- National Key Laboratory of Crop Genetic Improvement, Huazhong Agricultural University, Wuhan, China
DNA binding with one finger (Dof) proteins are plant-specific transcription factors with important and diverse functions in seed germination, flowering time, and biotic and abiotic stresses. In this study, haplotype-based association analysis was conducted between heading date and 30 Dof family genes in a worldwide germplasm collection. Of these, 22 Dof genes were associated with heading date. Multiple comparisons among haplotypes revealed their diverse functions in promoting and suppressing heading date under short-day (SD) and long-day (LD) conditions. They cumulatively made a considerable contribution to the missing heritability of heading date. A set of knockout mutants of 30 Dof genes generated by CRISPR/Cas9-mediated genome editing technology showed that 11 and 9 Dof genes regulated heading date under LD and SD, respectively. Phenotype measurement of mutants showed that these 11 and 9 Dof genes slightly regulated heading with effects of 2–5 days under LD and SD, respectively. Both mutant and natural variation assays indicated functional redundancy in regulating heading date among Dof family genes. Nucleotide diversity analysis suggested that most Dof genes have been subjected to selection during domestication and improvement. Beyond heading date, this set of mutants is also a good resource for evaluating the function of Dof genes in regulating stress tolerance and seed germination.
Introduction
Functional characterization of gene families in crops is important. Genes in the same family frequently have conserved functions (Jain et al., 2006; Li et al., 2016; Dong et al., 2018). However, in some cases, genes within a family have very distinct functions (Péret et al., 2012; Park et al., 2017; Zhang et al., 2017). For example, the AUXIN (AUX)/LIKE AUX (LAX) genes in Arabidopsis encode a family of auxin influx transporters comprising four highly conserved genes, AUX1, LAX1, LAX2, and LAX3. AUX1, LAX1, and LAX3 regulate distinct auxin-dependent developmental processes, but LAX2 regulates vascular patterning in cotyledons (Péret et al., 2012).
Functional characterization of gene families is often made by overexpression, knockout (gene editing), or knockdown (RNA interference). Overexpression sometimes causes ectopic expression and does not necessarily reflect the native function of target genes. Knocking out and knocking down genes are better ways to identify gene function because these methods precisely target the gene in situ, which does not cause genetic noise. However, the effect of manipulating single genes is reduced if functional redundancy exists within the gene family. Currently, the clustered regularly interspaced short palindromic repeats (CRISPR) system is a powerful tool to produce mutants in target genes (Cong et al., 2013; Hwang et al., 2013; Shan et al., 2013; Yin et al., 2017; Zhou et al., 2018). CRISPR technology relies on Cas9 and single guide RNA (sgRNA) to achieve precise cutting. CRISPR/Cas9 systems have been applied to multiplexed genome editing (Ma et al., 2015; Zhang et al., 2016), which will facilitate functional analysis of gene families. Transgene-free plants can be achieved through backcrossing and used directly in breeding. For example, the non-transgenic, low-gluten wheat lines were obtained through modification of the α-gliadin family genes using CRISPR/Cas9 technology and serve as source material to introgress this trait into elite wheat varieties (Sanchez-Leon et al., 2018).
Next-generation sequencing technology has developed rapidly and has greatly facilitated genome-wide association studies (GWAS) (Huang et al., 2010; Huang et al., 2017), which have been widely used to identify candidate genes associated with various complex traits in several species including humans, animals, and plants (Wellcome Trust Case Control C, 2007; Moreira et al., 2018; Su et al., 2018). Candidate gene-based association analysis is an option to identify potential functions of related genes. Moreover, association mapping at the haplotype level is a very effective method to establish the relationships between genes and traits (Han et al., 2016; Chen et al., 2018; Lu et al., 2018). Haplotype development is easy at the single gene level, which is helpful to identify the association between related genes and traits at the haplotype level.
DNA binding with one finger (Dof) proteins are plant-specific transcription factors (Yamagishi et al., 2002; Yamaguchi et al., 2004). Since the first Dof gene, MNB1, was identified in maize (Yanagisawa and Izui, 1993), an increasing number of Dof genes have been identified in various plant species. Recent studies have revealed the role of the Dof family genes in multiple plant developmental processes. In tomato, TDDF1 (TOMATO DOF DAILY FLUCTUATIONS 1) is involved in circadian regulation and stress resistance (Ewas et al., 2017). In maize, ZmDof3 regulates starch accumulation and aleurone development in maize endosperm (Qi et al., 2017). In rice, a total of 30 Dof genes have been predicted through genome analysis (Lijavetzky et al., 2003). All have a highly conserved DNA-binding domain (Dof domain) of 50 amino acid residues including a C2C2-type zinc finger motif (Huang et al., 2018). RDD1/OsDof2 overexpression promotes flowering in addition to regulating nutrient ion uptake and accumulation (Iwamoto et al., 2009; Iwamoto and Tagiri, 2016). OsDof4 has distinct flowering effects under long-day and short-day conditions (Wu et al., 2017). OsDof12 participates in the regulation of flowering time and plant architecture (Li et al., 2009; Wu et al., 2015). These studies indicate that Dof family genes in rice might have important functions in flowering. Therefore, we hypothesized that some other Dof family genes are probably related to flowering. To test this hypothesis, association analysis between heading date and haplotypes of Dof family genes were conducted in this study. On the one hand, candidate gene-based association analysis of Dof family genes with heading date was examined at the haplotype level in a worldwide germplasm collection of 529 rice accessions. On the other hand, the CRISPR/Cas9 gene editing technology was utilized to test which Dof family genes really function in the regulation of heading date. Our results showed that 11 and 9 Dof genes regulated heading date under long day (LD) and short day (SD), respectively. Nucleotide diversity analysis showed that most Dof genes regulating heading date have been subjected to selection during domestication and genetic improvement.
Materials and Methods
Plant Materials and Field Experiments
Zhonghua 11 (ZH11; Oryza sativa japonica) was used as the recipient for transformation. A total of 529 rice accessions from around the world, composed of a core Chinese collection of 202 cultivars and a core world collection of 327 cultivars, were grown in Wuhan (114°21′E, 30°28′N; the average day length is more than 13.5 h from the middle of May to the beginning of August; 23–31°C) in the summer of 2013 and Hainan (110°01′E, 18°30′N; the average day length was less than 12.5 h from December to the middle of March; 20–28°C) in the winter of 2013 (https://www.timeanddate.com, https://www.climatestotravel.com). The details of field management and measurement of heading date are described in a previous study (Han et al., 2016). The 529 accessions were genotyped via sequencing and classified into four subpopulations: indica, aus, japonica, and Admixture (Chen et al., 2014). Single nucleotide polymorphism (SNP) information about the 529 cultivars is available on RiceVarMap v1.0 (http://ricevarmap.ncpgr.cn/v1/), which is a comprehensive database of rice genomic variations. All transgenic plants were grown in the field in Wuhan (LD) in summer 2018 and Hainan (SD) in spring 2019. Heading date was individually scored as the number of days from sowing to the emergence of the first panicle on the plant. Plant height was measured from the surface to the top of the main panicle.
Haplotype Construction and Haplotype-Based Association Analysis
To obtain sequencing data for all Dof genes in rice, the conserved Dof domain sequence of the known protein SP3 was used to search the Rice Genome Annotation Project (http://rice.plantbiology.msu.edu) and NCBI (https://www.ncbi.nlm.nih.gov/) (Huang et al., 2018). A total of 30 Dof genes were identified.
For development of haplotypes, first, SNPs in Dof genes were extracted from the sequences containing a 2-kb promoter region and the gene body region. Then, PHASE software, which can estimate missing and heterozygous genotypes, was used to construct haplotypes (Stephens et al., 2001). Finally, the haplotypes with an allele frequency ≥0.01 (five accessions) were included for association analysis. Analysis of variance (ANOVA) was used to identify the association between haplotype and heading date. Duncan's test was employed to perform multiple comparisons between all possible haplotype pairs. The phenotypic variation explained by these 22 Dof genes was calculated by ANOVA with a linear model including all these genes.
Nucleotide Diversity Analyses
DNA sequences of each Dof family gene in 1,612 cultivar accessions and 446 Oryza rufipogon accessions were obtained from the ECOGEMS database (http://ecogems.ncpgr.cn). Each sequence contained a 2-kb promoter region and a gene body region. The details of these accessions and their sequencing data have been previously reported (Huang et al., 2012a). The nucleotide diversity (π), Tajima's D, and Fu and Li's D statistics were calculated both in cultivars and wild rice (O. rufipogon) using the DnaSP 6.0 program (Rozas et al., 2017).
Vector Construction and Transformation
To generate knockout mutants of Dof family genes by CRISPR/Cas9, 19- and 20-bp fragments in the exon regions of Dof genes were chosen as the candidate targets according to the design principles of the target sequences in the CRISPR/Cas9 system (Supplementary Table S1). At least one target was in the exon regions of each Dof gene (Supplementary Figure S1). The two target fragments were introduced into two sgRNA expression cassettes by dual-nested PCR, driven by the OsU6a and OsU6b promoters, respectively. Then, the multiple sgRNA expression cassettes were ligated to the CRISPR/Cas9 binary vector (pYLCRISPR/Cas9Pubi-H) based on Golden Gate cloning (Ma et al., 2015). Finally, 30 recombinant CRISPR/Cas9 constructs were introduced into Agrobacterium tumefaciens strain EHA105 and separately transferred into ZH11 by Agrobacterium-mediated transformation (Lin and Zhang, 2005).
Mutation Detection and Transgene-Free Line Screening
T0 transgenic plants were used to detect mutations. Genomic DNA was extracted from the leaves of T0 plants using the cetyltrimethyl ammonium bromide (CTAB) method. Primer pairs flanking the designated target sites were used to amplify the potentially mutated fragments. PCR products were sequenced to detect mutations. Homozygous mutations were identified using the Sequencher 5.1 Demo software and Degenerate Sequence Decoding method (DSDecodeM; Liu et al., 2015). In our study, the homozygous transgenic knockout lines were transgene-free (or transgene-clean) mutant lines, which were obtained by screening the recombinants between Cas9 and the targeted mutant gene in the selfing progeny. Thus, the phenotypic evaluation was performed between transgene-clean mutant lines and wild type, not the negative plants. The seeds were harvested from each homozygous T0 plant, and the transgene-free plants were identified from T1 homozygous families by agarose gel electrophoresis to separate PCR products with Cas9-specific primers. Cas9-negative plants were transgene-free. CRISPR/Cas9 plasmid DNA was used as a positive control, and ZH11 DNA and H2O were used as negative controls.
Results
Gene Structure and Haplotype Analysis of Dof Family Genes
The 30 Dof genes were not evenly distributed in the genome. Chromosomes 1 and 3 each had the largest number of genes (six), and no Dof genes were located on chromosome 11 (Table 1). In general, Dof family genes had relatively simple structures. Of all Dof genes, 17, 12, and 1 had no intron, one intron, and two introns, respectively (Supplementary Figure S1). The number of SNPs in the 2-kb promoter and entire genomic sequence of Dof family genes ranged from 14 (OsDof14) to 113 (OsDof10; Table 1). Based on these SNPs, we constructed the haplotypes of all 30 genes in the germplasm collection of 529 accessions. In the full population, the number of haplotypes ranged from 4 to 20, among which OsDof10 had the largest number (twenty) of haplotypes, whereas OsDof18 had the lowest number (four) of haplotypes. Additionally, with the exception of OsDof4, OsDof21, OsDof29, and OsDof30, all Dof genes had more haplotypes in the indica subpopulation than the japonica subpopulation (Table 1).
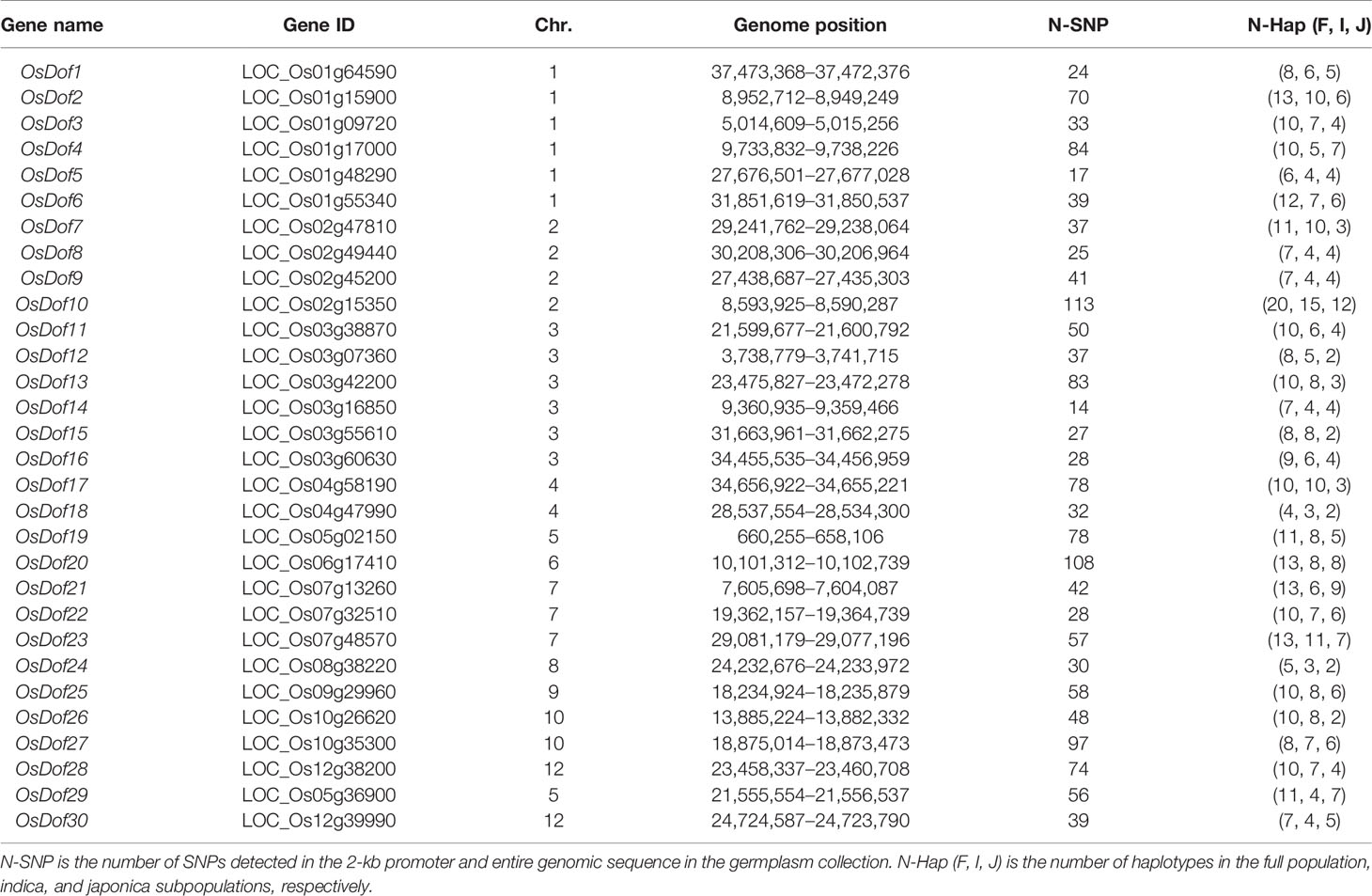
Table 1 Genome positions of Dof family genes and their numbers of SNPs and haplotypes in 529 accessions.
Haplotype-Based Association Analysis of Dof Genes With Heading Date
Heading date had a high heritability of 0.75 across both conditions. However, SNP-based GWAS only identified a few loci that were significantly associated with heading date, and they only explained a small fraction of heading date variance (Han et al., 2016). Dof family genes were not significantly associated with heading date in GWAS (Supplementary Figure S2). Candidate gene-based association analysis for heading date showed that more Dof genes could be associated with heading date under LD and SD conditions at the haplotype level (Table 2). Under LD, 17 genes (OsDof1, 3, 6, 7, 8, 10, 11, 12, 13, 16, 20, 21, 22, 23, 25, 29, and 30), 10 genes (OsDof1, 3, 6, 7, 8, 11, 13, 16, 20, and 21) and 7 genes (OsDof7, 10, 11, 12, 20, 23, and 25) were significantly associated with heading date in the full population, both the full population and the indica subpopulation, and both the full population and the japonica subpopulation, respectively. Three genes (OsDof7, 11, and 20) were commonly identified in the full population, the indica subpopulation, and the japonica subpopulation (Table 2). Under SD, 21 genes (OsDof2, 3, 6, 7, 8, 10, 11, 12, 13, 14, 16, 19, 20, 21, 22, 23, 25, 26, 27, 29, and 30), 7 genes (OsDof2, 6, 10, 11, 14, 16, and 20), and 14 genes (OsDof2, 6, 7, 10, 11, 19, 20, 21, 22, 23, 25, 27, 29, and 30) were significantly associated with heading date in the full population, both the full population and the indica subpopulation, and both the full population and the japonica subpopulation, respectively. Five genes (OsDof2, 6, 10, 11, and 20) were commonly associated with heading date in the full population, indica subpopulation, and japonica subpopulation (Table 2). Moreover, 16 genes (OsDof3, 6, 7, 8, 10, 11, 12, 13, 16, 20, 21, 22, 23, 25, 29, and 30) were commonly associated with heading date in the full population under both LD and SD. Two genes (OsDof11 and 20) were commonly detected in the full population and two subpopulations under both conditions (Table 2). These 22 Dof genes cumulatively explained about 18.4% of the variation in heading date in the whole collection.
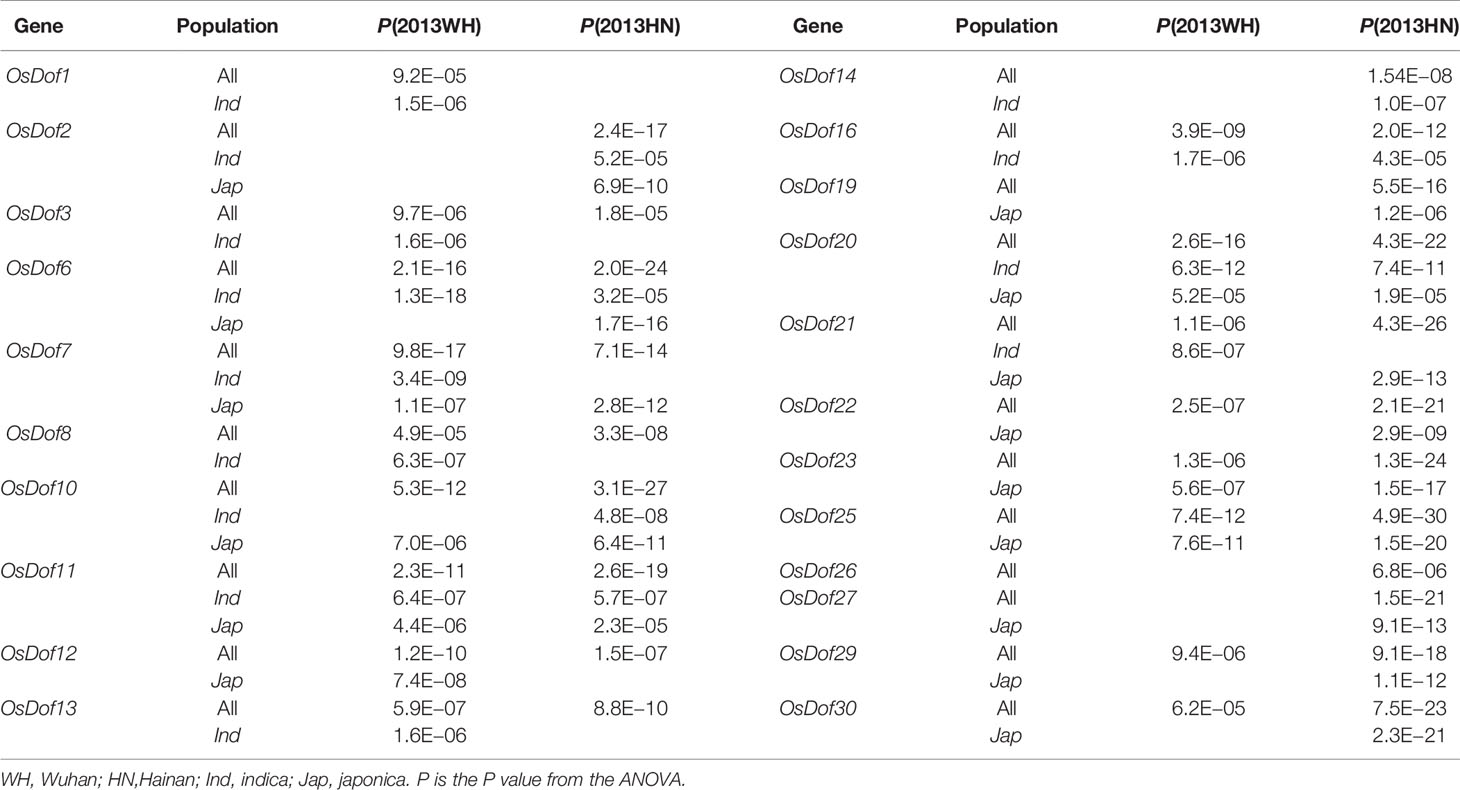
Table 2 Haplotype-based association analyses of Dof family genes for heading date in different environments.
Comparison of Haplotype Effects on Heading Date
Multiple comparisons of haplotypes were made for heading date-associated genes. Under LD, significant differences in heading date were detected among major haplotypes of 10 genes (OsDof1, 6, 7, 10, 11, 12, 13, 16, 20, and 21) in both indica and japonica accessions (Supplementary Table S2). Under SD, a similar result was also observed in these 10 genes except for OsDof1 and OsDof12 (Supplementary Table S2).
For example, in OsDof20, nine major haplotypes were obtained, and most indica accessions were classified into Hap1, Hap2, Hap3, Hap4, Hap5, and Hap6. The majority of the aus and japonica accessions carried Hap7, Hap8, and Hap9 (Figure 1A). In indica rice, Hap4 produced significantly delayed heading (127.7 ± 7.2 days) under LD and promoted heading (85.9 ± 3.7 days) under SD compared to other haplotypes (Figure 1B). In japonica rice, Hap8 significantly delayed heading date (114.9 ± 11.4 days) compared to Hap9 (93.4 ± 13.6 days) and Hap7 (103.1 ± 11.3 days) under LD. Under SD, Hap9 (88.6 ± 5.3 days) significantly promoted heading date compared to Hap7 (96.1 ± 10.7 days) (Figure 1C). Although no SNPs causing amino acid changes were detected in the OsDof20 coding region, some SNPs (sf0610098412, sf0610098666, sf0610099067, sf0610099607, sf0610100080, and sf0610100141) in the promoter region were associated with heading date (Figure 1A). Therefore, the genetic variation in the promoter regions of OsDof20 may lead to a significant difference in heading date.
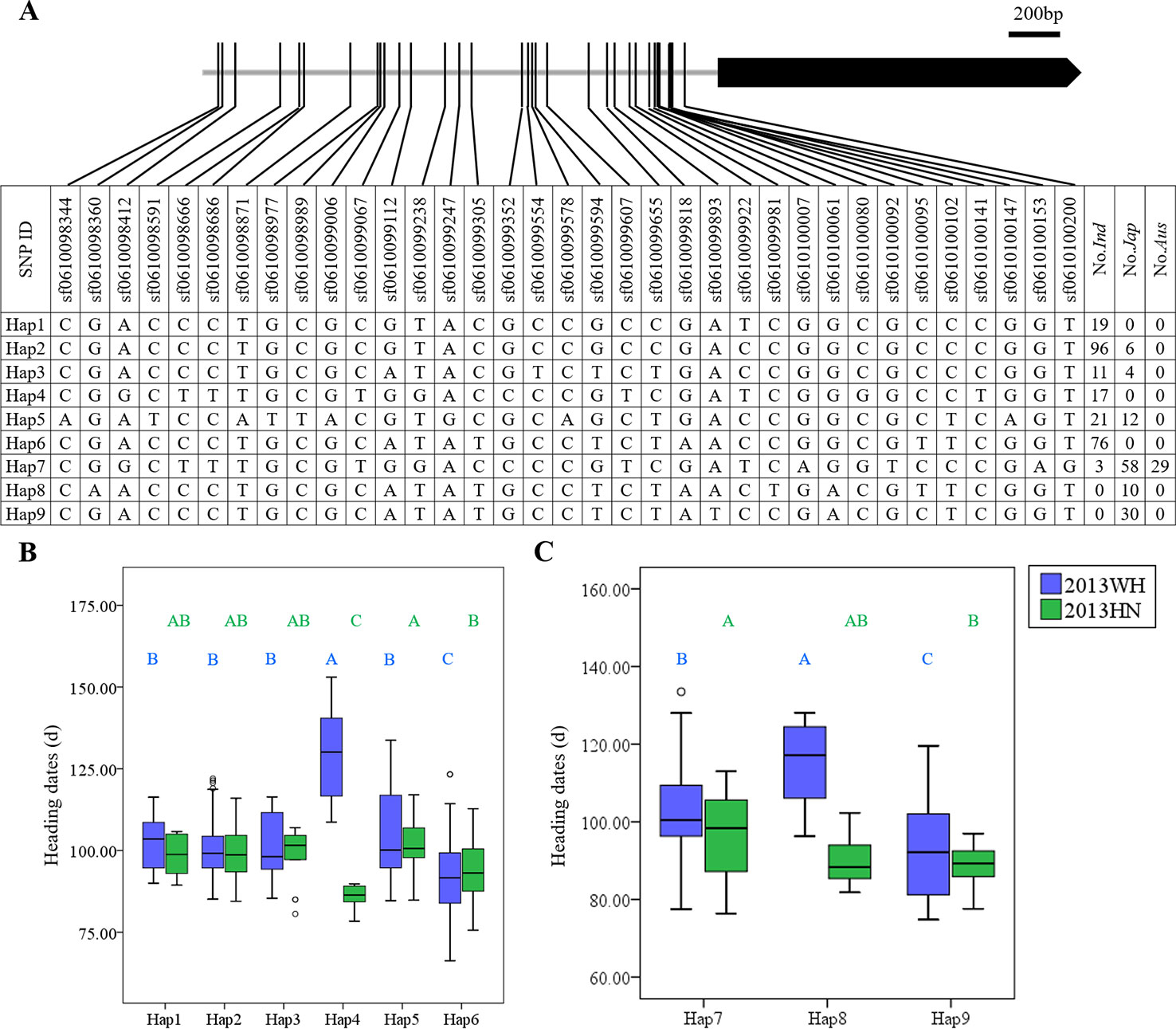
Figure 1 Haplotype analysis of OsDof20. (A) Major haplotypes (each haplotype contains more than 10 accessions) of OsDof20 in the full population according to SNPs data from RiceVarMap version 1. The region contains 2 kb upstream and coding region. (B) Comparison of haplotype effects in heading dates among Hap1–Hap6 in indica rice using a Duncan's test (P < 0.01), respectively. (C) Comparison of heading dates among Hap7–Hap9 in japonica rice using a Duncan's test (P < 0.01), respectively.
OsDof12 had six major haplotypes in the germplasm collection. Hap1, Hap2, and Hap3 were mainly harbored by the indica subpopulation, Hap4 and Hap5 were primarily harbored by the japonica subpopulation, and most aus accessions belonged to Hap6 (Figure 2A). Under LD, Hap2 (104.4 ± 5.9 days) and Hap3 (101.0 ± 10.7 days) significantly delayed heading date compared to Hap1 (91.1 ± 9.1 days) within indica rice (Figure 2B), and Hap4 (114.8 ± 10.9 days) significantly delayed heading date compared to Hap5 (94.2 ± 13.2 days) in japonica rice (Figure 2C). These functionally diverse haplotypes provide us with an opportunity to improve rice heading date for specific ecotypes. However, under SD, there were no significant differences in heading date among major haplotypes in either indica or japonica rice (Figures 2B, C). Interestingly, only one SNP (sf0303739795) causing a non-synonymous mutation (G267A) was detected in the coding region of OsDof12, and most aus, indica, and japonica accessions carried Gly at site 267 (Hap2–Hap6). Only a small minority of indica accessions carried Hap1 with Ala at site 267 (Figure 2A). Within indica rice, Hap2 with G267 and Hap3 with G267 significantly delayed heading date compared to Hap1 with A267 in LD (Figure 2B). Therefore, G267A might be the causal mutation for the variation of heading date in indica rice. Accordingly, OsDof1 had significant effects on heading date in both indica and in japonica rice under LD, whereas no significant effects were observed under SD (Supplementary Table S2).
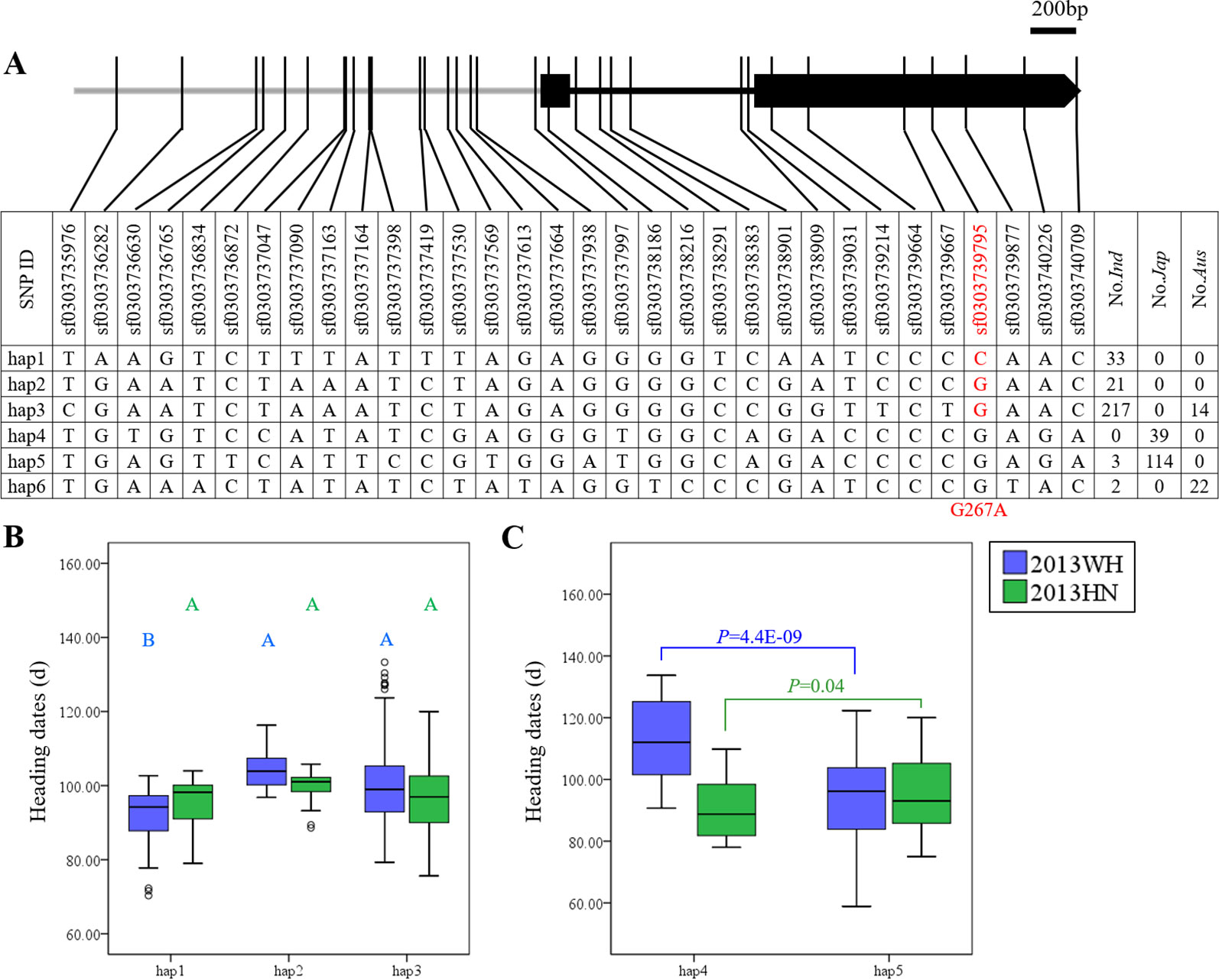
Figure 2 Haplotype analysis of OsDof12. (A) Major haplotypes (each haplotype contains more than 10 accessions) of OsDof12 in the full population according to SNP data from RiceVarMap version 1. The region contains 2 kb upstream and coding region. The SNP in red is non-synonymous. (B) Comparison of haplotype effects in heading dates among Hap1–Hap3 in indica rice using a Duncan's test (P < 0.01), respectively. (C) Comparison of heading dates between Hap4 and Hap5 in japonica rice by Student's t test.
In addition, five genes (OsDof2, 23, 25, 29, and 30) had significant effects in japonica accessions and not in indica rice under SD (Supplementary Table S2). These five genes, except OsDof2, also had similar effects under LD (Supplementary Table S2). In the case of OsDof23, six major haplotypes were obtained. Hap1, Hap2, and Hap3 were mainly harbored by the indica subpopulation, whereas Hap4, Hap5, and Hap6 were primarily harbored by the japonica subpopulation (Figure 3A). Two SNPs (sf0729076798 and sf0729080067) in the coding region caused amino acid changes. The SNP at site sf0729076798 caused a non-synonymous mutation (D400N). Most aus, indica, and japonica accessions were divided into Hap1–Hap5 and shared an Asp at site 400. A small proportion of japonica accessions possessed Hap6 with Asn at site 400 (Figure 3A). No significant effects were detected in indica rice in either LD or SD (Figure 3B), whereas the effects were significant in japonica rice (Figure 3C). Hap6 with N400 (69.2 ± 9.9 days under LD and 81.6 ± 4.3 days under SD) significantly promoted heading date compared to Hap4 with D400 (100.4 ± 5.4 days under LD and 105.5 ± 7.2 days under SD) and Hap5 with D400 (104.8 ± 13.5 days under LD and 89.9 ± 7.7 days under SD; Figure 3C). Therefore, sf0729076798 might be a functional nucleotide polymorphism site in japonica rice.
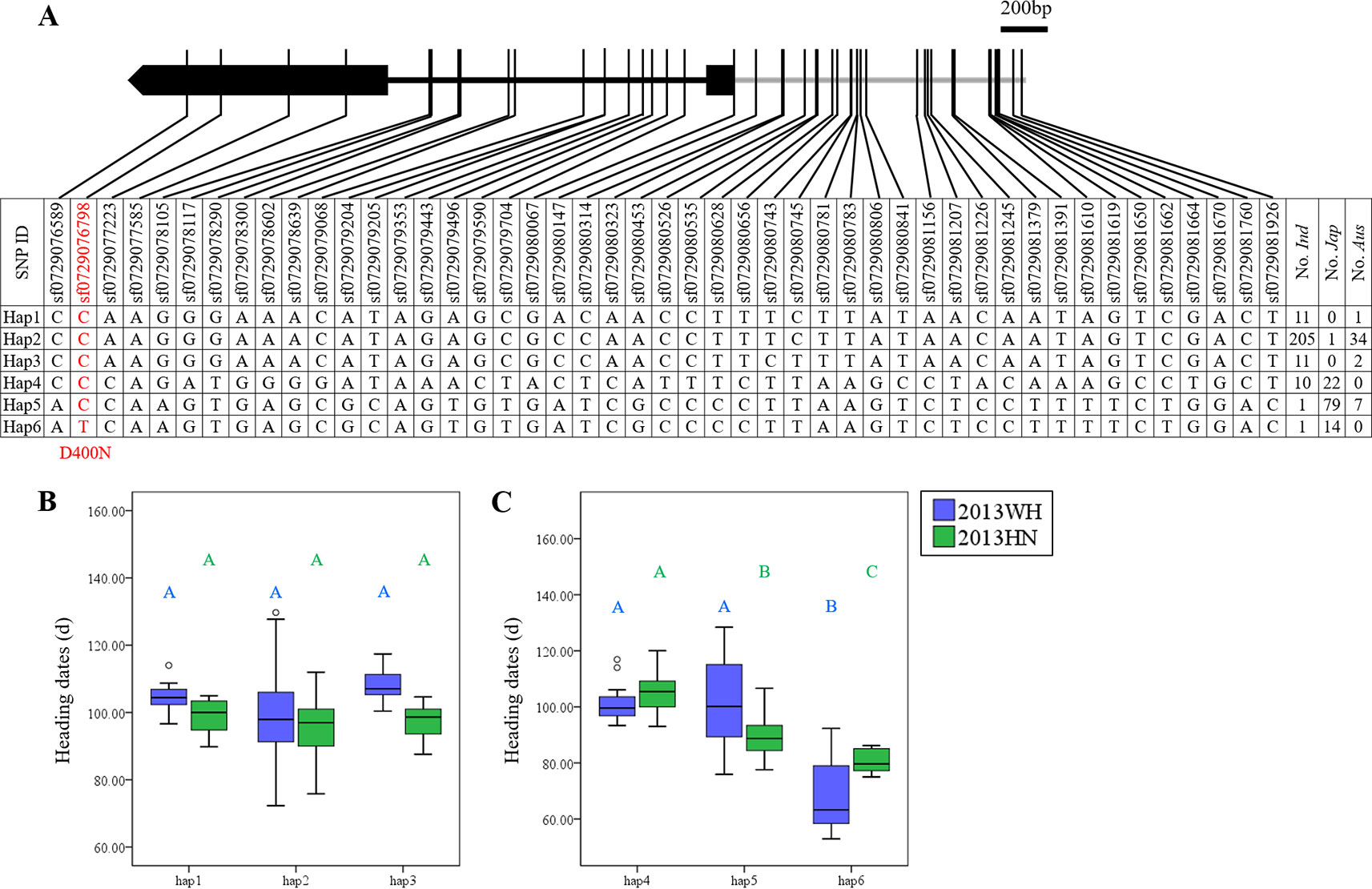
Figure 3 Haplotype analysis of OsDof23. (A) Major haplotypes (each haplotype contains more than 10 accessions) of OsDof23 in the full population according to SNP data from RiceVarMap version 1. The region contains 2 kb upstream and coding region. The SNP in red is non-synonymous. (B) Comparison of haplotype effects in heading dates among Hap1–Hap3 in indica rice using a Duncan's test (P < 0.01), respectively. (C) Comparison of heading dates among Hap4–Hap6 in japonica rice using a Duncan's test (P < 0.01), respectively.
Mutagenesis of Dof Genes by CRISPR/Cas9
Multiple positive plants for each gene were harvested from T0 transgenic plants of all Dof family genes generated by the CRISPR/Cas9 system (Supplementary Table S3). Transgene-free homozygous knockout lines with various mutations in 30 Dof genes were identified by sequencing. The mutations included small fragment insertions (1 bp), deletions (1–17 bp), and large fragment deletions (111–2,371 bp; Supplementary Figure S3). Because the two target sites were designed in the exon region (at least one target fell in the coding region) of Dof genes, each mutation occurred in the coding region and led to a frameshift or truncation mutation in the protein that may completely deactivate the protein function.
Identification of Dof Family Genes Regulating Heading Date Using Mutants
At least three independent mutant lines for each Dof gene were analyzed for heading date. Compared with the wild-type ZH11, the CRISPR/Cas9 mutated plants of four Dof family genes (OsDof1, 11, 21, and 29) promoted heading by approximately 3–4 days under LD (Figures 4A, B, E, Table 3, and Supplementary Figure S4). Under SD, a similar result was also observed in these four genes except for OsDof11 and OsDof29 (Supplementary Table S4 and Figure S4). Mutants of seven genes (OsDof2, 8, 9, 16, 22, 24, and 26) delayed heading by 2–5 days under both LD and SD (Figures 4C–E, Table 3, Supplementary Table S4, and Figure S4). The Dof family gene mutants also had effects on plant height (−14.6 to +14.1 cm under LD; −22 to +4.9 cm under SD; Figure 4F, Table 3, and Supplementary Table S4). Other members did not show any difference in heading date compared to the wild type (Table 3).
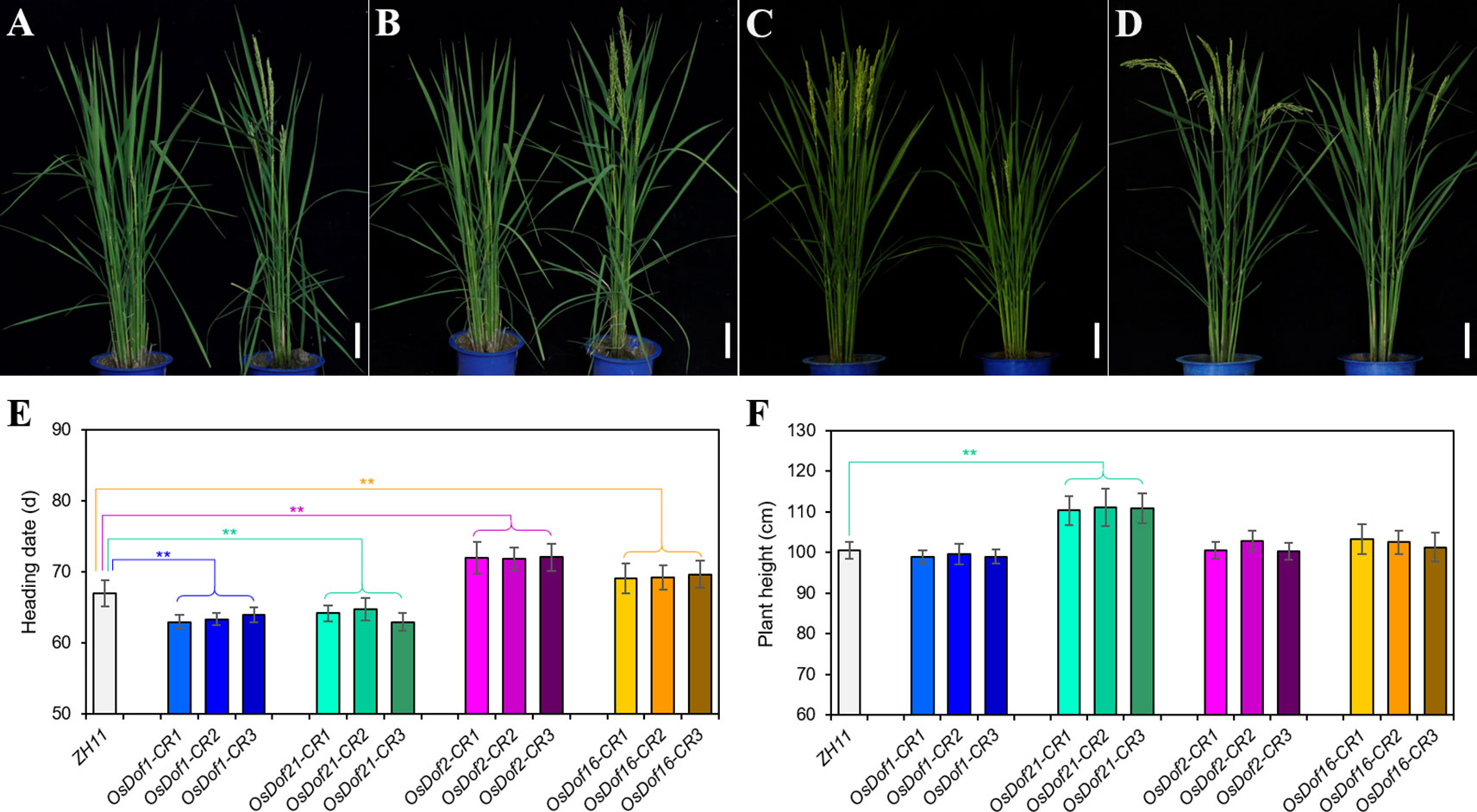
Figure 4 Performance of Dof gene mutants generated by utilizing CRISPR/Cas9 technology. (A–D) Plant appearance of OsDof1-CRISPR (OsDof1-CR) (A), OsDof21-CRISPR (OsDof21-CR) (B), OsDof2-CRISPR (OsDof2-CR) (C), and OsDof16-CRISPR (OsDof16-CR) (D) transgenic plants [all homozygous mutants in the T1 generation (right) and ZH11 wild type (left)], respectively. (E, F) Comparison of heading dates (E) and plant height (F) between the mutants (OsDof1-CR, OsDof21-CR, OsDof2-CR, and OsDof16-CR) and ZH11 wild type, respectively. **P < 0.01 [n = 20 plants for (E, F); Student's t test]. Scale bars = 10 cm in (A–D).
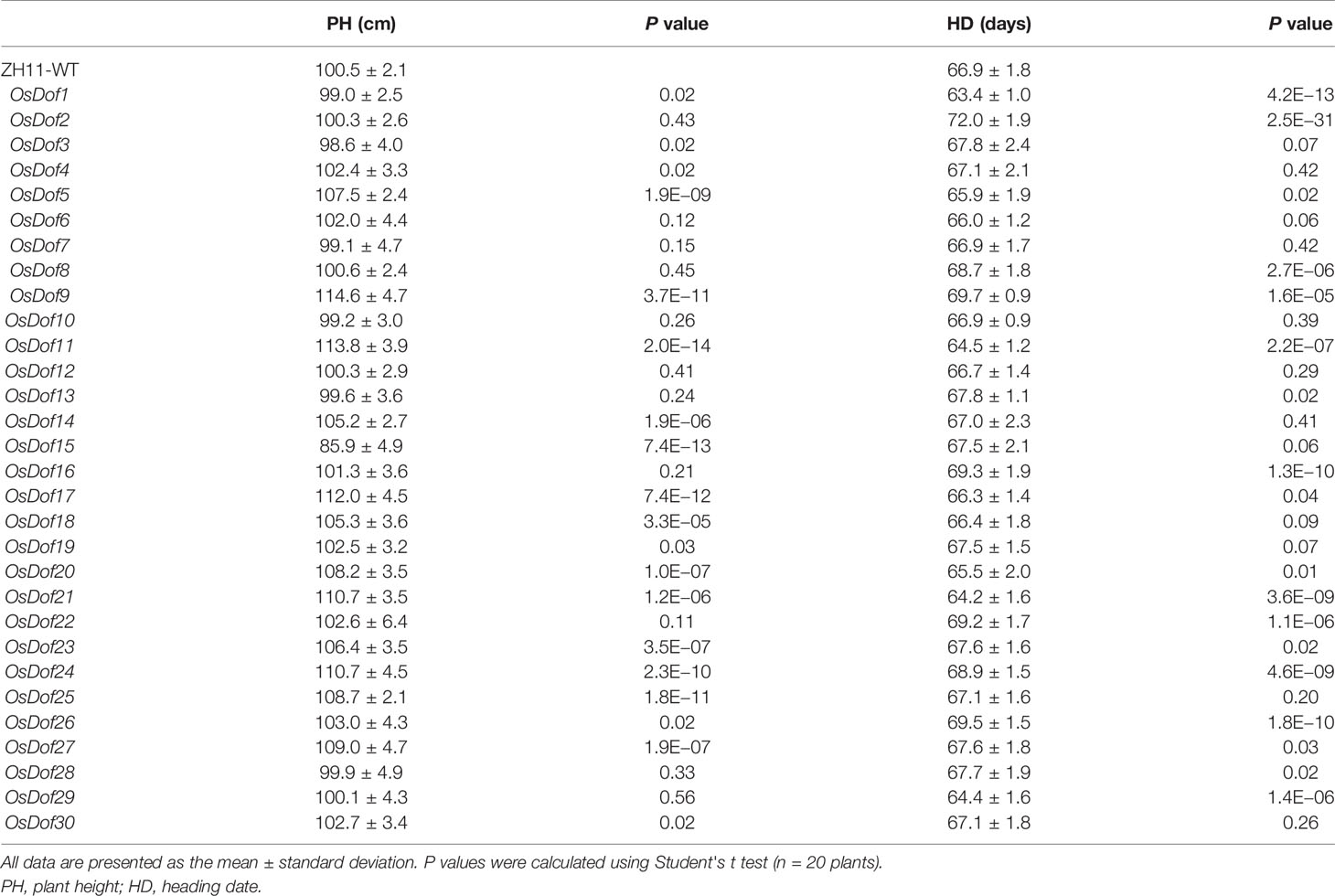
Table 3 Comparison of heading date and plant height traits in the CRISPR/Cas9-mediated knockout mutants of Dof family genes with the wild-type Zhonghua11 under long-day condition.
Nucleotide Diversity of Dof Genes
We analyzed the nucleotide diversity of Dof family genes in 446 wild rice and 1,612 cultivars. There were 2,822 SNPs across the entire sequence (including 2 kb of promoter and the gene body region) of the Dof family genes among 2,058 accessions (Supplementary Table S5). The pairwise nucleotide diversity ranged from 7 to 59 SNPs per kilobase for Dof genes (Supplementary Table S5). The average nucleotide diversity (π = 2.4 × 10−3) of Dof family genes in both O. sativa (π = 1.9 × 10−3) and wild rice (π = 2.9 × 10−3) was equivalent to that of the full O. sativa genome (π = 2.4 × 10−3). The ratio of nucleotide diversity in cultivars (πc) to nucleotide diversity in wild rice (πw) for OsDof2, 4, 10, 16, 18, and 24 was less than 0.5 (Supplementary Table S5), indicating that these genes underwent selection during domestication and genetic improvement. In particular, OsDof24 had a very low ratio of πc/πw of 0.17, a 5.8-fold reduction in genetic variation in O. sativa (Supplementary Table S5). Among the genes that have encountered selection, OsDof2, 16, and 24 regulated heading date. Tajima's D of two Dof genes (OsDof7 and 21) was negative in wild rice and reached a significant level (P < 0.05) (Supplementary Table S5), suggesting that OsDof7 and 21 have been subjected to purifying selection in wild rice. Moreover, Fu and Li's D value for OsDof5, 8, 12, 14, 17, 18, 22, and 29 in cultivars and OsDof3, 5, and 7 in wild rice deviated significantly from neutrality (P < 0.05) (Supplementary Table S5). These results suggested that these genes have been subjected to natural selection in wild rice and artificial selection in cultivar rice.
Discussion
Minor Effects of Dof Genes on Heading Date Has Contributed to the Missing Heritability in Rice
According to the “omnigenic” model, a complex quantitative trait is controlled by a few core genes (major genes) and a large number of peripheral genes (minor genes), and the sum of minor effects across peripheral genes can far exceed the genetic contribution of core genes (Boyle et al., 2017). This statement is supported by the so-called missing heritability (Manolio et al., 2009). Much missing heritability is due to peripheral genes whose effects are too small to reach the level of genome-wide significance (Yang et al., 2015). Heading date shows high heritability in linkage mapping populations and germplasm collections. Moreover, these core heading date genes explain only a fraction of the total heritability in both GWAS and biparental mapping populations (Xue et al., 2008; Huang et al., 2010; Yan et al., 2011; Huang et al., 2012b; Yan et al., 2013; Han et al., 2016; Yano et al., 2016). There must be many genes with minor effects spread widely across the genome. It is often difficult to identify unknown genes with minor effects due to weak association signals in GWAS at the SNP level and stringent thresholds in linkage analysis. Like our Dof family genes, whose effects are too small to be significantly associated with heading date in GWAS (Supplementary Figure S2).
Heading date shows high heritability (0.75), but quantitative trait loci (QTLs) revealed by GWAS only explain 40.6% of the phenotype variation (Han et al., 2016). In this study, 22 Dof genes were associated with heading date via candidate gene-based association analysis at the haplotype level (Supplementary Figure S4). They all together explained about 18.4% of the variation in heading date. Moreover, mutants in 11 Dof genes showed a 2- to 5-day difference in heading date compared to wild-type ZH11. It is very likely that there is gene redundancy among Dof family genes, which results in false negatives. In addition, it is possible that some Dof genes in ZH11 (the transformation background genotype) are weak-functional or non-functional because ZH11 is not strongly sensitive to photoperiod (Xue et al., 2008; Yan et al., 2013; Zhang et al., 2015a). Therefore, it is understandable that some knockout mutants show no phenotype change or minor effect. But at least 11 Dof genes probably regulate heading date, none of which has been previously mapped, with the exception of OsDof2. These Dof genes with minor effects adequately explain part of the missing heritability. In fact, there are CCT family genes and HAP family genes in rice, and some of them also control heading date, but they have not been mapped by QTL analysis (Zhang et al., 2015b; Li et al., 2016). These minor genes together contribute to the missing heritability of heading date.
Dof Family Genes Have Diverse Responses to Long-Day and Short-Day Conditions
In this study, we detected many Dof genes related to heading date using haplotype-based association analysis under LD (Wuhan) and SD (Hainan). Of them, 16 genes (OsDof3, 6, 7, 8, 10, 11, 12, 13, 16, 20, 21, 22, 23, 25, 29, and 30) in the full population, four genes (OsDof6, 11, 16, and 20) in indica, and six genes (OsDof7, 10, 11, 20, 23, and 25) in japonica were commonly detected under both LD and SD (Table 2), which indicated that these Dof genes simultaneously contributed to the heritability of heading date under both conditions. One gene (OsDof1) in the full population, six genes (OsDof1, 3, 7, 8, 13, and 21) in indica, and one gene (OsDof12) in japonica were detected under LD (Table 2), suggesting that these genes primarily function under LD. These results were supported by a previous report on OsDof12 in which overexpressed OsDof12 promoted rice flowering under LD, whereas overexpression of OsDof12 had no effect under SD (Li et al., 2009). In addition, five genes (OsDof2, 14, 19, 26, and 27) in the full population, three genes (OsDof2, 10, and 14) in indica, and eight genes (OsDof2, 6, 19, 21, 22, 27, 29, and 30) in japonica were detected under SD (Table 2), which implied that these genes primarily function under SD. Furthermore, OsDof2 loss-of-function mutants generated by CRISPR/Cas9 showed a delay in flowering time compared with wild-type plants under both LD and SD, which was consistent with previous studies (Iwamoto et al., 2009; Iwamoto and Tagiri, 2016). However, OsDof2 was only detected under SD in this study (Table 2). Taken together, Dof family genes have distinct responses to photoperiod.
There Is Functional Redundancy and Differentiation Among Dof Family Genes
In general, functional redundancy has been reported among related genes in rice (Zhang et al., 2015b; Li et al., 2016). When knockdown or knockout mutagenesis is used for studying the function of related genes, gene redundancy can cause unclear results. In our study, there were 19 genes (Table 2) whose knockout mutants had heading date that were indistinguishable from wild type. Phylogenetic analysis showed that six pairs of genes, OsDof1 and OsDof29, OsDof2 and OsDof23, OsDof9 and OsDof18, OsDof12 and OsDof26, OsDof13 and OsDof30, and OsDof24 and OsDof25, share a very conserved gene structure outside the Dof domain (Huang et al., 2018), which is probably responsible for their similar functions. For example, knockout mutants of both OsDof1 and OsDof29 promoted flowering time under LD (Table 3). Additionally, some Dof genes may have functional redundancy, which causes single mutants to have wild-type phenotypes. For example, knockout mutants of both OsDof13 and OsDof30 did not show any change in heading date from the wild type under LD. To specify which genes are functionally redundant, double or triple mutants are needed for phenotype analysis. In addition, knockout mutants of OsDof2, OsDof9, OsDof24, and OsDof26 all show delayed heading under LD, whereas mutants in their corresponding evolutionarily most closely related genes OsDof23, OsDof18, OsDof25, and OsDof12 did not show any difference in heading date, suggesting a possible functional differentiation between them. It has been reported that some Dof genes are probably involved in stress resistance (Corrales et al., 2014). Therefore, the mutants generated in this study can also be used to evaluate stress resistance.
Conclusion
In summary, out of 30 Dof genes, haplotype-based association analysis identified 22 genes associated with heading date either under long-day or short-day conditions. Favorable alleles were provided for improvement of varieties with different ecotypes. A set of knockout mutants of all 30 Dof genes showed that 11 and 9 Dof genes regulated heading date under long-day and short-day conditions, respectively. Of them, nine genes regulated heading date under both conditions. Functional redundancy might mask the phenotype of single mutants and result in missing identification of flowering Dof genes. The minor effects of Dof family genes that could not be mapped using GWAS make considerable contribution to the missing heritability of heading date in rice.
Data Availability Statement
All datasets generated for this study are included in the article/Supplementary Material.
Author Contributions
YX and YH designed the experiments. YH performed most of the experiments. YH and ZH analyzed and summed the data and drew the figures. NC, ML, and XB assisted in experiments and discussed the results. YH wrote the manuscript. YX revised the manuscript.
Funding
This work was supported by grants from the National Key Research and Development Program of China (2016YFD0100403), the National Natural Science Foundation of China (31571751) and the Natural Science Foundation of Hubei Province, China (2015CFA006), and the Project for Applied Basis Research, Wuhan, China (2016020101010090).
Conflict of Interest
The authors declare that the research was conducted in the absence of any commercial or financial relationships that could be construed as a potential conflict of interest.
Acknowledgments
We thank the farm technician Mr. JB Wang for his excellent work in the field. We thank Professor Yaoguang Liu in South China Agriculture University for kindly providing the robust CRISPR/Cas9 vector system.
Supplementary Material
The Supplementary Material for this article can be found online at: https://www.frontiersin.org/articles/10.3389/fpls.2019.01739/full#supplementary-material
References
Boyle, E. A., Li, Y. I., Pritchard, J. K. (2017). An expanded view of complex traits: from polygenic to omnigenic. Cell 169, 1177–1186. doi: 10.1016/j.cell.2017.05.038
Chen, W., Gao, Y., Xie, W., Gong, L., Lu, K., Wang, W., et al. (2014). Genome-wide association analyses provide genetic and biochemical insights into natural variation in rice metabolism. Nat. Genet. 46, 714–721. doi: 10.1038/ng.3007
Chen, P., Shen, Z., Ming, L., Li, Y., Dan, W., Lou, G., et al. (2018). Genetic basis of variation in rice seed storage protein (Albumin, Globulin, Prolamin, and Glutelin) content revealed by genome-wide association analysis. Front. Plant Sci. 9, 612. doi: 10.3389/fpls.2018.00612
Cong, L., Ran, F. A., Cox, D., Lin, S., Barretto, R., Habib, N., et al. (2013). Multiplex genome engineering using CRISPR/Cas systems. Science 339, 819–823. doi: 10.1126/science
Corrales, A. R., Nebauer, S. G., Carrillo, L., Fernandez-Nohales, P., Marques, J., Renau-Morata, B., et al. (2014). Characterization of tomato Cycling Dof Factors reveals conserved and new functions in the control of flowering time and abiotic stress responses. J. Exp. Bot. 65, 995–1012. doi: 10.1093/jxb/ert451
Dong, H., Zhao, H., Li, S., Han, Z., Hu, G., Liu, C., et al. (2018). Genome-wide association studies reveal that members of bHLH subfamily 16 share a conserved function in regulating flag leaf angle in rice (Oryza sativa). PloS Genet. 14, e1007323. doi: 10.1371/journal.pgen.1007323
Ewas, M., Khames, E., Ziaf, K., Shahzad, R., Nishawy, E., Ali, F., et al. (2017). The Tomato DOF Daily Fluctuations 1, TDDF1 acts as flowering accelerator and protector against various stresses. Sci. Rep. 7, 10299. doi: 10.1038/s41598-017-10399-7
Han, Z., Zhang, B., Zhao, H., Ayaad, M., Xing, Y. (2016). Genome-wide association studies reveal that diverse heading date genes respond to short and long day lengths between Indica and Japonica Rice. Front. Plant Sci. 7, 1270. doi: 10.3389/fpls.2016.01270
Huang, X., Sang, T., Zhao, Q., Feng, Q., Zhao, Y., Li, C., et al. (2010). Genome-wide association studies of 14 agronomic traits in rice landraces. Nat. Genet. 42, 961–967. doi: 10.1038/ng.695
Huang, X., Kurata, N., Wei, X., Wang, Z. X., Wang, A., Zhao, Q., et al. (2012a). A map of rice genome variation reveals the origin of cultivated rice. Nature 490, 497–501. doi: 10.1038/nature11532
Huang, X., Zhao, Y., Li, C., Wang, A., Zhao, Q., Li, W., et al. (2012b). Genome-wide association study of flowering time and grain yield traits in a worldwide collection of rice germplasm. Nat. Genet. 44, 32–39. doi: 10.1038/ng.1018
Huang, Y., Liu, H., Xing, Y. (2017). Next-generation sequencing promoted the release of reference genomes and discovered genome evolution in cereal crops. Curr. Issues Mol. Biol. 27, 37–50. doi: 10.21775/cimb.027.037
Huang, Y., Bai, X., Luo, M., Xing, Y. (2018). Short Panicle 3 controls panicle architecture by upregulating APO2/RFL and increasing cytokinin content in rice. J. Integr. Plant Biol. 61, 987–999. doi: 10.1111/jipb.12729
Hwang, W. Y., Fu, Y., Reyon, D., Maeder, M. L., Tsai, S. Q., Sander, J. D., et al. (2013). Efficient genome editing in zebrafish using a CRISPR-Cas system. Nat. Biotechnol. 31, 227–229. doi: 10.1038/nbt.2501
Iwamoto, M., Tagiri, A. (2016). MicroRNA-targeted transcription factor gene RDD1 promotes nutrient ion uptake and accumulation in rice. Plant J. 85, 466–477. doi: 10.1111/tpj.13117
Iwamoto, M., Higo, K., Takano, M. (2009). Circadian clock- and phytochrome-regulated Dof-like gene, Rdd1, is associated with grain size in rice. Plant Cell Environ. 32, 592–603. doi: 10.1111/j.1365-3040.2009.01954.x
Jain, M., Kaur, N., Tyagi, A. K., Khurana, J. P. (2006). The auxin-responsive GH3 gene family in rice (Oryza sativa). Funct. Integr. Genomics 6, 36–46. doi: 10.1007/s10142-005-0142-5
Li, D., Yang, C., Li, X., Gan, Q., Zhao, X., Zhu, L. (2009). Functional characterization of rice OsDof12. Planta 229, 1159–1169. doi: 10.1007/s00425-009-0893-7
Li, Q., Yan, W., Chen, H., Tan, C., Han, Z., Yao, W., et al. (2016). Duplication of OsHAP family genes and their association with heading date in rice. J. Exp. Bot. 67, 1759–1768. doi: 10.1093/jxb/erv566
Lijavetzky, D., Carbonero, P., Vicente-Carbajosa, J. (2003). Genome-wide comparative phylogenetic analysis of the rice and Arabidopsis Dof gene families. BMC Evol. Biol. 3, 17. doi: 10.1186/1471-2148-3-17
Lin, Y. J., Zhang, Q. (2005). Optimising the tissue culture conditions for high efficiency transformation of indica rice. Plant Cell Rep. 23, 540–547. doi: 10.1007/s00299-004-0843-6
Liu, W., Xie, X., Ma, X., Li, J., Chen, J., Liu, Y.-G. (2015). DSDecode: a web-based tool for decoding of sequencing chromatograms for genotyping of targeted mutations. Mol. Plant 8, 1431–1433. doi: 10.1016/j.molp.2015.05.009
Lu, K., Wu, B., Wang, J., Zhu, W., Nie, H., Qian, J., et al. (2018). Blocking amino acid transporter OsAAP3 improves grain yield by promoting outgrowth buds and increasing tiller number in rice. Plant Biotechnol. J. 16, 1710–1722. doi: 10.1111/pbi.12907
Ma, X., Zhang, Q., Zhu, Q., Liu, W., Chen, Y., Qiu, R., et al. (2015). A robust CRISPR/Cas9 system for convenient, high-efficiency multiplex genome editing in monocot and dicot plants. Mol. Plant 8, 1274–1284. doi: 10.1016/j.molp.2015.04.007
Manolio, T. A., Collins, F. S., Cox, N. J., Goldstein, D. B., Hindorff, L. A., Hunter, D. J., et al. (2009). Finding the missing heritability of complex diseases. Nature 461, 747–753. doi: 10.1038/nature08494
Moreira, G. C. M., Boschiero, C., Cesar, A. S. M., Reecy, J. M., Godoy, T. F., Trevisoli, P. A., et al. (2018). A genome-wide association study reveals novel genomic regions and positional candidate genes for fat deposition in broiler chickens. BMC Genomics 19, 374. doi: 10.1186/s12864-018-4779-6
Péret, B., Swarup, K., Ferguson, A., Seth, M., Yang, Y., Dhondt, S., et al. (2012). AUX/LAX genes encode a family of auxin influx transporters that perform distinct functions during Arabidopsis development. Plant Cell. 24, 2874–2885. doi: 10.1105/tpc.112.097766
Park, S. Y., Scranton, M. A., Stajich, J. E., Yee, A., Walling, L. L. (2017). Chlorophyte aspartyl aminopeptidases: ancient origins, expanded families, new locations, and secondary functions. PloS One 12, e0185492. doi: 10.1371/journal.pone.0185492
Qi, X., Li, S., Zhu, Y., Zhao, Q., Zhu, D., Yu, J. (2017). ZmDof3, a maize endosperm-specific Dof protein gene, regulates starch accumulation and aleurone development in maize endosperm. Plant Mol. Biol. 93, 7–20. doi: 10.1007/s11103-016-0543-y
Rozas, J., Ferrer-Mata, A., Sánchez-DelBarrio, J. C., Guirao-Rico, S., Librado, P., Ramos-Onsins, S. E., et al. (2017). DnaSP 6: DNA sequence polymorphism analysis of large data sets. Mol. Biol. Evol. 34, 3299–3302. doi: 10.1093/molbev/msx248
Sanchez-Leon, S., Gil-Humanes, J., Ozuna, C. V., Gimenez, M. J., Sousa, C., Voytas, D. F., et al. (2018). Low-gluten, nontransgenic wheat engineered with CRISPR/Cas9. Plant Biotechnol. J. 16, 902–910. doi: 10.1111/pbi.12837
Shan, Q., Wang, Y., Li, J., Zhang, Y., Chen, K., Liang, Z., et al. (2013). Targeted genome modification of crop plants using a CRISPR-Cas system. Nat. Biotechnol. 31, 686–688. doi: 10.1038/nbt.2650
Stephens, M., Smith, N. J., Donnelly, P. (2001). A new statistical method for haplotype reconstruction from population data. Am. J. Hum. Genet. 68, 978–989. doi: 10.1086/319501
Su, J., Li, L., Zhang, C., Wang, C., Gu, L., Wang, H., et al. (2018). Genome-wide association study identified genetic variations and candidate genes for plant architecture component traits in Chinese upland cotton. Theor. Appl. Genet. 131, 1299–1314. doi: 10.1007/s00122-018-3079-5
Wellcome Trust Case Control C (2007). Genome-wide association study of 14,000 cases of seven common diseases and 3,000 shared controls. Nature 447, 661–678. doi: 10.1038/nature05911
Wu, Q., Li, D., Li, D., Liu, X., Zhao, X., Li, X., et al. (2015). Overexpression of OsDof12 affects plant architecture in rice (Oryza sativa L.). Front. Plant Sci. 6, 833. doi: 10.3389/fpls.2015.00833
Wu, Q., Liu, X., Yin, D., Yuan, H., Xie, Q., Zhao, X., et al. (2017). Constitutive expression of OsDof4, encoding a C2-C2 zinc finger transcription factor, confesses its distinct flowering effects under long- and short-day photoperiods in rice (Oryza sativa L.). BMC Plant Biol. 17, 166. doi: 10.1186/s12870-017-1109-0
Xue, W., Xing, Y., Weng, X., Zhao, Y., Tang, W., Wang, L., et al. (2008). Natural variation in Ghd7 is an important regulator of heading date and yield potential in rice. Nat. Genet. 40, 761–767. doi: 10.1038/ng.143
Yamagishi, M., Takeuchi, Y., Kono, I., Yano, M. (2002). QTL analysis for panicle characteristics in temperate japonica rice. Euphytica 128, 219–224. doi: 10.1023/A:1020893731249
Yamaguchi, T., Nagasawa, N., Kawasaki, S., Matsuoka, M., Nagato, Y., Hirano, H.-Y. (2004). The YABBY gene DROOPING LEAF regulates carpel specification and midrib development in Oryza sativa. Plant Cell 16, 500–509. doi: 10.1105/tpc.018044
Yan, W. H., Wang, P., Chen, H. X., Zhou, H. J., Li, Q. P., Wang, C. R., et al. (2011). A major QTL, Ghd8, plays pleiotropic roles in regulating grain productivity, plant height, and heading date in rice. Mol. Plant 4, 319–330. doi: 10.1093/mp/ssq070
Yan, W., Liu, H., Zhou, X., Li, Q., Zhang, J., Lu, L., et al. (2013). Natural variation in Ghd7.1 plays an important role in grain yield and adaptation in rice. Cell Res. 23, 969–971. doi: 10.1038/cr.2013.43
Yanagisawa, S., Izui, K. (1993). Molecular cloning of two DNA-binding proteins of maize that are structurally different but interact with the same sequence motif. J. Biol. Chem. 268, 16028–16036.
Yang, J., Bakshi, A., Zhu, Z., Hemani, G., Vinkhuyzen, A. A., Lee, S. H., et al. (2015). Genetic variance estimation with imputed variants finds negligible missing heritability for human height and body mass index. Nat. Genet. 47, 1114–1120. doi: 10.1038/ng.3390
Yano, K., Yamamoto, E., Aya, K., Takeuchi, H., Lo, P.-c., Hu, L., et al. (2016). Genome-wide association study using whole-genome sequencing rapidly identifies new genes influencing agronomic traits in rice. Nat. Genet. 48, 927–934. doi: 10.1038/ng.3596
Yin, K., Gao, C., Qiu, J. L. (2017). Progress and prospects in plant genome editing. Nat. Plants 3, 17107. doi: 10.1038/nplants.2017.107
Zhang, J., Zhou, X., Yan, W., Zhang, Z., Lu, L., Han, Z., et al. (2015a). Combinations of the Ghd7, Ghd8 and Hd1 genes largely define the ecogeographical adaptation and yield potential of cultivated rice. New Phytol. 208, 1056–1066. doi: 10.1111/nph.13538
Zhang, L., Li, Q., Dong, H., He, Q., Liang, L., Tan, C., et al. (2015b). Three CCT domain-containing genes were identified to regulate heading date by candidate gene-based association mapping and transformation in rice. Sci. Rep. 5, 7663. doi: 10.1038/srep07663
Zhang, Z., Mao, Y., Ha, S., Liu, W., Botella, J. R., Zhu, J.-K. (2016). A multiplex CRISPR/Cas9 platform for fast and efficient editing of multiple genes in Arabidopsis. Plant Cell Rep. 35, 1519–1533. doi: 10.1007/s00299-015-1900-z
Zhang, H., Cao, Y., Shang, C., Li, J., Wang, J., Wu, Z., et al. (2017). Genome-wide characterization of GRAS family genes in Medicago truncatula reveals their evolutionary dynamics and functional diversification. PloS One 12, e0185439. doi: 10.1371/journal.pone.0185439
Keywords: rice, Dof family, heading date, haplotype-based association, knockout mutants, missing heritability
Citation: Huang Y, Han Z, Cheng N, Luo M, Bai X and Xing Y (2020) Minor Effects of 11 Dof Family Genes Contribute to the Missing Heritability of Heading Date in Rice (Oryza sativa L.). Front. Plant Sci. 10:1739. doi: 10.3389/fpls.2019.01739
Received: 03 September 2019; Accepted: 10 December 2019;
Published: 24 January 2020.
Edited by:
Soren K. Rasmussen, University of Copenhagen, DenmarkReviewed by:
Kazuki Matsubara, Institute of Crop Science (NARO), JapanKarim Sorkheh, Shahid Chamran University of Ahvaz, Iran
Copyright © 2020 Huang, Han, Cheng, Luo, Bai and Xing. This is an open-access article distributed under the terms of the Creative Commons Attribution License (CC BY). The use, distribution or reproduction in other forums is permitted, provided the original author(s) and the copyright owner(s) are credited and that the original publication in this journal is cited, in accordance with accepted academic practice. No use, distribution or reproduction is permitted which does not comply with these terms.
*Correspondence: Yongzhong Xing, yzxing@mail.hzau.edu.cn
†These authors have contributed equally to this work