- Graduate School of Life and Environmental Sciences, Osaka Prefecture University, Sakai, Japan
Parasitic plants infect a broad range of plant species including economically important crops. They survive by absorbing water, minerals, and photosynthates from their hosts. To support their way of life, parasitic plants generally establish parasitic organs that allow them to attach to their hosts and to efficiently absorb substances from the vascular system of the host. Here, we summarize the recent progress in understanding the mechanisms underlying the formation of these parasitic organs, focusing on the process depicted in the stem holoparasitic genus, Cuscuta. An attachment structure called “holdfast” on the stem surface is induced by the light and contact stimuli. Concomitantly with holdfast formation, development of an intrusive structure called haustorium initiates in the inner cortex of the Cuscuta stem, and it elongates through apoplastic space of the host tissue. When haustoria reaches to host vascular tissues, they begin to form vascular conductive elements to connect vascular tissue of Cuscuta stem to those of host. Recent studies have shown parasite-host interaction in the interfacial cell wall, and regulation of development of these parasitic structures in molecular level. We also briefly summarize the role of host receptor in the control of compatibility between Cuscuta and hosts, on which occurrence of attachment structure depends, and the role of plant-to-plant transfer of long-distance signals after the establishment of conductive structure.
Introduction
A group of plants called “parasitic plants” have been reported to consist of 4000 or more species, which is equivalent to approximately 1% of flowering plants, and are found all over the world (Nickrent, 2002). In many cases, the host range of a parasitic plant is wide, infesting many plant species including economically important crops (Lanini and Kogan, 2005). Thus, parasitic plants cause serious damage to crop production.
Parasitic plants can be classified into two classes: hemiparasites that retain the ability to perform photosynthesis, and holoparasites that have little or no photosynthetic capability. Consequently, holoparasites need to live a heterotrophic lifestyle by depriving nutrients and water from host plants Heide-Jørgensen, 2008). Parasitic plants belonging to the genus Cuscuta, a member of the family Convolvulaceae, infest a broad range of hosts and have been used as a model for the study of stem parasitic plants. The genus Cuscuta has been reported to consist of more than 150 species (Yuncker, 1932), and belong to the holoparasitic class with degenerated leaves and roots, and, as they do not perform photosynthesis, depend entirely on host plants for nutrients and water. To understand Cuscuta at genetic level and to prevent damage to crop production, the whole genomes of Cuscuta australis (Sun et al., 2018) and C. campestris (Vogel et al., 2018) have been recently sequenced.
After germination, Cuscuta extends a thread-like shoot. During shoot extension, the extending stem performs a swinging movement to increase the probability of contact with the host plant (Tada et al., 1996). It has been reported that Cuscuta perceives volatiles emitted from the host and extends toward it (Runyon et al., 2006). If Cuscuta cannot find a host plant, it will die in about 2 weeks after germination.
After contact with the host, the stem of Cuscuta forms a counterclockwise coil around the stem of the host (Figure 1A). The coiling behavior has been shown to be induced by the cooperative effects of far-red/blue light and tactile stimuli (Lane and Kasperbauer, 1965; Tada et al., 1996; Furuhashi et al., 1997). Effect of far-red light on the coiling of C. japonica was canceled by red light, suggesting the involvement of phytochrome (Furuhashi et al., 1997). Coiling and projection of haustoria of C. japonica can be induced by placing the stem between two glass plates to apply contact pressure under far-red or blue light, but was not induced under red- or white light, suggesting the cooperative effect of light and tactile stimuli (Tada et al., 1996).
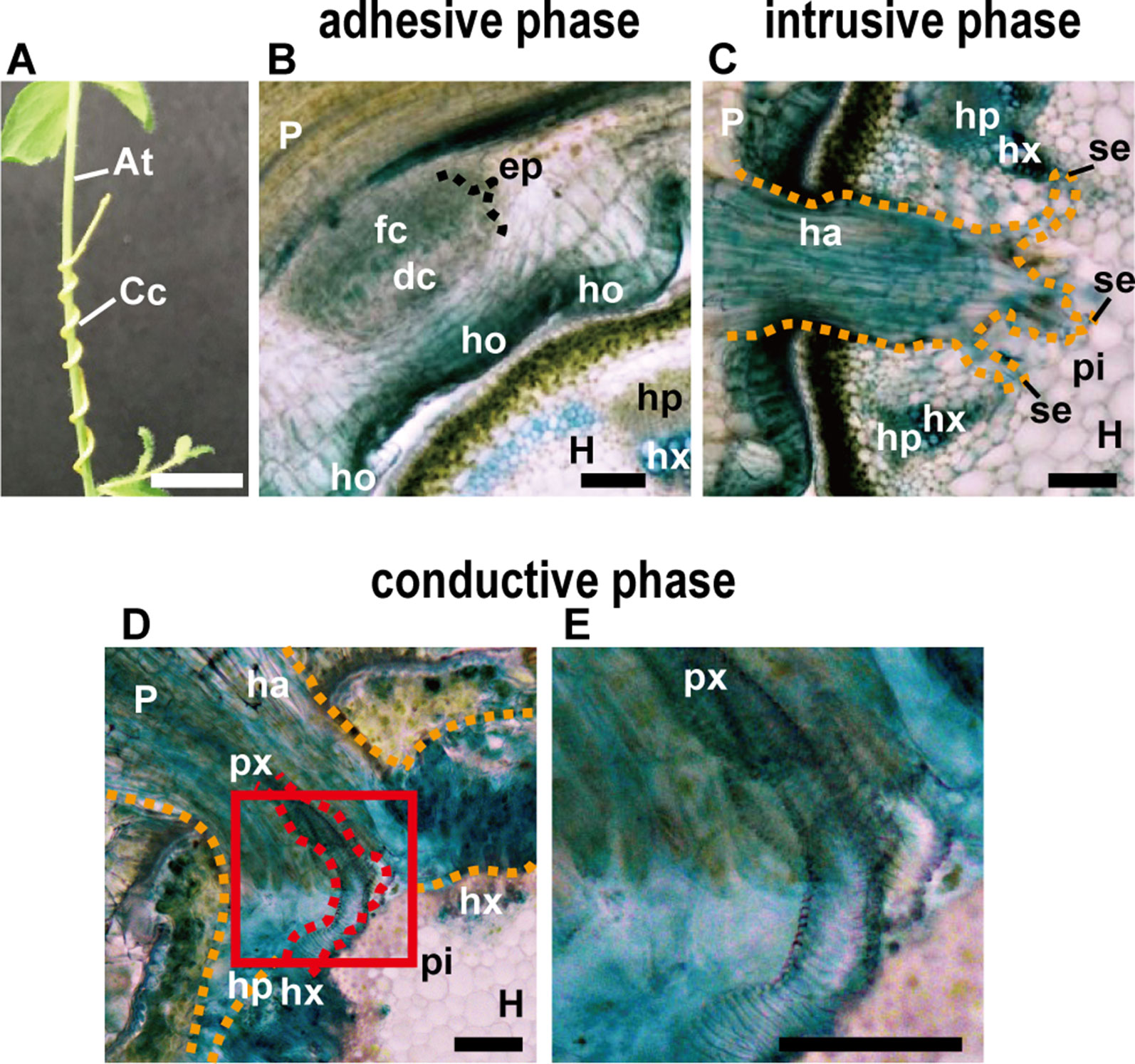
Figure 1 (A) Appearance of parasitic site formed between Cuscuta campestris (Cc) and Arabidopsis thaliana (At) from the outside. C. campestris coils around the inflorescence stem of Arabidopsis. Scale bar, 1 cm. (B–E) Transverse sections of the three phases of parasitic processes of Cuscuta. Scale bars, 200 μm. (B) Adhesive phase. Holdfast (ho) is formed on the host-attaching surface of C. campestris. Prehaustorium develops in the inner cortex of the stem right behind holdfast. In the endophyte primordium (ep), digitate cells (dc) and file cells (fc) differentiate and start to elongate. (C) Intrusive phase. Haustorium (ha) intrudes in the cortex of the host stem. It sometimes reaches to the pith (pi). (D) Conductive phase. (E) Area in the red square in (D) is magnified. Vascular conductive elements (px) are formed in the haustorim. P, parasite; H, host; ha, haustorium; hp, host phloem; hx, host xylem; px, parasite xylem; pi, pith; se, searching hypha; orange dotted line, outline of haustorium; red dotted line, outline of parasite xylem. In all panels, 200-μm-thick micro-slicer sections were stained with toluidine blue.
After coiling on the host stem, a series of organogenesis occurs to establish a parasitic connection, including formation of an adhesive disc-like organ, referred to as a “holdfast” on the surface of the Cuscuta stem in contact with the host stem, and the development of a “haustorium” that intrudes into the host stem and finally makes vascular connection to the xylem vessels and phloem sieve tubes of the host (Yoshida et al., 2016). In this review, we describe the mechanisms underlying the formation of these parasitic organs, and propose hypotheses for the involvement of putative host factors. Comparison of Cuscuta with other well-studied root parasites belonging to Orobanchaceae that are taxonomically distant from Cuscuta highlight diversity with respect to the structure and function of the parasitic organs. We also briefly summarize the role of host receptor in the control of compatibility between Cuscuta and hosts, and the role of plant-to-plant transfer of long-distance signals after the establishment of conductive structure.
Organogenesis Associated With Parasitic Connection
The parasitic processes of Cuscuta can be classified into three phases; the adhesive, intrusive, and conductive phases (Figures 1A–E) (Heide-Jørgensen, 2008). In the adhesive phase, a specialized adhesive organ called the holdfast is formed in the Cuscuta stem in contact with the stem of the host plant. Holdfast is formed essentially by the elongation of cells in the epidermal and cortical layers of Cuscuta stem, and characterized by the presence of secretory cells that secrete adhesive compounds (Heide-Jørgensen, 2008). In the intrusive phase, Cuscuta develops a specialized intrusive organ called the haustorium. When the haustorium reaches the vascular tissues of the host, a specific group of haustorial cells differentiate into vascular conductive cells and Cuscuta proceeds into the conductive phase. In the conductive phase, Cuscuta exchanges various information molecules with the host, as well as absorbs water and nutrients.
Adhesive Phase
After coiling (Figure 1A), epidermal cells of the Cuscuta stem in contact with the host elongate toward the contacting surface of the host epidermis and divide anticlinally to become digitate in form (Figure 1B; Vaughn, 2002). Tight adhesion between Cuscuta and the host can be achieved by secretion of adhesive substances and elongation of cells toward the host surface. The divided epidermal cells of Cuscuta campestris (synonymous with Cuscuta pentagona, Costea et al., 2015) secrete pectin-rich adhesive (cement) to make a tight adhesion (Vaughn, 2002). Homogalacturonan, which constitutes up to 65% of cell wall pectin, is synthesized in a methyl-esterified form (Ridley et al., 2001). Methyl esters are removed enzymatically by pectin methylesterases (PMEs) from homogalacturonan (Micheli, 2001; Pelloux et al., 2007). Several studies using Arabidopsis have shown that low-esterified pectin is responsible for the organ adhesion (Sieber et al., 2000; Sala et al., 2019). In the epidermal layer of Cuscuta holdfast, immunolabeling of cell wall using antibodies against low-esterified homogalacturonan, such as JIM5 and LM19, is relatively stronger than that using antibodies against high-esterified homogalacturonan, such as JIM7 and LM20 (Vaughn, 2002; Johnsen et al., 2015; Hozumi et al., 2017). These result suggested that low-esterified homogalacturonan is responsible for the adhesion of Cuscuta to the hosts (Figure 2A).
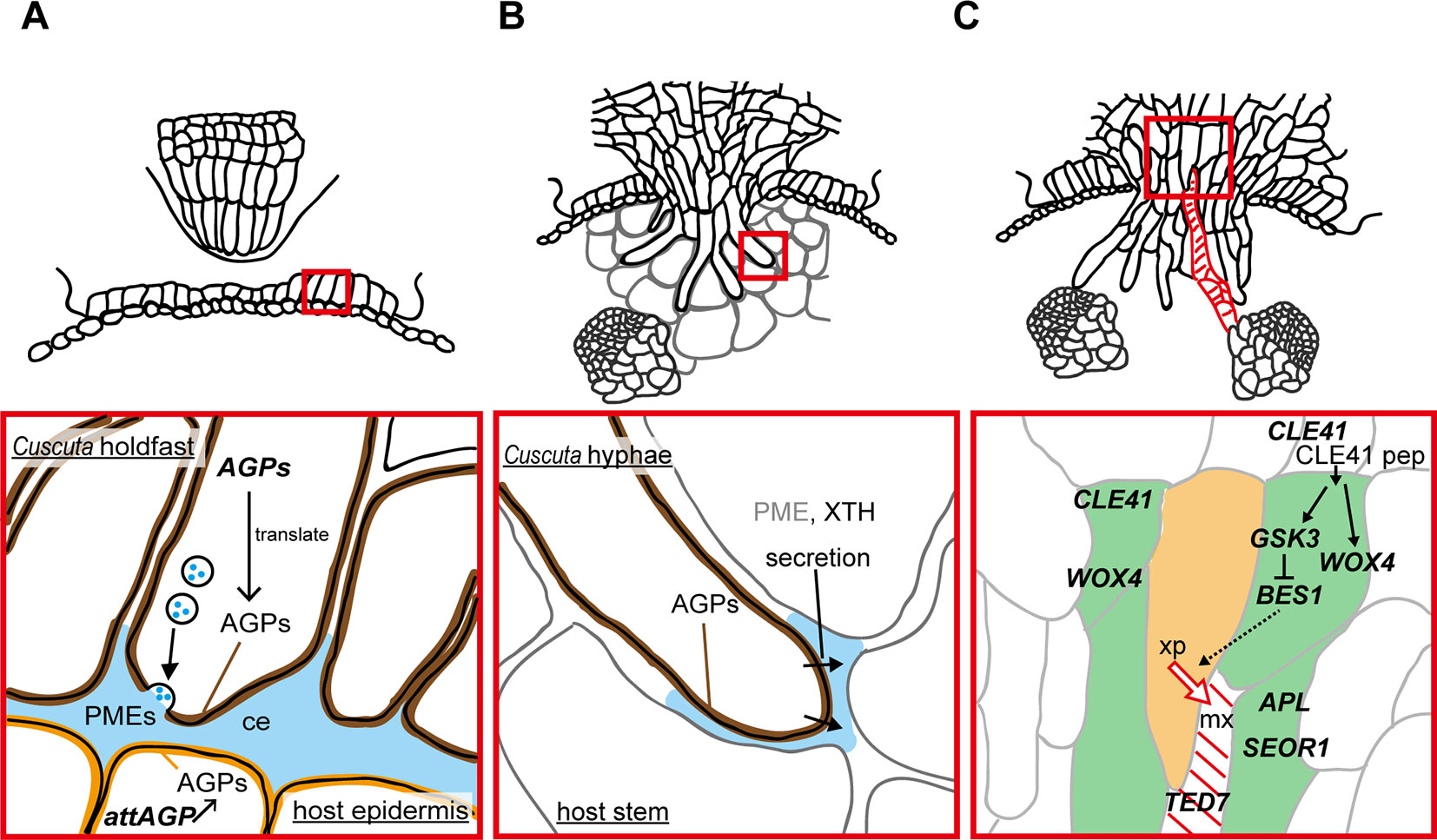
Figure 2 Functions of enzymes and genes associated with the parasitic processes. Panels in the bottom show magnified views of the areas in red squares in panels on the top. (A) Putative function of cell wall-modifying enzymes secreted from holdfast in the adhesive phase. Holdfast cells tighten the adhesion by pectin-rich cement (ce, blue). It has been shown that holdfast cells of Cuscuta campestris contain numerous secretion vesicles containing the components of cell-wall-loosening complexes. Pectin methylesterases (PMEs) are probably secreted to tighten the adhesion of Cuscuta to host. Specific members of genes encoding arabinogalactan proteins (AGPs) are expressed in searching hyphae, and accumulate AGP proteins (brown). AGP also have roles in host cell surface (orange) in the adhesion of parasite (Albert et al., 2006). (B) Secretion of cell wall-modifying enzymes to the cell walls adjacent to searching hyphae in the intrusive phase. Xyloglucan endotransglucosylation (XET) activity of XTH was detected in interface (blue) at the tip of haustoria of C. reflexa (Olsen and Krause, 2017). In C. campestris, searching hyphae-specific expression of FASCICLIN-LIKE genes causes the accumulation of AGPs in the interfacial cell walls surrounding searching hypha cells (brown) (Hozumi et al., 2017) Exact role of AGPs in the intrusive phase is still unknown. (C) Expression of genes associated with differentiation of vascular elements during the transition from intrusive phase to conductive phase in haustorim of Cuscuta japonica. Green, procambium/phloem region, orange, xylem precursor (xp), red diagonal lines, mature xylem vessel (mx). WOX4, WUSCHEL RELATED HOMEOBOX 4; CLE41, CLAVATA3/EMBRYO SURROUNDING REGION-RELATED 41; GSK3, GLYCOGEN SYNTHASE KINASE 3; BES1, BRI1-EMS-SUPPRESSOR 1; TED7, TRACHEARY ELEMENT DIFFERENTIATION-RELATED 7; APL, ALTERED PHLOEM DEVELOPMENT; SEOR1, SIEVE ELEMENT OCCLUSION-RELATED 1.
Arabinogalactan proteins (AGPs) have been reported to be found in common in many adhesion-based mechanisms (Bowling and Vaughn, 2008; Huang et al., 2016). Implication for the involvement of AGPs in Cuscuta adhesion to the host was obtained by accumulation of AGP in the surface of the holdfast (Figure 2A). Staining with LM2 antibody which recognizes carbohydrate moiety of AGPs demonstrate that AGPs accumulate in epidermal cells on the surface of holdfasts of Cuscuta reflexa (Striberny and Krause, 2015) and C. campestris (Hozumi et al., 2017). Staining with Yariv reagents and LM6 antibody further support AGPs accumulation in epidermal cells of holdfasts of C. campestris (Hozumi et al., 2017). Accumulation of AGPs are due to the cell type-specific expression of a subset of fasciclin-like family member genes, CcFLA7, 16 and 17. Accumulation of AGP on the contacting surface was also reported for host plants (Albert et al., 2006; Striberny and Krause, 2015). Contact of Cuscuta reflexa to the surface of tomato stem induces the expression of attAGP in tomato (Albert et al., 2006). Expression levels of tomato attAGP was positively correlated with the force of attachment. This result suggests a positive contribution of AGPs to parasite-host attachment (Albert et al., 2006). However, exact role of AGP in parasite-host attachment is still unknown.
To contact tightly to the host surface, divided epidermal cells of holdfast elongate toward the host surface (Figures 1B and 2A). Outgrowth of the epidermal cells of the holdfast contributes to tightening of the adhesion by accommodating the surface of the host plant (Vaughn, 2002). The surface of the holdfast, which was in a pointed fingerlike extension form, becomes flat or rounded (Figure 2A). This malleability of the holdfast epidermis facilitates the formation of tight seal with the host surface (Vaughn, 2002). Identity of the elongating cell was referred to as a secretary trichome which contains a large number of secretary vesicles (Vaughn, 2002). Epidermal cells of the Cuscuta holdfast likely to share common developmental mechanisms with root hair (Ishida et al., 2008) or leaf trichome (Wang et al., 2019), although the expression of marker genes for these types of cells have not been demonstrated yet.
Initiation of Intrusive Phase
The intrusive phase is characterized by the development of a haustorium (Figure 1C). To be accurate, primordia of haustoria have already been initiated in the adhesive phase. When Cuscuta develops holdfasts after contact to the host’s stem, the precursor of mature haustorium, or so-called prehaustorium, is differentiated in the cortex near the vascular cylinders right behind the holdfast (Figure 1B).
Initiation of the haustorium development appears to be a host-independent process. Development of haustoria in Cuscuta species can be induced even when Cuscuta coiled to non-biological object (Tada et al., 1996; Heide-Jørgensen, 2008; Hong et al., 2011). Microscopic studies have shown that meristem cells of haustorium develop simultaneously with the development of holdfast (Lee and Lee, 1989; Lee, 2007; Heide-Jørgensen, 2008). Initiation of haustorium development requires far-red light, and also blue light even though the effect is weaker than far-red light (Furuhashi et al., 1995), and by contact stimuli concomitantly applied with light (Tada et al., 1996; Furuhashi et al., 1997). Red or white light did not induce haustorium, and haustorium induction by far-red light can be cancelled by the following red light, suggesting the involvement of phytochrome in the regulation of haustorium development (Tada et al., 1996; Furuhashi et al., 1997). Cryptochrome is involved in blue light perception (Cashmore et al., 1999), and mechanosensitive ion channels are likely to be involved in the perception of contact stimuli (Hamilton et al., 2015; however, primary receptors for these stimuli have not been identified yet in Cuscuta.
Cytokinin has been reported to induce haustorium of Cuscuta reflexa in the absence of the host (Ramasubramanian et al., 1988), and in the dark (Haidar et al., 1998). These results imply that cytokinin may be a downstream signal of light and contact stimuli.
Genetic networks involved in the initiation of haustorium development have not yet been elucidated. Haustoria of Cuscuta develop as lateral protrusion of parasite stems, thus classified as “lateral haustoria” (Joel, 2013; Yoshida et al., 2016). Mechanisms involved in the formation of lateral organs, such as lateral roots and adventitious roots, have been studied in detail in Arabidopsis (Hu and Xu, 2016; Liu et al., 2018; Ibáñez et al., 2019; Lee et al., 2019), which may serve as a reference model for the initiation of Cuscuta haustorium.
Development of Haustorium
Initial cells of Cuscuta haustorium are formed in the stem inner cortex, which then divide anticlinally and periclinally to give rise meristem cells (Lee and Lee, 1989; Lee, 2007). Meristem cells are then organized in “endophyte primordium” consisting of two cell types; elongate digitate cells and smaller file cells, before intruding into the host (Figure 1B; Lee, 2007). These cells become intrusive and force their ways through the stem cortex cells in front of them, the epidermal layer of its own stem and the epidermal layer and the cortex of the host. Cells in between the meristem and the stele also divide and form tabular cells, which are added to the file cell layer. This morphological observation suggests that the intrusive cells of Cuscuta originate from the cortex. This is different from the case of Phtheirospermum japonicum, a root hemiparasitic plant belonging to Orobancheceae, whose intrusive cells have been shown to originate from the root epidermal cells (Wakatake et al., 2018) (Figures 3A, B). During intrusive growth in the host’s cortex, intrusive cells advance in the apoplastic space by pushing the cells. At the front of haustorial intrusive part, the elongate digitate cells search for the host’s vascular tissues, and, thus are called “searching hyphae” (Figure 1C; Vaughn, 2003).
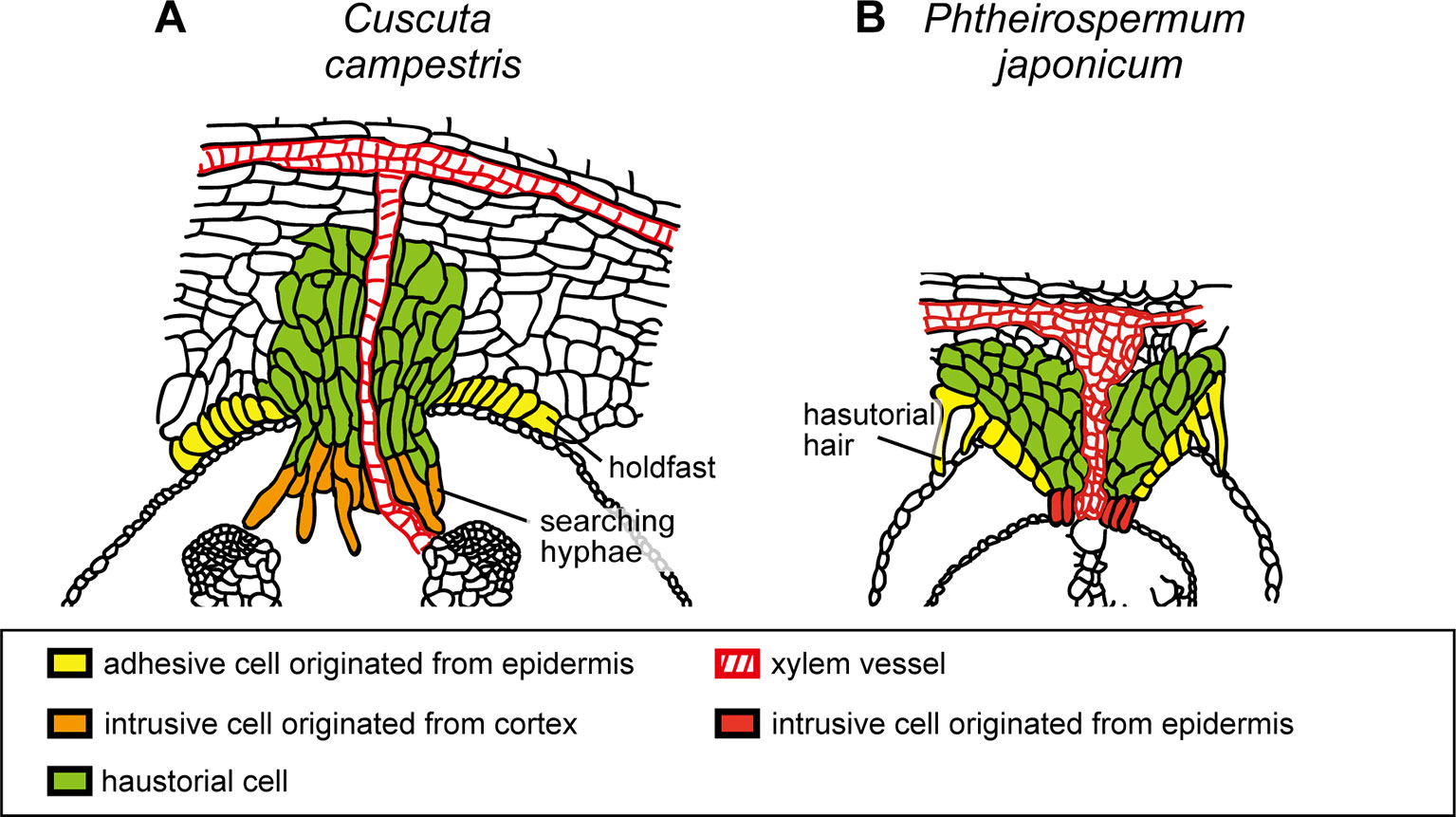
Figure 3 Schematic illustration of the structures of haustoria of Cuscuta campestris and Phtheirospermum japonicum. (A)Cuscuta campestris, a holoparasitic plant belonging to Convolvulaceae, develops lateral haustoria. (B)Phtheirospermum japonicum, a hemiparasitic plant belonging to Orobanchaceae, develops lateral haustoria. Holdfast of C. campestris and haustorial hair of P. japonicum are likely to be analogous that develop from epidermal cells and contribute to the adhesion of parasite to host. Intrusive cells of C. campestris, searching hyphae, develop from digitate cells which have been differentiated from the cortex or endodermal cells of the stem. On the other hand, intrusive cells of P. japonicum are shown to be differentiated from the epidermal cells.
Intrusive cells grow through apoplastic space by pushing host cells aside, rather than by crushing them. Cuscuta secretes enzymes to the interfacial cell walls to loosen the cell wall and aid the elongation of intrusive cells in the apoplastic space. In Cuscuta reflexa, haustorium-specific expression of gene encoding a cysteine protease, namely cuscutain, were reported (Bleischwitz et al., 2010). In the parasitic interface of C. japonica and the host, Glycine max, expression of C. japonica genes encoding cell wall degrading- and modifying- enzymes, such as PME, pectate lyase, polygalacturonase, and xyloglucan endotransglucosylase/hydrolase (XTH) were up-regulated (Ikeue et al., 2015). In the far-red light-induced haustoria of C. reflexa and C. gronovii, two XTH genes have shown to be up-regulated (Olsen et al., 2016b). One of the two enzymatic activities of XTH, xyloglucan endotransglucosylation (XET), were secreted from haustoria, and localized at the host-parasite border of the endophytically growing haustoria of C. reflexa, C. campestris and C. platyloba (Olsen and Krause, 2017). Because XET activity of XTH grafts the reducing end of the cleaved xyloglucan onto an acceptor xyloglucan chain (Rose et al., 2002; Olsen et al., 2016a), these results indicate that Cuscuta XTHs play a role in invading growth of haustoria (Figure 2B).
In addition to cell wall modifying enzymes, searching hyphae of C. campestris and C. japonica, which develop on the haustorial tip, accumulate AGPs in the cell surface (Figure 2B). In C. campestris, hyphal AGP accumulation is accompanied by the expression of hyphae-specific FASCICLIN-LIKE family members (Hozumi et al., 2017). However, roles of hyphal AGP in intrusive growth is still unclear.
Transition From Intrusive Phase to Conductive Phase
Once searching hyphae reach the host’s vascular tissues, the invasion process is almost complete. Searching hyphae acquire identities as xylem- and phloem-conductive elements (Figures 1D, E; Vaughn, 2006; Shimizu et al., 2018), which is concomitantly associated by the differentiation of vascular conducting elements in the center of haustorium (Figure 2C). Cells that have a procambium-attribute, from which vascular elements are differentiated, have emerged before contact with the host’s vascular elements. Cells with a procambium-attribute can be identified by the expression of WUSCHEL RELATED HOMEOBOX 4 (WOX4) (Hirakawa et al., 2010). Expression of C. japonica WOX4, CjWOX4, was detected in the central region of the basal haustorium, and in cells surrounding the precursor cells which later differentiate into xylem vessels (Figure 2C; Shimizu et al., 2018).
Differentiation of searching hyphae into xylem starts near the tip. Searching hyphae penetrate into host xylem vessels through the pits, and starts a series of changes to differentiate xylem vessels (Vaughn, 2006). Xylem differentiation in haustoria of C. japonica include many processes in common with those elucidated in vascular tissues of model plants (Ito et al., 2006; Hirakawa et al., 2008). Before the onset of xylem differentiation, high expression of C. japonica CLAVATA3/EMBRYO SURROUNDING REGION-RELATED 41 (CjCLE41), and CLE41 peptide is likely to be secreted to repress the differentiation of the procambium-like cells into tracheary elements. Expression of CjCLE41 begins to decrease upon the onset of xylem differentiation, which probably down-regulates the kinase activity of GLYCOGEN SYNTHASE KINASE 3 (GSK3) protein. Down-regulation of GSK3 releases the expression of BRI1-EMS-SUPPRESSOR 1 (CjBES1) from the deactivated state. Consequently, activated CjBES1 expression induces the xylem differentiation processes (Shimizu et al., 2018). Expression of the gene specific to developing xylem vessels, TRACHEARY ELEMENT DIFFERENTIATION-RELATED 7 (CjTED7), is under the detection limit before the onset of xylem differentiation, whereas up-regulated with xylem vessel formation (Shimizu et al., 2018).
Compared to xylem, differentiation of phloem in haustoria has been rather controversial and appears to differ from species to species. In C. japonica, marker genes of phloem companion cell, ALTERED PHLOEM DEVELOPMENT (CjAPL; Bonke et al., 2003), and of developing sieve elements, SIEVE ELEMENT OCCLUSION-RELATED 1 (CjSEOR1; Knoblauch et al., 2014), were detected in the intruding haustoria (Figure 2C; Shimizu et al., 2018). Substances from the host’s sieve tube to Cuscuta translocate in distinct arrays of conductive cells (Birschwilks et al., 2006; Shimizu et al., 2018), indicating that phloem conductive cells develop in haustoria and are symplastically separated from surrounding cells. However, in situ hybridization for CjCLE41, whose Arabidopsis ortholog was expressed in phloem cells and adjacent pericycles (Hirakawa et al., 2008), demonstrated that it is expressed in cells overlapping with the region where CjWOX4 is expressed (Shimizu et al., 2018). This incomplete compartmentalization implies immaturity of haustorial phloem relative to that in the conventional vascular bundles.
Differentiation processes of vascular cells in Cuscuta haustoria contain common and different processes compared to those in other parasitic plants. In Phtheirospermum japonicum, expression of procambium-specific genes, PjWOX4, HOMEOBOX PROTEIN 8 (PjHB8) and PjHB1, were detected before the formation of xylem vessels, (Wakatake et al., 2018). This demonstrates that the development of procambium-like cells precedes the differentiation of haustorial vascular cells, as seen in C. japonica. On the other hand, organization of haustorial vascular cells appears to be different from that of C. japonica. Although the presence of xylem vessels are apparent, absence of AtAPL promoter activity, which is expressed in phloem, in haustoria suggest that phloem does not develops in P. japonicum haustoria (Spallek et al., 2017; Wakatake et al., 2018). Similarly, immaturity of the phloem is also shown in the haustoria of root holoparasitic plant, Phelipanche aegyptiaca, which also belongs to Orobanchaceae (Ekawa and Aoki, 2017). On the other hand, formation of mature sieve elements in haustoria has been reported for Orobanche crenata and O. cumana (Dörr and Kollmann, 1995; Krupp et al., 2019). These results, together with haustorial development of Cuscuta described in this section, suggest that development of procambium-like cells and haustorial xylem vessels are observed in common, on the other hand, development of phloem is different between different parasitic plants species. Mechanisms that bring diversity to phloem development have not been elucidated yet.
Conductive Phase
Cuscuta becomes a strong sink after the establishment of the haustorial bridge and competes with sink organs of the host itself for assimilates. Searching hypha cells that contact to host xylem vessels invade vessels through the pits in the cell wall (Heide-Jørgensen, 2008). Then, the ends of hypha cell wall become thin and perforated, finally forms an open connection with host xylem vessels (Figure 4A). Vaughn (2006) mentioned that the nature of the opening between host xylem and hyphal xylem appears to be dependent on the angle and orientation of hyphae with respect to host xylem. The open connection allows the translocation of xylem-mobile dyes, for example, fluorescently labeled 10-kDa dextran (Figure 4B).
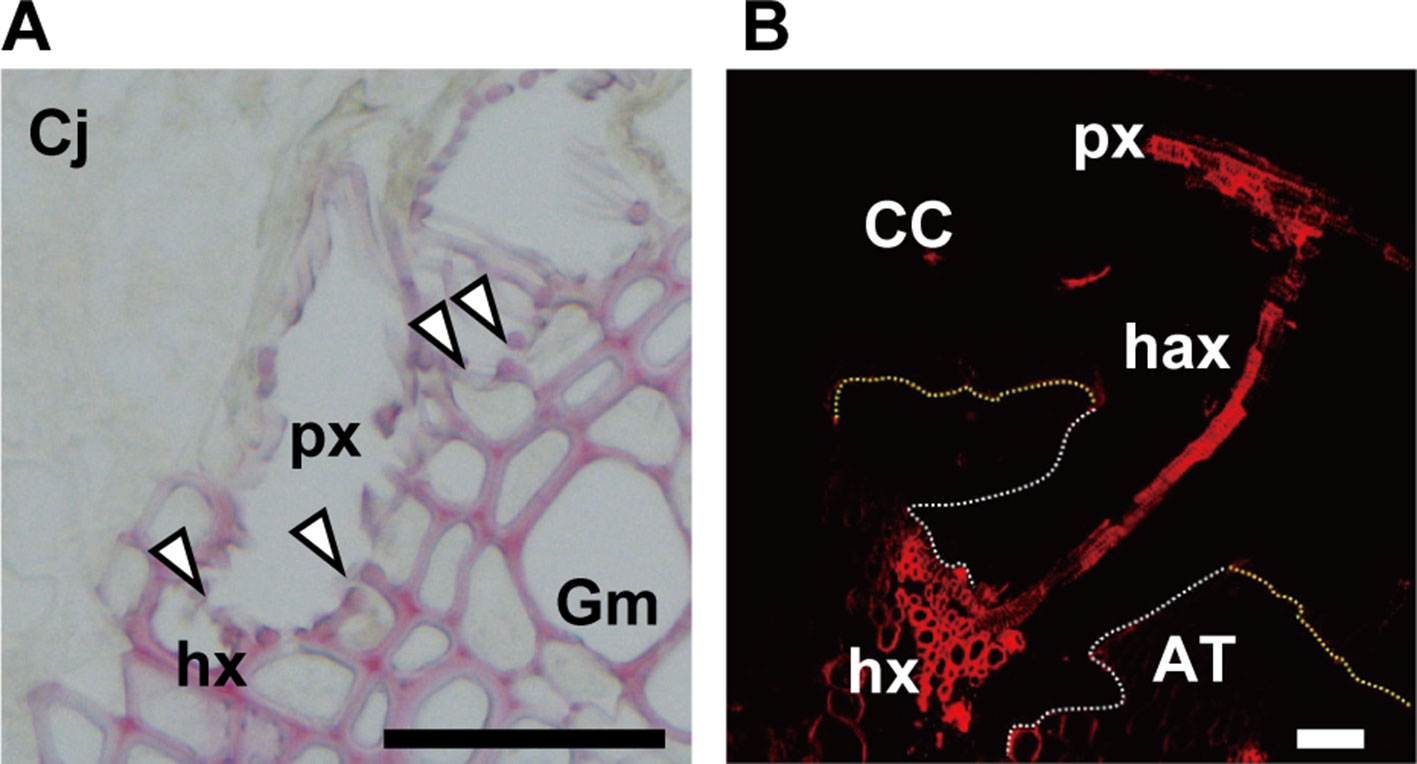
Figure 4 (A) Open connection (arrowheads) between xylem vessels of parasite (px) and host (hx) in the parasitic interface of Cuscuta japonica (Cj) with Glycine max (Gm). Scale bar, 50 μm. A 20-μm-thick paraffin-embedded section was stained with phloroglucinol. (B) Transfer of 5-carboxytetramethylrhodamine (TMR) 10-kDa dextran (red) from host xylem vessel to haustorial xylem vessels, and then to Cuscuta stem xylem. White dotted line; outline of haustorium, yellow dotted line; outline of attachment boundary between Cuscuta and host Arabidopsis. CC, Cuscuta campestris; AT, Arabidopsis thaliana; px, parasite xylem vessel; hax, haustorial xylem vessel; hx, host xylem vessel. Scale bar, 200 μm.
The nature of phloem connection has been controversial. Ultrastructural studies demonstrated that phloem continuity is achieved by a contacting searching hyphae which split in finger-formed elongation, and the plasmodesmata and sieve pores are absent between the searching hypha and host sieve tube, suggesting an apoplastic transfer of xylem solutes via transfer-type cells (Heide-Jørgensen, 2008). On the other hand, Cuscuta has been known as a vector for transmission of virus and phytoplasma (Hosford, 1967; Heintz, 1989), and the transport requires a symplastic connection. Finally, evidence for the presence of symplastic connection was given by the translocation of GFP from sieve tubes of hosts to Cuscuta (Haupt et al., 2001). Various phloem-mobile compounds, including sucrose, amino acids, plant hormones, and xenobiotics have been shown to translocate from the host to parasite (Birschwilks et al., 2006). The transport rate does not show any selectivity with respect to the compounds, suggesting that phloem-mobile compounds are transported through an open symplastic connection.
Flow of water from hosts via xylem to Cuscuta is probably driven by the gradient of water potential between the host and the parasite. In Orobanchaceae, Orobanche cernua accumulates a higher level of potassium than the host (Hibberd et al., 1999), and Striga hermonthica and Phelipanche ramosa accumulates mannitol (Robert et al., 1999). On the other hand, the direction of transport via phloem can occur from the parasite to host, and, thus, is bi-directional. The bi-directional nature of phloem transport lays foundations for mutual control between the parasite and the host.
Interaction With Host
Host Receptor for Immune Response Against Cuscuta
Cuscuta spp. have a broad host range, but there are a few plants that are resistant to Cuscuta (Kaiser et al., 2015). Interestingly, cultivated tomato species, Solanum lycopersicum, is resistant to Cuscuta reflexa (Ihl et al., 1988; Albert et al., 2004; Runyon et al., 2010; Kaiser et al., 2015), while a wild relative of tomato, Solanum pennellii, is susceptible (Hegenauer et al., 2016; Krause et al., 2018). At the end of the attachment phase, epidermal cells of resistant S. lycopersicum die following a hypersensitive-type response, and hypodermal cells are modified to protect intrusion from haustoria (Ihl et al., 1988). Cuscuta factor (CuF), a 2-kDa peptide with O-esterified modification, was identified to trigger defense response of the host plant including production of reactive oxygen species and ethylene (Hegenauer et al., 2016). Analysis of introgression lines of S. lycopersicum × S. pennellii (Eshed and Zamir, 1995) lead to the identification of a gene for tomato receptor of CuF, CuRe1, which encodes a leucine-rich repeat receptor like protein (LRR-RLP) (S. lycopersicum allele, Solyc08g016270) (Hegenauer et al., 2016). Stable introduction of S. lycopersicum CuRe1 into susceptible S. pennellii, and N. benthamiana confers responsiveness to the CuF and increased resistance to C. reflexa (Hegenauer et al., 2016). These results suggest that defense response, likely pattern-triggered immunity (PTI) response, of incompatible tomato species could be induced by the perception of the CuF by the receptor CuRe1, although either the molecular identity of CuF or direct binding of CuF to CuRe1 have not been demonstrated yet (Hegenauer et al., 2016). The presence of additional CuRe1-like receptors is also suggested, and the identification of their ligand will pave the way to investigate parasite-host recognition and its relation to plant immunity (Fürst et al., 2016).
Involvement of Host Factors for Parasitic Organ Development
Host-derived signal substances, or “host factors,” control the organ development processes of parasites. A well-known example of the host factors are strigolactones, that are exuded from host root, that trigger germination of seeds of Orobancaceae plants (for reviews, see Xie et al., 2010; Lumba et al., 2017). In the case of Cuscuta, volatiles emitted from the host is known to mediate host location by Cuscuta (Runyon et al., 2006). On the other hand, haustoria can be induced in a host-independent manner (Furuhashi et al., 1995; Tada et al., 1996). Although haustorium initiation can occur host independently, the latter steps, such as elongation of searching hyphae and their differentiation to conductive cells, may require host factors (Figure 5).
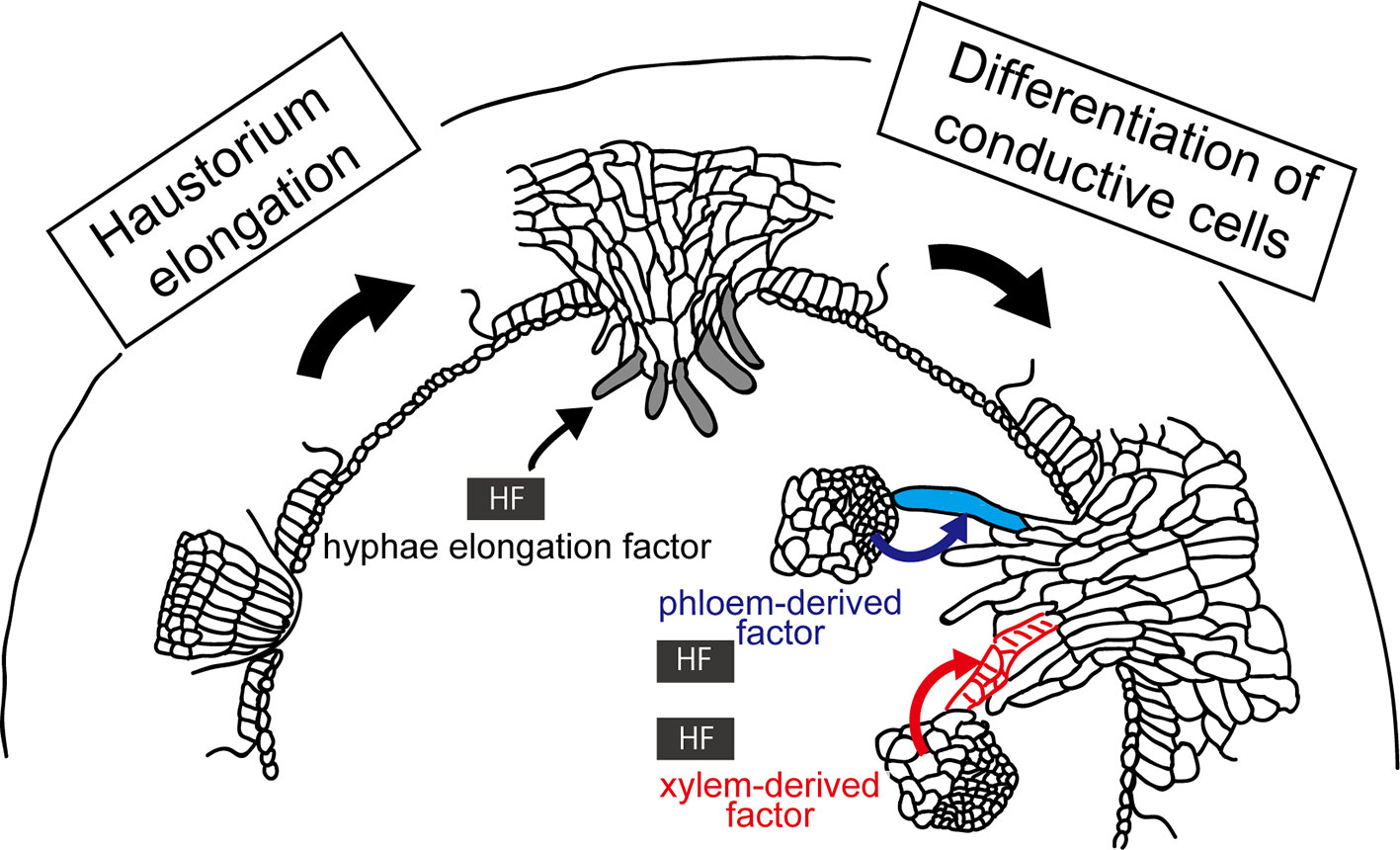
Figure 5 Involvement of host factors (HFs) in the elongation and differentiation of searching hyphae. (Left) HF inducing elongation of searching hyphae (left) has been hypothesized because digitate cells or file cells of C. campestris initiated in a host-independent manner do not show further development of searching hyphae without host. (Right) HF has been implied in the differentiation of searching hypha cells into xylem (red lines) and phloem conductive elements (blue), because upon contacting xylem vessels or phloem sieve tubes of the host, the hyphal cells starts to differentiate into respective conductive elements. These HFs have not been identified yet.
First, elongation of searching hyphae should be initiated by host factors. The rationale for this is that, although elongation of endophyte primodium of Cuscuta initiated by attaching to non-biological substances, such as acryl rod and bamboo stick, develops file cells and digitate cells, they do not show further elongation or development of searching haypha cells (Heide-Jørgensen, 2008; Hong et al., 2011). Host factors involved in this elongation process have not yet been identified.
Second, host factors may be involved in the differentiation of searching hypha cells into xylem and phloem conductive elements (Vaughn, 2006; Krupp et al., 2019). Upon contacting xylem vessels or phloem sieve tubes of the host, the hyphal cells of haustorium starts to differentiate into respective conductive elements, implying that hyphal cells recognize the type of host conductive elements they hit in order to differentiate into the correct elements. Although it is not clear whether this process happens in all cases or not, establishing the right connection between right elements must be essential for the survival of parasitic plants. This raises questions; what the cues of hyphal differentiation are, and whether hyphal cells have multipotency or not. Further study is needed to answer these questions.
We mention that host factors inducing haustorium, or “haustorium inducing factors (HIFs)” are well characterized for Orobancaceae root parasitic plants. Cytokinin (Goyet et al., 2017), 2,6-dimethoxy-1,4-benzoquinone (DMBQ) (Lynn et al., 1981) and lignin-related compounds (Cui et al., 2018) have been shown to have HIF activity. Orobancaceae root parasitic plants may also require host factor(s) for the elongation of intrusive cells because the elongation does not happen when prehaustorium is induced solely by HIFs (Estabrook and Yoder, 1998).
Parasitic Plants Modulate Organs of the Host?
We so far focused on the organ formation in parasitic plants. On the contrary, modulation of organ morphology by the parasite also occurs in the host plants. Parasitization often causes the swelling of host tissues, which is called “hypertrophy” (Heide-Jørgensen, 2008). In the recent study on the parasitic complex of Phtheirospermum japonicum and the host Arabidopsis, thickening of Arabidopsis roots is reported to be induced by the cytokinin produced in P. japonicum (Spallek et al., 2017).
In the host plants parasitized by Cuscuta, induction of new vascular elements in the host was previously reported (Dawson et al., 1994). However, in Impatiens balsaminea parasitized by Cuscuta pentagona, little or no new growth of host vascular elements were observed (Vaughn, 2006). In Glycine max parasitized by Cuscuta japonica, changes in the expression levels were observed for genes responsible for vascular development and cell proliferation, although apparent increase of cell number was not observed in the area adjacent to the invading haustoria (Ikeue et al., 2015). Further study needs to clarify whether invasion of Cuscuta affects the morphology of host organ or not.
Transfer of Long-Distance Signals After Conductive Phase
RNA Movement
Translocation of mRNAs and small RNAs between Cuscuta and the host plant have been shown (Alakonya et al., 2012; LeBlanc et al., 2013) and selectivity of the mobility or uptake of RNA has also been suggested (LeBlanc et al., 2013). A recent study using high-throughput RNA sequencing technology revealed that mRNAs representing more than 8000 genes of the parasite Cuscuta campestris and those representing more than 9000 genes of the host Arabidopsis move to the parasitic partner (Kim et al., 2014). Although an unexpectedly large number of RNAs were shown to move from plant to plant, biological relevance and necessity of the movement of mRNAs in the establishment of parasitic relationship are still unclear.
Trans-species movement of small RNAs (sRNA) has been documented for artificially induced short interfering RNA. Trans-silencing of a target gene was employed to demonstrate the role of SHOOT MERISTEMLESS-LIKE 1 in haustorium development in C. campestris (Alakonya et al., 2012). Recently, induction of microRNA (miRNA) was demonstrated in C. campestris in the parasitic interface with the host Arabidopsis (Shahid et al., 2018). The miRNAs target transcripts encoding defense-related proteins, such as AtSEOR1, BOTRYTIS-INDUCED KINASE 1 (AtBIK1), and members of the TRANSPORT INHIBITOR RESPONSE 1 (AtTIR1)/AUXIN SIGNALING F-BOX 2 and 3 (AtAFB2/AtAFB3) family, and accumulation of these transcripts were reduced during parasitization. Although direct evidence for the enhancement of vigor of the parasite has not yet been obtained, the biomass of C. campestris on Arabidopsis loss-of-function mutants, seor1 and afb3-4, increased, suggesting that repression of these defense-related genes by miRNAs from the parasite may have biological significance (Shahid et al., 2018).
Trans-species movement of mRNA likely recruit the mechanisms for long-distance movement via phloem. Although experimental mRNA mobility can be explained by abundance and half-life of transcripts (Calderwood et al., 2016), presence of sequence motifs such as tRNA-like motifs have been reported selective long-distance movement of mRNA through graft union (Thieme et al., 2015; Zhang et al., 2016). It will be of interest whether the same motifs are functional in the trans-specific movement or not. The mechanisms involved in sRNA transfer needs to be elucidated as well.
Signals in Response to Herbivory Feeding
Responses to herbivory-feeding in one host plant can transfer to the second host plant connected by the bridging Cuscuta australis (Hettenhausen et al., 2017), indicating the feeding signals transfer from host to parasite on the first host, and the other way round on the second host. Feeding by green pea aphid, Myzus persicae, induces a local response to C. australis, and the signal moves to the soybean host and induces the expression of the herbivory response (Zhuang et al., 2018). These results demonstrate that Cuscuta can transmit and receive the systemic signal for herbivory response, although the systemic signal has not been identified yet.
Conclusion and Future Perspectives
Elucidation of cellular and molecular processes involved in the formation of parasitic organs of Cuscuta has unveiled mechanisms hidden in the parasitic interface tissues. Cuscuta probably recruits genetic networks shared by other vascular plants, such as the genetic network for protrusive outgrowth of the epidermal cells and for formation of vascular tissues. They use the set of genes in a non-canonical way, though, as seen in the patterning of procambium, xylem, and phloem cells in haustorium. In addition to the formation of parasitic organs, trans-species trafficking of macromolecules, such as RNAs, through parasitic interface suggests a possibility of bi-directional control of biological processes between host and parasite. Understanding of Cuscuta will suggest parallels with other multi-organism processes, such as grafting, nematode infection, and formation of insect galls (Melnyk and Meyerowitz, 2015; Viera and Gleason, 2019). Comparative analyses of these processes will reveal the fundamental roles of extracellular and intracellular communication in multi-organism complexes.
Author Contributions
KS and KA conceived and wrote the manuscript. KS prepared figures. All authors read and approved the final manuscript.
Funding
This work was supported by Grant-in-Aid for Scientific Research, Grant Numbers 18H03950 and 19H00944 to KA.
Conflict of Interest
The authors declare that the research was conducted in the absence of any commercial or financial relationships that could be construed as a potential conflict of interest.
References
Alakonya, A., Kumar, R., Koenig, D., Kimura, S., Townsley, B., Runo, S., et al, (2012). Interspecific RNA interference of SHOOT MERISTEMLESS-like disrupts Cuscuta pentagona plant parasitism. Plant Cell 24, 3153–3166. doi: 10.1105/tpc.112.099994
Albert, M., Werner, M., Proksch, P., Fry, S. C., Kaldenhoff, R. (2004). The cell wall-modifying xyloglucan endotransglycosylase/hydrolase LeXTH1 is expressed during the defence reaction of tomato against the plant parasite Cuscuta reflexa. Plant Biol. 6, 402–407. doi: 10.1055/s-2004-817959
Albert, M., Belastegui-Macadam, X., Kaldenhoff, R. (2006). An attack of the plant parasite Cuscuta reflexa induces the expression of attAGP, an attachment protein of the host tomato. Plant J. 48, 548–556. doi: 10.1111/j.1365-313X.2006.02897.x
Birschwilks, M., Haupt, S., Hofius, D., Neumann, S. (2006). Transfer of phloem-mobile substances from the host plants to the holoparasite Cuscuta sp. J. Exp. Bot. 57, 911–921. doi: 10.1093/jxb/erj076
Bleischwitz, M., Albert, M., Fuchsbauer, H. L., Kaldenhoff, R. (2010). Significance of Cuscutain, a cysteine protease from Cuscuta reflexa, in host-parasite interactions. BMC Plant Biol. 10, 227. doi: 10.1186/1471-2229-10-227
Bonke, M., Thitamadee, S., Mähönen, A. P., Hauser, M. T., Helariutta, Y. (2003). APL regulates vascular tissue identity in Arabidopsis. Nature 426, 181–186. doi: 10.1038/nature02100
Bowling, A. J., Vaughn, K. C. (2008). Structural and immunocytochemical characterization of the adhesive tendril of Virginia creeper (Parthenocissus quinquefolia [L.] Planch.). Protoplasma 232, 153–163. doi: 10.1007/s00709-008-0287-x
Calderwood, A., Kopriva, S., Morris, R. J. (2016). Transcript Abundance Explains mRNA Mobility Data in Arabidopsis thaliana. Plant Cell 28, 610–615. doi: 10.1105/tpc.15.00956
Cashmore, A. R., Jarillo, J. A., Wu, Y. J., Liu, D. (1999). Cryptochromes: blue light receptors for plants and animals. Science 284, 760–765. doi: 10.1126/science.284.5415.760
Costea, M., García, M. A., Baute, K., Stefanović, S. (2015). Entangled evolutionary history of Cuscuta pentagona clade: A story involving hybridization and Darwin in the Galapagos. Taxon 64, 1225–1242. doi: 10.12705/646.7
Cui, S., Wada, S., Tobimatsu, Y., Takeda, Y., Saucet, S. B., Takano, T., et al. (2018). Host lignin composition affects haustorium induction in the parasitic plants Phtheirospermum japonicum and Striga hermonthica. New Phytol. 218, 710–723. doi: 10.1111/nph.15033
Dawson, J. H., Musselman, L. J., Wolswinkel, P., Dörr, I. (1994). Biology and control of Cuscuta. Rev. Weed Sci. 6, 265–317.
Dörr, I. (1997). How Striga parasitizes its host: a TEM and SEM study. Ann. Bot. 79, 463–472. doi: 10.1006/anbo.1996.0385
Dörr, I., Kollmann, R. (1995). Symplasmic sieve element continuity between Orobanche and its host. Plant Biol. 108, 47–55. doi: 10.1111/j.1438-8677.1995.tb00830.x
Ekawa, M., Aoki, K. (2017). Phloem-Conducting Cells in Haustoria of the Root-Parasitic Plant Phelipanche aegyptiaca Retain Nuclei and Are Not Mature Sieve Elements. Plants (Basel) 6, E60. doi: 10.3390/plants6040060
Eshed, Y., Zamir, D. (1995). An introgression line population of Lycopersicon pennellii in the cultivated tomato enables the identification and fine mapping of yield-associated QTL. Genetics 141, 1147–1162.
Estabrook, E. M., Yoder, J. I. (1998). Plant-Plant Communications: Rhizosphere Signaling between Parasitic Angiosperms and Their Hosts. Plant Physiol. 116, 1–7. doi: 10.1104/pp.116.1.1
Fürst, U., Hegenauer, V., Kaiser, B., Körner, M., Welz, M., Albert, M. (2016). Parasitic Cuscuta factor(s) and the detection by tomato initiates plant defense. Commun. Integr. Biol. 9, e1244590. doi: 10.1080/19420889.2016.1244590
Furuhashi, K., Kanno, M., Morita, T. (1995). Photocontrol of parasitism in a parasitic flowering plant, Cuscuta japonica Chois, cultured in vitro. Plant Cell Physiol. 36, 533–536. doi: 10.1093/oxfordjournals.pcp.a078790
Furuhashi, K., Tada, Y., Okamoto, K., Sugai, M., Kubota, M., Watanabe, M. (1997). Phytochrome participation in induction of haustoria in Cuscuta japonica, a holoparasitic flowering plant. Plant Cell Physiol. 38, 935–940. doi: 10.1093/oxfordjournals.pcp.a029254
Goyet, V., Billard, E., Pouvreau, J. B., Lechat, M. M., Pelletier, S., Bahut, M., et al. (2017). Haustorium initiation in the obligate parasitic plant Phelipanche ramosa involves a host-exudated cytokinin signal. J. Exp. Bot. 68, 5539–5552. doi: 10.1093/jxb/erx359
Haidar, M. A., Orr, G. L., Westra, P. (1998). The response of dodder (Cuscuta spp.) seedlings to phytohormones under various light regimes. Ann. Appl. Biol. 132, 331–338. doi: 10.1111/j.1744-7348.1998.tb05208.x
Hamilton, E. S., Schlegel, A. M., Haswell, E. S. (2015). United in diversity: mechanosensitive ion channels in plants. Annu. Rev. Plant Biol. 66, 113–137. doi: 10.1146/annurev-arplant-043014-114700
Haupt, S., Oparka, K. J., Sauer, N., Neumann, S. (2001). Macromolecular trafficking between Nicotiana tabacum and the holoparasite Cuscuta reflexa. J. Exp. Bot. 52, 173–177. doi: 10.1093/jexbot/52.354.173
Heide-Jørgensen, H. (2008). Parasitic flowering plants. Leiden, The Netherlands: Brill. doi: 10.1163/ej.9789004167506.i-438
Heintz, W. (1989). Transmission of a new mycoplasma-like organism (MLO) from Cuscuta odorata (Ruiz & Pav.) to herbaceous plants and attempts to its elimination in the vector. J. Phytopathol. 125, 171–186. doi: 10.1111/j.1439-0434.1989.tb00652.x
Hegenauer, V., Fürst, U., Kaiser, B., Smoker, M., Zipfel, C., Felix, G., et al. (2016). Detection of the plant parasite Cuscuta reflexa by a tomato cell surface receptor. Science 353, 478–481. doi: 10.1126/science.aaf3919
Hettenhausen, C., Li, J., Zhuang, H., Sun, H., Xu, Y., Qi, J., et al. (2017). Stem parasitic plant Cuscuta australis (dodder) transfers herbivory-induced signals among plants. Proc. Natl. Acad. Sci. U S A 114, E6703–E6709. doi: 10.1073/pnas.1704536114
Hibberd, J. M., Quick, W. P., Press, M. C., Scholes, J. D., Jeschke, W. D. (1999). Solute fluxes from tobacco to the parasitic angiosperm Orobanche cernua and the influence of infection on host carbon and nitrogen relations. Plant Cell Environ. 22, 937–947. doi: 10.1046/j.1365-3040.1999.00462.x
Hirakawa, Y., Shinohara, H., Kondo, Y., Inoue, A., Nakanomyo, I., Ogawa, M., et al. (2008). Non-cell-autonomous control of vascular stem cell fate by a CLE peptide/receptor system. Proc. Natl. Acad. Sci. U S A 105, 15208–15213. doi: 10.1073/pnas.0808444105
Hirakawa, Y., Kondo, Y., Fukuda, H. (2010). TDIF Peptide Signaling Regulates Vascular Stem Cell Proliferation via the WOX4 Homeobox Gene in Arabidopsis. Plant Cell 22, 2618–2629. doi: 10.1105/tpc.110.076083
Hong, L., Shen, H., Chen, H., Li, L., Hu, X., Xu, X., et al. (2011). The morphology and anatomy of the haustoria of the holoparasitic angiosperm Cuscuta campestris. Pak. J. Bot. 43, 1853–1859.
Hosford, R. M. Jr. (1967). Transmission of plant viruses by dodder. Botanical Rev. 33, 387–406. doi: 10.1007/BF02858742
Hozumi, A., Bera, S., Fujiwara, D., Obayashi, T., Yokoyama, R., Nishitani, K., et al. (2017). Arabinogalactan Proteins Accumulate in the Cell Walls of Searching Hyphae of the Stem Parasitic Plants, Cuscuta campestris and Cuscuta japonica. Plant Cell Physiol. 58, 1868–1877. doi: 10.1093/pcp/pcx121
Huang, Y., Wang, Y., Tan, L., Sun, L., Petrosino, J., Cui, M. Z., et al. (2016). Nanospherical arabinogalactan proteins are a key component of the high-strength adhesive secreted by English ivy. Proc. Natl. Acad. Sci. U.S.A. 23, E3193–E3202. doi: 10.1073/pnas.1600406113
Hu, Z., Xu, L. (2016). Transcription Factors WOX11/12 Directly Activate WOX5/7 to Promote Root Primordia Initiation and Organogenesis. Plant Physiol. 172, 2363–2373. doi: 10.1104/pp.16.01067
Ibáñez, S., Ruiz-Cano, H., Fernández, M., Sánchez-García, A. B., Villanova, J., Micol, J. L., et al. (2019). A Network-Guided Genetic Approach to Identify Novel Regulators of Adventitious Root Formation in Arabidopsis thaliana. Front. Plant Sci. 10, 461. doi: 10.3389/fpls.2019.00461
Ichihashi, Y., Kusano, M., Kobayashi, M., Suetsugu, K., Yoshida, S., Wakatake, T., et al. (2018). Transcriptomic and metabolomic reprogramming from roots to haustoria in the parasitic plant, Thesium chinense. Plant Cell Physiol. 59, 724–733. doi: 10.1093/pcp/pcx200
Ihl, B., Tutakhil, N., Hagen, A., Jacob, F. (1988). Studies on Cuscuta reflexa Roxb.7. Defense-mechanisms of Lycopersicon esculentum Mill. Flora 181, 383–393.
Ikeue, D., Schudoma, C., Zhang, W., Ogata, Y., Sakamoto, T., Kurata, T., et al. (2015). A bioinformatics approach to distinguish plant parasite and host transcriptomes in interface tissue by classifying RNA-Seq reads. Plant Methods 11, 34. doi: 10.1186/s13007-015-0066-6
Ishida, T., Kurata, T., Okada, K., Wada, T. (2008). A genetic regulatory network in the development of trichomes and root hairs. Annu. Rev. Plant Biol. 59, 365–386. doi: 10.1146/annurev.arplant.59.032607.092949
Ito, Y., Nakanomyo, I., Motose, H., Iwamoto, K., Sawa, S., Dohmae, N., et al. (2006). Dodeca-CLE peptides as suppressors of plant stem cell differentiation. Science 313, 842–845. doi: 10.1126/science.1128436
Joel, D. M. (2013). “Functional structure of the mature haustorium,” in Parasitic Orobanchaceae: Parasitic Mechanisms and Control Strategies. Eds. Joel, D. M., Gressel, J., Musselman, L. J. (Springer-Verlag Berlin Heidelberg), 25–60.
Johnsen, H. R., Striberny, B., Olsen, S., Vidal-Melgosa, S., Fangel, J. U., Willats, W. G. T., et al. (2015). Cell wall composition profiling of parasitic giant dodder (Cuscuta reflexa) and its hosts: a priori differences and induced changes. New Phytol. 207, 805–816. doi: 10.1111/nph.13378
Kaiser, B., Vogg, G., Furst, U. B., Albert, M. (2015). Parasitic plants of the genus Cuscuta and their interaction with susceptible and resistant host plants. Front. Plant Sci. 6, 45. doi: 10.3389/fpls.2015.00045
Kim, G., LeBlanc, M. L., Wafula, E. K., Pamphilis, C. W., Westwood, J. H. (2014). Plant science. Genomic-scale exchange of mRNA between a parasitic plant and its hosts. Science 345, 808–811. doi: 10.1126/science.1253122
Knoblauch, M., Froelich, D. R., Pickard, W. F., Peters, W. S. (2014). SEORious business: Structural proteins in sieve tubes and their involvement in sieve element occlusion. J. Exp. Bot. 65, 1879–1893. doi: 10.1093/jxb/eru071
Krause, K., Johnsen, H. R., Pielach, A., Lund, L., Fischer, K., Rose, J. K. C. (2018). Identification of tomato introgression lines with enhanced susceptibility or resistance to infection by parasitic giant dodder (Cuscuta reflexa). Physiol. Plant 162, 205–218. doi: 10.1111/ppl.12660
Krupp, A., Heller, A., Spring, O. (2019). Development of phloem connection between the parasitic plant Orobanche cumana and its host sunflower. Protoplasma 256, 1385–1397. doi: 10.1007/s00709-019-01393-z.
Lane, H. C., Kasperbauer, M. J. (1965). Photomorphogenic Responses of Dodder Seedlings. Plant Physiol. 40, 109–116. doi: 10.1104/pp.40.1.109
Lanini, W. T., Kogan, M. (2005). Biology and management of Cuscuta in crops. Cienc. Investig. Agrar 32, 127–141. doi: 10.7764/rcia.v32i3.317
LeBlanc, M., Kim, G., Patel, B., Stromberg, V., Westwood, J. (2013). Quantification of tomato and Arabidopsis mobile RNAs trafficking into the parasitic plant Cuscuta pentagona. New Phytol. 200, 1225–1233. doi: 10.1111/nph.12439
Lee, H. W., Cho, C., Pandey, S. K., Park, Y., Kim, M. J., Kim, J. (2019). LBD16 and LBD18 acting downstream of ARF7 and ARF19 are involved in adventitious root formation in Arabidopsis. BMC Plant Biol. 19, 46. doi: 10.1186/s12870-019-1659-4
Lee, K. B. (2007). Structure and development of the upper haustorium in the parasitic flowering plant Cuscuta japonica (Convoluvulaceae). Am. J. Bot. 94, 735–745. doi: 10.3732/ajb.94.5.737
Lee, K. B., Lee, C. D. (1989). The structure and development of the haustorium in Cuscuta australis. Can. J. Bot. 67, 2975–2982. doi: 10.1139/b89-381
Liu, W., Yu, J., Ge, Y., Qin, P., Xu, L. (2018). Pivotal role of LBD16 in root and root-like organ initiation. Cell. Mol. Life Sci. 75, 3329–3338. doi: 10.1007/s00018-018-2861-5
Lumba, S., Bunsick, M., McCourt, P. (2017). Chemical genetics and strigolactone perception. Res. 6, 975. doi: 10.12688/f1000research.11379.1. eCollection 2017.
Lynn, D. G., Steffens, J. C., Kamut, V. S., Graden, D. W., Shabanowitz, J., Riopel, J. L. (1981). Isolation and characterization of the first host recognition substance for parasitic angiosperms. J. Am. Chem. Soc 103, 1868–1870. doi: 10.1021/ja00397a062
Mangeon, A., Bell, E. M., Lin, W. C., Jablonska, B., Springer, P. S. (2011). Misregulation of the LOB domain gene DDA1 suggests possible functions in auxin signalling and photomorphogenesis. J. Exp. Bot. 62, 221–233. doi: 10.1093/jxb/erq259
Melnyk, C. W., Meyerowitz, E. M. (2015). Plant grafting. Curr. Biol. 25, R183–R188. doi: 10.1016/j.cub.2015.01.029
Micheli, F. (2001). Pectin methylesterases: cell wall enzymes with important roles in plant physiology. Trends Plant Sci. 6, 414–419. doi: 10.1016/S1360-1385(01)02045-3
Ndambi, B., Cadisch, G., Elzein, A., Heller, A. (2011). Colonization and control of Striga hermonthica by Fusarium oxysporum f. sp. Strigae, a mycoherbicide component: an anatomical study. Biol. Cont 58, 149–159. doi: 10.1016/j.biocontrol.2011.04.015
Nickrent, D. L. (2002). “Parasitic Plants of the World,” in Parasitic Plants of the Iberian Peninsula and Balearic Islands. Eds. López-Sáez, J. A., Catalán, P., Sáez, L. (Madrid: Mundi-Prensa Libros S.A), 7–27.
Olsen, S., Krause, K. (2017). Activity of xyloglucan endotransglucosylases/hydrolases suggests a role during host invasion by the parasitic plant Cuscuta reflexa. PloS One 12, e0176754. doi: 10.1371/journal.pone.0176754
Olsen, S., Popper, Z. A., Krause, K. (2016a). Two sides of the same coin: Xyloglucan endotransglucosylases/hydrolases in host infection by the parasitic plant Cuscuta. Plant Signal Behav. 11, e1145336. doi: 10.1080/15592324.2016.1145336
Olsen, S., Striberny, B., Hollmann, J., Schwacke, R., Popper, Z., Krause, K. (2016b). Getting ready for host invasion: elevated expression and action of xyloglucan endotransglucosylases/hydrolases in developing haustoria of the holoparasitic angiosperm Cuscuta. J. Exp. Bot. 67, 695–708. doi: 10.1093/jxb/erv482
Pelloux, J., Resterucci, C., Mellerowicz, E. J. (2007). New insights into pectin methylesterase structure and function. Trends Plant Sci. 12, 267–277. doi: 10.1016/j.tplants.2007.04.001
Ramasubramanian, T. S., Paliyath, G., Rajagopal, I., Maheshwari, R., Mahadevan, S. (1988). Hormones and Cuscuta development: In vitro induction of haustoria by cytokinin and its inhibition by other hormones. J. Plant Growth Reg 7, 133–144. doi: 10.1007/BF02024676
Rehker, J., Lachnit, M., Kaldenhoff, R. (2012). Molecular convergence of the parasitic plant species Cuscuta reflexa and Phelipanche aegyptiaca. Planta 236, 557–566. doi: 10.1007/s00425-012-1626-x
Ridley, B. L., O’Neill, M. A., Mohnen, D. (2001). Pectins: structure, biosynthesis, and oligogalacturonide-related signaling. Phytochemistry 57, 926–967. doi: 10.1016/S0031-9422(01)00113-3
Robert, S., Simier, P., Fer, A. (1999). Purification and characterization of mannose 6-phosphate reductase, a potential target for the control of Striga hermonthica and Orobanche ramosa. Aust. J. Pant Physiol. 26, 233–237. doi: 10.1071/pp98138
Rose, J. K. C., Braam, J., Fry, S. C., Nishitani, K. (2002). The XTH family of enzymes involved in xyloglucan endotransglucosylation and endohydrolysis: current perspectives and a new unifying nomenclature. Plant Cell Physiol. 43, 1421–1435. doi: 10.1093/pcp/pcf171
Runyon, J. B., Mescher, M. C., De Moraes, C. M. (2006). Volatile chemical cues guide host location and host selection by parasitic plants. Science 313, 1964–1967. doi: 10.1126/science.1131371
Runyon, J. B., Mescher, M. C., Felton, G. W., De Moraes, C. M. (2010). Parasitism by Cuscuta pentagona sequentially induces JA and SA defence pathways in tomato. Plant Cell Environ. 33, 290–303. doi: 10.1111/j.1365-3040.2009.02082.x
Sala, K., Karcz, J., Rypień, A., Kurczyńska, E. U. (2019). Unmethyl-esterified homogalacturonan and extensins seal Arabidopsis graft union. BMC Plant Biol. 19, 0151. https://doi.org/10.1186/s12870-019-1748-4.
Shahid, S., Kim, G., Johnson, N. R., Wafula, E., Wang, F., Coruh, C., et al. (2018). MicroRNAs from the parasitic plant Cuscuta campestris target host messenger RNAs. Nature 553, 82–85. doi: 10.1038/nature25027
Shimizu, K., Hozumi, A., Aoki, K. (2018). Organization of Vascular Cells in the Haustorium of the Parasitic Flowering Plant Cuscuta japonica. Plant Cell Physiol. 59, 715–723. doi: 10.1093/pcp/pcx197
Sieber, P., Schorderet, M., Ryser, U., Buchala, A., Kolattukudy, P., Métraux, J. P., et al. (2000). Transgenic Arabidopsis Plants Expressing a Fungal Cutinase Show Alterations in the Structure and Properties of the Cuticle and Postgenital Organ Fusions. Plant Cell 12, 721–737. doi: 10.1105/tpc.12.5.721
Spallek, T., Melnyk, C. W., Wakatake, T., Zhang, J., Sakamoto, Y., Kiba, T., et al. (2017). Interspecies hormonal control of host root morphology by parasitic plants. Proc. Natl. Acad. Sci. U S A 114, 5283–5288. doi: 10.1073/pnas.1619078114
Spichal, L. (2012). Cytokinins—recent news and views of evolutionally old molecules. Funct. Plant Biol. 39, 267–284. doi: 10.1071/FP11276
Striberny, B., Krause, K. (2015) Cell wall glycoproteins at interaction sites between parasitic giant dodder (Cuscuta reflexa) and its host Pelargonium zonale. Plant Signal Behav. 10, e1086858. doi: 10.1080/15592324.2015.1086858
Sun, G., Xu, Y., Liu, H., Sun, T., Zhang, J., Hettenhausen, C., et al. (2018). Large-scale gene losses underlie the genome evolution of parasitic plant Cuscuta australis. Nat. Commun. 9, 2683. doi: 10.1038/s41467-018-04721-8
Tada, Y., Sugai, M., Furuhashi, K. (1996). Haustoria of Cuscuta japonica, a Holoparasitic Flowering Plant, Are Induced by the Cooperative Effects of Far-Red Light and Tactile Stimuli. Plant Cell Physiol. 37, 1049–1053. doi: 10.1093/oxfordjournals.pcp.a029052
Thieme, C. J., Rojas-Triana, M., Stecyk, E., Schudoma, C., Zhang, W., Yang, L., et al. (2015). Endogenous Arabidopsis messenger RNAs transported to distant tissues. Nat. Plants 1, 15025. doi: 10.1038/nplants.2015.25
Vaughn, K. C. (2006). Conversion of the searching hyphae of dodder into xylic and phlolic hyphae: A cytochemical and immunocytochemical investigation. Int. J. Plant Sci. 167, 1099–1114. doi: 10.1086/507872
Vaughn, K. C. (2003). Dodder hyphae invade the host: A structural and immunocytochemical characterization. Protoplasma 220, 189–200. doi: 10.1007/s00709-002-0038-3
Vaughn, K. C. (2002). Attachment of the parasitic weed dodder to the host. Protoplasma 219, 227–237. doi: 10.1007/s007090200024
Viera, P., Gleason, C. (2019). Plant-parasitic nematode effectors — insights into their diversity and new tools for their identification. Curr. Opin. Plant Biol. 50, 37–43. doi: 10.1016/j.pbi.2019.02.007
Vogel, A., Schwacke, R., Denton, A. K., Usadel, B., Hollmann, J., Fischer, K., et al. (2018). Footprints of parasitism in the genome of the parasitic flowering plant Cuscuta campestris. Nat. Commun. 9, 2515. doi: 10.1038/s41467-018-04344-z
Wakatake, T., Yoshida, S., Shirasu, K. (2018). Induced cell fate transitions at multiple cell layers configure haustorium development in parasitic plants. Development 145, dev164848. doi: 10.1242/dev.164848
Wang, Z., Yang, Z., Li, F. (2019). Updates on molecular mechanisms in the development of branched trichome in Arabidopsis and non-branched in cotton. Plant Biotechnol. J. 17, 1706–1722. doi: 10.1111/pbi.13167
Xie, X., Yoneyama, K., Yoneyama, K. (2010). The strigolactone story. Annu. Rev. Phytopathol. 48, 93–117. doi: 10.1146/annurev-phyto-073009-114453
Yoshida, S., Cui, S., Ichihashi, Y., Shirasu, K. (2016). The Haustorium, a Specialized Invasive Organ in Parasitic Plants. Annu. Rev. Plant Biol. 67, 643–667. doi: 10.1146/annurev-arplant-043015-111702
Zhang, W., Thieme, C. J., Kollwig, G., Apelt, F., Yang, L., Winter, N., et al. (2016). tRNA-Related Sequences Trigger Systemic mRNA Transport in Plants. Plant Cell 28, 1237–1249. doi: 10.1105/tpc.15.01056
Keywords: attachment cells, conductive cells, Cuscuta, haustorium, host factors, intrusive cells, parasitic organs, parasitic plants
Citation: Shimizu K and Aoki K (2019) Development of Parasitic Organs of a Stem Holoparasitic Plant in Genus Cuscuta. Front. Plant Sci. 10:1435. doi: 10.3389/fpls.2019.01435
Received: 28 June 2019; Accepted: 16 October 2019;
Published: 12 November 2019.
Edited by:
Masa H. Sato, Kyoto Prefectural University, JapanReviewed by:
Satoko Yoshida, Nara Institute of Science and Technology (NAIST), JapanTakashi Ishida, Kumamoto University, Japan
Copyright © 2019 Shimizu and Aoki. This is an open-access article distributed under the terms of the Creative Commons Attribution License (CC BY). The use, distribution or reproduction in other forums is permitted, provided the original author(s) and the copyright owner(s) are credited and that the original publication in this journal is cited, in accordance with accepted academic practice. No use, distribution or reproduction is permitted which does not comply with these terms.
*Correspondence: Koh Aoki, kaoki@plant.osakafu-u.ac.jp