical efficiency of PSII (ΦPSII), photochemical quenching coefficient (qP), and non-photochemical quenching coefficient (NPQ). Light-adapted components of chlorophyll fluorescence, including steady-state yield (F) and maximum fluorescence yield (Fm′), were measured and calculated as ΦPSII = Fm'–F/Fm' (Genty et al., 1989); dark-adapted components of chlorophyll fluorescence minimum fluorescence yield (Fo) and maximum fluorescence yield (Fm) were measured and calculated as Fv/Fm = (Fm–Fo)/Fm (Fleck et al., 1998), qP = (Fm'–F)/(Fm'–Fo') (Oxborough and Baker, 1997); and non-photochemical quenching was calculated as NPQ = (Fm/Fm′) –1(Barker and Adams, 1997).
Antioxidant Enzymes Activities Measurement
Fresh leaf samples (0.4g) were ground in liquid nitrogen using a mortar and pestle, then the ground samples were homogenized under ice-cold conditions in 4.5 mL of the extraction buffer (0.1 M phosphate buffer containing 0.5 mM ethylenediaminetetraacetic acid, pH 7.4). Then, the homogenate was centrifuged at 8000 ×g for 15 min at 4°C. The supernatant was stored at -70°C for the analysis of antioxidant enzymes. SOD activity was measured by the percentage reduction of nitroblue tetrazolium (NBT) at 560 nm (Giannopolitis and Ries, 1977). One unit of SOD activity was defined as the amount of enzyme that leads to 50% inhibition of NBT reduction. Guaiacol oxidation by H2O2 at 420 nm was measured for the POD activity (Kochhar et al., 1979). The reaction mixture contained 2.4 mL of 0.1 M potassium phosphate buffer (pH 7.4), 0.3 mL 2% H2O2, 100 µL of the enzyme extract, and 1 mL of 50 mM guaiacol. Catalase (CAT) activity was measured according to the consumption of H2O2 at 240 nm (Trevor et al., 1994). One unit of CAT activity was defined as the amount of enzyme catalyzing the decomposition of 1 nmol of H2O2 per min. The reaction mixture contained 1 mL of 0.1 M potassium phosphate buffer (pH 7.4), 50 µL of the enzyme extract, and 0.1 mL of 0.1 M H2O2.
Rubisco Activity Measurement
When measuring Rubisco activity, 0.1 g frozen leaf tissues was ground to a fine powder in mortar with liquid nitrogen according to Hu et al. (2010). 6 ml cooling extraction buffer was added, containing 50 mM Hepe-KOH (pH 7.5), 10 mM DTT, 2 mM EDTA, 10% glycerin(w/v), 1% BSA (w/v), 1% TritonX-100 (v/v), and 1.5% PVPP (w/v). Then, 1 ml homogenate was centrifuged at 14000 g for 10 min at 4°C. The supernatant was taken for Rubisco activity measurement in the 900 μl reaction medium containing 100 mM bicine (pH 8.0 at 25°C), 25 mM KHCO3, 20 mM MgCl2, 3.5 mM ATP, 5 mM phosphocreatine, 5 units glyceraldehyde-3-phosphate dehydrogenase, 5 units 3-phosphoglyceric phosphokinase, and 17.5 units creatine phosphokinase and 0.25 mM NADH. We added 50 μl enzyme extracting solution and let the solution rest for 15 min at 25°C, then added 50 μl Rubp, and recorded the absorbance every 10 s for 2 min at 340 nm.
Statistical Analysis
All data were pooled in Excel 2016. Statistical analyses of data were performed using SPSS 22.0. Analysis of variance (ANOVA) was used to detect the effects of warming, N deposition, and their interactions. Post hoc comparisons were performed using Duncan's multiple tests at a significance level of P < 0.05. R package networkD3 was used to draw the relations. Structural equation modeling (SEM) was performed to identify the direct and indirect pathways that may affect photosynthesis of L. secalinus in response to warming and N deposition, which was performed by AMOS 22.0 (Amos Development, Spring House, PA, USA).
Results
Warming and N Deposition Lead to Different Effects on the Gas Exchange
There existed a significant difference of gas exchange parameters under warming and N deposition (Figure 2). Compared with the CK, N and NW treatment significantly (P < 0.05) increased Anet of L. secalinus, while W treatment significantly (P < 0.05) decreased Anet of L. secalinus. The Anet of Leymus secalinus under NW treatment was lower than that under N treatment alone (P < 0.05, Figure 2A). Similarly, the change of gs, Ci, and Tr showed a same dynamic with the Anet under W and N treatment (Figures 2A–D). The VPD significantly increased in W treatment and decreased in N treatment (Figure 2E). Besides, the Ls showed an opposite variation with gs under W and N treatment (Figures 2B, D).
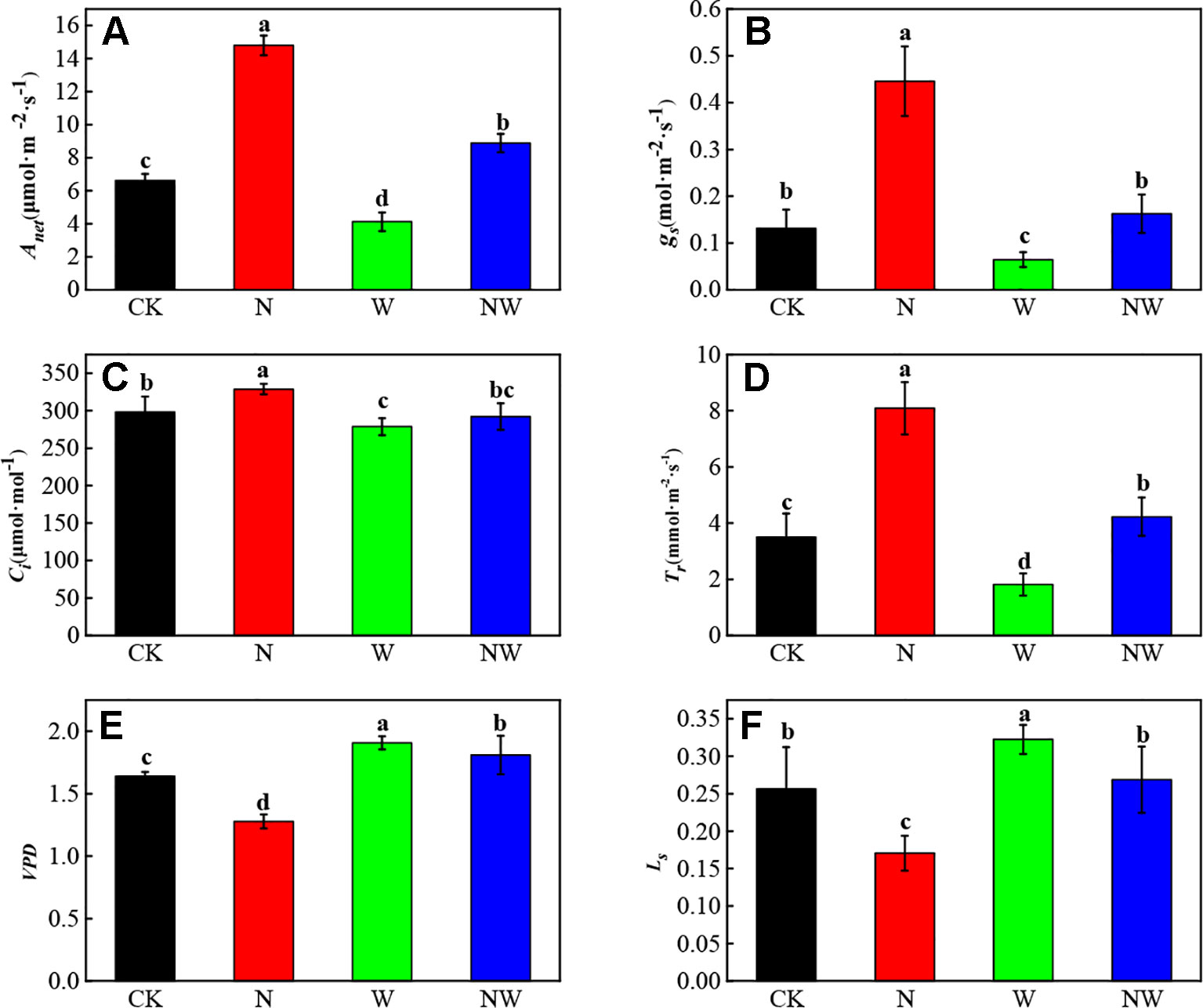
Figure 2 Effects of warming and N deposition on gas exchange parameters of L. secalinus. Vertical bars represent ± SE of the mean, and different letters on the SE bars indicate significant differences among all treatments (P < 0.05). (A) net photosynthetic rate (B) stomatal conductance (C) intercellular CO2 concentration, (D) transpiration rate (E) vapor pressure deficit (F) stomatal limitation value. Each treatment has three replicated plots, and nine leaves from nine L. secalinus with similar growth status were chosen in each plot as a biological replication for analysis (3 biological replicates were performed).
Warming and N Deposition Change in the Leaf N and Chlorophyll Content
According to Figure 3A, leaf N content significantly increased in N and NW treatment, while it decreased in W treatment compared with the CK (P < 0.05). The leaf chlorophyll content showed a similar variation with leaf N content under all treatments (Figures 3B–D).
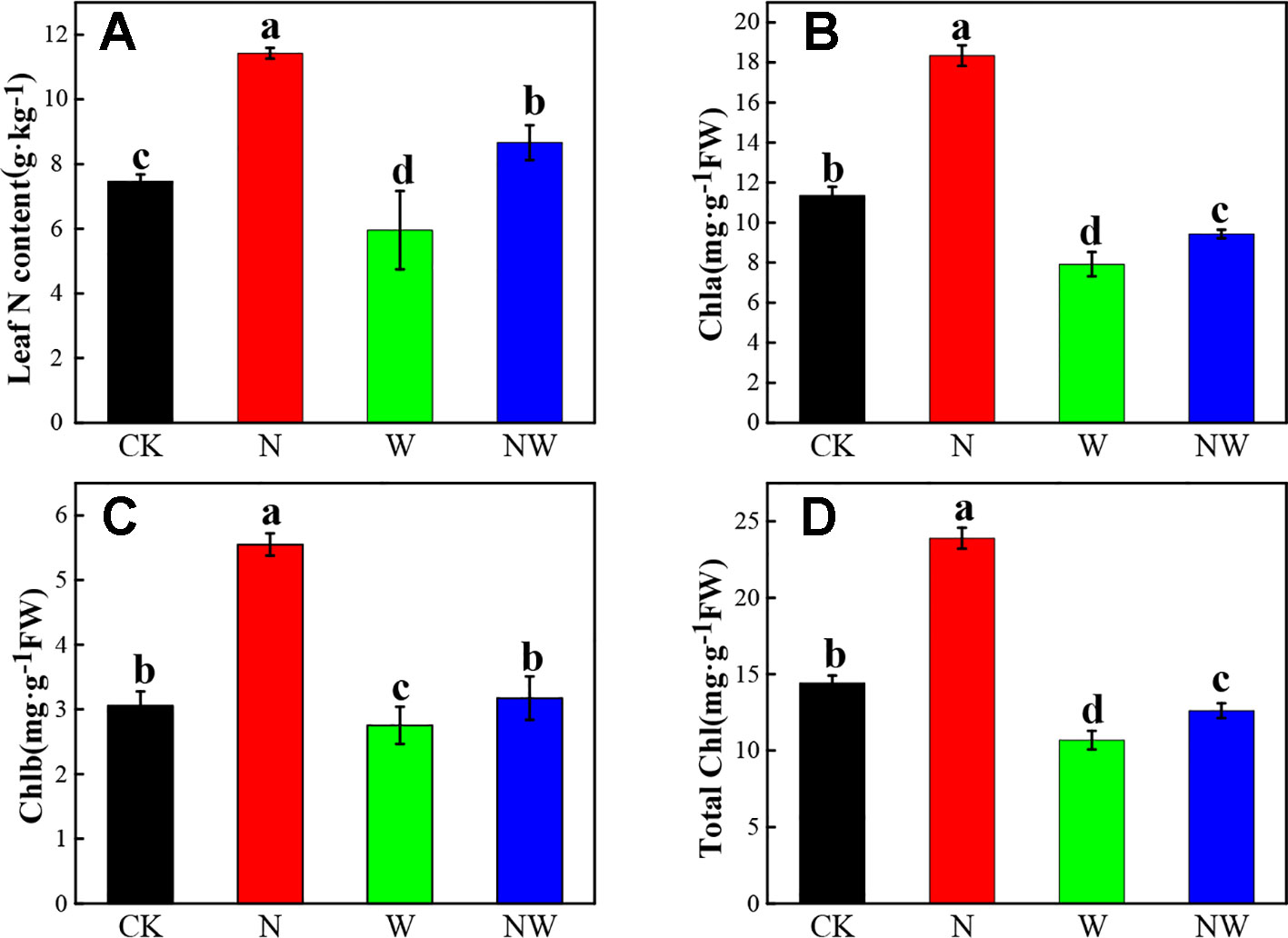
Figure 3 Effect of warming and N deposition on Leaf N and chlorophyll content of L. secalinus. Vertical bars represent ± SE of the mean, and different letters on the SE bars indicate significant differences among all treatments (P < 0.05). (A) Leaf N content (B) Chla (C) Chlb (D) total Chl. Each treatment has three replicated plots, and nine leaves from nine L. secalinus with similar growth status were chosen in each plot as a biological replication for analysis (3 biological replicates were performed).
Warming and N Deposition Change in the Antioxidant System
The MDA content of L. secalinus significantly decreased in the N treatment, while it increased in W and NW treatment (P < 0.05) (Figure 4A). Additionally, the MDA content of L. secalinus under N and NW treatment was significantly (P < 0.05) lower than that under W treatment alone. N, W and NW treatment significantly enhanced the SOD, POD, and CAT activity (P < 0.05), and the activity of three antioxidant enzymes under W treatment were highest among all the treatments (Figures 4B–D).
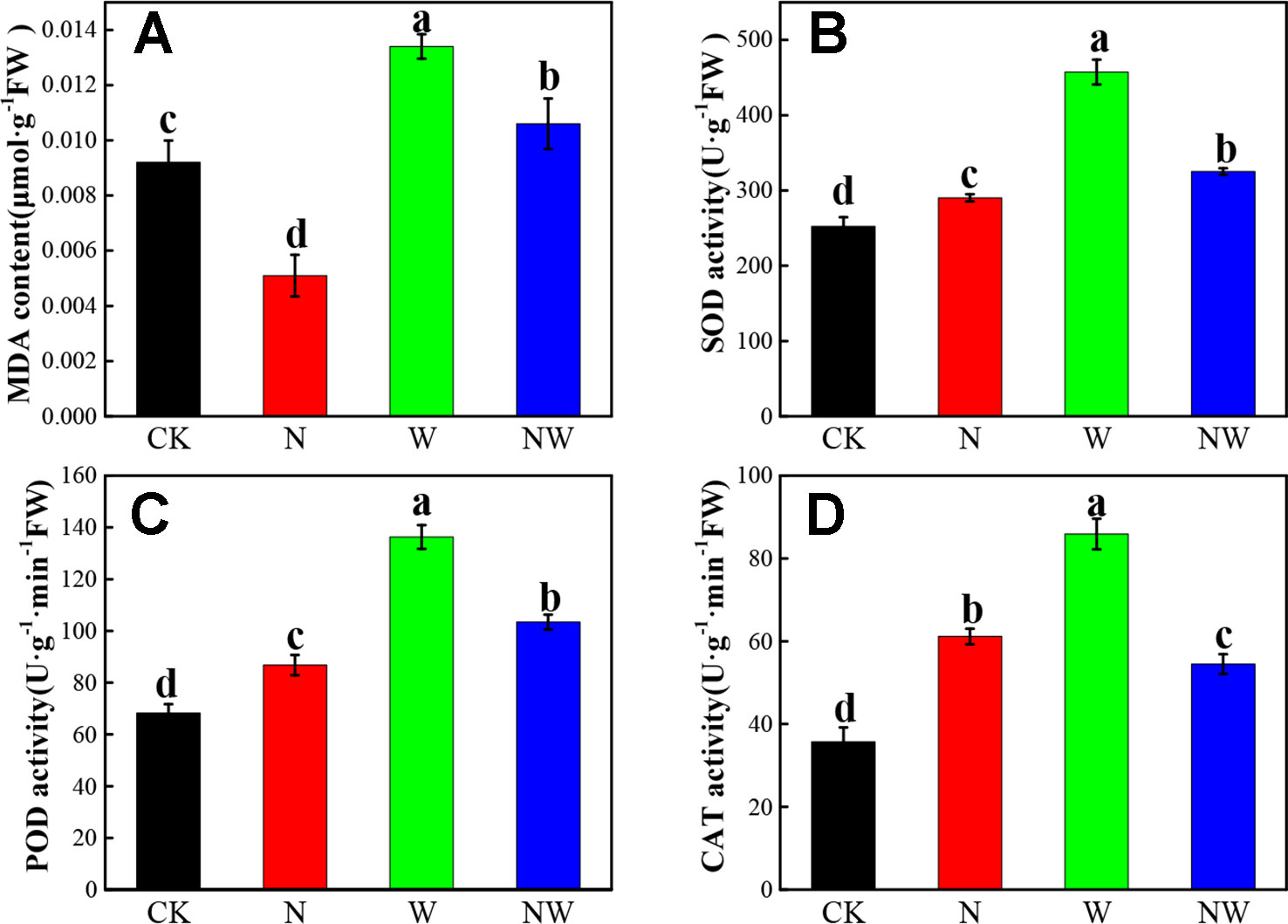
Figure 4 Effect of warming and N deposition on the MDA and antioxidant enzyme activity of L. secalinus. Vertical bars represent ± SE of the mean, and different letters on the SE bars indicate significant differences among all treatments (P < 0.05). (A) MDA content (B) SOD activity (C) POD activity (D) CAT activity. Each treatment has three replicated plots, and nine leaves from nine L. secalinus with similar growth status were chosen in each plot as a biological replication for analysis (3 biological replicates were performed).
Warming and N Deposition Lead to Differential Variation of Photosynthetic Enzyme
N and NW treatment significantly (P < 0.05) increased the Rubisco activity of L. secalinus. W treatment had no significant (P > 0.05) influence (Figure 5).
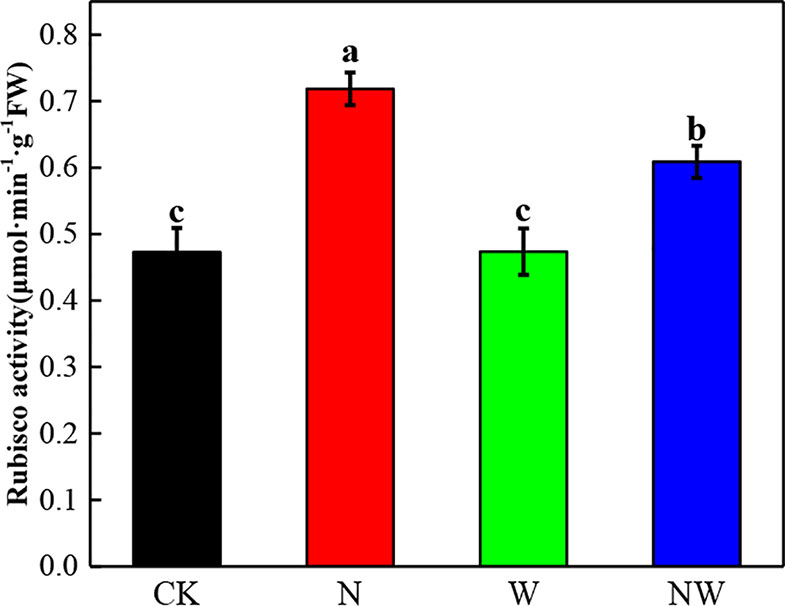
Figure 5 Effect of warming and N deposition on Rubisco activity of L. secalinus. Vertical bars represent ± SE of the mean, and different letters on the SE bars indicate significant differences among all treatments (P < 0.05). Each treatment has three replicated plots, and nine leaves from nine L. secalinus with similar growth status were chosen in each plot as a biological replication for analysis (3 biological replicates were performed).
Warming and N Deposition Lead to Obvious Variation of Chlorophyll Fluorescence
The Fv/Fm, ΦPSII, and qP of L. secalinus showed a similar variation in N and W treatment (Figures 6A, B, D). N and NW treatment significantly increased the Fv/Fm, ΦPSII, and qP of L. secalinus, while W treatment significantly decreased theses three parameters (P < 0.05). N treatment significantly decreased the NPQ, while W and NW treatment significantly increased that index (Figure 6C) (P < 0.05).
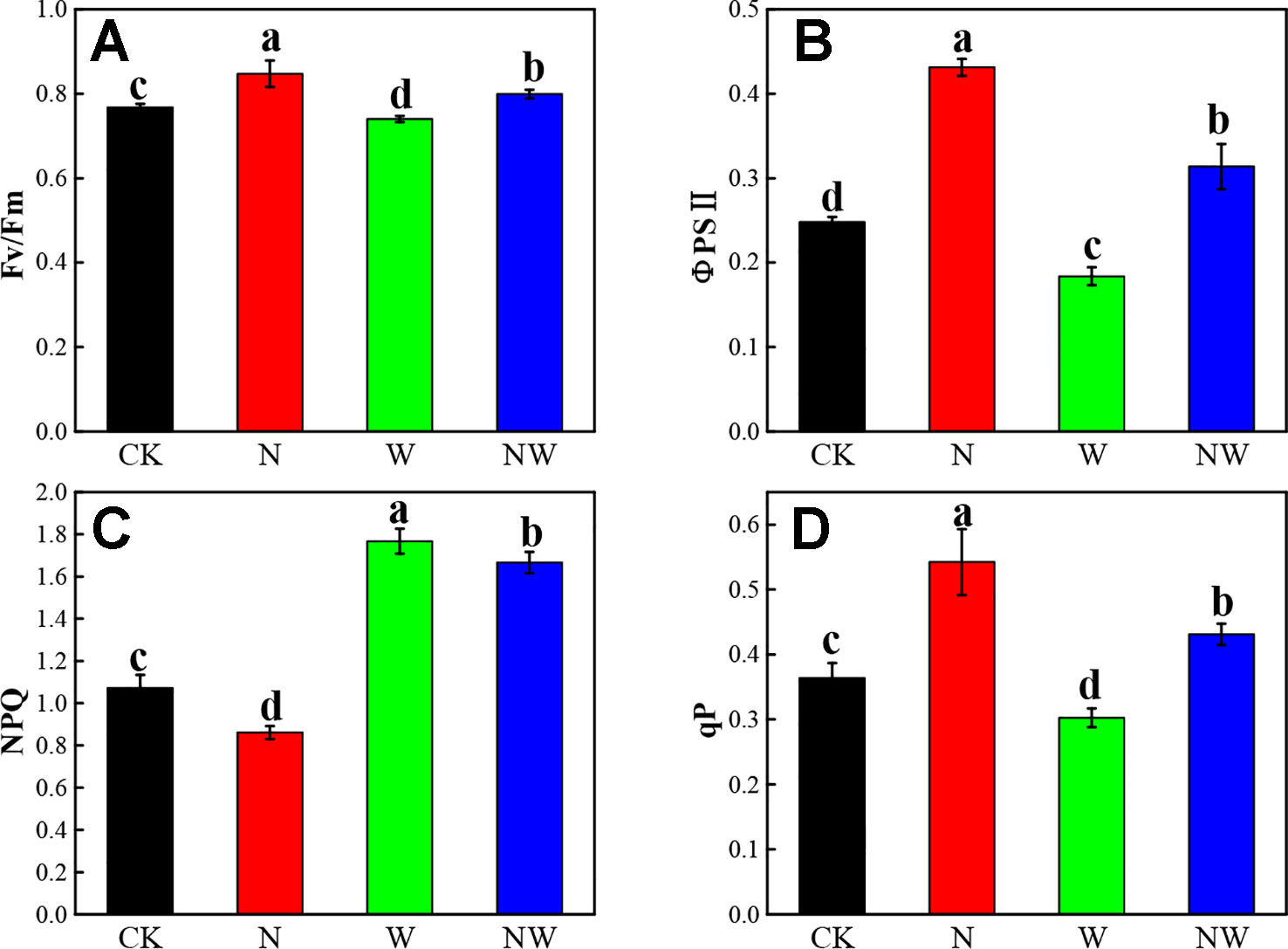
Figure 6 Effect of warming and N deposition on chlorophyll fluorescence of L. secalinus. Vertical bars represent ± SE of the mean, and different letters on the SE bars indicate significant differences among all treatments (P < 0.05). (A) Fv/Fm (B) ΦPSII (C) NPQ (D) qP. Each treatment has three replicated plots, and nine leaves from nine L. secalinus with similar growth status were chosen in each plot as a biological replication for analysis (3 biological replicates were performed).
Main Factors Affect Anet Under Warming and N Deposition
In the CK, gs, Chl, Chla, and Rubisco activity were positively correlated with Anet (r = 0.795, P < 0.05; r = 0.836, P < 0.01; r = 0.679, P < 0.05, and r = 0.805, P < 0.01, respectively) (Figure 7A). Under the N alone treatment, gs, Chl, Chla, Chlb, and Rubisco activity were positively correlated with Anet (r = 0.982, P < 0.01; r = 0.901, P < 0.01; r = 0.894, P < 0.01; r = 0.892, P < 0.01; and r = 0.877, P < 0.01, respectively) (Figure 7B). Under the warming treatment alone, only gs was positively correlated with Anet (r = 0.967, P < 0.01) (Figure 7C). Under the warming treatment plus N deposition, gs, Chla, and Rubisco activity were positively correlated with Anet (r = 0.964, P < 0.01; r = 0.710, P < 0.05; and r = 0.860, P < 0.01, respectively) (Figure 7D).
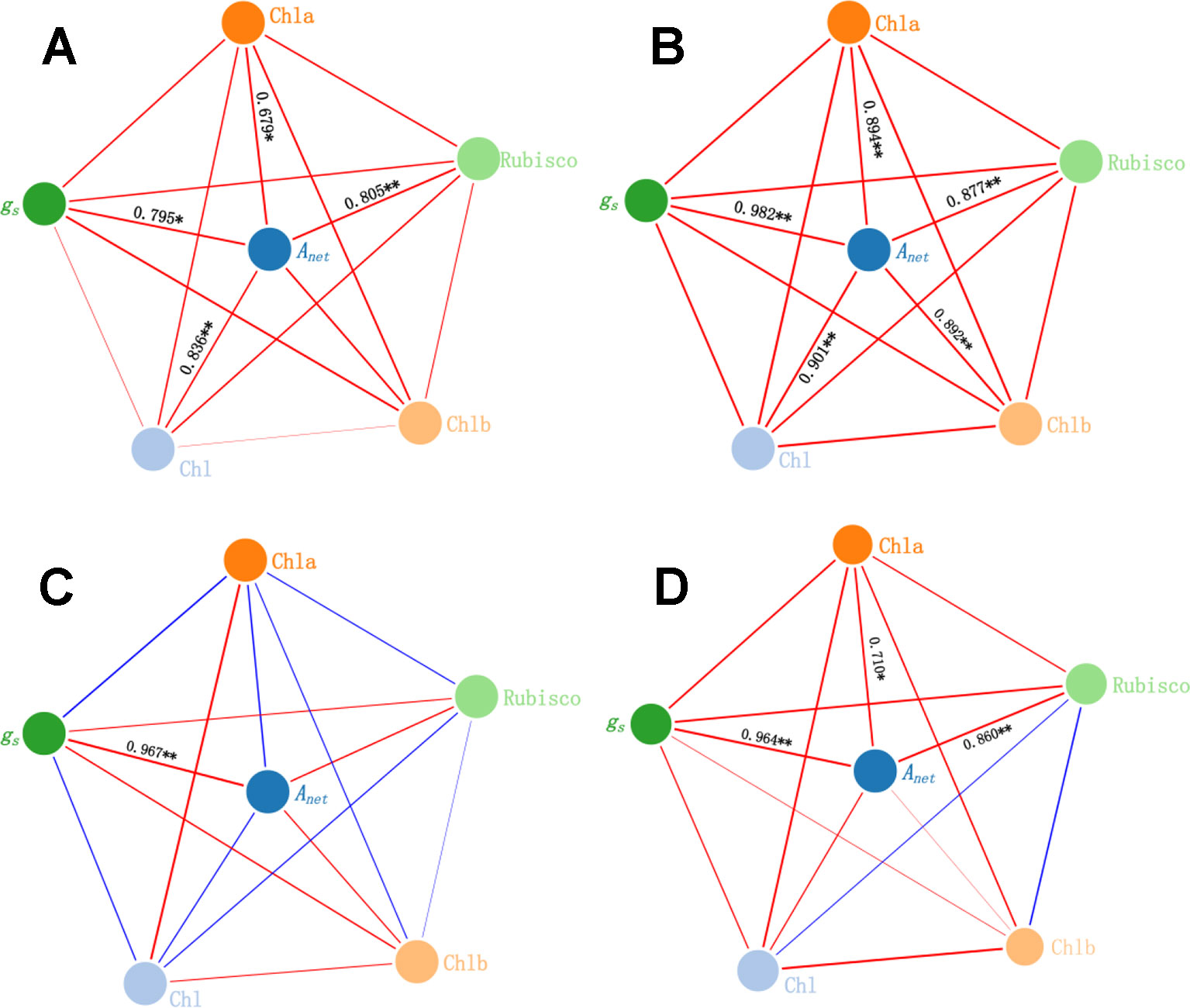
Figure 7 Relationship among gs, Chl, and Rubisco activity and Anet under warming and N deposition. Red lines represent positive relations, while blue lines represent negative relations. The boldness of the lines indicates the strength of the relations. Asterisk (*P < 0.05, **P < 0.01) represents significant difference. Four treatments: (A) CK, (B) N, (C) W, (D) NW.
Path Analysis Under Warming and N Deposition
A structural equation model (SEM) was successfully established. The paths under warming and N deposition were different (Figure 8).Warming caused the decrease of Anet directly through reducing gs and leaf Chl content (P < 0.05), while it had no significant influence on Rubisco activity (P > 0.05) (Figure 8A). N deposition significantly increased the gs, leaf Chl content, and Rubisco activity (P < 0.05), while it mainly increased the Anet through the enhancement of leaf Chl content and Rubisco activity (Figure 8B).
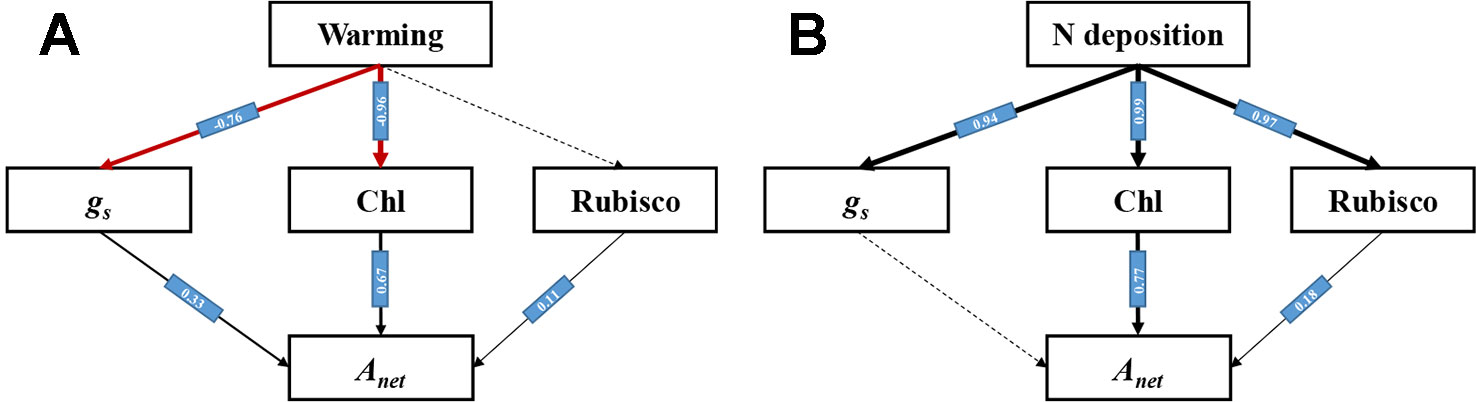
Figure 8 Path model (χ2 = 4.6, P > 0.05, CFI = 0.991) of warming and N deposition on net photosynthetic rate of L. secalinus. Black arrows with standardized coefficients show significant positive effects, while red arrows show significant negative effects (P < 0.05). Dash arrows show non-significant effects. (A) Path analysis under warming (B) Path analysis under N deposition.
Discussion
N Deposition Has Positive Effects on Photosynthetic Capacity While Warming Causes Negative Effects
Warming can alleviate low temperature stress in the alpine region to some degree (Lu et al., 2013) and lead to water stress by increasing evapotranspiration as well (Zhang et al., 2018b). Positive effects of warming on gas exchange of some plant species have been reported (Loik et al., 2004), while a few researchers argued that temperature increase in the warm seasons may also limit plant gas exchange due to overheating (Llorens et al., 2004). In this study, we found that the net photosynthetic rate of L. secalimus significantly decreased under warming treatment, indicating that warming can negatively limit plant photosynthesis. We found that the soil moisture also significantly decreased under warming, suggesting that warming-induced decrease in soil moisture might be the main cause of photosynthesis inhabitation (Loik et al., 2000). Warming can also reduce the diffusion of CO2 to leaf leading to decreased stomatal conductance caused by an increase in vapor pressure deficit that usually occurs as temperature rises (Farquhar and Sharkey, 1982). Additionally, the stomatal conductance was significantly lower and stomatal limitation value was much higher under warming in our study, suggesting that warming can undermine plant photosynthesis through alteration of plant stomatal traits, such as decrease of stomatal density (Ferris et al., 1996). It is well known that plant stomatal conductance is an important factor affecting carbon gain and water use efficiency (Zheng et al., 2013), and it has been widely used to quantify the efficiency of gas exchange (Franks et al., 2009). In our study, warming caused the decline of gs and Ci, indicating that stomatal limitation played a dominant role (Flexas and Medrano, 2002). However, under the warming plus N deposition treatment, the gs and Ci were increased simultaneously, suggesting the predominance of non-stomatal limitation to photosynthesis, and N deposition was the main factor that drive the improvement of photosynthesis in QTP.
Additionally, the effects of warming on plants are always regulated by N deposition. Previous researchers have found that N deposition could affect plant gas exchange (Li et al., 2003). In agreement with (Zhao and Liu, 2008), we found that N deposition significantly induced a positive effect on Anet of L. secalimus. Further, Anet was significantly increased under combination of warming and N deposition, yet it was significantly decreased under warming alone, indicating N deposition may compensate for the warming-induced impact on photosynthesis. Under N deposition treatment, Anet, gs, and Ci were significantly increased, suggesting that adequate supply of N can promote CO2 fixation.
The chlorophyll fluorescence reflects the basic function of the photosynthetic apparatus and the capacity and performance of photosynthesis (Zhao and Liu, 2008). Previous study showed that warming might promote plant photosynthesis through increasing apparent quantum yield (Awada et al., 2003). However, the results in our study showed that warming decreased the PSII efficiency of L. secalimus and induced photo-inhibition. Warming-induced soil drying could lead to a loss of excitation energy transfer and could impact the PSII efficiency (Shi et al., 2010), as PSII efficiency usually depends on temperature and water stress (Valentini et al., 1995). The Fv/Fm, ΦPSII, and qP values were significantly increased by N supply, suggesting that N was positively related to the enhanced efficiency of PSII center, as N deposition could significantly promote the synthesis of light-harvesting pigments, Chla and Chlb. The NPQ of L. secalimus significantly increased under warming, indicating that more energy participated in heat dissipation in order to alleviate oxidative stress (Shi et al., 2010). Additionally, our study on the Rubisco activity suggested that warming didn't cause enough or severe water stress to limit Rubisco activity. Rubisco wouldn't limit photosynthesis until severe or long-term water stress is encountered (Flexas et al., 2006).
Warming and N Deposition Alter Leaf N Content, and Thus Influence the Synthesis of Chlorophyll
Leaf N content is always related to plant eco-physiological process that can reflect adaptation mechanism of the plants to environmental factors (Sardans et al., 2012). Warming and N deposition could affect the leaf N dynamics of plants (Zhang et al., 2017). In general, leaf N content is always positively correlated with plant photosynthetic capacity because most of leaf N constitutes photosynthetic enzymes and chlorophyll (Fu et al., 2015). In our study, we observed that warming significantly reduced plant N accumulation, and this is similar to Sardans et al.'s (2008) findings. Leaf N content is generally closely related with photosynthetic carbon fixation (Sardans et al., 2008). Previous researchers reported that warming can promote CO2 fixation in high latitude ecosystems, as temperature there might be far from optimum (Keeling et al., 1996). However, warming might also limit photosynthetic capacity for decrease of soil moisture, inducing negative effects on CO2 assimilation (Xu et al., 2014). Our results suggested that warming can decrease plant leaf N level for biochemistry process. Leaf N content is always enhanced by N deposition for higher soil N availability (Hobbie et al., 2001). N deposition can significantly increase plant N concentration and decrease C/N ratio (Esmeijer-Liu et al., 2009). These findings are in agreement with our results. Additionally, we found that N deposition can regulate the warming-induced impact on leaf N content.
Chlorophyll is of significant importance in determining plant photosynthesis (Zhang et al., 2016).
Some scholars have found that warming could cause no obvious impact on plant chlorophyll concentrations (Ren et al., 2010). In this study, we found that warming significantly decreased the chlorophyll content of L. secalimus. This is in agreement with Shen et al.'s (2013) findings. Nitrogen deposition induced a significant increase in the chlorophyll contents of L. secalimus. This is consistent with (Zhou et al., 2011) results. The possible reason might be that N deposition can further increase soil N availability and promote plant N uptake, leading to enhanced photosynthetic pigment synthesis (Zhang et al., 2013). Additionally, increase of chlorophyll suggested that N deposition could also promote photosynthesis (Zhang et al., 2016). The combined effects of warming and N deposition also significantly promoted the synthesis of photosynthetic pigments, while the impacts were not as stronger as warming alone. These results suggested that combined effects of warming and N deposition on plant photosynthetic pigments are still positive, though warming may limit the process of photosynthetic pigments synthesis.
Warming and N Deposition Have Different Effects on the Antioxidant System and Photosynthetic Enzyme
Antioxidant enzyme activity is an important defense mechanism that influences stress tolerance in plants (Naudts et al., 2014). It is well known that reactive oxygen species could cause lipid peroxidation and impact cell membrane (Foyer et al., 1994). Membrane lipid peroxidation can reflect stress induced accumulation of reactive oxygen species (ROS) and produce malondialdehyde (MDA), which is widely used as an indicator of membrane injury (Zhou et al., 2018). We found in this study that warming significantly increased the MDA contents of L. secalimus, while N deposition alone decreased the MDA contents, indicating that warming can cause plasma membrane damage in L. secalimus. N deposition may enhance plant ROS scavenging capacity, and thus alleviate exterior stress on plant plasma membrane damage. Plants have developed a protective enzyme system as defense against oxygen toxicity under stress, such as SOD, POD, and CAT (Zhao and Liu, 2009). Our results suggested that warming led to a much higher antioxidant activity in L. secalimus, indicating that warming under stress can significantly motivate the antioxidant enzyme system. Nitrogen could promote synthesis of physiological activities of antioxidant enzymes (Zhao and Liu, 2009). Under warming plus N deposition, the activity of antioxidant enzymes was much lower than that under warming alone; this might be attributed to the fact that N deposition could alleviate the negative effects induced by warming to some degree.
Main Path Determining the Change of Anet Under Warming and N Deposition
Warming may cause water deficit and reduced stomatal conductance, which will result in lower Ci, and consequently lead to a decrease in CO2 concentration at the carboxylation site of ribulose-1,5-bisphosphate (RuBP) carboxylase/oxygenase (Rubisco), thereby decreasing net photosynthesis (Chastain et al., 2014). gs was usually used as an indicator to evaluate stomatal and non-stomatal limitations to photosynthesis under water deficit (Brodribb, 1996). In our study, warming directly restrained Anet by reducing the gs and Chl, suggesting that both stomatal limitation and non-stomatal limitation play an important role in determining the decrease of photosynthetic capacity under warming, and the downregulation of photosynthesis can result from lower CO2 availability caused by stomatal closure and the lower synthesis of Chl. In addition, though N deposition significantly increased gs, Chl content, and Rubisco activity, it directly increased Anet by increasing the Chl content and Rubisco activity, indicating that non-stomatal limitation plays a vital role in determining the increase of photosynthetic capacity under N deposition.
N deposition could regulate plant responses to warming (Zong et al., 2018b), and change in temperature is also likely to interact with the effects of N deposition (Suddick et al., 2013). Up to now, little is known about the physiological responses of plants under warming and N deposition.
Conclusion
Our study showed that climate warming can cause impact on photosynthesis of L. secalimus, while N deposition could induce positive effects. Warming reduced plant photosynthetic capacity mainly through both stomatal limitation and non-stomatal limitation. N deposition enhanced plant photosynthetic capacity mainly through non-stomatal limitation. Additionally, both warming and N deposition enhanced plant resistance by improving antioxidant enzyme systems. N deposition is beneficial for it can significantly increase stomatal conductance, chlorophyll content, and Rubisco activity, which are positively correlated with photosynthesis. Overall, warming could impair the photosynthetic potential of L. secalinus enhanced by N deposition in alpine grassland of Qinghai- Tibetan plateau. Future researches are needed to explore the causes of the different responses of L. secalimus, the dominant plant species of alpine grasslands, to the combination of climate warming and N deposition.
Data Availability Statement
The datasets generated for this study are available on request to the corresponding author.
Author Contributions
SD, HS planned and designed the research. SD, WW, SLi, JX, MY, JZ, XG, YX and YZ helped to perform the experiments. SD, WW, SLiu, QD and HZ helped to give some useful suggestions in writing. SD, WW, HS, JY revised this manuscript. HS analyzed the data and wrote this paper.
Funding
This research was financially supported by the grants from the National Key R & D Program of China (2016YFC0501906), Qinghai Provincial Key R & D program in Qinghai Province (2019-SF-145 & 2018-NK-A2), and State Key Joint Laboratory of Environmental Simulation and Pollution Control (Beijing Normal University) (17L03ESP). The authors would also thank the anonymous reviewers for their helpful comments; the endeavor of editors and reviewers was also appreciated.
Conflict of Interest
The authors declare that the research was conducted in the absence of any commercial or financial relationships that could be construed as a potential conflict of interest.
Acknowledgments
The authors wish to express the gratitude to the reviewers and editors for their time and effort. The endeavor of editors and reviewers was also appreciated.
Abbreviations
Anet, net photosynthetic rate; gs, stomatal conductance; Ci, intercellular CO2 concentration; Tr, transpiration rate; VPD, Vapor pressure deficit; Ls, stomatal limitation; Chla, Chlorophyll a; Chlb, Chlorophyll b; Chl, total Chlorophyll; MDA, malondialdehyde; SOD, superoxide dismutase; POD, peroxidase; CAT, catalase; Rubisco, ribulose-1,5-bisphosphate carboxylase/oxygenase; Fv/Fm, maximal quantum yield of PSII; ΦPSII, effective quantum yield of PSII; qP, photochemical quenching; NPQ, non-photochemical quenching; FW, fresh weight.
References
Allison, S. D., Romero-Olivares, A. L., Lu, Y., Taylor, J. W., Treseder, K. K. (2018). Temperature sensitivities of extracellular enzyme Vmax and Km across thermal environments. Global Change Biol. 24 (7), 2884–2897. doi: 10.1111/gcb.14045
Atkin, O. K., Scheurwater, I., Pons, T. L. (2006). High thermal acclimation potential of both photosynthesis and respiration in two lowland Plantago species in contrast to an alpine congeneric. Global Change Biol. 12 (3), 500–515. doi: 10.1111/j.1365-2486.2006.01114.x
Awada, T., Radoglou, K., Fotelli, M. N., Constantinidou, H. I. (2003). Ecophysiology of seedlings of three Mediterranean pine species in contrasting light regimes. Tree Physiol. 23 (1), 33–41. doi: 10.1093/treephys/23.1.33
Barker, D. H., Adams, W. W., III (1997). The xanthophyll cycle and energy dissipation in differently oriented faces of the cactus Opuntia macrorhiza. Oecologia 109 (3), 353–361. doi: 10.1007/s004420050093
Brodribb, T. (1996). Dynamics of changing intercellular CO2 concentration (ci) during drought and determination of minimum functional ci. Plant Physiol. 111 (1), 179–185. doi: 10.1104/pp.111.1.179
Chastain, D. R., Snider, J. L., Collins, G. D., Perry, C. D., Whitaker, J., Byrd, S. A. (2014). Water deficit in field-grown Gossypium hirsutum primarily limits net photosynthesis by decreasing stomatal conductance, increasing photorespiration, and increasing the ratio of dark respiration to gross photosynthesis. J. Plant Physiol. 171 (17), 1576–1585. doi: 10.1016/j.jplph.2014.07.014
Chen, B., Zhang, X., Tao, J., Wu, J., Wang, J., Shi, P., et al. (2014). The impact of climate change and anthropogenic activities on alpine grassland over the Qinghai-Tibet Plateau. Agr. For. Meteorol. 189, 11–18. doi: 10.1016/j.agrformet.2014.01.002
Cheng, L., Fuchigami, L. H. (2000). Rubisco activation state decreases with increasing nitrogen content in apple leaves. J. Exp. Bot. 51 (351), 1687–1694. doi: 10.1093/jexbot/51.351.1687
Demmig-Adams, B., Adams, W. W., III (1996). The role of xanthophyll cycle carotenoids in the protection of photosynthesis. Trends Plant Sci. 1 (1), 21–26. doi: 10.1016/S1360-1385(96)80019-7
Dong, S., Sherman, R. (2015). Enhancing the resilience of coupled human and natural systems of alpine rangelands on the Qinghai-Tibetan plateau. Rangeland J. 37 (1), i–iii. doi: 10.1071/RJ14117
Esmeijer-Liu, A. J., Aerts, R., Kürschner, W. M., Bobbink, R., Lotter, A. F., Verhoeven, J. T. A. (2009). Nitrogen enrichment lowers Betula pendula green and yellow leaf stoichiometry irrespective of effects of elevated carbon dioxide. Plant Soil 316 (1-2), 311–322. doi: 10.1007/s11104-008-9783-1
Farquhar, G. D., Sharkey, T. D. (1982). Stomatal conductance and photosynthesis. Annu. Rev. Plant Physiol. 33 (1), 317–345. doi: 10.1146/annurev.pp.33.060182.001533
Ferris, R., Nijs, I., Behaeghe, T., Impens, I. (1996). Elevated CO2 and temperature have different effects on leaf anatomy of perennial ryegrass in spring and summer. Ann. Bot. 78 (4), 489–497. doi: 10.1006/anbo.1996.0146
Fleck, I., Hogan, K. P., Llorens, L., Abadía, A., Aranda, X. (1998). Photosynthesis and photoprotection in Quercus ilex resprouts after fire. Tree Physiol. 18 (8-9), 607–614. doi: 10.1093/treephys/18.8-9.607
Flexas, J., Medrano, H. (2002). Drought-inhibition of photosynthesis in C3 plants: stomatal and non-stomatal limitations revisited. Ann. Bot. 89 (2), 183–189. doi: 10.1093/aob/mcf027
Flexas, J., Bota, J., Galmes, J., Medrano, H., Ribas Carbó, M. (2006). Keeping a positive carbon balance under adverse conditions: responses of photosynthesis and respiration to water stress. Physiol. Plantarum 127 (3), 343–352. doi: 10.1111/j.1399-3054.2006.00621.x
Foyer, C. H., Lelandais, M., Kunert, K. J. (1994). Photooxidative stress in plants. Physiol. Plantarum 92 (4), 696–717. doi: 10.1111/j.1399-3054.1994.tb03042.x
Franks, P. J., Drake, P. L., Beerling, D. J. (2009). Plasticity in maximum stomatal conductance constrained by negative correlation between stomatal size and density: an analysis using Eucalyptus globulus. Plant Cell Environ. 32 (12), 1737–1748. doi: 10.1111/j.1365-3040.2009.002031.x
Fu, G., Shen, Z. X., Sun, W., Zhong, Z. M., Zhang, X. Z., Zhou, Y. T. (2015). A meta-analysis of the effects of experimental warming on plant physiology and growth on the Tibetan Plateau. J. Plant Growth Regul. 34 (1), 57–65. doi: 10.1007/s00344-014-9442-0
Ganjurjav, H., Hu, G., Wan, Y., Li, Y., Danjiu, L., Gao, Q. (2018). Different responses of ecosystem carbon exchange to warming in three types of alpine grassland on the central Qinghai–Tibetan Plateau. Ecol. Evol. 8 (3), 1507–1520. doi: 10.1002/ece3.3741
Genty, B., Briantais, J. M., Baker, N. R. (1989). The relationship between the quantum yield of photosynthetic electron transport and quenching of chlorophyll fluorescence. Biochimica et Biophysica Acta (BBA)-General Subjects 990(1), 87–92. doi: 10.1016/S0304-4165(89)80016-9
Giannopolitis, C. N., Ries, S. K. (1977). Superoxide dismutases: I. Occurrence in higher plants. Plant Physiol. 59 (2), 309–314. doi: 10.1104/pp.59.2.309
Harte, J., Shaw, R. (1995). Shifting dominance within a montane vegetation community: results of a climate-warming experiment. Science 267 (5199), 876–880. doi: 10.1126/science.267.5199.876
He, W. M., Dong, M. (2003). Plasticity in physiology and growth of Salix matsudana in response to simulated atmospheric temperature rise in the Mu Us Sandland. Photosynthetica 41 (2), 297–300. doi: 10.1023/B:PHOT.0000011966.30235.91
Heath, R. L., Packer, L. (1965). Effect of light on lipid peroxidation in chloroplasts. Biochem. Bioph. Res. Co. 19 (6), 716–720. doi: 10.1016/0006-291X(65)90316-5
Hobbie, E. A., Olszyk, D. M., Rygiewicz, P. T., Tingey, D. T., Johnson, M. G. (2001). Foliar nitrogen concentrations and natural abundance of 15 N suggest nitrogen allocation patterns of Douglas-fir and mycorrhizal fungi during development in elevated carbon dioxide concentration and temperature. Tree Physiol. 21, 1113–1122. doi: 10.1093/treephys/21.15.1113
Hu, L., Wang, Z., Huang, B. (2010). Diffusion limitations and metabolic factors associated with inhibition and recovery of photosynthesis from drought stress in a C3 perennial grass species. Physiol. Plantarum 139 (1), 93–106. doi: 10.1111/j.1399-3054.2010.01350.x
Hughes, T. P., Kerry, J. T., Baird, A. H., Connolly, S. R., Dietzel, A., Eakin, C. M., et al. (2018). Global warming transforms coral reef assemblages. Nature 556 (7702), 492. doi: 10.1038/s41586-018-0041-2
IPCC (2013). Climate change 2013: the physical science basis. Working Group I: Contribution to the Intergovernmental Panel on Climate Change Fifth Assessment Report (Cambridge: Cambridge University Press).
Keeling, C. D., Chin, J. F. S., Whorf, T. P. (1996). Increased activity of northern vegetation inferred from atmospheric CO2 measurements. Nature 382 (6587), 146. doi: 10.1038/382146a0
Kochhar, S., Kochhar, V. K., Khanduja, S. D. (1979). Changes in the pattern of isoperoxidases during maturation of grape berries cv Gulabi as affected by ethephon (2-chloroethyl) phosphonic acid. Am. J. Enol. Viticult. 30 (4), 275–277.
Li, D., Mo, J., Fang, Y., Peng, S., Per, G. (2003). Impact of nitrogen deposition on forest plants. Acta Ecol. Sin. 23 (9), 1891–1900.
Lichtenthaler, H. K. (1987). Chlorophylls and carotenoids: pigments of photosynthetic biomembranes. Meth. Enzymol. 148 350–382. doi: 10.1016/0076-6879(87)48036-1
Llorens, L., Penuelas, J., Estiarte, M., Bruna, P. (2004). Contrasting growth changes in two dominant species of a Mediterranean shrubland submitted to experimental drought and warming. Ann. Bot. 94 (6), 843–853. doi: 10.1093/aob/mch211
Loik, M. E., Redar, S. P., Harte, J. (2000). Photosynthetic responses to a climate-warming manipulation for contrasting meadow species in the Rocky Mountains, Colorado, USA. Funct. Ecol. 14 (2), 166–175. doi: 10.1046/j.1365-2435.2000.00411.x
Loik, M. E., Still, C. J., Huxman, T. E., Harte, J. (2004). In situ photosynthetic freezing tolerance for plants exposed to a global warming manipulation in the Rocky Mountains, Colorado, USA. New Phytol. 162 (2), 331–341. doi: 10.1111/j.1469-8137.2004.01002.x
Lü, C., Tian, H. (2007). Spatial and temporal patterns of nitrogen deposition in China: synthesis of observational data. J. Geophys. Res-Atmos. 112 (D22S05). doi: 10.1029/2006JD007990
Lu, M., Zhou, X., Yang, Q., Li, H., Luo, Y., Fang, C., et al. (2013). Responses of ecosystem carbon cycle to experimental warming: a meta-analysis. Ecology 94 (3), 726–738. doi: 10.1890/12-0279.1
Marañón-Jiménez, S., Peñuelas, J., Richter, A., Sigurdsson, B. D., Fuchslueger, L., Leblans, N. I., et al. (2019). Coupled carbon and nitrogen losses in response to seven years of chronic warming in subarctic soils. Soil Biol. Biochem. 134, 152–161. doi: 10.1016/j.soilbio.2019.03.028
Mou, X. M., Li, X. G., Zhao, N., Yu, Y. W., Kuzyakov, Y. (2018). Tibetan sedges sequester more carbon belowground than grasses: a 13 C labeling study. Plant Soil 426, 287–298. doi: 10.1007/s11104-018-3634-5
Nakaji, T., Fukami, M., Dokiya, Y., Izuta, T. (2001). Effects of high nitrogen load on growth, photosynthesis and nutrient status of Cryptomeria japonica and Pinus densiflora seedlings. Trees 15 (8), 453–461. doi: 10.1007/s00468-001-0130-x
Naudts, K., Van den Berge, J., Farfan, E., Rose, P., AbdElgawad, H., Ceulemans, R., et al. (2014). Future climate alleviates stress impact on grassland productivity through altered antioxidant capacity. Environ. Exp. Bot. 99, 150–158. doi: 10.1016/j.envexpbot.2013.11.003
Niu, S., Li, Z., Xia, J., Han, Y., Wu, M., Wan, S. (2008). Climatic warming changes plant photosynthesis and its temperature dependence in a temperate steppe of northern China. Environ. Exp. Bot. 63 (1-3), 91–101. doi: 10.1016/j.envexpbot.2007.10.016
Oxborough, K., Baker, N. R. (1997). An instrument capable of imaging chlorophyll a fluorescence from intact leaves at very low irradiance and at cellular and subcellular levels of organization. Plant Cell Environ. 20 (12), 1473–1483. doi: 10.1046/j.1365-3040.1997.d01-42.x
Qin, Y., Yi, S., Ren, S., Li, N., Chen, J. (2014). Responses of typical grasslands in a semi-arid basin on the Qinghai-Tibetan Plateau to climate change and disturbances. Environ. Earth Sci. 71 (3), 1421–1431. doi: 10.1007/s12665-013-2547-0
Ren, F., Zhou, H. K., Zhao, X. Q., Han, F., Shi, L. N., Duan, J. C., et al. (2010). Influence of simulated warming using OTC on physiological–biochemical characteristics of Elymus nutans in alpine meadow on Qinghai-Tibetan plateau. Acta Ecol. Sin. 30 (3), 166–171. doi: 10.1016/j.chnaes.2010.04.007
Samaniego, L., Thober, S., Kumar, R., Wanders, N., Rakovec, O., Pan, M., et al. (2018). Anthropogenic warming exacerbates European soil moisture droughts. Nat. Clim. Change 8 (5), 421. doi: 10.1038/s41558-018-0138-5
Sardans, J., PeNUuelas, J., Estiarte, M., Prieto, P. (2008). Warming and drought alter C and N concentration, allocation and accumulation in a Mediterranean shrubland. Global Change Biol. 14 (10), 2304–2316. doi: 10.1111/j.1365-2486.2008.01656.x
Sardans, J., Rivas-Ubach, A., Penuelas, J. (2012). The elemental stoichiometry of aquatic and terrestrial ecosystems and its relationships with organismic lifestyle and ecosystem structure and function: a review and perspectives. Biogeochemistry 111 (1-3), 1–39. doi: 10.1007/s10533-011-9640-9
Shaw, M. R., Loik, M. E., Harte, J. (2000). Gas exchange and water relations of two Rocky Mountain shrub species exposed to a climate change manipulation. Plant Ecol. 146 (2), 195–204. doi: 10.1023/A:1009863201751
Shen, H., Wang, S., Tang, Y. (2013). Grazing alters warming effects on leaf photosynthesis and respiration in Gentiana straminea, an alpine forb species. J. Plant Ecol. 6 (5), 418–427. doi: 10.1093/jpe/rtt010
Shi, F. S., Wu, Y., Wu, N., Luo, P. (2010). Different growth and physiological responses to experimental warming of two dominant plant species Elymus nutans and Potentilla anserina in an alpine meadow of the eastern Tibetan Plateau. Photosynthetica 48 (3), 437–445. doi: 10.1007/s11099-010-0058-8
Suddick, E. C., Whitney, P., Townsend, A. R., Davidson, E. A. (2013). The role of nitrogen in climate change and the impacts of nitrogen–climate interactions in the United States: foreword to thematic issue. Biogeochemistry 114 (1-3), 1–10. doi: 10.1007/s10533-012-9795-z
Trevor, E., Kraus, R., Austin, F. (1994). Paclobutrazol protects wheat seedlings from heat and paraquat injury is detoxification of active oxygen involved. Plant Cell Physiol. 35, 45–52. doi: 10.1093/oxfordjournals.pcp.a078569
Valentini, R., Epron, D., De Angelis, P., Matteucci, G., Dreyer, E. (1995). In situ estimation of net CO2 assimilation, photosynthetic electron flow and photorespiration in Turkey oak (Q. cerris L.) leaves: diurnal cycles under different levels of water supply. Plant Cell Environ. 18 (6), 631–640. doi: 10.1111/j.1365-3040.1995.tb00564.x
Valladares, F., Pearcy, R. W. (1997). Interactions between water stress, sun-shade acclimation, heat tolerance and photoinhibition in the sclerophyll Heteromeles arbutifolia. Plant Cell Environ. 20 (1), 25–36. doi: 10.1046/j.1365-3040.1997.d01-8.x
Wang, M., Zhang, W. W., Li, N., Liu, Y. Y., Zheng, X. B., Hao, G. Y. (2018). Photosynthesis and growth responses of Fraxinus mandshurica Rupr. seedlings to a gradient of simulated nitrogen deposition. Ann. For. Sci. 75 (1), 1. doi: 10.1007/s13595-017-0678-2
Xu, Z., Shimizu, H., Ito, S., Yagasaki, Y., Zou, C., Zhou, G., Zheng, Y. (2014). Effects of elevated CO 2, warming and precipitation change on plant growth, photosynthesis and peroxidation in dominant species from North China grassland. Planta 239(1–2), 421–435. doi: 10.1007/s00425-013-1987-9
Yin, C. Y., Berninger, F., Li, C. Y. (2006). Photosynthetic responses of Populus przewalski subjected to drought stress. Photosynthetica 44 (1), 62–68. doi: 10.1007/s11099-005-0159-y
Yu, L., Song, M., Xia, Z., Korpelainen, H., Niinemets, Ü., Li, C. (2019). Elevated temperature differently affects growth, photosynthetic capacity, nutrient absorption and leaf ultrastructure of Abies faxoniana and Picea purpurea under intra-and interspecific competition. Tree Physiol 39, 1342–1357. doi: 10.1093/treephys/tpz044
Zhang, L. R., Niu, H. S., Wang, S. P., Li, Y. N., Zhao, X. Q. (2010). Effects of temperature increase and grazing on stomatal density and length of four alpine Kobresia meadow species, Qinghai-Tibetan Plateau. Acta Ecol. Sin. 30, 6961–6969.
Zhang, N., Guo, R., Song, P., Guo, J., Gao, Y. (2013). Effects of warming and nitrogen deposition on the coupling mechanism between soil nitrogen and phosphorus in Songnen Meadow Steppe, northeastern China. Soil Biol. Biochem. 65, 96–104. doi: 10.1016/j.soilbio.2013.05.015
Zhang, T., Yang, S., Guo, R., Guo, J. (2016). Warming and nitrogen addition alter photosynthetic pigments, sugars and nutrients in a temperate meadow ecosystem. PloS One 11 (5), e0155375. doi: 10.1371/journal.pone.0155375
Zhang, Q., Xie, J., Lyu, M., Xiong, D., Wang, J., Chen, Y., Yang, Y. (2017). Short-term effects of soil warming and nitrogen addition on the N: P stoichiometry of Cunninghamia lanceolata in subtropical regions. Plant Soil 411(1–2), 395–407. doi: 10.1007/s11104-016-3037-4
Zhang, N. N., Sun, G., Liang, J., Wang, E. T., Shi, C. G., He, J., et al. (2018a). Response of ammonium oxidizers to the application of nitrogen fertilizer in an alpine meadow on the Qinghai-Tibetan Plateau. Appl. Soil Ecol. 124, 266–274. doi: 10.1016/j.apsoil.2017.11.018
Zhang, Q., Yang, Z., Hao, X., Yue, P. (2018b). Conversion features of evapotranspiration responding to climate warming in transitional climate regions in northern China. Clim. Dynam., 1–13. doi: 10.1007/s00382-018-4364-3
Zhao, C., Liu, Q. (2008). Growth and photosynthetic responses of two coniferous species to experimental warming and nitrogen fertilization. Can. J. For. Res. 39 (1), 1–11. doi: 10.1139/X08-152
Zhao, C., Liu, Q. (2009). Growth and physiological responses of Picea asperata seedlings to elevated temperature and to nitrogen fertilization. Acta Physiol. Plant 31 (1), 163. doi: 10.1007/s11738-008-0217-8
Zhao, Z., Dong, S., Jiang, X., Liu, S., Ji, H., Li, Y., et al. (2017). Effects of warming and nitrogen deposition on CH4, CO2 and N2O emissions in alpine grassland ecosystems of the Qinghai-Tibetan Plateau. Sci. Total Environ. 592, 565–572. doi: 10.1016/j.scitotenv.2017.03.082
Zheng, Y., Xu, M., Hou, R., Shen, R., Qiu, S., Ouyang, Z. (2013). Effects of experimental warming on stomatal traits in leaves of maize (Z ea may L.). Ecol. Evol. 3 (9), 3095–3111. doi: 10.1002/ece3.674
Zhou, X., Zhang, Y., Ji, X., Downing, A., Serpe, M. (2011). Combined effects of nitrogen deposition and water stress on growth and physiological responses of two annual desert plants in northwestern China. Environ. Exp. Bot. 74, 1–8. doi: 10.1016/j.envexpbot.2010.12.005
Zhou, Y., Yin, X., Wan, S., Hu, Y., Xie, Q., Li, R., et al. (2018). The Sesuvium portulacastrum plasma membrane Na+/H+ antiporter SpSOS1 complemented the salt sensitivity of transgenic Arabidopsis sos1 mutant plants. Plant Mol. Biol. Rep. 36 (4), 553–563. doi: 10.1007/s11105-018-1099-6
Zong, N., Geng, S., Duan, C., Shi, P., Chai, X., Zhang, X. (2018a). The effects of warming and nitrogen addition on ecosystem respiration in a Tibetan alpine meadow: The significance of winter warming. Ecol. Evol. 8 (20), 10113–10125. doi: 10.1002/ece3.4484
Keywords: Qinghai-Tibetan plateau, stomatal conductance, antioxidant enzymes, photosynthesis, Leymus secalinus
Citation: Shen H, Dong S, Li S, Wang W, Xiao J, Yang M, Zhang J, Gao X, Xu Y, Zhi Y, Liu S, Dong Q, Zhou H and Yeomans JC (2020) Effects of Warming and N Deposition on the Physiological Performances of Leymus secalinus in Alpine Meadow of Qinghai-Tibetan Plateau. Front. Plant Sci. 10:1804. doi: 10.3389/fpls.2019.01804
Received: 18 September 2019; Accepted: 24 December 2019;
Published: 21 February 2020.
Edited by:
Boris Rewald, University of Natural Resources and Life Sciences Vienna, AustriaCopyright © 2020 Shen, Dong, Li, Wang, Xiao, Yang, Zhang, Gao, Xu, Zhi, Liu, Dong, Zhou and Yeomans. This is an open-access article distributed under the terms of the Creative Commons Attribution License (CC BY). The use, distribution or reproduction in other forums is permitted, provided the original author(s) and the copyright owner(s) are credited and that the original publication in this journal is cited, in accordance with accepted academic practice. No use, distribution or reproduction is permitted which does not comply with these terms.
*Correspondence: Shikui Dong, ZHNrMDMwMzdAYm51LmVkdS5jbg==