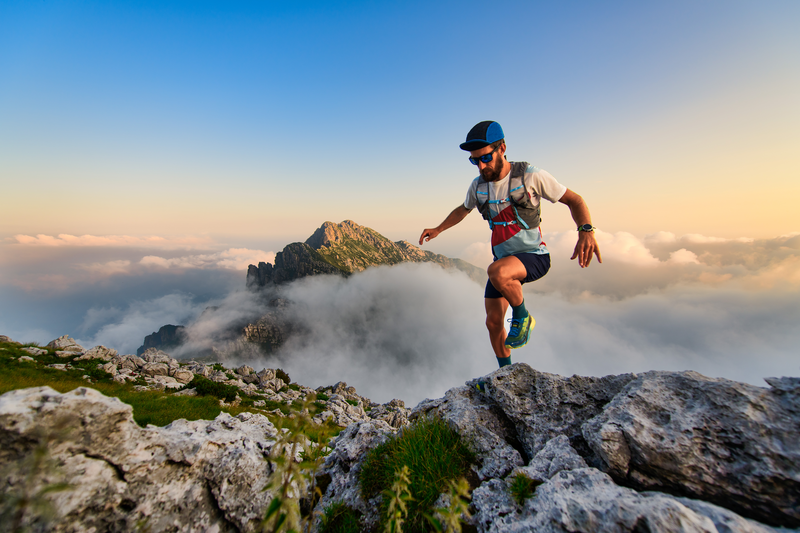
94% of researchers rate our articles as excellent or good
Learn more about the work of our research integrity team to safeguard the quality of each article we publish.
Find out more
ORIGINAL RESEARCH article
Front. Plant Sci. , 22 October 2019
Sec. Plant Nutrition
Volume 10 - 2019 | https://doi.org/10.3389/fpls.2019.01308
This article is part of the Research Topic Importance of Root Symbiomes for Plant Nutrition: New Insights, Perspectives and Future Challenges View all 13 articles
Our objective was to better understand how organic and inorganic nitrogen (N) forms supplied to a tree, Robinia pseudoacacia, and a perennial forb, Lupinus latifolius, affected plant growth and performance of their symbiotic, N-fixing rhizobia. In one experiment, we tested five sources of N [none; three inorganic forms (ammonium, nitrate, ammonium-nitrate); and an organic form (arginine)] in combination with or without rhizobia inoculation. We measured seedling morphology, allometry, nodule biomass, and N status. A second experiment explored combinations of supplied 15N and inoculation to examine if inorganic or organic N was deleterious to nodule N-fixation. Plant growth was similar among N forms. A positive response of nodule biomass to N was greater in Robinia than Lupinus. For Robinia, inorganic ammonium promoted more nodule biomass than organic arginine. N-fixation was concurrent with robust supply of either inorganic or organic N, and N supply and inoculation significantly interacted to enhance growth of Robinia. For Lupinus, the main effects of inoculation and N supply increased growth but no interaction was observed. Our results indicate that these important restoration species for forest ecosystems respond well to organic or inorganic N forms (or various forms of inorganic N), suggest that the nodulation response may depend on plant species, and show that, in terms of plant growth, N supply and nodulation can be synergistic.
Nitrogen (N) has long been demonstrated as the most critical element for enhanced productivity of plant growth. Soil N is present in inorganic forms, such as ammonium and nitrate, and organic forms, such as amino acids (Christou et al., 2006). Ammonium and nitrate are the predominant inorganic N forms usually taken up by plants (Hawkins and Robbins, 2010). Studies confirmed, however, that amino acids sometimes serve as a primary N source for vegetation (Kielland, 1994; Persson and Näsholm, 2001; Inselsbacher and Näsholm, 2012; Whiteside et al., 2012; Wei et al., 2015) and plants have a reduced carbon cost when assimilating organic N into proteins (Franklin et al., 2017). Organic N can be an efficient and environmentally favorable N source (Öhlund and Näsholm, 2002).
Plants may show different growth responses to the form of N they are provided. For trees, Bigg and Daniel (1978) and Yao et al. (2011) collectively examined six conifer species and found that three species grow better with ammonium than nitrate (Picea engelmannii, Pinus contorta, Pinus yunnanensis), two grow better with nitrate than ammonium (Pseudotsuga menziesii, Pinus densata), and one had no preference (Pinus tabuliformis). Picea abies and Pinus sylvestris seedlings, with an ability to uptake intact amino acids, grow as well as, or better, when they are supplied with amino acids compared to seedlings with access to inorganic N sources (Öhlund and Näsholm, 2001; Gruffman et al., 2012). Conversely, a field study with Abies fraseri, Pinus resinosa, and Populus nigra × Populus maximowiczii found that seedling growth and foliar N response resulting after an amino acid supply was similar to inorganic N applications only when the organic form was applied at rates two or three times higher than that of the inorganic form (Wilson et al., 2013). The N form provided in the growth substrate affects not only plant growth but also plant biomass allocation. Amino acids were suggested to have a direct positive effect on root growth of the small herb Arabidopsis thaliana cultivated in a sterile agar growth system (Cambui et al., 2011). Picea abies and P. sylvestris seedlings given organic N (arginine) in a container nursery had larger root systems and a greater root-to-shoot ratio than did seedlings with access to inorganic N (Gruffman et al., 2012).
Inoculation of seeds with rhizobia is known to increase nodulation, leading to enhanced N content, growth, and yield of legume crops (Adgo and Schulze, 2002; Gan et al., 2010; Namvar et al., 2011) and woody species (Dan et al., 1993; Diabate et al., 2005; Jayakumar and Tan, 2006; Dumroese et al., 2009). The effectiveness of inoculation on nodulation and subsequent plant growth response can be affected by N fertilization, but the conclusions are mixed. While broadly concluded that mineral soil N [nitrate (NO3−) and ammonium (NH4+)] inhibits nodulation, nodule growth, and activity in legumes (Imsande, 1986; Streeter and Wong, 1988; Guo et al., 1992; Bollman and Vessey, 2006; Camargos and Sodek, 2010), studies have shown that low (Rohm and Werner, 1991; Vessey and Waterer, 1992; Gulden and Vessey, 1997; Goicoechea et al., 2005; Yashima et al., 2005) and moderate (Peoples et al., 1995; Bado et al., 2006) supplies of N can stimulate nodulation and N fixation. Nodulation with apparent N-fixing ability has also been noted when N was supplied in high amounts (Dumroese et al., 2009). These differences may be a function of either the symbiosis or the N source.
The response of legumes to N level is species specific and depends upon the particular rhizobia-legume symbiosis (Manhart and Wong, 1980; Harper and Gibson, 1983). Nodules of Lupinus angustifolius infected with Rhizobium sp. 127E15 were unaffected by addition of 15 mM NO3−, whereas all other combinations of rhizobia–L. angustifolius and rhizobia–Vigna unguiculata averaged a 30% reduction in nodule biomass (Manhart and Wong, 1980). Nitrate was found to induce an inhibitory effect on nodulation and N fixation in pea plants at levels as low as 0.1 mM, while the level at which NH4+ became inhibitory was 1.0 mM and higher (Rys and Phung, 1984; Silsbury et al., 1986; Waterer and Vessey, 1993a, Waterer and Vessey, 1993b; Svenning et al., 1996). While sensitivity to NO3− has been found to occur in all legume species studied so far (Streeter and Wong, 1988; Lucinski et al., 2002; Fei and Vessey, 2003), the impact of NH4+ on symbiosis is weaker than that observed for NO3− (Bollman and Vessey, 2006). Streeter and Wong (1988) noted that nitrate may affect the symbiotic process by reducing nodule formation, reducing nitrogenase activity, or by affecting the ratio of nodule dry mass to whole plant mass. These lines of research continue (Lucinski et al., 2002) but with increasing attention to molecular changes that influence N-fixation brought about by NO3− (e.g., Valkov et al., 2017). Despite this intensive effort, the combined effects of N and rhizobia inoculation on nonagronomic legumes have received little attention. To our knowledge, no studies have examined the effects of organic N on nodule formation and subsequent plant growth.
In this study, we assessed the plant response of two Fabaceae (legume) species supplied with different sources of N and rhizobia inoculation in a controlled greenhouse system. Lupinus latifolius, hereafter lupine, is a native perennial forb occurring in forests of western North America from British Columbia to Baja California to New Mexico. It has a bushy, densely branched growth habit originating from a woody caudex (Antos and Halpern, 1997). It is commonly used for rehabilitation of disturbed forest sites and for erosion control because it grows well on droughty and/or low-fertility sites, has a deep root system for stabilizing soil, and forms associations with N-fixing microorganisms (Halverson et al., 1986; Doede, 2005). Robinia pseudoacacia, hereafter black locust, is a medium-sized tree native to the southeastern United States that has been widely planted and naturalized elsewhere in temperate North America, Europe, southern Africa, and Asia (Olson and Karrfalt, 2008). In the United States, it has been used for afforestation of abandoned farmlands, timber, erosion control, and soil stabilization on disturbed sites (Keresztesi, 1980; Roberts et al., 1983). Both species are commonly grown as container seedlings in some nurseries in North America, and so determining proper N supply and inoculation regimes will undoubtedly have a significant impact on the availability of these two species for restoration activities.
Therefore, our goal was to investigate the growth and allometry, physiological responses, and nodulation status of lupine and black locust provided N in organic and inorganic forms, with or without rhizobia inoculation. To the best of our knowledge, this is the first study to examine the effects of supplying organic N to seedlings and subsequent rhizobia nodule development and function. Arginine was chosen as our model amino acid partly because it had been used in other studies (Öhlund and Näsholm, 2001; Öhlund and Näsholm, 2002; Persson et al., 2003; Öhlund and Näsholm, 2004; Gruffman et al., 2013; Wilson et al., 2013) and partly because of its chemical properties. Arginine is an N-rich molecule containing four N atoms, which collectively contribute 32% of its molecular weight. It is positively charged and thus acts as a basic cation in soils with acidic pH, binding to negatively charged soil particles, which restricts its mobility. Our objectives were to see whether (1) seedlings supplied with organic and inorganic N forms have similar growth; (2) inoculated and noninoculated seedlings supplied with organic and inorganic N forms have similar rhizobia nodule biomass; and (3) high rates of organic and inorganic N supply discourage N fixation.
To meet our objectives, we grew lupine (collected in 2010 at 919 m elevation on the Newport Ranger District, Colville National Forest, Washington, USA) and black locust (collected in 2012 in Kentucky, USA; Lawyer Nursery, Inc., Plains, Montana, USA). Seeds were treated with hot water scarification before sowing. Lupine seeds were submerged 1 min in 100°C water (seeds:water = 10 g:800 ml), immediately soaked in running tap water for 48 h, and stratified 15 days at 3°C. Black locust seeds were submerged in 70°C water (seeds:water = 15 g:500 ml), the heat source was immediately removed, and the seeds and water were allowed to cool for 24 h. Expanded seeds of each species were sown.
Our first experiment was a completely randomized design with two independent factors: rhizobia inoculation and N source. We employed two levels of rhizobia inoculation (control or inoculated) and five sources of N: none; three inorganic forms [ammonium (NH4+), nitrate (NO3−), ammonium-nitrate (NH4NO3)]; and an organic form (L-arginine; Sigma-Aldrich Co., St. Louis, Missouri, USA). At the time of planting, one half of the seeds was inoculated with either lupine- or black locust-appropriate rhizobia. The lupine inoculant included three proprietary isolates, whereas black locust was a combination of a proprietary isolate and USDA 3436 (Plant Probiotics Company, Indianapolis, Indiana, USA). For each inocula replication, we slightly moistened the scarified seeds, applied each peat-based inocula at a rate of 1 g (∼3 × 108 CFUs) per 100 g of seeds, thoroughly mixed the seeds and inocula, and immediately hand-planted 3 seeds into Ray LeachTM Super Cells (164 cm3, 3.8-cm diameter, 21-cm depth; Stuewe & Sons, Inc., Tangent, Oregon, USA) filled with a 1:1 (v:v) mix of Sphagnum peatmoss:vermiculite (Forestry #1, SunGro Horticulture Canada Ltd., Canada). Sown seeds were covered with 1 cm of coarse grit to reduce evaporation and cryptogam growth. We planted eight cells for each species, inoculation, and N source combination (each seedling served as a replicate). Sown cells were randomly placed onto a table inside a fully controlled greenhouse at the U.S. Department of Agriculture, Forest Service, Rocky Mountain Research Station in Moscow, Idaho (46.7232°, −117.0029°; 798 m elevation), thinned to a single seedling after germination, and rearranged during each irrigation to minimize edge effects.
Seedling irrigation needs were determined gravimetrically for each species, inoculation, and N source combination. When mass of the water reached 75% of the total water mass at container capacity, seedlings were irrigated with just enough water to return the cells to container capacity (Dumroese et al., 2015); this occurred one or more times each week. Fourteen days after planting (DAP), we began supplying N. Each lupine seedling received a total of 6 mg N during a 6-week period (1 mg per week; 14 to 56 DAP), whereas black locust seedlings each received a total of 24 mg N during a 12-week period (2 mg per week; 14 to 98 DAP). We used three different nutrient stock solutions, differing only with respect to the chemical form of N (Table 1). Each stock solution also included micronutrients [sulfur (S), 13%; manganese (Mn), 8%; iron (Fe), 7.5%; copper (Cu), 2.3%; boron (B), 1.35%; molybdenum (Mo), 0.04%; Peters Professional® S.T.E.M.TM, the Scotts Company, Marysville, Ohio, USA] at 15 mg L−1 and Sprint 330 [10% Fe (chelated); Becker Underwood, Inc., Ames, Iowa, USA] at 20 mg L−1. Once each week, when gravimetric weights indicated irrigation was needed, we calculated the amount of water required to replenish the medium for each seedling to container capacity. This value was always >15 ml seedling−1. Starting with 15 ml of stock solution, we added the appropriate N source at either 1 (lupine) or 2 (black locust) mg N and applied the subsequent solution to an individual seedling via syringe. As required, we then added plain water to reach the total irrigation amount calculated for each seedling based on the gravimetric measurements. The resulting solutions had 67 (lupine) and 134 (black locust) mg N L−1 with concentrations of phosphorus (P), potassium (K), S, calcium (Ca), and magnesium (Mg) provided at ratios of 1.0, 0.7, 1.1, 0.9, and 1.2 to 1.0 N (Table 1). We reinoculated seedlings 28 DAP to ensure inoculation; 0.5 g of inoculant was diluted in 1.25 L water and then 5 ml of inoculant solution (2 mg inoculant) was applied to each seedling.
For lupine, the longest petiole length and the widest leaf width were measured weekly from 28 DAP until harvest 56 DAP. For black locust, seedling height and root-collar diameter (RCD) were measured every 14 days from 42 DAP until harvest 112 DAP. At harvest, we gently washed root systems of both species free of medium and counted and harvested nodules. Shoots (leaf and stems for block locust), roots, and nodules were separated and oven-dried 72 h at 65°C for biomass determination. The oven dried shoots and roots for each treatment were subsequently ground to pass a 0.44-mm mesh and analyzed for N concentration by dry combustion in a LECO CN600 (LECO Corp., St. Joseph, Michigan, USA).
Based on our results from the first experiment, we sowed a second crop of each species as described above (except for N source, total N applied, and growing time) to measure differential dilution of absorbed 15N-labeled fertilizer by N inputs through N-fixation by rhizobia (Hardarson and Danso, 1993). Because preliminary data analyses revealed no significant differences among inorganic N forms for seedling growth, we supplied inorganic N only as NH4NO3 amended with NH4NO3 enriched to 98 atom % 15N (Sigma Aldrich, St. Louis, Missouri, USA). Organic N was supplied as arginine hydrochloride amended with L-arginine-α-hydrochloride enriched to 98 atom % 15N (Cambridge Isotope Laboratory, Inc., Tewksbury, Massachusetts, USA). The 15N:14N was about 0.0037:1. Irrigation and N were supplied as described above, but this time, the black locust seedlings were only treated for 8 weeks (16 mg N total).
Individual seedlings were harvested 56 DAP (lupine) and 70 DAP (black locust). Seedlings were washed free of growing media and rinsed in deionized water, partitioned into leaves, stems, and roots, then oven dried 48 h at 60°C, weighed, and ground as previously described to determine biomass. At least five seedlings from each species × N combination were analyzed for isotopic N. The concentrations of 15N and 14N in each tissue were determined with a continuous flow isotope ratio mass spectrometer (Finnigan Delta PlusXP, Thermo Fisher Scientific, Breman, Germany) at the Washington State University Stable Isotope Core Laboratory (Pullman, Washington, USA). Nitrogen isotope ratio was expressed as follows:
Both experiments were analyzed as factorial designs. Analysis of variance (two-way ANOVA) was used to examine the effects of the independent variables (N, rhizobia inoculation) and their interaction on final seedling petiole length (cm); leaf width (mm); height (cm); RCD (mm); dry biomass (g) of leaves, stems, shoots, roots, and nodules; ratio of shoot dry biomass to root dry biomass (S:R); N concentration (g kg−1) and content (mg kg−1) of leaves, stems, shoots, and roots; and δ15N. Residual plots were used to assure data met model assumptions for homoscedasticity and normality. We used Tukey’s HSD for post hoc tests of differences between model means. Results were considered significant at α = 0.05. Box-plot visualizations were created using SigmaPlot (version 13.0; Systat Software, San Jose, California, USA).
In the first experiment, none of the final measured response variables were affected by an N × inoculation interaction. While N overall was a significant factor, only the presence of N (as opposed to no N) significantly affected every measured response variable except shoot-to-root ratio (S:R) and nodule biomass (Table 2). Seedlings supplied with N had significantly longer petioles and greater leaf width, and a trend toward greater shoot and root biomass, than those not supplied N. Inoculation significantly affected every final measured response variable (Table 2; Figure 1). Inoculated seedlings had significantly longer petioles and wider leaves, as well as significantly more shoot, root, and nodule biomass than the noninoculated seedlings, but noninoculated seedlings had a significantly higher S:R.
Table 2 Mean morphological parameters of lupine seedlings inoculated (I) with (+) or without (−) rhizobia and supplied with different nitrogen (N) sources [none, ammonium (NH4), nitrate (NO3), ammonium nitrate (NH4NO3), or arginine (A)] for 6 weeks (n = 8). Main effect (ME) means are presented in italics. Different letters within a dependent variable indicate significant differences (α = 0.05) using Tukey’s HSD.
Figure 1 Growth responses of black locust and lupine seedlings (98 and 56 days after planting, respectively) supplied inorganic or organic nitrogen with or without rhizobia inoculation and grown in a greenhouse. Noninoculated (A) and inoculated (B) black locust seedlings. Lupine seedlings fertilized with arginine (left plants noninoculated, right plants inoculated) (C) or ammonium-nitrate (left plants noninoculated, right plants inoculated) (D).
Nitrogen significantly affected shoot and root N concentration and content (Table 3). The shoot N concentration was largest for seedlings supplied with inorganic N forms, with arginine and the no-N treatment having the lowest concentrations. Inorganic NH4+ and NH4NO3 had significantly greater root N concentration than seedlings not supplied N, whereas NO3− and arginine were intermediate, yielding results similar to the no-N treatment and the other inorganic sources. Seedlings supplied inorganic and organic N had significantly more shoot N content than no-N seedlings. The largest root N content was provided by NH4NO3 and was significantly more than the no-N treatment, while NH4+, NO3−, and arginine were intermediate, yielding results similar to the no-N treatment and NH4NO3. Inoculation significantly affected shoot and root N concentration and N content (Table 3). Inoculated seedlings had 2×, 1.5×, 10×, and 7× the shoot N concentration, root N concentration, shoot N content, and root N content of the noninoculated seedlings.
Table 3 Mean nitrogen (N) concentrations and contents of lupine seedlings inoculated (I) with (+) or without (−) rhizobia and supplied with different N sources [none, ammonium (NH4), nitrate (NO3), ammonium nitrate (NH4NO3), or arginine (A)] for 6 weeks (n = 8). Main effect (ME) means are presented in italics. Different letters within a dependent variable indicate significant differences (α = 0.05) using Tukey’s HSD.
In the nodule activity experiment, no interaction of N form (arginine and NH4NO3) and inoculation was observed for shoot, root, or total seedling biomass. Although N form was not significant for any biomass parameter, inoculation was (all p ≤ 0.0088). For arginine and NH4NO3, including inoculation increased total biomass (Figure 1). N form and inoculation significantly interacted (all p ≤ 0.0051) to affect shoot, root, and total seedling N isotope ratio (δ15N); δ15N values were lower when seedlings were inoculated but the magnitude of difference was greater in seedlings supplied arginine than for those supplied NH4NO3 (Figure 2). No significant N form × inoculation interaction was observed for shoot or root N content and the main effect of N form was not significant. Inoculation was, however, significant for N content of shoot and root (p < 0.0001), with inoculated seedlings having significantly more N (Figure 2).
Figure 2 Nitrogen isotope ratio (δ15N) and N content in shoots and roots of lupine seedlings 56 days after planting in the greenhouse. Seedlings were supplied with either arginine (A and B) or ammonium-nitrate (C and D) after either being inoculated (white boxes) or not inoculated (gray boxes) with rhizobia. Vertical boxes represent approximately 50% of the observations and lines extending from each box are the upper and lower 25% of the distribution. The solid horizontal line in the center of each box is the median value and the dotted line is the mean.
In the first experiment, N and inoculation significantly interacted to affect final measurements of seedling height, leaf biomass, stem biomass, shoot biomass, and root biomass (Table 4). The pattern of the interaction was orderly and similar: without inoculation, seedlings supplied N were taller and had greater biomass than those not supplied N; adding inoculation resulted in additional growth to seedlings either supplied N or not supplied N, but the increase in growth was much greater for those supplied N (Table 4; Figure 1).
Table 4 Mean morphological parameters of black locust seedlings inoculated (I) with (+) or without (−) rhizobia and supplied with different nitrogen (N) sources [none, ammonium (NH4), nitrate (NO3), ammonium nitrate (NH4NO3), or arginine (A)] for 12 weeks (n = 8). Main effect (ME) means are presented in italics. Different letters within a dependent variable indicate significant differences (α = 0.05) using Tukey’s HSD.
Nitrogen significantly affected every final measured response variable except S:R (Table 4). In general, N-supplied seedlings had significantly more height, RCD, leaf biomass, and stem, shoot, nodule, and root biomass than seedlings not supplied N, but values among N forms were similar. For N-supplied seedlings, N form did not affect seedling size but it did significantly affect nodule biomass, with NH4+ producing the most, followed by NO3−, NH4NO3, and arginine; the no-N treatment yielded the least amount of nodule biomass. Similar to N, inoculation significantly affected every final measured response variable except S:R (Table 4), with inoculated seedlings having greater values than their noninoculated cohorts.
The N × inoculation interaction was significant for leaf N concentration (Table 5). Noninoculated and inoculated seedlings grown without N had similar leaf N concentration, but for seedlings supplied with N, noninoculated seedlings had significantly greater N concentration than inoculated ones. N significantly affected N concentration in leaves and stems but not roots: leaf N concentration was greatest for seedlings not supplied N followed by those supplied with NH4+ or NH4NO3, with those supplied with arginine or NO3− producing the lowest leaf N concentration. In general, no-N seedlings had significantly larger stem N concentration than N-supplied seedlings, but seedling stem N concentrations for seedlings supplied with N were similar regardless of N form. Inoculation had no effect on N concentration of leaves, stems, or roots (Table 5).
Table 5 Mean nitrogen (N) concentrations and contents of black locust seedlings inoculated (I) with (+) or without (−) rhizobia and supplied with different nitrogen (N) sources [none, ammonium (NH4), nitrate (NO3), ammonium nitrate (NH4NO3), or arginine (A)] for 12 weeks (n = 8). Main effect (ME) means are presented in italics. Different letters within a dependent variable indicate significant differences (ခα = 0.05) using Tukey’s HSD.
Nitrogen and inoculation interacted to affect root N content (Table 5). The pattern followed the interaction observed for seedling growth: without N, noninoculated and inoculated seedlings had the same root N content, whereas with N, inoculated seedlings had more N than their noninoculated cohorts. Nitrogen significantly affected leaf, stem, and root N content. In general, N-supplied seedlings had significantly higher N content in leaves, stems, and roots than those not receiving N, but seedling N content was similar regardless of N form. Inoculation significantly increased N content of leaves, stems, and roots (Table 5).
In the nodule activity experiment, no interaction of N form (arginine and NH4NO3) and inoculation was observed for shoot, root, or total seedling biomass, but N form and inoculation were both significant (p ≤ 0.0151), except for stem biomass. Regardless of N form, inoculation increased total biomass about 35%. The interaction of N form and inoculation was significant for all tissue types and total seedling δ15N (all p ≤ 0.0212); δ15N decreased with inoculation (Figure 3). For N content, the interaction was not significant for any tissue type or total seedling, N form had no effect on total seedling N content, and inoculation significantly (p < 0.0013) increased N content (Figure 3).
Figure 3 Nitrogen isotope ratio (δ15N) and N content in leaves, stems, and roots of black locust seedlings 70 days after planting in the greenhouse. Seedlings were supplied with either arginine (A and B) or ammonium-nitrate (C and D) after either being inoculated (white boxes) or not inoculated (gray boxes) with rhizobia. Vertical boxes represent approximately 50% of the observations and lines extending from each box are the upper and lower 25% of the distribution. The solid horizontal line in the center of each box is the median value and the dotted line is the mean.
In this study, noninoculated lupine and black locust seedlings supplied organic or inorganic N had similar growth; moreover, these seedlings grew significantly larger than their cohorts not supplied N (Tables 2 and 4). Inoculation with rhizobia, with or without N supply, improved seedling growth and increased N content compared to noninoculated seedlings (Tables 2‒5), similar to the response noted for other legume plants (Dan et al., 1993; Adgo and Schulze, 2002; Diabate et al., 2005; Jayakumar and Tan, 2006; Dumroese et al., 2009; Gan et al., 2010; Namvar et al., 2011). Inoculated black locust seedlings supplied N had 157% more biomass than those not supplied N (Uselman et al., 2000). We noted, however, that inoculation decreased the S:R for lupine seedlings (Table 2) but not for black locust seedlings (Table 4), suggesting that the subsequent allocation response of the plant to improved N status may be either species or plant form dependent. Although we observed minor and sporadic nodulation on noninoculated plants, we did not evaluate their N-fixing availability; other research suggests that these types of nodules may not actively fix atmospheric N (Tirichine et al., 2006).
It has long been proposed that mineral N inhibits nodulation, nodule growth, and activity in legumes (Imsande, 1986; Streeter and Wong, 1988; Guo et al., 1992; Bollman and Vessey, 2006; Camargos and Sodek, 2010). While low N rates improved nodule formation in black locust (Rohm and Werner, 1991), N rates exceeding 0.84 mg N week−1 reduced nodule number and nodule biomass (Roberts et al., 1983; Rohm and Werner, 1991). Our results, however, show that, although N form did not affect seedling size, N supplied at 2 mg N week−1 significantly enhanced nodule formation on black locust compared to seedlings not supplied N (Table 4); NH4+ seemed to favor nodulation the most, confirming that the impact of NH4+ on symbiosis is weaker than that observed for NO3− (Bollman and Vessey, 2006). Our data suggests that more robust N supply may not preclude nodule formation, similar to results observed in some species (Peoples et al., 1995; Bado et al., 2006; Dumroese et al., 2009). A similar result was, however, not apparent on lupine seedlings (Table 2). Nodulation on lupine was unaffected by N form, but for black locust, N supplied as NH4+ was superior to arginine, suggesting that the nodulation response may be plant species dependent (Manhart and Wong, 1980; Harper and Gibson, 1983).
Although increasing N rates have been associated with decreasing nodule number on black locust (Roberts et al., 1983; Rohm and Werner, 1991), the nitrogenase activity of the nodules was either unaffected (Roberts et al., 1983) or increased (Rohm and Werner, 1991). Our results of the N isotope ratio in different parts of lupine and black locust seedlings supplied with organic or inorganic N showed that δ15N was decreased in different tissue types in inoculated seedlings relative to noninoculated seedlings, indicating dilution within the seedling caused by additions of atmospheric N-fixation within the nodules (Hardarson and Danso, 1993). Indeed, our seedlings had 3× (black locust; Table 5) to 9× (lupine; Table 3) more N content when inoculation was combined with additions of N.
Thus, it is not surprising that N supply and inoculation significantly interacted to affect morphology and root N content of black locust (Tables 4 and 5). The combination of inoculation and access to N promoted more growth (i.e., height, biomass, nodules) than did inoculation alone. Despite higher N contents in inoculated black locust seedlings supplied N, their leaf N concentration was lower than noninoculated seedlings supplied N. This lower N concentration is due to dilution (Salifu and Jacobs, 2006) that likely occurred in these rapidly growing plants during the three weeks between the final application and harvest. Thus, for black locust, the combination of inoculation and access to N improved growth more than either factor alone. Uselman et al. (2000) also found that the combination of inoculation and N supply produced larger seedlings than inoculation alone. For lupine, we were unable to detect a significant N supply by inoculation interaction (Tables 2 and 3) but N content data (Table 3) followed a pattern similar to that for black locust. For noninoculated black locust seedlings, we noted N contents that exceeded those expected by supply of N alone. The additional N may originate from the substrate we used. Peat initially contains appreciable N that may have subsequently been mineralized and available for plant uptake. In their review, Cheng and Kuzyakov, (2005) report that rapid soil wetting-drying cycles tend to increase mineral soil organic matter mineralization; this condition is common in small-volume seedling containers such as the ones we used, especially as the seedlings became larger (Landis et al., 1989). Moreover, the presence of roots can lead to a threefold increase in mineralization (Cheng and Kuzyakov, 2005).
Many plant species (e.g., Persson and Näsholm, 2001; Metcalfe et al., 2011), including trees (e.g., Wei et al., 2015; Uscola et al., 2017) show the capacity for intact uptake of amino acids, especially through their mycorrhizal networks (McFarland et al., 2010; Whiteside et al., 2012). Microbes utilize more amino acid N in the short term (e.g., Warren, 2009; Farrell et al., 2014). In the longer term (more than 2 days), seedlings may capitalize on the rapid cycling of amino acid N through the microbial biomass (Harrison et al., 2008; Warren, 2009), which may explain the results of studies in greenhouses showing that plants grown on amino acid N performed as well as those given inorganic N (Öhlund and Näsholm, 2001; Gruffman et al., 2012). Amino acid application in field settings, however, could alter the plant-microorganism balance due to an enhanced carbon environmnet (Schobert et al., 1988), leading to unexpected varations in N taken up by plants, which may explain the reduced growth with amino acids observed by Wilson et al. (2013). Our results showed that seedlings of both broadleaved plant species supplied with arginine grew as well as seedlings given inorganic N, although we did not measure if the organic N was taken up intact by either species.
Although amino acids are suggested to have a direct positive effect on root growth (Cambui et al., 2011), in our study, neither root growth or S:R of lupine or black locust seedlings were affected (Tables 2 and 4). The stimulatory effect on root growth is thought to be associated with development of mycorrhizal symbioses (Cambui et al., 2011), an aspect that we did not examine in our study.
Successful use of seedlings in forest restoration activities requires that they meet specific target criteria needed to overcome environmental limitations on their intended outplanting site (Dumroese et al., 2016). Fertilization in the nursery can provide a more rapid method for obtaining target plant size than the sole use of an N-fixing symbiont on a leguminous tree and can be done within current operational standards (Dumroese et al., 2009). This is important because nursery efficiency (e.g., plant growth per unit time) is one of many factors supporting successful nursery operation (Dumroese et al., 2011). In addition, Villar-Salvador et al. (2008) report that seedlings supplied high rates of fertilizer during nursery production grew larger and had improved morphological and physiological traits (i.e., greater biomass, root growth capacity, photosynthetic rates, and N concentrations) than their cohorts supplied low rates of fertilizer regardless of rhizobial inoculation; this translated into more survival and growth after outplanting. Our results demonstrate that robust fertilization need not sacrifice development of nodules capable of N-fixation.
In summary, we grew a forb and tree species using inorganic and organic N forms with and without genus-specific (lupine) or species-specific (black locust) rhizobia inoculation. We found that seedlings grew equally well with either N form; nodule biomass in lupine was unaffected by N form, whereas in black locust, nodule biomass in NH4+-supplied seedlings was greater than that in arginine-supplied seedlings, an apparent species-specific response; and that N-fixation readily occurred in the presence of high levels of N supply, leading to enhance seedling N content. Our results suggest that these potentially important species for forest restoration activities can be produced in nurseries to acceptable morphological targets using robust levels of either organic or inorganic forms of N and have functioning symbionts as well.
PZ, KD, and JP shared equally in conceiving the research, designing the experiment, interpreting results, and drafting the manuscript. RD and JP provided research funding and technical expertise in seedling production. PZ tended and sampled seedlings. KZ and JP performed the data analyses.
This study was funded by the Fundamental Research Funds for the Central Universities (China; 2572019CP16), U.S. Department of Agriculture, Forest Service (USFS) Rocky Mountain Research Station (RMRS), and the USFS National Center for Reforestation, Nurseries, and Genetic Resources.
The authors declare that the research was conducted in the absence of any commercial or financial relationships that could be construed as a potential conflict of interest.
We thank Jasmine Drapeau and Nathan Robertson at the USFS Coeur d’Alene Nursery for providing lupine seeds, Thomas Wacek at Plant Probiotics Company for providing rhizobia inoculants, and USFS RMRS employees Katherine McBurney and Charles Eckman for assistance with sample processing. We also thank the associate editor and reviewers for their comments.
Adgo, E., Schulze, J. (2002). Nitrogen fixation and assimilation efficiency in Ethiopian and German pea varieties. Plant Soil 239, 291–299. doi: 10.1023/A:1015048331366
Antos, J. A., Halpern, C. B. (1997). Root system differences among species: implications for early successional changes in forest of western Oregon. Am. Midl. Nat. 138, 97–108. doi: 10.2307/2426658
Bado, B. V., Bationo, A., Cescas, M. P. (2006). Assessment of cowpea and groundnut contributions to soil fertility and succeeding sorghum yields in the Guinean savannah zone of Burkina Faso (West Africa). Biol. Fertil. Soils 43, 171–176. doi: 10.1007/s00374-006-0076-7
Bigg, W. L., Daniel, T. W. (1978). Effects of nitrate, ammonium and pH on the growth of conifer seedlings and their production of nitrate reductase. Plant Soil 50, 371–385. doi: 10.1007/BF02107186
Bollman, M. I., Vessey, J. K. (2006). Differential effects of nitrate and ammonium supply on nodule initiation, development, and distribution on roots of pea (Pisum sativum). Can. J. Bot. 84, 893–903. doi: 10.1139/b06-027
Camargos, L. S., Sodek, L. (2010). Nodule growth and nitrogen fixation of Calopogonium mucunoides L. show low sensitivity to nitrate. Symbiosis 51, 167–174. doi: 10.1007/s13199-010-0063-5
Cambui, C. A., Svennerstam, H., Gruffman, L., Nordin, A., Ganeteg, U., Näsholm, T. (2011). Patterns of plant biomass partitioning depend on nitrogen source. PloS One 6, e19211. doi: 10.1371/journal.pone.0019211
Cheng, W., Kuzyakov, Y. (2005). “Root effects on soil organic matter decomposition,” in Roots and Soil Management: Interactions between Roots and the Soil, Agronomy Monograph 48. Eds. Wright, S., Zobel, R. (Madison, WI: American Society of Agronomy), 119–143.
Christou, M., Avramides, E. J., Jones, D. L. (2006). Dissolved organic nitrogen dynamics in a Mediterranean vineyard soil. Soil Biol. Biochem. 38, 2265–2277. doi: 10.1016/j.soilbio.2006.01.025
Dan, T., Keyser, H. H., Singleton, P. W. (1993). Response of tree legumes to rhizobial inoculation in relation to the population density of indigenous rhizobia. Soil Biol. Biochem. 25, 75–81. doi: 10.1016/0038-0717(93)90244-6
Diabate, M., Munive, A., de Faria, S. M., Ba, A., Dreyfus, B., Galiana, A. (2005). Occurrence of nodulation in unexplored leguminous trees native to the West African tropical rainforest and inoculation response of native species useful in reforestation. New Phytol. 166, 231–239. doi: 10.1111/j.1469-8137.2005.01318.x
Doede, D. L. (2005). Genetic variation in broadleaf lupine Lupinus latifolius on the Mt Hood National Forest and implications for seed collection and deployment. Native Plants J. 6, 36–48. doi: 10.1353/npj.2005.0018
Dumroese, R. K., Jacobs, D. F., Davis, A. S. (2009). Inoculating Acacia koa with Bradyrhizobium and applying fertilizer in the nursery: effects on nodule formation and seedling growth. HortScience 44, 443–446. doi: 10.21273/HORTSCI.44.2.443
Dumroese, R. K., Davis, A. S., Jacobs, D. F. (2011). Nursery response of Acacia koa seedlings to container size, irrigation method, and fertilization rate. J. Plant Nutr. 34, 877–887. doi: 10.1080/01904167.2011.544356
Dumroese, R. K., Montville, M. E., Pinto, J. R. (2015). Using container weights to determine irrigation needs: a simple method. Native Plants J. 16, 67–71. doi: 10.3368/npj.16.1.67
Dumroese, R. K., Landis, T. D., Pinto, J. R., Haase, D. L., Wilkinson, K. M., Davis, A. S. (2016). Meeting forest restoration challenges: using the Target Plant Concept. Reforesta 1, 37‒52. doi: 10.21750/REFOR.1.03.3
Fei, H., Vessey, J. K. (2003). Involvement of cytokinin in the stimulation of nodulation by low concentrations of ammonium in Pisum sativum L. Physiol. Plant. 118, 447–455. doi: 10.1034/j.1399-3054.2003.00123.x
Farrell, M., Prendergast-Miller, M., Jones, D. L., Hill, P. W., Condron, L. M. (2014). Soil microbial organic nitrogen uptake is regulated by carbon availability. Soil Biol. Biochem. 77, 261–267. doi: 10.1016/j.soilbio.2014.07.003
Franklin, O., Cambui, C. A., Gruffman, L., Palmroth, S., Oren, R., Näsholm, T. (2017). The carbon bonus of organic nitrogen enhances nitrogen use efficiency of plants. Plant Cell Environ. 40, 25–35. doi: 10.1111/pce.12772
Gan, Y. T., Johnston, A. M., Knight, J. D., McDonald, C., Stevenson, C. (2010). Nitrogen dynamics of chickpea: effects of cultivar choice, N fertilization, Rhizobium inoculation, and cropping systems. Can. J. Plant Sci. 90, 655–666. doi: 10.4141/CJPS10019
Goicoechea, N., Merino, S., Sanchez-Diaz, M. (2005). Management of phosphorus and nitrogen fertilization to optimize Anthyllis-Glomus-Rhizobium symbiosis for revegetation of desertification semiarid areas. J. Plant Nutr. 27, 1395–1413. doi: 10.1081/PLN-200025850
Gruffman, L., Ishida, T., Nordin, A., Näsholm, T. (2012). Cultivation of Norway spruce and Scots pine on organic nitrogen improves seedling morphology and field performance. Forest Ecol. Manag. 276, 118–124. doi: 10.1016/j.foreco.2012.03.030
Gruffman, L., Palmroth, S., Näsholm, T. (2013). Organic nitrogen uptake of Scots pine seedlings is independent of current carbohydrate supply. Tree Physiol. 33, 590–600. doi: 10.1093/treephys/tpt041
Gulden, R. H., Vessey, J. K. (1997). The stimulating effect of ammonium on nodulation in Pisum sativum L. is not long lived once ammonium supply is discontinued. Plant Soil 195, 195–205. doi: 10.1023/A:1004249017255
Guo, R., Silsbury, J. H., Graham, R. D. (1992). Effect of four nitrogen compounds on nodulation and nitrogen fixation in faba bean, white lupin and medic plants. Aust. J. Plant Physiol. 19, 501–508. doi: 10.1071/PP9920501
Halverson, N. M., Lesher, R., McClure, R. H., Riley, J., Topik, C. (1986). Major indicator shrubs and herbs on national forests of western Oregon and southwestern Washington. Portland, OR: U.S. Department of Agriculture, Forest Service, Pacific Northwest Region, R6-TM-229, 180.
Hardarson, G., Danso, S. K. A. (1993). Methods for measuring biological nitrogen-fixation in grain legumes. Plant Soil 152, 19–23. doi: 10.1007/BF00016330
Harper, J. E., Gibson, A. H. (1983). Differential nodulation tolerance to nitrate among legume species. Crop Sci. 24, 797–801. doi: 10.2135/cropsci1984.0011183X002400040040x
Harrison, K. A., Bol, R., Bardgett, R. D. (2008). Do plant species with different growth strategies vary in their ability to compete with soil microbes for chemical forms of nitrogen? Soil Biol. Biochem. 40, 228–237. doi: 10.1016/j.soilbio.2007.08.004
Hawkins, B. J., Robbins, S. (2010). pH affects ammonium, nitrate and proton fluxes in the apical region of conifer and soybean roots. Physiol. Plant. 138, 238–247. doi: 10.1111/j.1399-3054.2009.01317.x
Imsande, J. (1986). Inhibition of nodule development in soybean by nitrate or reduced nitrogen. J. Exp. Bot. 37, 348–355. doi: 10.1093/jxb/37.3.348
Inselsbacher, E., Näsholm, T. (2012). The below-ground perspective of forest plants: soil provides mainly organic nitrogen for plants and mycorrhizal fungi. New Phytol. 195, 329–334. doi: 10.1111/j.1469-8137.2012.04169.x
Jayakumar, P., Tan, T. K. (2006). Variations in the responses of Acacia mangium to inoculation with different strains of Bradyrhizobium sp. under nursery conditions. Symbiosis 41, 31–37.
Kielland, K. (1994). Amino-acid-absorption by Arctic plants: implications for plant nutrition and nitrogen cycling. Ecology 75, 2373–2383. doi: 10.2307/1940891
Landis, T. D., Tinus, R. W., McDonald, S. E., Barnett, J. P. (1989). Seedling nutrition and irrigation, volume 4. in The Container Tree Nursery Manual. Agriculture Handbook 674, (Washington, DC: U.S. Department of Agriculture, Forest Service), 119 p.
Lucinski, R., Polcyn, W., Ratajczac, L. (2002). Nitrate reduction and nitrogen fixation in symbiotic association Rhizobium-legumes. Acta Biochim. Pol. 49, 537–546.
Manhart, J. R., Wong, P. P. (1980). Nitrate effect on nitrogen-fixation (acetylene reduction)—activities of legume root-nodules induced by rhizobia with varied nitrate reductase activities. Plant Physiol. 65, 502–505. doi: 10.1104/pp.65.3.502
McFarland, J. W., Ruess, R. W., Kielland, K., Pregitzer, K., Hendrick, R., Allen, M. (2010). Cross-ecosystem comparisons of in situ plant uptake of amino acid-N and NH4+. Ecosystems 13, 177–193. doi: 10.1007/s10021-009-9309-6
Metcalfe, R. J., Nault, J., Hawkins, B. J. (2011). Adaptations to nitrogen form: comparing inorganic nitrogen and amino acid availability and uptake by four temperate forest plants. Can. J. Forest Res. 41, 1626–1637. doi: 10.1139/x11-090
Namvar, A., Sharifi, R. S., Sedghi, M., Zakaria, R. A., Khandan, T., Eskandarpour, B. (2011). Study on the effects of organic and inorganic nitrogen fertilizer on yield, yield components, and nodulation state of chickpea (Cicer arietinum L.). Commun. Soil Sci. Plan. 42, 1097–1109. doi: 10.1080/00103624.2011.562587
Öhlund, J., Näsholm, T. (2001). Growth of conifer seedlings on organic and inorganic nitrogen sources. Tree Physiol. 21, 1319–1326. doi: 10.1093/treephys/21.18.1319
Öhlund, J., Näsholm, T. (2002). Low nitrogen losses with a new source of nitrogen for cultivation of conifer seedlings. Environ. Sci. Technol. 36, 4854–4859. doi: 10.1021/es025629b
Öhlund, J., Näsholm, T. (2004). Regulation of organic and inorganic nitrogen uptake in Scots pine (Pinus sylvestris) seedlings. Tree Physiol. 24, 1397–1402. doi: 10.1093/treephys/24.12.1397
Olson, D. F., Jr., Karrfalt, R. P. (2008). “Robinia L.” in The Woody Plant Seed Manual. Agriculture Handbook 727, Eds. Bonner, F. T., Karrfalt, R. P. (Washington, DC: U.S. Department of Agriculture, Forest Service), 969–973.
Peoples, M. B., Ladha, J. K., Herridge, D. F. (1995). Enhancing legume N2 fixation through plant and soil management. Plant Soil 174, 83–101. doi: 10.1007/978-94-011-0055-7_4
Persson, J., Hogberg, P., Ekblad, A., Hogberg, M. N., Nordgren, A., Näsholm, T. (2003). Nitrogen acquisition from inorganic and organic sources by boreal forest plants in the field. Oecologia 137, 252–257. doi: 10.1007/s00442-003-1334-0
Persson, J., Näsholm, T. (2001). Amino acid uptake: a widespread ability among boreal forest plants. Ecol. Lett. 4, 434–438. doi: 10.1046/j.1461-0248.2001.00260.x
Roberts, D. R., Zimmerman, R. W., Stringer, J. W., Carpenter, S. B. (1983). The effects of combined nitrogen on growth, nodulation, and nitrogen fixation of black locust seedlings. Can. J. Forest Res. 13, 1251–1254. doi: 10.1139/x83-165
Rohm, M., Werner, D. (1991). Nitrate levels affect the development of the black locust‒Rhizobium symbiosis. Trees-Struct. Funct. 5, 227–231. doi: 10.1007/BF00227529
Rys, G. J., Phung, T. (1984). Effect of nitrogen form and counterion on establishment of the Rhizobium trifolii–Trifolium repens symbiosis. J. Exp. Bot. 35, 1811–1819. doi: 10.1093/jxb/35.12.1811
Salifu, K. F., Jacobs, D. F. (2006). Characterizing fertility targets and multi-element interactions in nursery culture of Quercus rubra seedlings. Ann. For. Sci. 63, 231–237. doi: 10.1051/forest:2006001
Schobert, C., Köckenberger, W., Komor, E. (1988). Uptake of amino acids by plants from the soil: a comparative study with castor bean seedlings grown under natural and axenic soil conditions. Plant Soil 109, 181–188. doi: 10.1007/BF02202082
Silsbury, J. H., Catchpoole, D. W., Wallace, W. (1986). Effects of nitrate and ammonium on nitrogenase (C2H2 reduction) activity of swards of subterranean clover, Trifolium subterraneum L. Aust. J. Plant Physiol. 13, 257–273. doi: 10.1071/PP9860257
Streeter, J. G., Wong, P. P. (1988). Inhibition of legume nodule formation and N2 fixation by nitrate. CRC Crit. Rev. Plant Sci. 7, 1–23. doi: 10.1080/07352688809382257
Svenning, M. M., Junttila, O., Macduff, J. H. (1996). Differential rates of inhibition of N2 fixation by sustained low concentrations of NH4 and NO3 in northern ecotypes of white clover (Trifolium repens L.). J. Exp. Bot. 47, 729–738. doi: 10.1093/jxb/47.6.729
Tirichine, L., Imaizumi-Anraku, H., Yoshida, S., Murakami, Y., Madsen, L. H., Miwa, H., et al. (2006). Deregulation of a Ca2+/calmodulin-dependent kinase leads to spontaneous nodule development. Nature 441, 1153–1156. doi: 10.1038/nature04862
Uscola, M., Villar-Salvador, P., Oliet, J., Warren, C. R. (2017). Root uptake of inorganic and organic N chemical forms in two coexisting Mediterranean forest trees. Plant Soil 415, 387–392. doi: 10.1007/s11104-017-3172-6
Uselman, S. M., Qualls, R. G., Thomas, R. B. (2000). Effects of increased atmospheric CO2, temperature, and soil N availability on root exudation of dissolved organic carbon by a N-fixing tree (Robinia pseudoacacia L.). Plant Soil 222, 191–202. doi: 10.1023/A:1004705416108
Valkov, V. T., Rogato, A., Alves, L. M., Sol, S., Noguero, M., Léran, S., et al. (2017). The nitrate transporter family protein LjNPF8.6 controls the N-fixing nodule activity. Plant Physiol. 175, 1269‒1282. doi: 10.1104/pp.17.01187
Vessey, J. K., Waterer, J. (1992). In search of the mechanism of nitrate inhibition of nitrogenase activity in legume nodules: recent developments. Physiol. Plant. 84, 171–176. doi: 10.1111/j.1399-3054.1992.tb08780.x
Villar-Salvador, P., Valladares, F., Domínguez-Lerena, S., Ruiz-Díez, B., Fernäández-Pascual, M., Delgado, A., et al. (2008). Functional traits related to seedling performance in the Mediterranean leguminous shrub Retama sphaerocarpa: insights from a provenance, fertilization, and rhizobial noculation study. Environ. Exp. Bot. 64, 145–154. doi: 10.1016/j.envexpbot.2008.04.005
Warren, C. R. (2009). Uptake of inorganic and amino acid nitrogen from soil by Eucalyptus regnans and Eucalyptus pauciflora seedlings. Tree Physiol. 29, 401–409. doi: 10.1093/treephys/tpn037
Waterer, J. G., Vessey, J. K. (1993a). Effect of low static nitrate concentrations on mineral nitrogen uptake, nodulation, and nitrogen fixation in field pea. J. Plant Nutr. 16, 1775–1789. doi: 10.1080/01904169309364649
Waterer, J. G., Vessey, J. K. (1993b). Nodulation response of autoregulated or NH4+-inhibited pea (Pisum sativum) after transfer to stimulatory (low) concentrations of NH4+. Physiol. Plant. 88, 460–466. doi: 10.1111/j.1399-3054.1993.tb01360.x
Wei, L., Chen, C., Yu, S. (2015). Uptake of organic nitrogen and preference for inorganic nitrogen by two Australian native Araucariaceae species. Plant Ecol. Divers. 8, 259–264. doi: 10.1080/17550874.2013.871656
Whiteside, M. D., Garcia, M. O., Treseder, K. K. (2012). Amino acid uptake in arbuscular mycorrhizal plants. PLoS One 7, e47643. doi: 10.1371/journal.pone.0047643
Wilson, A. R., Nzokou, P., Guney, D., Kulac, S. (2013). Growth response and nitrogen use physiology of Fraser fir (Abies fraseri), red pine (Pinus resinosa), and hybrid poplar under amino acid nutrition. New For. 44, 281–295. doi: 10.1007/s11056-012-9317-9
Yao, B., Cao, J., Zhao, C., Rengel, Z. (2011). Influence of ammonium and nitrate supply on growth, nitrate reductase activity and N-use efficiency in a natural hybrid pine and its parents. J. Plant Ecol. 4, 275–282. doi: 10.1093/jpe/rtq033
Yashima, H., Fujikake, H., Yamazaki, A., Ito, S., Sato, T., Tewari, K., et al. (2005). Long-term effect of nitrate application from lower part roots on nodulation and N2 fixation in upper part roots of soybean (Glycine max (L.) Merr.) in two-layered pot experiment. Soil Sci. Plant Nutr. 7, 981–990. doi: 10.1111/j.1747-0765.2005.tb00137.x
Keywords: amino acid, arginine, inorganic nitrogen, isotopic nitrogen, Lupinus latifolius, nitrogen fixation, organic nitrogen, Robinia pseudoacacia
Citation: Zhang P, Dumroese RK and Pinto JR (2019) Organic or Inorganic Nitrogen and Rhizobia Inoculation Provide Synergistic Growth Response of a Leguminous Forb and Tree. Front. Plant Sci. 10:1308. doi: 10.3389/fpls.2019.01308
Received: 23 January 2019; Accepted: 19 September 2019;
Published: 22 October 2019.
Edited by:
Heike Bücking, South Dakota State University, United StatesReviewed by:
Corina Carranca, National Institute of Agricultural and Veterinary Research (INIAV), PortugalAt least a portion of this work is authored by R. Kasten Dumroese and Jeremiah R. Pinto on behalf of the U.S. Government and, as regards Dr. Dumroese, Dr. Pinto, and the U.S. Government, is not subject to copyright protection in the United States. Foreign and other copyrights may apply. This is an open-access article distributed under the terms of the Creative Commons Attribution License (CC BY). The use, distribution or reproduction in other forums is permitted, provided the original author(s) and the copyright owner(s) are credited and that the original publication in this journal is cited, in accordance with accepted academic practice. No use, distribution or reproduction is permitted which does not comply with these terms.
*Correspondence: R. Kasten Dumroese, a2FzLmR1bXJvZXNlQHVzZGEuZ292
Disclaimer: All claims expressed in this article are solely those of the authors and do not necessarily represent those of their affiliated organizations, or those of the publisher, the editors and the reviewers. Any product that may be evaluated in this article or claim that may be made by its manufacturer is not guaranteed or endorsed by the publisher.
Research integrity at Frontiers
Learn more about the work of our research integrity team to safeguard the quality of each article we publish.