- 1College of Plant Science & Technology, Huazhong Agricultural University, Wuhan, China
- 2College of Agronomy and Biotechnology, China Agricultural University, Beijing, China
- 3Department of Botany and Plant Sciences, University of California, Riverside, CA, United States
Salinity threatens agricultural production systems across the globe. While the major focus of plant researchers working in the field of salinity stress tolerance has always been on sodium and potassium, the transport patterns and physiological roles of Cl− in plant salt stress responses are studied much less. In recent years, the role of Cl− in plant salinity stress tolerance has been revisited and has received more attention. This review attempts to address the gap in knowledge of the role of Cl− transport in plant salinity stress tolerance. Cl− transport, Cl− exclusion, vacuolar Cl− sequestration, the specificity of mechanisms employed in different plant species to control shoot Cl− accumulation, and the identity of channels and transporters involved in Cl− transport in salt stressed plants are discussed. The importance of the electrochemical gradient across the tonoplast, for vacuolar Cl− sequestration, is highlighted. The toxicity of Cl− from CaCl2 is briefly reviewed separately to that of Cl− from NaCl.
Introduction
Soil salinity affects nearly 50% of all irrigated land in the world, and is a major constraint to crop yield (Fita et al., 2015). To meet the projected demand of feeding 9.3 billion people by 2050, global agricultural production must be increased by 60% from its 2005–2007 levels (van Ittersum et al., 2016). Therefore, understanding the mechanisms that underlie plant salt tolerance, especially its ion transport-related traits, is important, since it would allow the breeding of salt tolerant crops and thus mitigate the possible food shortage in the future.
Traditionally, adverse effects of soil salinity have been attributed to with Na+ toxicity, prompting the majority of studies on this topic (Munns and Tester, 2008; Horie et al., 2012; Deinlein et al., 2014; Maathuis, 2014; Wu et al., 2015; Hanin et al., 2016; Wu et al.,, 2018a). However, an increase in Na+ content (Munns and Tester, 2008; Wu, 2018) is always accompanied by Cl– accumulation (Tavakkoli et al., 2010) and K+ loss (Wu et al., 2018b) in plants exposed to salt (NaCl) stress. K+ is the major inorganic nutrient cation in non-halophytes (Dreyer and Uozumi, 2011), and plays important roles in plant cell activities (Anschütz et al., 2014; Shabala and Pottosin, 2014; Wu et al., 2018c) and stress responses (Wang et al., 2013). Cl− is a plant micronutrient and regulates leaf osmotic potential, and turgor, and stimulates growth in plants (Franco-Navarro et al., 2016). However high Cl− solutions are toxic, and impair photosynthesis and growth (Tavakkoli et al., 2010; Tavakkoli et al., 2011). Specific reasons for these detrimental effects are much less understood than those of Na+, but the excessive accumulation of Cl− in chloroplasts is one effect (Seemann and Critchley, 1985; Geilfus, 2018b).
In recent years, the role of Cl− in plant salinity stress tolerance has attracted more attention. The role of Cl− in halophytes was reviewed by (Bazihizina et al., 2019). They suggest that rather than targeting Cl− exclusion, a better way to breed salt tolerant crops would be to improve the selectivity of the broadly selective anion-transporting proteins. Cl− as an essential micronutrient and its beneficial role in plants (Raven, 2017; Wege et al., 2017), the role of Cl− in organelle development (Geilfus, 2018a; Geilfus, 2018b), and control of Cl− transport in plants (Li et al., 2017b) have been recently reviewed. Moreover, it is suggested nowadays that Cl– is a beneficial macronutrient for plants (Franco-Navarro et al., 2016; Franco-Navarro et al., 2019). Unlike the above-mentioned recent reviews, the present mini review is focused on the main traits related to controlling Cl− transport, and its role in plant salt tolerance.
Cl–, a Largely Overlooked Ion in Plant Salt Tolerance
Cl– is an essential micronutrient in plants. In some plant species e.g. soybean, and woody plants such as avocado, Cl– showed more significant toxic effect than Na+ since they are better at excluding Na+ from the leaf blades than Cl– (Munns and Tester, 2008). The toxicity threshold of Cl– is estimated to be 15-50 and 4-7 mg per gram dry weight for Cl– tolerant and sensitive species, respectively (Xu et al., 1999). Besides its roles in photosynthesis and in membrane potential stabilization, Cl– also regulates enzyme activities in the cytoplasm and is involved in turgor and pH regulation (Teakle and Tyerman, 2010). Low Cl– (< 5 mM) resulted in increased leaf area and biomass in tobacco plants (Franco-Navarro et al., 2016), whereas high Cl– (> 120 mM) resulted in decreased biomass production in barley plants (Tavakkoli et al., 2011). Whereas high Cl– (over 4 mg g–1 FW) shows toxicity due to interference with PSII quantum yield and photosynthetic electron transport rate (Tavakkoli et al., 2011; Carillo et al., 2019), Cl− in a range of 0.2–2 mg g–1 FW (fresh weight) can function in the stabilization of the oxygen-evolving complex of photosystem II, in the maintenance of electrical potential in cell membranes, regulation of tonoplast H+-ATPase and enzyme activities (Marschner 1995; Broadly et al., 2012; Carillo et al., 2019). In tobacco, up to 4 mg g–1 FW, Cl– can be important for the maintenance of water homeostasis (Franco-Navarro et al., 2016).
For most crop plants, Na+ is more toxic than Cl–. Salinity stress in some plant species, e.g. soybeans and woody plant species such as citrus, is due to more pronounced Cl− toxicity (White and Broadley 2001). Such species are comparatively effective in excluding Na+, but do not prevent Cl– from accumulating to toxic levels in leaves (Munns and Tester, 2008). It is known that Na+ toxicity is due to the similarity of the hydrated ionic radii of Na+ to that of K+, and is thus in competition with K+ for binding sites on enzymes that depend on K+ for activation. This is clearly not the case of Cl–. Antagonism between Cl– and NO3– can result in a reduction in the uptake and storage of nitrogen (Li et al., 2017b), which is the important source for protein synthesis and many metabolism products. At high concentrations of Cl–, limited nitrogen supply as a result of antagonism between Cl– and NO3– could be a reason for its toxicity in salinized plants. In the presence of high Cl–, a decrease of shoot NO3– to Cl– ratio is correlated with a significant shoot biomass reduction in Arabidopsis, and the overexpression SLAH1 (a homologue of the slow type anion channel SLAC1) (Qiu et al., 2016) (Cubero-Font et al., 2016). Li et al. (2017b) recently proposed that the NO3–/Cl– ratio might be used as a salt tolerance indicator, similar to the K+/Na+ ratio.
CaCl2 Based Cl– Toxicity in Ornamental and Horticultural Plant Species
Besides sodium salts, a wide range of other dissolved salts (e.g. those of Mg2+ and Ca2+ salts) are also present in saline soils (Tavakkoli et al., 2010). When reclaimed water is used for irrigation, (Wang et al., 2017), B3+, Ca2+, Cl–, Na+, and SO42– and their salts may be prevalent (Martinez and Clark, 2012; Nackley et al., 2015). In this context, there is potential for Cl− toxicity to arise from CaCl2 instead of NaCl. Further, Cl− toxicity may arise from the use of Cl− salts, (such as KCl and CaCl2), as fertilizers. For example, to decrease the accumulation of nitrate in leafy vegetables, (to meet EU directives), calcium Cl− is sometimes substituted for calcium nitrate (Carillo et al., 2019). Low level Ca2+ (below 10 mM) is known to ameliorate NaCl induced salinity stress symptoms in many plant species, e.g. Arabidopsis (Shabala et al., 2006), rice (Rahman et al., 2016), wheat (Al-Whaibi et al., 2012), barley (Cramer et al., 1991), and Calligonum mongolicum (Xu et al., 2017), etc. However, under moderate CaCl2 treatment (above 20 mM), greater Cl– toxicity was observed in some ornamental, (e.g. Callistemon citrinus and Viburnum lucidum), and horticultural species, (e.g. Ocimumu basilicum), (Borghesi et al., 2013; Collaa et al., 2013; Borgognone et al., 2014; Carillo et al., 2019; Cirillo1 et al., 2019). These studies showed that CaCl2 salinity is able to induce ion imbalance and hyperosmotic stress even more severe than that of NaCl, strongly reducing plant growth and yield. These results can be partly attributed to the toxic effects of Cl–, (since CaCl2 has twice the Cl− that the same number of moles of NaCl carries), for which the uptake and transport to leaves could be less controlled than that of Na+ (Collaa et al., 2013) in these studied ornamental and horticultural plant species.
Channels and Transporters in Cl– Transport
To date, the most commonly reported gene families for Cl− channels are characterized as slow anion channels (SLAC channels), Cl− channels (CLC), and aluminium activated malate transporters (ALMT transporters) (Skerrett and Tyerman, 1994). Seven CLCs (AtCLCa to AtCLCg, channels or transporters), localized in various intracellular membranes including the tonoplast (AtCLCa, AtCLCb, AtCLCc, and AtCLCg), thylakoid membrane (AtCLCe), and Golgi membrane (AtCLCd and AtCLCf), were found in Arabidopsis (Barbier-Brygoo et al., 2011; Herdean et al., 2016a). Other possible Cl− candidate channels (including the putative one) are mechanosensitive channels (Maksaev and Haswell, 2012; Hamilton et al., 2015), AtVCCN1 (Herdean et al., 2016b), VvNRT1.4, and VvNAXT1 (Henderson et al., 2014). For Cl− transporters, ALMT (aluminium-activated malate transporters) (De Angeli et al., 2013), CCC (cation chloride co-transporter) (Colmenero-Flores et al., 2007), DTX/MATE (detoxification efflux carrier/multidrug and toxic compound extrusion transporters) (Zhang et al., 2017), and AtNPF2 (Li et al., 2017b) are reported to be involved in Cl− transport in salinized plants.
Besides Na+ toxicity, salinity (both Na+ and Cl–) also causes osmotic stress in plants. Cl– influx has been observed in plant cells subjected to salinity (Shabala et al., 2005). Interestingly, hyperosmotic stress also induced net Cl– uptake in bean mesophyll cells (Shabala et al., 2000). This leads to the question of whether the mechanosensitive channels might play a direct or indirect role in Cl– transport in plant cells under salt stress. Falke et al. (1988) identified the first mechanosensitive Cl− channel in tobacco protoplasts. Maksaev and Haswell (2012) found that MSL10 (mechanosensitive channel-like 10) shows a preference for Cl– over Na+ (6:1) and might be involved in Cl– efflux to relieve the membrane tension once the channel opens. MSL8 (Cl– over Na+, 6.3:1) was also found to sense and respond to changes in Arabidopsis pollen hydration, and also to germination associated membrane tension (Hamilton et al., 2015). The role of mechanosensitive channels in Cl– transport in plant salt stress responses should be studied in greater detail.
The channels and transporters involved in Cl− transport in plants under salt stress are summarized in Figure 1. Interesting topics with a potential for future study include dissecting the possible role and contributions of Cl– transport in plant overall salt tolerance, identifying specific channels/transporters involved in Cl– transport, and establishing which contribute to salt tolerance, as well as investigating a possible link to other signalling events e.g. Ca2+ and ROS waves.
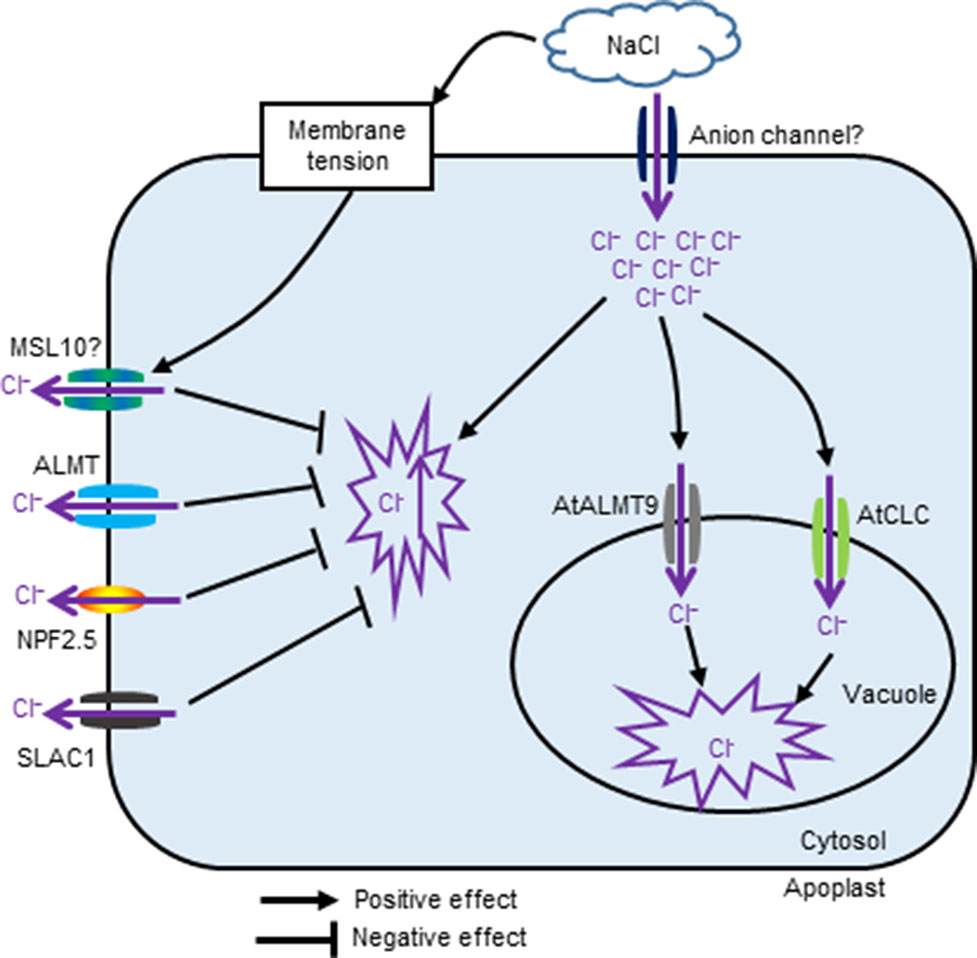
Figure 1 The proposed schematic showing channels and transporters involved in Cl− transport in plants under salinity stress.
Regulation of Cl– Transport in Plant Salinity Stress Response
Controlling Shoot Cl– Accumulation: The Role of Root and Shoot Cl– Exclusion Differs Between Plant Species
Cl− in soil presents mainly as Cl–, and its movement within the soil is largely determined by water flows (White and Broadley, 2001). Compared to the advanced study of Na+ transport, much less data is available for Cl– transport in plants under salinity. Teakle and Tyerman (2010) summarized several traits of Cl– movement related to plant salt tolerance: 1) reduced net Cl– uptake by roots, 2) reduced net xylem loading of Cl–, 3) intercellular compartmentation of Cl–, 4) intracellular compartmentation of Cl–, and 5) phloem recirculation and translocation within the plant. Efficient exclusion of Cl– from either roots or shoots could avoid excessive accumulation of Cl– in plant tissues.
A significantly lower shoot Cl– concentration was found in salt tolerant barley varieties than in sensitive ones (Tavakkoli et al., 2011), suggesting that controlling the accumulation of shoot Cl– is important for overall salt tolerance. However, whether this is due to root Cl– exclusion or shoot Cl– exclusion still needs to be answered. A question also remains over the differences that may exist between plant species. Chen et al. (2013) showed that wild type soybean has stronger root Cl– exclusion (efflux) than the relatively salt sensitive cultivated soybean varieties, suggesting the important role of root Cl– exclusion in soybean salt tolerance. In citrus rootstocks, tolerance in salt tolerant species, which have significantly lower shoot Cl–, is mostly conferred by superior root resistance to Cl– uptake (Moya et al., 2003). This is further demonstrated by Brumos et al. (2010). In barley, to the contrary, less accumulation of shoot Cl– in salt tolerant than sensitive species (Tavakkoli et al., 2011) may not be due to root Cl– exclusion. It has been shown that although cytosolic and vacuolar Cl– concentration is not different between salt tolerant and sensitive barley varieties, a 73% higher (P < 0.001) apoplast Cl– in root cortical cells was found in salt sensitive compared to tolerant barley varieties (Flowers and Hajibagheri, 2001). Also, a strong and positive correlation was found between grain yield and transcripts of HvSLAH1 and HvSLAC1, (anion channels that mediate Cl− efflux), in barley leaves (Liu et al., 2014). This suggests that shoot Cl– exclusion in barley is associated with overall salt tolerance. Shoot Cl− exclusion is also associated with salt tolerance in some other species, e.g. grapevine, Lotus, and Glycine etc. (Teakle and Tyerman, 2010). Significantly lower xylem Cl– was found in relatively salt tolerant varieties compared to salt sensitive varieties of durum wheat (Line 149 vs Tamaroi) (Läuchli et al., 2008), and Lotus corniculatus (L. tenuis vs L. corniculatus) (Teakle et al., 2007). Overall, the mechanisms controlling shoot Cl– accumulation evidently do differ between plant species.
Shoot and Root Cl– Exclusion: Which Channels or Transporters Play the Major Roles?
To date, the topic of key transporters and channels involved in shoot and root Cl− exclusion is still not yet fully explored. In terms of breeding salt tolerant species, knowing the key transporters and channels controlling Cl− exclusion could be very helpful. For example, overexpression of the genes SOS1, (encoding a key Na+/H+ antiporter for Na+ extrusion from cytosol to apoplast), (Yang et al., 2009; Yue et al., 2012), and NHX1, (encoding a key K+, Na+/H+ exchanger for sequestrating Na+ from cytosol to vacuole), (Apse et al., 1999; Zhang and Blumwald, 2001; Chen et al., 2007; Gouiaa et al., 2012), resulted in increased overall salt tolerance in many plant species. Pyramiding with the approach proposed by Bazihizina et al. (2019), (that of improving the selectivity of the broadly selective anion-transporting proteins), may yield enhanced Cl− exclusion ability and be an important strategy for breeding salt tolerant crops.
SLAC1, a channel located in the plasma membrane, is highly permeable to malate and Cl− (Negi et al., 2008). Transgenic overexpression of AtSLAC1 in tobacco BY-2 cells enhanced cryptogein (an elicitor able to induce ROS production)-induced Cl− efflux (Kurusu et al., 2013). Plants with a mutation in slac1, (the gene for SLAC1 which mediates Cl− efflux), also showed increased Cl− content in guard cells (Negi et al., 2008). Together, these reports suggest that SLAC1 is an anion channel which is involved in Cl− exclusion. SLAH1, a homologue of SLAC1, modulates shoot Cl− accumulation, and thus salt tolerance, in Arabidopsis (Cubero-Font et al., 2016; Qiu et al., 2016). It has been shown that the upregulation of the genes HvSLAH1 and HvSLAC1 in leaves is linked with higher barley grain yield in the field (Liu et al., 2014).
Besides SLAC1, CLC channels/transporters and ALMT transporters might also play a role in Cl− exclusion. Herdean et al. (2016a) proposed that the thylakoid Cl− channel AtCLCe functions in Cl− homeostasis. Wei et al. (2016) showed that by regulating Cl− accumulation, the GmCLC1 channel confers enhanced salt tolerance in soybean. The ALMT (aluminium activated malate transporter) protein family is unique to plants and is able to mediate anion fluxes across cellular membranes (Barbier-Brygoo et al., 2011). At high external Cl−, the permeability ratio of TaALMT1, (Piñeros et al., 2008), and ZmALMT2 (Ligaba et al., 2012), towards malate and Cl− is around 1, suggesting that these channels might be able to mediate a substantial amount of Cl− efflux. AtALMT12, a plasma membrane targeted anion transporter, is mainly permeable to Cl− and nitrate and is involved in stomatal closure (Meyer et al., 2010). Furthermore, Li et al. (2017a) found that AtNPF2.5 (NRT1/PTR Family protein), a Cl– permeable transporter predominantly expressed in root cortical cells, modulates Cl− efflux from roots. Mutation of the AtNPF2.5 gene in Arabidopsis resulted in impaired root exclusion and thus a higher shoot Cl– accumulation (Li et al., 2017a). Overexpression of this gene might increase root Cl– exclusion and thus may allow enhancement of overall salt tolerance in plants. Further, besides its role in limiting shoot Na+ accumulation, the endoplasmic reticulum located transporter GmSALT3 (encoded by “salt tolerance-associated gene on chromosome 3”) (Guan et al., 2014) was found to be involved in leaf Cl− exclusion in soybean under salinity stress (Liu et al., 2016). Moreover, the possibility of involvement of the CCC (cation chloride co-transporter) family in Cl− exclusion cannot be completely ruled out. For example, a ccc2 mutant showed significant higher shoot Cl− than wild type Arabidopsis, and shoot Cl− content was significantly reduced in the ccc2 lines expressing VviCCC under salt stress, thus suggesting its role in shoot Cl− exclusion (Henderson et al., 2015). Overall, the current literature suggests that, among the above-mentioned Cl− channels and transporters, SLAC1 and NPF2.5 are most likely to play an important role in shoot and root Cl− exclusion.
Vacuolar Cl– Sequestration: Another Possible Component for Plant Salt Stress Tolerance?
The vacuole is an organelle that occupies up to 90% of the volume of plant cells (Gao et al., 2015). Depending on the plant species, cell type, and measurement method, reports of electrical difference across the tonoplast vary from -31 to +50 mV (Ginsburg and Ginzburg, 1974; Mertz and Higinbotham, 1976; Rona et al., 1980; Kikuyama, 1986; Miller et al., 2001; Wang et al., 2015). This electrochemical gradient across the tonoplast could possibly facilitate movement of Cl– from the cytosol to the vacuole through channels or transporters, even if vacuolar Cl– is higher than that of the cytosol. Furthermore, the rise of Ca2+ has been seen to cause a transient change in both the plasma membrane and tonoplast potentials in Nitella (Kikuyama, 1986). Furthermore, tonoplast potential is shown to be +9 in barley root cells treated with 0.5 mM CaSO4, and +35 mV when treated with 1 mM KCl + 0.5 mM CaSO4 (Mertz and Higinbotham, 1976), thus demonstrating the variability of tonoplast potential according to media. Under saline conditions, the tonoplast potential could be changed to be more negative or positive. Tonoplast potential in barley leaf mesophyll cells grown in non-saline conditions has been reported to be -4 mV, whereas it waxed negatively to -7 mV in barley plants subjected to salinity, (although this is still close to 0) (Cuin et al., 2003). It is thus shown that a more negative tonoplast potential exists in salt grown plants, which would allow for a more efficient active transport of Cl− from the cytosol into the vacuole. The changes of tonoplast membrane potential induced by salinity may vary significantly between plant species, and cell types. A direct measurement of tonoplast potential in salt grown plants, of a species that is more intolerant to Cl– than Na+, would help answer the question of the importance of vacuolar Cl– in overall salt tolerance. If tonoplast potential is around zero in plants under salt stress which means the driven force for operating active transport is limited, then the movement of Cl− will be predominantly dependent on the Cl− gradient between the cytosol and vacuole, which may not allow the vacuole to sequestrate a large amount of Cl−. In terms of Cl– vacuolar sequestration, after salt stress onset a certain time points (the time point could be varied in plant species and cell types), vacuolar Cl– concentration is higher than cytosol and thus the ion channels with the nature of “downhill” activity will not be able to help in the vacuolar sequestration process. In this case, vacuolar sequestration of Cl– is not likely to happen over a considerable period of time and is thus hardly an important component for plant salt tolerance. This challenges the proposed hypothesis that plants can store Cl– in vacuoles to maintain cytosolic ion homeostasis, since in the above case, without the active transport of Cl–, it cannot be moved from the cytosol to vacuole against the proposed Cl– gradient (high Cl– stored in vacuole). Possibly, with the high cost of ATP, Cl− transporters might play a role in this process. With tens of negative or positive tonoplast potentials, the expense on ATP for active Cl– transport by Cl− transporters can be significantly reduced and thus can allow plants to have a longer time period of vacuolar Cl– sequestration under salt stress. We argue here that if some plant species have tens of negative or positive tonoplast potentials under saline conditions, vacuolar Cl– sequestration could be one of the components of overall salt tolerance. Further studies are required to answer the above questions.
The location of AtCLCa, AtCLCb, AtCLCc, AtCLCg, and AtALMT9 Cl− channels and transporters are known in the tonoplast, (De Angeli et al., 2013; Wei et al., 2015), and hints at the possible contribution of the vacuolar sequestration of Cl- to salt tolerance in plants. Overexpression of the tonoplast located AtCLCc results in higher Cl− accumulation and increased overall salt tolerance in the overexpression line in wild type Arabidopsis (Nguyen et al., 2016; Hu et al., 2017). Also, overexpression of GmCLC1 in soybean hair roots results in more Cl− sequestration in roots and thus less Cl− being transported to the soybean shoot (Wei et al., 2016). AtALMT9, (a malate-activated Cl− channel located in the tonoplast), is involved in vacuolar Cl– sequestration and is required for stomatal opening (De Angeli et al., 2013). Furthermore, AtALMT9 is transcriptionally up-regulated under salt stress, and almt9 knockout mutants have reduced shoot accumulation of Cl− (Baetz et al., 2016). Altogether, the above results suggest that vacuolar Cl– sequestration might be another component for overall plant salt tolerance. However, large scale experiments are still needed to validate this supposition.
Inconsistent Results of Cl– Content in Plant Species With Contrasting Salinity Tolerance: the Importance of Intracellular Distribution of Cl– in Plant Salt Tolerance
It has been argued that plant salt tolerance is related to the ability to regulate both Na+ and Cl– transport to avoid toxicity (Tavakkoli et al., 2011). Under salinity stress, the most tolerant Brassica species showed less accumulation of Cl– in the leaf than the sensitive one (Chakraborty et al., 2016). Interestingly, a significantly lower shoot Cl– concentration was found in the salt sensitive bread wheat variety Krichuaff, than in the tolerant variety Berkut, under mild saline conditions (Genc et al., 2010). Also, Cl– concentration in the xylem sap extracted from cut stems is significantly lower in melon, (which is relatively salt sensitive), than in pumpkin grafted on melon (which is relatively salt tolerant) (Edelstein et al., 2011). With the knockout of the vacuolar Cl– channel ALMT9, which is highly expressed in the vasculature of shoots and roots, a reduced accumulation of Cl- in the shoot was observed, showing that vacuolar Cl– loading is crucial in controlling whole-plant ion movement during exposure to salinity (Baetz et al., 2016). The inconsistent relationship between Cl- accumulation and genotypic salt tolerance suggests that the intracellular distribution of Cl– is also important for plant salt tolerance. This is again in support of the viewpoint that vacuolar sequestration of Cl– is important in salt tolerance of plants.
Conclusion
Compared with well-studied Na+ and K+, knowledge on Cl– in plant responses to salt stress is relatively limited. In this review, we argue that vacuolar Cl– sequestration could play a role in salt tolerance, at least in plant species that have either positive or negative tonoplast potential amounting to tens of mV. We also suggest that the key channels/transporters of Cl– exclusion in plants under saline condition still need to be investigated. The restriction of shoot Cl– accumulation, both by root and/or shoot Cl– exclusion, in different plant species is discussed in this review. Further, the toxicity of Cl– originating from CaCl2, (which is particularly relevant to horticultural crops and ornamental plants), is briefly reviewed, and compared with the toxicity of Cl– from NaCl.
Author Contributions
HW and ZL wrote the manuscript.
Funding
This work was supported by funding from Huazhong Agricultural University (11041910138) to HW and NSFC funding to ZL and to HW (31901464).
Conflict of Interest
The authors declare that the research was conducted in the absence of any commercial or financial relationships that could be construed as a potential conflict of interest.
Acknowledgments
We thank Prof. Sergey Shabala from the University of Tasmania, Australia for his help in preparation of this manuscript. We also thank Mr. Joseph Hartley from the University of Tasmania for his help in polishing the English of the manuscript.
References
Al-Whaibi, M. H., Siddiqui, M. H., Basalah, M. O. (2012). Salicylic acid and calcium-induced protection of wheat against salinity. Protoplasma 249, 769–778. doi: 10.1007/s00709-011-0322-1
Anschütz, U., Becker, D., Shabala, S. (2014). Going beyond nutrition: Regulation of potassium homoeostasis as a common denominator of plant adaptive responses to environment. J. Plant Physiol. 171, 670–687. doi: 10.1016/j.jplph.2014.01.009
Apse, M. P., Aharon, G. S., Snedden, W. A., Blumwald, E. (1999). Salt tolerance conferred by overexpression of a vacuolar Na+/H+ antiport in Arabidopsis. Science 285 (80), 1256–1258. doi: 10.1126/science.285.5431.1256
Baetz, U., Eisenach, C., Tohge, T., Martinoia, E., De Angeli, A. (2016). Vacuolar chloride fluxes impact ion content and distribution during early salinity stress. Plant Physiol. 172, 1167–1181. doi: 10.1104/pp.16.00183
Barbier-Brygoo, H., De Angeli, A., Filleur, S., Frachisse, J. M., Gambale, F., Thomine, S., et al. (2011). Anion channels/transporters in plants: from molecular bases to regulatory networks. Annu. Rev. Plant Biol. 62, 25–51. doi: 10.1146/annurev-arplant-042110-103741
Bazihizina, N., Colmer, T. D., Cuin, T. A., Mancuso, S., Shabala, S. (2019). Friend or Foe? Chloride Patterning in Halophytes. Trends Plant Sci. 24, 142–151. doi: 10.1016/j.tplants.2018.11.003
Borghesi, E., Carmassi, G., Uguccioni, M. C., Vernieri, P., Malorgio, F. (2013). Effects of calcium and salinity stress on quality of lettuce in soilless culture. J. Plant Nutrit. 36, 677−690. doi: 10.1080/01904167.2012.721909
Borgognone, D., Cardarelli, M., Lucini, L., Colla, G. (2014). Does CaCl2 play a role in improving biomass yield and quality of cardoon grown in a floating system under saline conditions? HortSci. 49, 1523–1528. doi: 10.21273/HORTSCI.49.12.1523
Broadley, M., Brown, P., Cakmak, I., Rengel, Z., Zhao, F. (2012) “Chapter 7—Function of nutrients: micronutrients.” in Marschner’s mineral nutrition of higher plants, 3rd ed. Marschner, P., Ed. (San Diego, CA, USA : Academic Press ) 191–248. doi: 10.1016/B978-0-12-384905-2.00007-8
Brumos, J., Talon, M., Bouhlal, R., Colmenero-Flores, J. M. (2010). Cl− homeostasis in includer and excluder citrus rootstocks: transport mechanisms and identification of candidate genes. Plant Cell Environ. 33, 2012−2027. doi: 10.1111/j.1365-3040.2010.02202.x
Carillo, P., Woodrow, P., Raimondi, G., El-Nakhel, C., Pannico, A., Kyriacou, M. C., et al. (2019). Omeprazole Promotes Chloride Exclusion and induces salt tolerance in greenhouse basil. Agron. 9, 355. doi: 10.3390/agronomy9070355
Cirillo1, C., De Micco, V., Arena, C., Carillo, P., Pannico, A., De Pascale, S., et al. (2019). Biochemical, physiological and anatomical mechanisms of adaptation of Callistemon citrinus and Viburnum lucidum to NaCl and CaCl2 salinization. Front. Plant Sci. 10, 742. doi: 10.3389/fpls.2019.00742
Chakraborty, K., Bose, J., Shabala, L., Eyles, A., Shabala, S. (2016). Evaluating relative contribution of osmotolerance and tissue tolerance mechanisms toward salinity stress tolerance in three Brassica species. Physiol. Plant 158, 135–151. doi: 10.1111/ppl.12447
Chen, H., An, R., Tang, J. H., Cui, X. H., Hao, F. S., Chen, J., et al. (2007). Over-expression of a vacuolar Na+/H+ antiporter gene improves salt tolerance in an upland rice. Mol. Breed. 19, 215–225. doi: 10.1007/s11032-006-9048-8
Chen, P., Yan, K., Shao, H., Zhao, S. (2013). Physiological mechanisms for high salt tolerance in wild soybean (Glycine soja) from Yellow River Delta, China: Photosynthesis, osmotic regulation, ion flux and antioxidant capacity. PloS One 8, 1–12. doi: 10.1371/journal.pone.0083227
Collaa, G., Rouphaelb, Y., Jawadb, R., Kumara, P., Reac, E., Cardarelli, M. (2013). The effectiveness of grafting to improve NaCl and CaCl2 tolerance in cucumber. Sci. Horiticul. 164, 380–391. doi: 10.1016/j.scienta.2013.09.023
Colmenero-Flores, J. M., Martínez, G., Gamba, G., Vázquez, N., Iglesias, D. J., Brumós, J., et al. (2007). Identification and functional characterization of cation-chloride cotransporters in plants. Plant J. 50, 278–292. doi: 10.1111/j.1365-313X.2007.03048.x
Cubero-Font, P., Maierhofer, T., Jaslan, J., Miguel, A., Rosales, M. A., Espartero, J., et al. (2016). Silent S-type anion channel subunit SLAH1 gates SLAH3 open for chloride root-to-shoot translocation. Curr. Biol. 26, 1–8. doi: 10.1016/j.cub.2016.06.045
Cuin, T. A., Miller, A. J., Laurie, S. A., Leigh, R. A. (2003). Potassium activities in cell compartments of salt-grown barley leaves. J. Exp. Bot. 54, 657–661. doi: 10.1093/jxb/erg072
Cramer, G. R., Epstein, E., Lauchli, A. (1991). Effects of sodium, potassium and calcium on salt-stressed barley. II. Elemental analysis. Physiol. Plant. 81, 197−202. doi: 10.1111/j.1399-3054.1991.tb02129.x
De Angeli, A., Zhang, J., Meyer, S., Martinoia, E. (2013). AtALMT9 is a malate-activated vacuolar chloride channel required for stomatal opening in Arabidopsis. Nat. Commun. 4, 1804. doi: 10.1038/ncomms2815
Deinlein, U., Stephan, A. B., Horie, T., Luo, W., Xu, G., Schroeder, J. I. (2014). Plant salt-tolerance mechanisms. Trends Plant Sci. 19, 371–379. doi: 10.1016/j.tplants.2014.02.001
Dreyer, I., Uozumi, N. (2011). Potassium channels in plant cells. FEBS J. 278, 4293–4303. doi: 10.1111/j.1742-4658.2011.08371.x
Edelstein, M., Plaut, Z., Ben-Hur, M. (2011). Sodium and chloride exclusion and retention by non-grafted and grafted melon and Cucurbita plants. J. Exp. Bot. 62, 177–184. doi: 10.1093/jxb/erq255
Falke, L. C., Edwards, K. L., Pickard, B. G., Misler, S. (1988). A stretch-activated anion channel in tobacco protoplasts. FEBS Lett. 237, 141–144. doi: 10.1016/0014-5793(88)80188-1
Fita, A., Rodríguez-Burruezo, A., Boscaiu, M., Prohens, J., Vicente, O. (2015). Breeding and domesticating crops adapted to drought and salinity: A new paradigm for increasing food production. Front. Plant Sci. 6, 978. doi: 10.3389/fpls.2015.00978
Flowers, T. J., Hajibagheri, M. A. (2001). Salinity tolerance in Hordeum vulgare: ion concentrations in root cells of cultivars differing in salt tolerance Plant and Soil 231, 1–9. doi: 10.1023/A:1010372213938
Franco-Navarro, J. D., Brumós, J., Rosales, M. A., Cubero-Font, P., Talón, M., Colmenero-Flores, J. M. (2016). Chloride regulates leaf cell size and water relations in tobacco plants. J. Exp. Bot. 67, 873–891. doi: 10.1093/jxb/erv502
Franco-Navarro, J. D., Rosales, M. A., Cubero-Font, P., Calvo, P., Álvarez, R., Diaz-Espejo, A., et al. (2019). Chloride as macronutrient increases water use efficiency by anatomically-driven reduced stomatal conductance and increased mesophyll diffusion to CO 2 Running Title: Cl – simultaneously reduces g s and increases g m. Plant J. 99, 1–17. doi: 10.1111/tpj.14423
Gao, C., Zhao, Q., Jiang, L. (2015). Vacuoles protect plants from high magnesium stress. Proc. Natl. Acad. Sci. 112, 2931–2932. doi: 10.1073/pnas.1501318112
Geilfus, C. M. (2018a). Chloride: From Nutrient to Toxicant. Plant Cell Physiol. 59, 877–886. doi: 10.1093/pcp/pcy071
Geilfus, C. M. (2018b). Review on the significance of chlorine for crop yield and quality. Plant Sci. 270, 114–122. doi: 10.1016/j.plantsci.2018.02.014
Genc, Y., Tester, M., McDonald, G. K. (2010). Calcium requirement of wheat in saline and non-saline conditions. Plant Soil 327, 331–345. doi: 10.1007/s11104-009-0057-3
Ginsburg, H., Ginzburg, B. Z. (1974). Radial water and solute flows in roots of Zea mays: I. Water flow. J. Exp. Bot. 25, 28–35. doi: 10.1093/jxb/21.3.580
Gouiaa, S., Khoudi, H., Leidi, E. O., Pardo, J. M., Masmoudi, K. (2012). Expression of wheat Na+/H+ antiporter TNHXS1 and H+- pyrophosphatase TVP1 genes in tobacco from a bicistronic transcriptional unit improves salt tolerance. Plant Mol. Biol. 79, 137–155. doi: 10.1007/s11103-012-9901-6
Guan, R., Qu, Y., Guo, Y., Yu, L., Liu, Y., Jiang, J. (2014). Salinity tolerance in soybean is modulated by natural variation in GmSALT3. Plant J. 80, 937–950. doi: 10.1111/tpj.12695
Hamilton, E. S., Jensen, G. S., Maksaev, G., Katims, A., Sherp, A. M., Haswell, E. S. (2015). Mechanosensitive channel MSL8 regulates osmotic forces during pollen hydration and germination. Science 350 (80), 438–441. doi: 10.1126/science.aac6014
Hanin, M., Ebel, C., Ngom, M., Laplaze, L., Masmoudi, K. (2016). New insights on plant salt tolerance mechanisms and their potential use for breeding. Front. Plant Sci. 7, 1–17. doi: 10.3389/fpls.2016.01787
Henderson, S. W., Baumann, U., Blackmore, D. H., Walker, A. R., Walker, R. R., Gilliham, M. (2014). Shoot chloride exclusion and salt tolerance in grapevine is associated with differential ion transporter expression in roots. BMC Plant Biol. 14, 273. doi: 10.1186/s12870-014-0273-8
Henderson, S. W., Wege, S., Qiu, J., Blackmore, D. H., Walker, A. R., Tyerman, S. D., et al. (2015) Grapevine and Arabidopsis cation-chloride cotransporters localize to the Golgi and trans-Golgi network and indirectly influence long-distance ion transport and plant salt tolerance. Plant Physiol. 169, 2215–2229. doi: 10.1104/pp.15.00499
Herdean, A., Nziengui, H., Zsiros, O., Solymosi, K., Garab, G., Lundin, B., et al. (2016a). The Arabidopsis thylakoid chloride channel AtCLCe functions in chloride homeostasis and regulation of photosynthetic electron transport. Front. Plant Sci. 7, 1–15. doi: 10.3389/fpls.2016.00115
Herdean, A., Teardo, E., Nilsson, A. K., Pfeil, B. E., Johansson, O. N., Ünnep, R., et al. (2016b). A voltage-dependent chloride channel fine-tunes photosynthesis in plants. Nat. Commun. 7, 11654. doi: 10.1038/ncomms11654
Horie, T., Karahara, I., Katsuhara, M. (2012). Salinity tolerance mechanisms in glycophytes: An overview with the central focus on rice plants. Rice 5, 1–18. doi: 10.1186/1939-8433-5-11
Hu, R., Zhu, Y., Wei, J., Chen, J., Shi, H., Shen, G., et al. (2017). Overexpression of PP2A-C5 that encodes the catalytic subunit 5 of protein phosphatase 2A in Arabidopsis confers better root and shoot development under salt conditions. Plant Cell Environ. 40, 150–164. doi: 10.1111/pce.12837
Kikuyama, M. (1986). Tonoplast action potential of Characeae. Plant Cell Physiol. 27, 1461–1468. doi: 10.1093/oxfordjournals.pcp.a077246
Kurusu, T., Saito, K., Horikoshi, S., Hanamata, S., Negi, J., Yagi, C., et al. (2013). An S-type anion channel SLAC1 is involved in cryptogein-induced ion fluxes and modulates hypersensitive responses in tobacco BY-2 cells. PloS One 8, e70623. doi: 10.1371/journal.pone.0070623
Läuchli, A., James, R. A., Huang, C. X., McCully, M., Munns, R. (2008). Cell-specific localization of Na+ in roots of durum wheat and possible control points for salt exclusion. Plant Cell Environ. 31, 1565–1574. doi: 10.1111/j.1365-3040.2008.01864.x
Li, B., Qiu, J., Jayakannan, M., Xu, B., Li, Y., Mayo, G. M., et al. (2017a). AtNPF2.5 modulates chloride (Cl–) efflux from roots of Arabidopsis thaliana. Front. Plant Sci. 7, 2013. doi: 10.3389/fpls.2016.02013
Li, B., Tester, M., Gilliham, M. (2017b). Chloride on the Move. Trends Plant Sci. 22, 236–248. doi: 10.1016/j.tplants.2016.12.004
Ligaba, A., Maron, L., Shaff, J., Kochian, L., Piñeros, M. (2012). Maize ZmALMT2 is a root anion transporter that mediates constitutive root malate efflux. Plant Cell Environ. 35, 1185–1200. doi: 10.1111/j.1365-3040.2011.02479.x
Liu, X., Mak, M., Babla, M., Wang, F., Chen, G., Veljanoski, F., et al. (2014). Linking stomatal traits and expression of slow anion channel genes HvSLAH1 and HvSLAC1 with grain yield for increasing salinity tolerance in barley. Front. Plant Sci. 5, 1–12. doi: 10.3389/fpls.2014.00634
Liu, Y., Yu, L., Qu, Y., Chen, J., Liu, X., Hong, H., et al. (2016). GmSALT3, which confers improved soybean salt tolerance in the field, increases leaf Cl− exclusion prior to Na+ exclusion but does not improve early vigor under salinity. Front. Plant Sci. 7, 1485. doi: 10.3389/fpls.2016.01485
Maathuis, F. J. M. (2014). Sodium in plants: Perception, signalling, and regulation of sodium fluxes. J. Exp. Bot. 65, 849–858. doi: 10.1093/jxb/ert326
Maksaev, G., Haswell, E. S. (2012). MscS-Like10 is a stretch-activated ion channel from Arabidopsis thaliana with a preference for anions. Proc. Natl. Acad. Sci. 109, 19015–19020. doi: 10.1073/pnas.1213931109
Martinez, C. J., Clark, M. W. (2012). Using reclaimed water for landscape irrigation. Gainesville, FL, USA: Florida Cooperative Extension Service, University of Florida.
Mertz, S. T., Jr., Higinbotham, N. (1976). Transmembrane electropotential in barley roots as related to cell type, cell location, and cutting and aging effects. Plant Physiol. 57, 123–128. doi: 10.1104/pp.57.2.123
Meyer, S., Mumm, P., Imes, D., Endler, A., Weder, B., Al-Rasheid, K. A. S., et al. (2010). AtALMT12 represents an R-type anion channel required for stomatal movement in Arabidopsis guard cells. Plant J. 63, 1054–1062. doi: 10.1111/j.1365-313X.2010.04302.x
Miller, A. J., Cookson, S. J., Smith, S. J., Wells, D. M. (2001). The use of microelectrodes to investigate compartmentation and the transport of metabolized inorganic ions in plants. J. Exp. Bot. 52, 541–549. doi: 10.1093/JEXBOT/52.356.541
Moya, J. L., Gómez-Cadenas, A., Primo-Millo, E., Talon, M. (2003). Chloride absorption in salt-sensitive Carrizo citrange and salt-tolerant Cleopatra mandarin citrus rootstocks is linked to water use. J. Exp. Bot. 54, 825–833. doi: 10.1093/jxb/erg064
Munns, R., Tester, M. (2008). Mechanisms of salinity tolerance. Annu. Rev. Plant Biol. 59, 651–681. doi: 10.1146/annurev.arplant.59.032607.092911
Nackley, L. L., Barnes, C., Oki, L. R. (2015) Investigating the impacts of recycled water on long-lived conifers. AoB Plants 7, plv035. doi: 10.1093/aobpla/plv035
Negi, J., Matsuda, O., Nagasawa, T., Oba, Y., Takahashi, H., Kawai-Yamada, M., et al. (2008). CO2 regulator SLAC1 and its homologues are essential for anion homeostasis in plant cells. Nature 452, 483–486. doi: 10.1038/nature06720
Nguyen, C. T., Agorio, A., Jossier, M., Depré, S., Thomine, S., Filleur, S. (2016). Characterization of the chloride channel-like, AtCLCg, involved in chloride tolerance in Arabidopsis thaliana. Plant Cell Physiol. 57, 764–775. doi: 10.1093/pcp/pcv169
Piñeros, M. A., Cançado, G. M. A., Kochian, L. V. (2008). Novel properties of the wheat aluminum tolerance organic acid transporter (TaALMT1) revealed by electrophysiological characterization in Xenopus Oocytes: functional and structural implications. Plant Physiol. 147, 2131–2146. doi: 10.1104/pp.108.119636
Qiu, J., Henderson, S. W., Tester, M., Roy, S. J., Gilliham, M. (2016). SLAH1, a homologue of the slow type anion channel SLAC1, modulates shoot Cl- accumulation and salt tolerance in Arabidopsis thaliana. J. Exp. Bot. 67, 4495–4505. doi: 10.1093/jxb/erw237
Rahman, A., Nahar, K., Hasanuzzaman, M., Fujita, M. (2016). Calcium supplementation improves Na+/K+ ratio, antioxidant defense and glyoxalase systems in salt-stressed rice seedlings. Front. Plant Sci. 7, 609. doi: 10.3389/fpls.2016.00609
Raven, J. A. (2017). Chloride: Essential micronutrient and multifunctional beneficial ion. J. Exp. Bot. 68, 359–367. doi: 10.1093/jxb/erw421
Rona, J. P., Pitman, M. G., Lüttge, U., Ball, E. (1980). Electrochemical data on compartmentation into cell wall, cytoplasm, and vacuole of leaf cells in the CAM genus Kalanchoë. J. Membr. Biol. 57, 25–35. doi: 10.1007/BF01868983
Seemann, J. R., Critchley, C. (1985). Effects of salt stress on the growth, ion content, stomatal behaviour and photosynthetic capacity of a salt-sensitive species, Phaseolus vulgaris L. Planta. 164, 151–162. doi: 10.1007/BF00396077
Shabala, S., Babourina, O., Newman, I. (2000). Ion-specific mechanisms of osmoregulation in bean mesophyll cells. J. Exp. Bot. 51, 1243–1253. doi: 10.1093/jexbot/51.348.1243
Shabala, S., Demidchik, V., Shabala, L., Cuin, T. A., Smith, S. J., Miller, A. J., et al. (2006). Extracellular Ca2+ ameliorates NaCl-induced K+ loss from Arabidopsis root and leaf cells by controlling plasma membrane K+-permeable channels. Plant Physiol. 141, 1653–1665. doi: 10.1104/pp.106.082388
Shabala, S., Pottosin, I. (2014). Regulation of potassium transport in plants under hostile conditions: Implications for abiotic and biotic stress tolerance. Physiol. Plant 151, 257–279. doi: 10.1111/ppl.12165
Shabala, S., Shabala, L., Van Volkenburgh, E., Newman, I. (2005). Effect of divalent cations on ion fluxes and leaf photochemistry in salinized barley leaves. J. Exp. Bot. 56, 1369–1378. doi: 10.1093/jxb/eri138
Skerrett, M., Tyerman, S. D. (1994). A channel that allows inwardly directed fluxes of anions in protoplasts derived from wheat roots. Planta 192, 295–305. doi: 10.1007/BF00198563
Tavakkoli, E., Fatehi, F., Coventry, S., Rengasamy, P., McDonald, G. K. (2011). Additive effects of Na+ and Cl- ions on barley growth under salinity stress. J. Exp. Bot. 62, 2189–2203. doi: 10.1093/jxb/erq422
Tavakkoli, E., Rengasamy, P., McDonald, G. K. (2010). High concentrations of Na+ and Cl- ions in soil solution have simultaneous detrimental effects on growth of faba bean under salinity stress. J. Exp. Bot. 61, 4449–4459. doi: 10.1093/jxb/erq251
Teakle, N. L., Flowers, T. J., Real, D., Colmer, T. D. (2007). Lotus tenuis tolerates the interactive effects of salinity and waterlogging by “excluding” Na+ and Cl- from the xylem. J. Exp. Bot. 58, 2169–2180. doi: 10.1093/jxb/erm102
Teakle, N. L., Tyerman, S. D. (2010). Mechanisms of Cl- transport contributing to salt tolerance. Plant Cell Environ. 33, 566–589. doi: 10.1111/j.1365-3040.2009.02060.x
van Ittersum, M. K., van Bussel, L. G. J., Wolf, J., Grassini, P., van Wart, J., Guilpart, N., et al. (2016). Can sub-Saharan Africa feed itself? Proc. Natl. Acad. Sci. 113, 14964–14969. doi: 10.1073/pnas.1610359113
Wang, M., Zheng, Q., Shen, Q., Guo, S. (2013). The critical role of potassium in plant stress response. Int. J. Mol. Sci. 14, 7370–7390. doi: 10.3390/ijms14047370
Wang, Y., Dindas, J., Rienmüller, F., Krebs, M., Waadt, R., Schumacher, K., et al. (2015). Cytosolic Ca2+ signals enhance the vacuolar ion conductivity of bulging Arabidopsis root hair cells. Mol. Plant 8, 1665–1674. doi: 10.1016/j.molp.2015.07.009
Wang, Z., Li, J., Li, Y. (2017). Using reclaimed water for agricultural and landscape irrigation in China: a review. Irrig. Drain. 66, 672−686. doi: 10.1002/ird.2129
Wege, S., Gilliham, M., Henderson, S. W. (2017). Chloride: Not simply a “cheap osmoticum”, but a beneficial plant macronutrient. J. Exp. Bot. 68, 3057–3069. doi: 10.1093/jxb/erx050
Wei, P., Wang, L., Liu, A., Yu, B., Lam, H.-M. (2016). GmCLC1 confers enhanced salt tolerance through regulating chloride accumulation in soybean. Front. Plant Sci. 7, 1–11. doi: 10.3389/fpls.2016.01082
Wei, Q. J., Gu, Q. Q., Wang, N. N., Yang, C. Q., Peng, S. A. (2015). Molecular cloning and characterization of the chloride channel gene family in trifoliate orange. Biol. Plant 59, 645–653. doi: 10.1007/s10535-015-0532-z
White, P., Broadley, M. R. (2001). Chloride in soils and its uptake and movement within the plant: A review. Ann. Bot. 88, 967–988. doi: 10.1006/anbo.2001.1540
Wu, H. (2018). Plant salt tolerance and Na + sensing and transport. Crop J. 6, 215–225. doi: 10.1016/j.cj.2018.01.003
Wu, H., Shabala, L., Azzarello, E., Huang, Y., Pandolfi, C., Su, N., et al. (2018a). Na + extrusion from the cytosol and tissue-specific Na + sequestration in roots confer differential salt stress tolerance between durum and bread wheat. J. Exp. Bot. 69, 3987–4001. doi: 10.1093/jxb/ery194
Wu, H., Shabala, L., Liu, X., Azzarello, E., Zhou, M., Pandolfi, C., et al. (2015). Linking salinity stress tolerance with tissue-specific Na+ sequestration in wheat roots. Front. Plant Sci. 6, 71. doi: 10.3389/fpls.2015.00071
Wu, H., Shabala, L., Shabala, S., Giraldo, J. P. (2018b). Hydroxyl radical scavenging by cerium oxide nanoparticles improves Arabidopsis salinity tolerance by enhancing leaf mesophyll potassium retention. Environ. Sci. Nano 5, 1567–1583. doi: 10.1039/c8en00323h
Wu, H., Zhang, X., Giraldo, J. P., Shabala, S. (2018c). It is not all about sodium: revealing tissue specificity and signalling roles of potassium in plant responses to salt stress. Plant Soil 431, 1–17. doi: 10.1007/s11104-018-3770-y
Xu, X., Magen, H., Tarchitzky, J., Kafkafi, U. (1999). Advances in chloride nutrition of plants. Adv. Agron. 68, 97–150. doi: 10.1016/S0065-2113(08)60844-5
Xu, D., Wang, W., Gao, T., Fang, X., Gao, X., Li, J., et al. (2017). Calcium alleviates decreases in photosynthesis under salt stress by enhancing antioxidant metabolism and adjusting solute accumulation in Calligonum mongolicum. Conserv. Physiol. 5, cox060. doi: 10.1093/conphys/cox060
Yang, Q., Chen, Z. Z., Zhou, X. F., Yin, H. B., Li, X., Xin, X. F., et al. (2009). Overexpression of SOS (salt overly sensitive) genes increases salt tolerance in transgenic Arabidopsis. Mol. Plant 2, 22–31. doi: 10.1093/mp/ssn058
Yue, Y., Zhang, M., Zhang, J., Duan, L., Li, Z. (2012). SOS1 gene overexpression increased salt tolerance in transgenic tobacco by maintaining a higher K+/Na+ ratio. J. Plant Physiol. 169, 255–261. doi: 10.1016/j.jplph.2011.10.007
Zhang, H. X., Blumwald, E. (2001). Transgenic salt-tolerant tomato plants accumulate salt in foliage but not in fruit. Nat. Biotechnol. 19, 765–768. doi: 10.1038/90824
Keywords: Cl− exclusion, Cl− transport, ion channels and transporters, salinity stress tolerance, vacuolar Cl− sequestration
Citation: Wu H and Li Z (2019) The Importance of Cl− Exclusion and Vacuolar Cl− Sequestration: Revisiting the Role of Cl− Transport in Plant Salt Tolerance. Front. Plant Sci. 10:1418. doi: 10.3389/fpls.2019.01418
Received: 02 July 2019; Accepted: 11 October 2019;
Published: 08 November 2019.
Edited by:
Meixue Zhou, University of Tasmania, AustraliaReviewed by:
Petronia Carillo, University of Campania, Luigi Vanvitelli, ItalyMiguel A. Rosales, Institute of Natural Resources and Agrobiology of Seville (CSIC), Spain
Copyright © 2019 Wu and Li. This is an open-access article distributed under the terms of the Creative Commons Attribution License (CC BY). The use, distribution or reproduction in other forums is permitted, provided the original author(s) and the copyright owner(s) are credited and that the original publication in this journal is cited, in accordance with accepted academic practice. No use, distribution or reproduction is permitted which does not comply with these terms.
*Correspondence: Honghong Wu, aG9uZ2hvbmcud3VAbWFpbC5oemF1LmVkdS5jbg==; Zhaohu Li, bGl6aGFvaHVAY2F1LmVkdS5jbg==