- 1Dipartimento di Scienze Agro-Ambientali e Territoriali, Università degli Studi di Bari Aldo Moro, Bari, Italy
- 2Department of Irrigation, Centro de Edafología y Biología Aplicada del Segura (CEBAS-CSIC), Murcia, Spain
The 70% worldwide surface of olive orchards is irrigated. The evaluation of non-conventional water resources and water-saving techniques has gained importance during the last decades in arid and semiarid environments. This study evaluated the effects of irrigation with two water sources: low-cost water DEsalination and SEnsoR Technology (DESERT) desalinated water (DW) ECw ∼1 dS m−1) and reclaimed water (RW) (ECw ∼ 3 dS m−1) combined with two irrigation strategies: full irrigation (FI) (100% of ETc) and regulated deficit irrigation (RDI, 50% of ETc) on fruit yield, ripening indices, and oil yield and quality of olive trees cv Arbosana planted in Mediterranean conditions. Our results showed that RW without water restrictions increased the fruit yield by 35% due to a slight increase in the fruit weight and, mainly, to a greater fruit set than the control trees; although this did not result in a higher oil yield (g tree−1) since the oil content per fruit dry weight was reduced. The RDI strategy did not decrease the fruit yield despite the fact that olive weight tended to decrease, and it increased the oil yield by ∼14.5%. The combination of both stresses (RW and RDI) neither decreased the fruit yield; however, it significantly reduced oil yield (25% less in 2018) since oil content per fruit dry weight was strongly reduced (40%) compared to control trees. Both RDI treatments, regardless water source, determined acidity levels in olive paste lower than in FI treatments; however, it reduced oil extractability and fatty yield. The finding about oil quality indicated that olive exposure to RW, regardless of the water amount, decreased oil quality mainly due to the reduction of oleic acid and the increase of C18:2/C18:3 ratio and peroxides; on the contrary, both RW and RDI improved the total polyphenols. In all cases, the parameters met the legislation. In short, with appropriate management, RW and RDI have great potential to manage oil olive production; nevertheless, studies subjected to long-term use of these techniques should be experienced to ensure the sustainability of oil yields and quality.
Introduction
Water is essential for agricultural production and food security. Our freshwater resources are dwindling at an alarming rate. It is estimated that by 2025, around 2 billion people will be affected by absolute water scarcity (Riemenschneider et al., 2016). Thus, growing water scarcity is now one of the leading challenges for sustainable development. This challenge will become more pressing as the world’s population continues to grow, their living standards increase, diets change, and the effects of climate change intensify, increasing temperatures across the world. In this context, more frequent and severe droughts are already having an impact on agricultural production, where rising temperatures translate into increased crop water demand.
Agriculture, which is the most water-demanding economic sector worldwide, is both a major cause and casualty of water scarcity. Farming accounts for almost 70% of all water withdrawals, and up to 95% in some developing countries, with freshwater resources heavily stressed by irrigation and food production (FAO, 2018). In Italy, the dimensions of economic loss in the farming sector were predicting losses of 2 billion Euros (EC, 2018) due to the droughts of summer 2017. In particular, Apulia region (southeast of Italy), which exhibits a Mediterranean climate characterized by hot, dry summer, requires a high volume of irrigation water because many hectares of fruit tree crops (olive, grapes, almond, sweet cherry) account for 80% of the region’s irrigated land (Arborea et al., 2017). Besides, extensive exploitation of wells by Apulian regional farmers is causing the progressive salinization and depletion of relevant portions of the regional aquifers reducing the water available for agriculture (Vivaldi et al., 2019).
Thus, techniques for optimizing water productivity as the regulated deficit irrigation (RDI), where water deficits are imposed during phenological periods when the tree is the least sensitive to water stress, and with little impact on fruit yield (Romero-Trigueros et al., 2017) or the use of non-conventional water sources in agriculture as a component of effective water conservation strategies, are required in regions with water scarcity (Romero-Trigueros et al., 2019). Doing so will not prevent a drought from occurring, but it can help in preventing droughts to result in famine and socioeconomic disruption (FAO, 2018).
With respect to non-conventional water sources, reused reclaimed water (RW) is considered non-expensive and reliable, particularly for irrigation in agriculture. It is estimated that, globally, the market for reuse was on the verge of expansion and expected to outpace desalination in the future. It is foreseen that, by 2030, water reuse will represent about 1.7% (26 billion m3 year−1) of the total water use (Global Water Market, 2017). RW usually may contain not only essential nutrients, which are beneficial for crop growth and economy of the growers, but also salts, toxic ions, and micropollutants, which discharge into the environment and can accumulate in the soil and crops over time, affecting plants, soils, and underground water bodies (Romero-Trigueros et al., 2014). For this reason, reducing salt concentrations in these water sources, leading to desalinated water, using technologies, could be an imperative need. Nevertheless, the technologies used must be adequately validated in real crops, ensuring their sustainability, and knowing how it affects the fruit yield and quality. Olive crop is considered moderately tolerant to salinity (Gucci and Tattini, 1997; Erel et al., 2019) with water electrical conductivity (ECw) between 3 and 6 dS m−1, causing no effect to growth or yields (Ayers and Westcot, 1985). In addition, in the Mediterranean region, olive is a major tree crop, and more than 90% of the world’s olive oil is produced. Concretely, in Apulia region, olive is the most representative fruit tree crop irrigated with 383,650 ha cultivated (33 % of total olive orchards in Italy) and with a production of 205,983 t of olive (48% of total Italian production) (ISTAT, 2017; ISMEA, 2018). Salinity tolerance mechanisms of olive trees apparently include a strong ability to exclude potentially toxic ions from above-ground tissues (Kchaou et al., 2010). There are some studies where the saline RW has been used to irrigate olive trees in Mediterranean countries (Greece, Israel, Italy, Spain, Jordan, Egypt, and Tunisia), which reported that the tolerance to salinity depends on the olive varieties. Most of the works evaluated soil properties and leaf nutrients (Aragüés et al., 2005; Ben Rouina et al., 2011; Segal et al., 2011; Petousi et al., 2015; Bourazanis et al., 2016; Erel et al., 2019), root nutrient (Bedbabis et al., 2014), vegetative growth (Kchaou et al., 2010; Ben-Gal et al., 2017), fruit nutrient (Melgar et al., 2009; Batarseh et al., 2011; Bedbabis et al., 2014), and oil yield and quality (Melgar et al., 2009; Ayoub et al., 2016; Tietel et al., 2019).
Regarding the effects of RDI in olive crop, recent works showed that linoleic acid content in olive oil (Hernández et al., 2018), the vegetative growth (Rosecrance et al., 2015; Hernández-Santana et al., 2018), the fruit yield (Gucci et al., 2019) were decreased by water stress. However, a moderate water stress can increase olive oil yield and quality and accelerate fruit maturity (Rosecrance et al., 2015).
To our knowledge, however, nothing has been published about the effects of (i) the irrigation with desalinized water (DW) or of the combination of both water sources (DW and RW) with the RDI strategy and (ii) the strategies on cultivar Arbosana, which is the variety object of study of this work. This cultivar is characterized by early bearing (2nd year after planting), low vigor, and slow canopy growth so that it is the most suitable cultivar to new super high-density olive (SHD) cropping systems (Godini et al., 2011; Rallo and Provenzano, 2013; Vivaldi et al., 2015). SHD olive orchards are spread on over 200,000 ha all over the world on five continents (Olint, 2018). Arbosana, despite being a cultivar widely cultivated has been under-assessed; only Kchaou et al. (2010) studied it in a greenhouse pot experiment with nutrient solution. Indeed, most of works used other olive cultivated varieties, such as Barnea, Leccino (Segal et al., 2011; Ben-Gal et al., 2017; Erel et al., 2019; Tietel et al., 2019), Arbequina (Aragüés et al., 2005; Rosecrance et al., 2015; Hernández et al., 2018; Hernandez-Santana et al., 2018), Chemlali (Ben Rouina et al., 2011; Bedbabis et al., 2014; Bedbabis and Ferrara, 2018), Koroneiki (Petousi et al., 2015; Bourazanis et al., 2016), Nabali Muhassan (Ayoub et al., 2016), Frantoio (Gucci et al., 2019), and Picual (Melgar et al., 2009).
This work intends to assess the effects of the use of desalinated and saline RW combined with two irrigation strategies, full irrigation (FI) and RDI on (i) fruit yield and ripening indices and (ii) oil yield and quality of olive trees cv Arbosana.
Materials and Methods
Experimental Site and Plant Material
The study was conducted at an experimental site located in the southeast of Italy (Bari, Apulia Region) (41°06′41′′N, 16°52′57′′E) (5 m above sea level) during 2017 and 2018. The crop used was 2 years self-rooted olive trees (cv Arbosana) planted on not covered 100-L polyethylene pots (diameter, 50 cm; height, 65 cm). Pots were on the ground with a 1.85 × 2.10 m planting system in rows oriented N-NE to S-SW. The soil texture within the first 90 cm depth was classified as loam (44.78 % sand, 12.32 % clay, and 42.90 % silt) (USDA textural soil classification).
Irrigation Treatments
Two irrigation water sources were examined. First was low-cost water DEsalination and SEnsoR Technology (DESERT) DW, obtained by treating secondary wastewater coming from Bari secondary wastewater treatment plant with ECw 1.2 dS m−1 by ultrafiltration, active carbon, and reverse osmosis till reaching an ECw of 1.0 dS m−1. DESERT is an innovative water desalination and sensor technology compact module for continuously monitoring water quality that has been developed in the framework of the DESERT European project (Water JPI, 2016) with participating partners from Italy, Spain, and Belgium. DESERT technology, to contrast water scarcity and to increase the water quality, enhances the energy savings using solar energy to treat the non-conventional water. The second one is saline RW, which is obtained by mixing the secondary wastewater (ECw 1.2 dS m−1) with the brine produced on the DESERT prototype till reaching an ECw of 3 dS m−1).
Two irrigation treatments were established for each water source. The first treatment was FI treatment throughout the growing season to fully satisfy crop water requirements (100% ETc). The second one was an RDI treatment with an irrigation regime similar to FI, except during the initiation of the first stage of oil accumulation, when it received half the water as applied to the FI (50% ETc). This RDI period was chosen because it corresponded to approximately the end of maximum rate of pit hardening and before the rapid phase of fruit growth and oil accumulation begins, thus avoiding the fruit set period (Stage 1), when olive trees are more sensitive to water stress (Gucci et al., 2019; Rosecrance et al., 2015). The DW-RDI was considered as the control treatment. The irrigation was scheduled on the basis of daily evapotranspiration of the crop (ETc) accumulated during the previous week. ETc values were estimated by multiplying reference evapotranspiration (ET0) (Equation 1) as recommended by FAO (Allen et al., 1998):
where Kr is reduction coefficient (Kr = 0.75) and Kc (0.40 Kcini, 0.90 Kcmid, 0.65 Kcend) is crop coefficient. ET0 was calculated by Penman–Monteith methodology, and all data were provided by a climate station located 100 m far from the experimental platform. The monthly evolution of the ET0 during the experiment is shown in Figure 1. The water was supplied by drip irrigation with three pressure compensated drippers per tree, each with a flow rate of 2 L h−1.
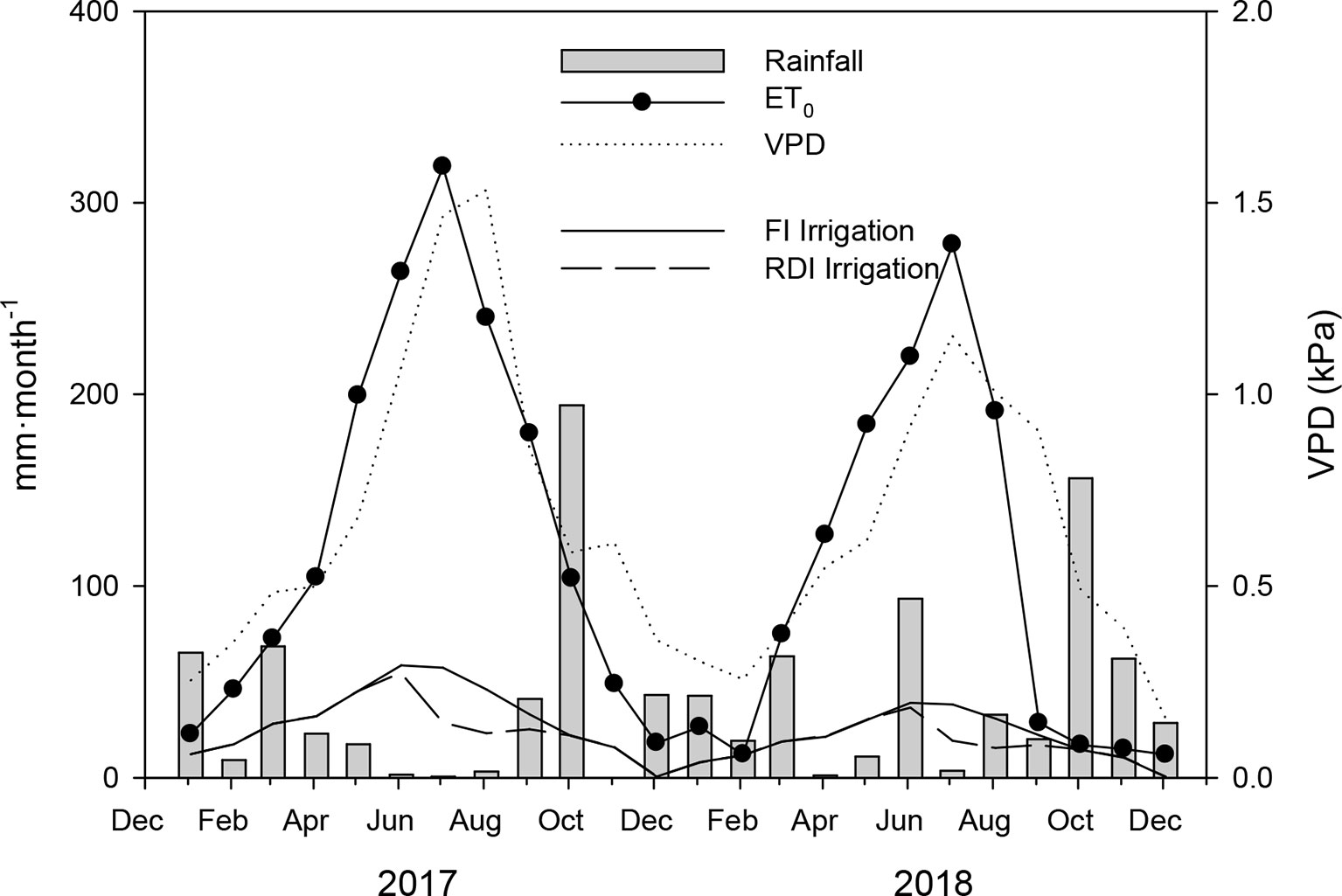
Figure 1 Seasonal evolution of rainfall (mm·month−1), reference evapotranspiration (ET0, mm·month−1), vapor pressure deficit (VPD, kPa), and full (FI) and regulated deficit (RDI) irrigation depths (mm·month−1) during 2017 and 2018.
All trees received the same amount of NPK macronutrients through a drip irrigation system. Integrated pest management and pruning were those commonly used by growers in the area, and no weeds were allowed to develop within the orchard.
Water Quality and Plant Water Status
The inorganic solute content, pH and ECw of each irrigation water source were assessed monthly during the irrigation seasons in 2017 and 2018. The samples were collected in glass bottles, transported in an ice chest to the laboratory, and stored at 5°C before being processed for chemical and physical analyses. The concentrations of macronutrients (N, K, P, Ca, and Mg) and micronutrients including B were determined by inductively coupled plasma optical emission spectrometer (ICP-ICAP 6500 DUO Thermo, England). Anions (Cl−, NO3−, PO43−, and SO42−) were analyzed by ion chromatography with a liquid chromatograph (Metrohm, Switzerland). ECw was determined using a PC-2700 meter (Eutech Instruments, Singapore), and pH was measured with a pH-meter Crison-507 (Crison Instruments S.A., Barcelona, Spain).
Stem water potential (SWP) was determined weekly during the irrigation periods at midday, using a pressure chamber (model 3000, Soil Moisture Equipment Corp., California, USA), according to Scholander et al. (1965), on one fully expanded leaf per tree from the mid-shoot area, which were bagged within foil-covered aluminum envelopes at least 2 h before the measurement (Shackel et al., 1997).
Fruit Yield and Ripening Indices
The harvesting was made on October 31, 2017, and November 21, 2018, at the appropriate ripening stage, when detachment index reached at least 2 N g−1. All olives were manually and separately collected and weighed to determine the fruit yield (g tree−1). The fruit number per tree was calculated by dividing the fruit yield by average single fruit weight.
Fifty olives from each tree of each treatment were randomly sampled immediately after harvest to determine the different fruit ripening indices, following the methodology reported by Camposeo et al. (2013). For fresh weight (FWe), dry weight (DWe), and water content (WC) calculated as (FWe-DWe)·FWe−1·100, fruits were brought to the laboratory to determine the FWe on a digital balance (XS105 Dual Range, Mettler Toledo, Greifensee, Switzerland). Then, these were oven-dried for 48 h at 65°C, cooled for 30 min in a desiccator and again weighted. The detachment index (DI; N g−1) was calculated as: DI = DF·FWe−1 where DF is the detachment force (N) measured using a manual dynamometer (Somfy Tec). Fruit firmness was measured with a penetrometer ADEMVA (mod.TR) using a tip Ø 2 mm on the equatorial zone.
Fruit color was determined as pigmentation index (PI) calculated in Equation (2):
where i is the number of the group, ni is the number of fruits per group, N is the total number of fruits in the sample. The procedure consisted in distributing the sample of olives in six groups, according to the following characteristics: group 0, green skin; group 1, <50% black skin with white flesh; group 2, ≥50% black skin with white flesh; group 3, 100% black skin with white flesh; group 4, 100% black skin with <50% purple flesh; and group 5, 100% black skin and ≥50% purple flesh (0 ≤ PI ≤ 5).
Oil Extraction and Quality
Fatty yield (%), humidity (%), and acidity (%) were determined by near-infrared spectroscopy in part of fruits which were crushed in a hammer mill (FOSS Olivia™, Barcelona, Spain), the resulting olive paste malaxed at 25°C for 30 min (Servili et al., 2007).
To determine oil industrial extractability (%) after olive paste was obtained, the oil was extracted and separated by vertical centrifugation, collected, and left to decant. The oil samples were filtered and stored at 14°C in a dark and cool place in amber glass bottles until analysis. Results were also expressed as oil content per dry and fresh fruit weight (%) and oil yield (goil tree).
Olive oil free acidity (FA), given as percent of oleic acid (C18:1), (% C18:1 per 100 g olive oil), peroxide value (meq O2 kg−1 oil), and UV determinations (K232, K270, and ΔK) were carried out according to the European Union Commission Reg. 61/2011 (EEC, 2011) and International Olive Council (IOC) standard methods. The parameters or extinction coefficients, K232 and K270, have oil absorbance at 232 and 270 nm, respectively, and ΔK was calculated from the absorbances at 232, 268, and 274 nm. Spectrophotometric determinations, K232, K270, and ΔK analyses, were carried out using a Shimadzu UV-1601 spectrophotometer (Shimadzu, Kyoto, Japan).
Profiles of fatty acids methyl esters (FAME) were determined by gas chromatography (EEC, 2011). Olive oil was diluted in hexane (1% oil) and 0.4-ml solution was added to 0.2-ml methanol solution with KOH 2 N. The mixture was vigorously shaken for 1 min and 2 µL of the hexane organic phase was collected for the GC injection. A Shimadzu mod. GC-17A equipped with flame ionization detected (FID) (Shimadzu Italia, Milan, Italy) was used for the analysis. The acquisition software was Class-VP Chromatography data system 4.6 (Shimadzu Italia, Milano, Italy). A FAME capillary column, 60 m, 0.25 mm i.d. with 0.25 mm 50% cyanopropyl-methyl phenyl silicone, was used (Quadrex Corporation, New Heave, USA). Chamber was held at 170°C for 20 min using a rate of 10°C min−1 until 220°C, held for 5 min. Injector temperature and FID temperature was 250°C; carrier gas, Helium; column flow, 2 ml min−1; split ratio, 1/60; injected volume, 20 µl. Peaks identification was performed by comparing retention times of fatty acids with those of pure compounds (mixture of pure methyl esters of fatty acids; Larodan, Malmoe, Sweden) injected in the same condition.
Phenolic compounds were extracted and determined according to Caponio et al. (2015) with slight modifications. Extraction was carried out on 1 g of oil by adding 1 ml of hexane and 5 ml of methanol/water (60:40v/v). After vortexing for 1 min and centrifuging at 4000 rpm for 10 min, the hydroalcoholic phase was recovered and filtered through nylon filters (pore size 0.45 μm, Sigma-Aldrich, Milan, Italy). Then, 100 ml of extract were mixed with 100 ml of Folin–Ciocalteu reagent by Folin and Ciocalteau (1927) and after 4 min, with 800 ml of a 5% (w/v) solution of sodium carbonate. The mixture was stored in the dark for 30 min, and the total phenol content was determined at 750 nm by a Shimadzu UV-1601 spectrophotometer (Shimadzu, Kyoto, Japan). The total phenolic content was expressed as gallic acid equivalents (mg·kg−1).
Chlorophyll and carotenoids determination was carried out by measuring the absorption of the oil/hexane solution (1:1 v/v) at wavelengths of 670 nm for chlorophyll and 450 nm for carotenoids using a Shimadzu UV-1601 spectrophotometer (Shimadzu, Kyoto, Japan) (Minguez et al., 1991).
Experimental Design and Statistical Analysis
A total of 40 trees were used in this study (10 per treatment). The experimental design of each irrigation treatment was five replicates distributed following a completely randomized design. Each replica consisted of two trees. To evaluate the fruit yield and ripening indices, all trees per treatment (10 trees per treatment) were used. For the study of the SWP, oil yield and quality five trees per treatment (one per replicate) were evaluated.
A weighted analysis of variance (ANOVA) followed by Tukey’s test (P ≤ 0.05) was used for assessing differences among treatments. Linear regressions among the different variables measured were calculated. Pearson’s correlation coefficients were used to assess the significance of these relationships. These statistical analyses were performed using SPSS (vers. 23.0 for Windows, SPSS Inc., Chicago, IL). To discriminate significant differences among parameters of different linear regressions (slope and intercept) analysis of covariance (ANCOVA) were performed using Statgraphics software (Statgraphics Plus for Windows Version 4.1). The data also were analyzed using a two-way ANOVA with water quality and water amount as the main factors.
Results
Climatic Data
Climate conditions at the experimental site followed typical Mediterranean patterns, with hot and dry weather from May to September, reaching ET0 values of 318.81 and 278.28 mm·month−1 in 2017 and 2018, respectively, and being mild and wet for the rest of the year. The total rainfall was 467 and 535 mm in 2017 and 2018, respectively. Most of the annual rainfall occurred between September and May (Figure 1). The daily average vapor pressure deficit (VPD) values reached 1.53 and 1.15 kPa in August of 2017 and 2018, respectively (Figure 1). There was a clear different climatic pattern between years. The first year was hotter and more arid than the second one. In particular, during the ripening period (from September to November), the sum of ET0 values was much higher in 2017 than in 2018 (332.5 mm vs 60.6 mm, respectively).
Irrigation Water Quality and Plant Water Status
The results of the chemical analysis of both irrigation water sources used in the experiment, DW and RW, during 2017 and 2018, are presented in Table 1. In general, both waters were characterized by a slight alkaline; within the range of proper irrigation water (Bedbabis et al., 2010). The pH values were weakly higher in RW than in DW, and both within the limits allowed by the D.L. 185/2003. RW had significantly higher salinity than DW (31 ds·m−1 versus 1 ds·m−1). The sodium absorption ratio (SAR) also was higher in RW than DW, and both sources presented values below the limit. The Cl− concentrations were around 200 mg·L−1 for DW and almost double (380 mg·L−1) for RW, exceeding, in this last case, the threshold values indicated in D.L. 185/2003. Likewise, Na presented higher levels in the RW than in the DW, mainly in 2017. Regarding nutrients and elements considered essential for plant growth and development, RW contained higher amounts of NO3−, PO43−, and K, with respect to DW. Similarly, Mg and Ca concentrations in RW were around the double in comparison with DW. Boron, an important micronutrient for olive tree production (Saadati et al., 2013) was found in both sources at a quite similar concentration. The micronutrients as Fe, Mn, and Zn also were slightly higher in RW than in DW (Fe, only in 2018, and Mn presented values about almost double both years). Others toxic heavy metals as Cd, Cr, and Pb were not detected in any of the water sources, whereas the highest Al and Ni levels were in RW.
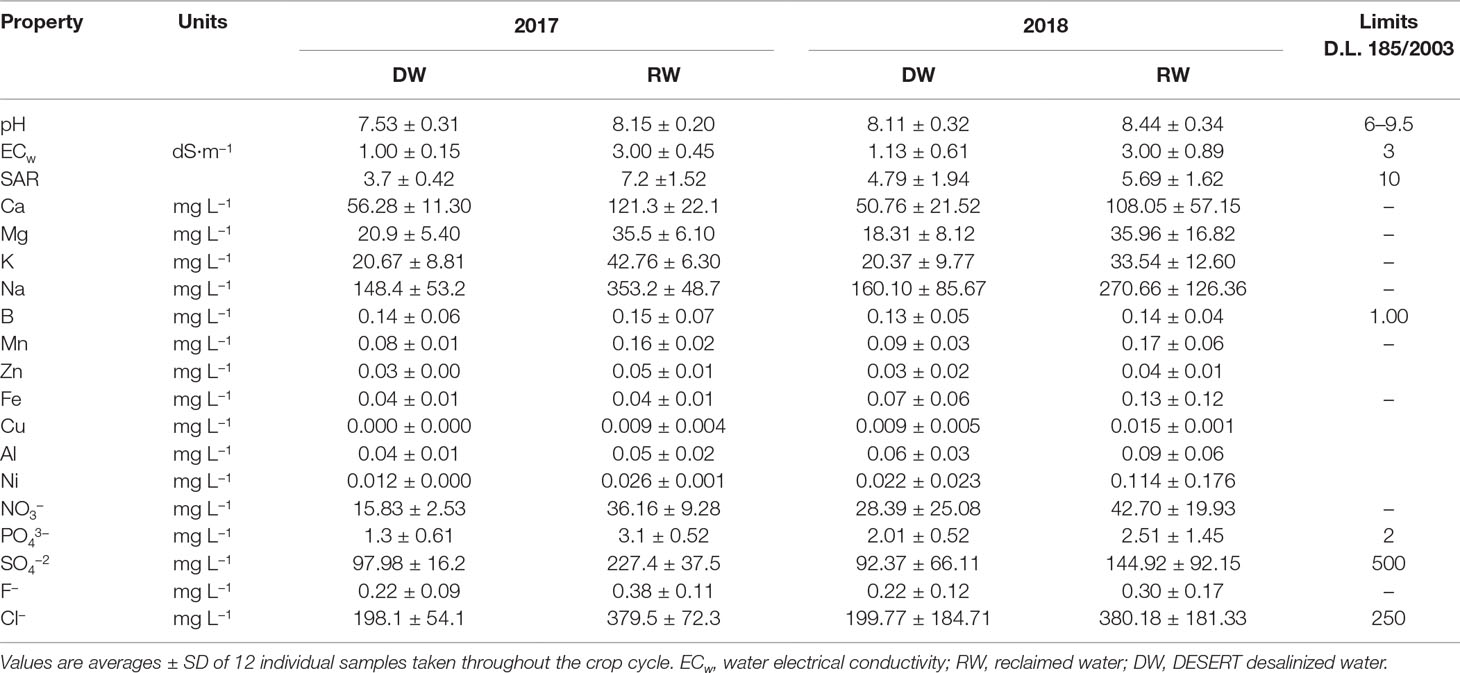
Table 1 Physical and chemical properties for DESERT desalinated water (DW) and reclaimed water (RW) in 2017 and 2018.
The irrigation season lasted from May 1 to October 31 and from May 15 to November 9 for 2017 and 2018, respectively. The amounts of water applied were 3679.63 and 3062.34 m3·ha−1 for FI and RDI treatments in 2017 and 2460.49 and 2011.23 m3·ha−1 for FI and RDI treatments in 2018, respectively (Figure 1). Therefore, the RDI treatment saved about 21% of irrigation water. The RDI period in 2017 began on DOY 180 (29th June 2017) and ended on 213 (August 1, 2017). The RDI period in 2018 started on DOY 180 (June 29, 2018) and ended on DOY 243 (August 31, 2018). The RDI period in 2017 lasted 1 month less than in 2018 for two reasons: (i) the trees were very young and (ii) the trees were shortly transplanted (1 year).
The water quality did not affect the SWP. However, the RDI treatments reached SWP values significantly more negative (−1.90 and −3.05 MPa in 2017 and 2018, respectively) than the control treatment (−1.02 and −1.29 MPa in 2017 and 2018, respectively) during the RDI periods. The SWP was lower in the water stressed trees during the second year than in the first one because the RDI period was longer.
Properties of the Fruit Production
Harvest data for each year are shown in Figure 2. In general, the fruit yield was very low in the first year of the experiment with an average value of 218 g per tree. In the following year, Arbosana trees started to bearing, with a yield of 2.01 kg per tree. The average individual fruit weight ranged between 2.2 and 2.5 g and between 2.0 and 2.6 g for 2017 and 2018, respectively. The fruit number per tree ranged from 81.3 to 110.3 in 2017 and from 734.3 to 972.0 in 2018. Considering the different treatments, there were no differences among them neither in yield, nor in fruit weight or number of fruit in 2017. However, the RW-FI treatment had a fruit yield significantly higher than the rest of treatments, about 35% more than DW-FI (Figure 2A) in 2018. This was mainly due to an increase in the olive weight (7%) and to a greater number of fruits per tree (21%), although there were no significant differences in these two parameters (Figures 2B, C). Water stress caused by the RDI strategies did not affect significantly the fruit yield, regardless of the water quality, although we observed an increase in the number of fruits per tree (32.4% and 23.5% for DW-RDI and RW-RDI, respectively) and a decrease in the fruit weight (21% and 6.8% for DW-RDI and RW-RDI, respectively) with respect to the DW-FI, mainly in the DW-RDI.
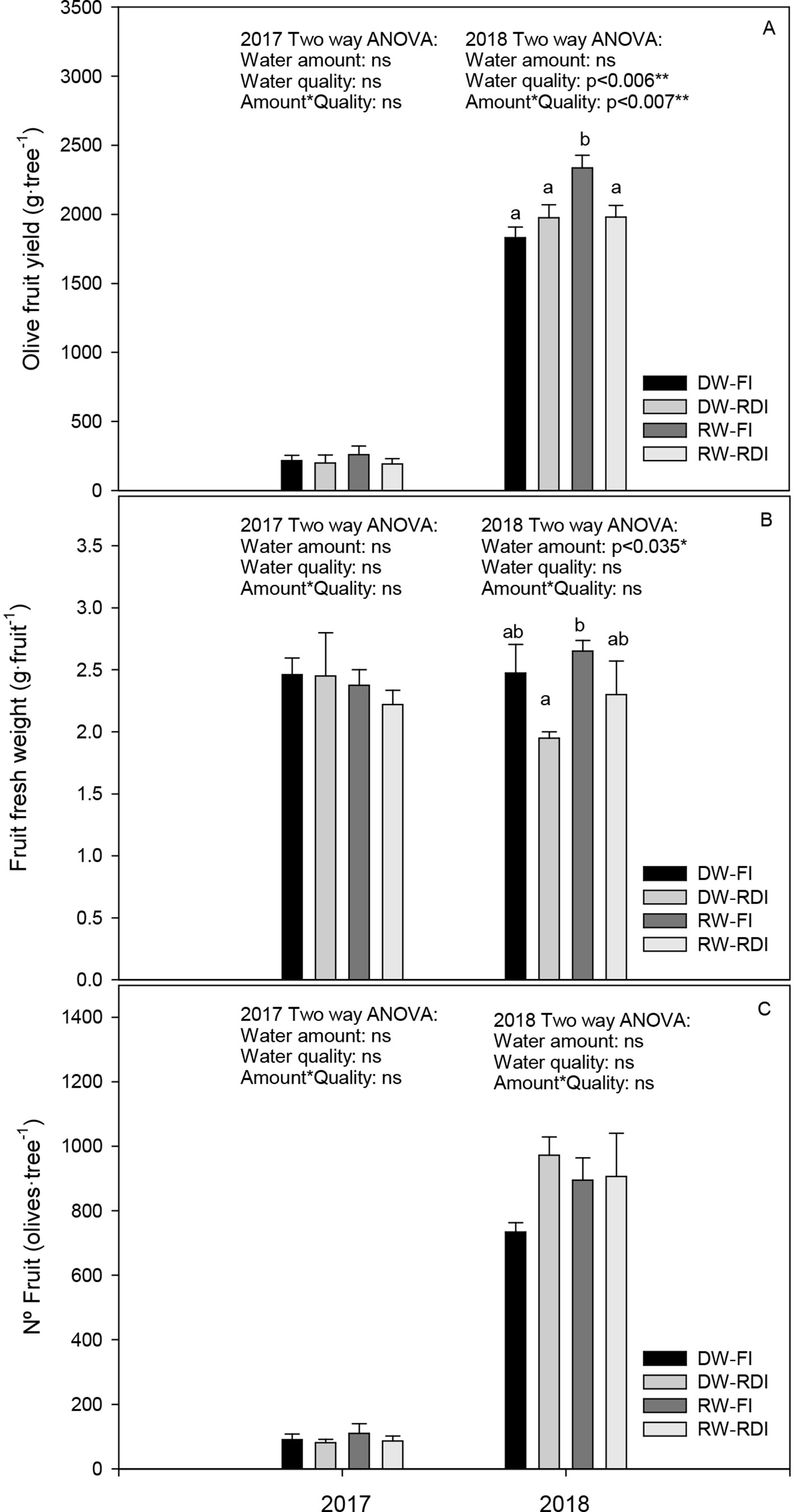
Figure 2 (A) Olive fruit yield (g tree−1), (B) fruit fresh weight (g fruit−1) and (C) number of fruit (olives tree−1) for each treatment: DW-FI (DESERT water-full irrigation), DW-RDI (DESERT water-regulated deficit irrigation), RW-FI (reclaimed water-full irrigation), and RW-RDI (reclaimed water-regulated deficit irrigation) for 2017 and 2018.
Regarding fruit ripening indices at harvest (Table 2), neither quality water (RW treatments) nor water amount (RDI treatments) affected significantly detachment index, fruit firmness, and PI. Nevertheless, some trends were observed. Fruits from RW trees (i) were detached more easily than control trees, although the fruit detachment force was not significantly lower in the RW-FI versus the DW-FI (Table 2), and (ii) had a higher PI than the DW-FI in the first year (63%). Besides, in 2017, the mean PI was 1.5 and in the second one decreased about 0.9. During the RDI period, DW-RDI fruits looked wrinkled. When such period ended, fruits visually recovered the firmness; although the lowest values of firmness were observed in this treatment at harvest. Finally, the fruit WC was significantly higher in the RDI treatments in 2018 (4.9% and 4.2% for DW-RDI and RW-RDI, respectively).
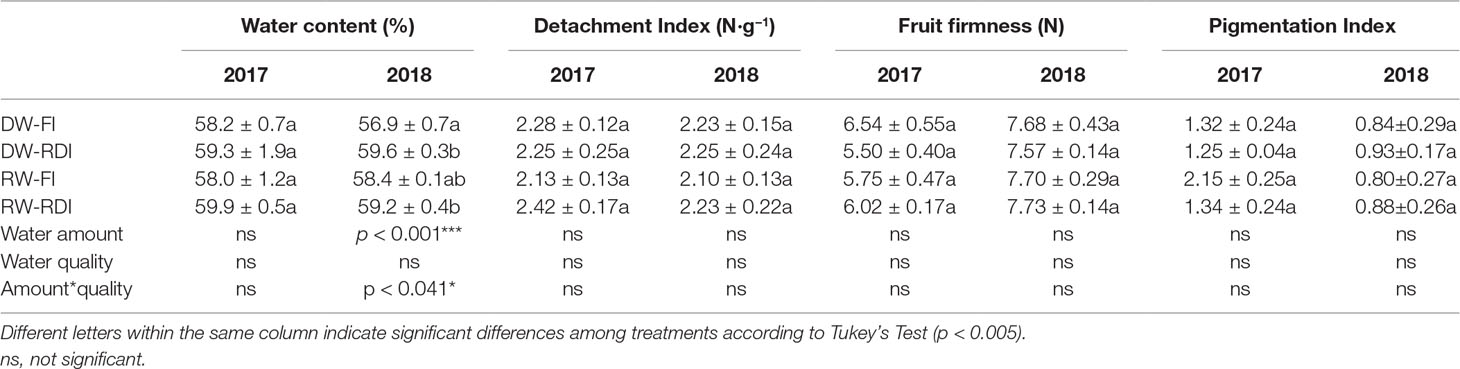
Table 2 Ripening indices: water content, detachment index, fruit firmness and pigmentation index for each treatment: DW-FI (DESERT water-full irrigation), DW-RDI (DESERT water-regulated deficit irrigation), RW-FI (reclaimed water-full irrigation) and RW-RDI (reclaimed water-regulated deficit irrigation) in 2017 and 2018.
Oil Determination
The fatty yield was affected by water stress in 2018 because both RDI treatments had less fatty performance than the DW-FI (a reduction of 15.2 and 11.0% for DW-RDI and RW-RDI, respectively) (Figure 3A). Similarly, oil industrial extractability also was decreased by RDI strategies in 2018 (a reduction of 16.2% and 11.6% for DW-RDI and RW-RDI, respectively) (Figure 3B). Humidity percentage in olive paste was higher in all stressed treatments compared with DW-FI in the second year. With respect to acidity, olive paste of RDI treatments also presented a mild reduction in its levels in both years, being significant for the DW-RDI in 2018 (6.1%) (Figure 3C). Moreover, taking into account all the treatments, humidity was significantly correlated to fatty yield (R = 0.82, p < 0.005***) and oil extractability (R = 0.80, p < 0.005***), so that as humidity increased, the fatty yield and oil extractability decreased. With less significance, humidity also correlated negatively with acidity (R = 0.60, p < 0.01**). In this sense, overall, the levels of fatty yield and oil extractability decreased by 4% and 1.2%, respectively, from 2017 to 2018. The fatty yield average values were around 24% in 2017 and decreased about 20% in 2018 and, like the oil extractability averages, were 16.4% in 2017 and 15.2% in 2018. On the contrary, humidity was higher in 2018 (61%) than 2017 (53%).
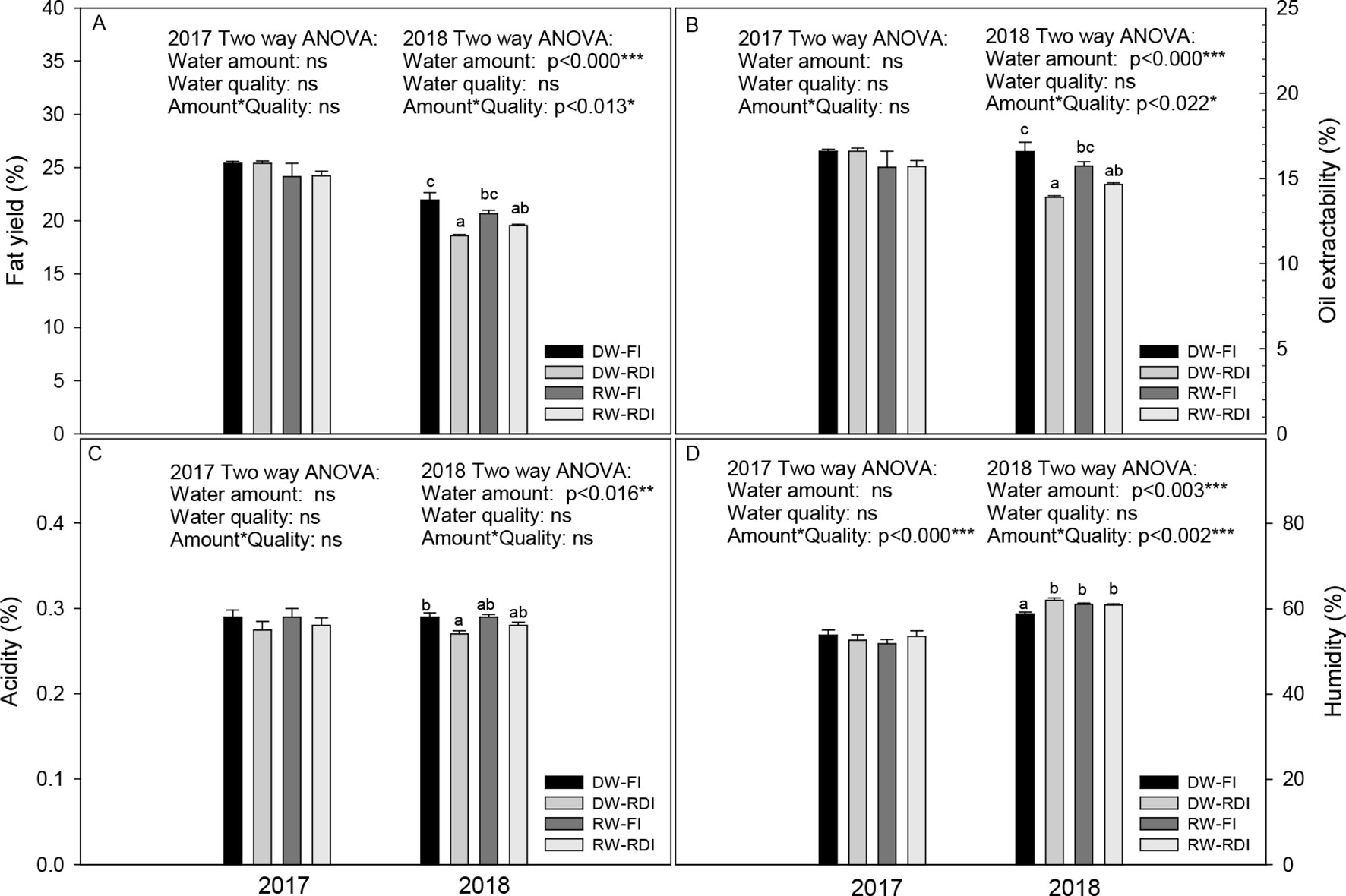
Figure 3 (A) Fatty yield (%), (B) oil extractability (%), (C) acidity (%) and (D) humidity (%) for each treatment: DW-FI (DESERT water-full irrigation), DW-RDI (DESERT water-regulated deficit irrigation), RW-FI (reclaimed water-full irrigation), and RW-RDI (reclaimed water-regulated deficit irrigation) in 2017 and 2018.
There were no significant differences among treatments for the oil content per fresh and dry fruit weight and for the oil yield in the first year of the experiment. However, oil content was affected by the water quality in the next year by two-way ANOVA (Figures 4A, B). Concretely, the oil content based on fresh fruit weight ranged from 8.20% to 13.50% and in the RW-FI and RW-RDI a reduction by 25% and 33%, respectively, was found (Figure 4A). In DW-RDI, on the contrary, a tendency to increase (10.6%) was observed. Oil content based on dry fruit weight showed the same behavior than oil content based on fresh weight in 2018, with values between 20% and 33%. A decrease by 22.9% in RW-FI and also a strong decrease by 39.93% in the RW-RDI, with respect to the DW-FI, were observed. Contrary, we observed a slight increase by 18.3% in the DW-RDI (Figure 4B).The oil yield was 0.22, 0.25, 0.21, and 0.16 goil tree−1 for DW-FI, DW-RDI, RW-FI, and RW-RDI, respectively, in 2018. Despite the two-way ANOVA indicating that this parameter was not affected by the quality or quantity of water (Figure 4C), Tukey’s test did show a tendency, that is, the oil yield decreased by 1.72% in RW-FI, despite such treatment having the highest olive fruit production, and markedly decreased by 24.8% in trees with the combination RWRDI. An increase by 14.5% in the DW-RDI was also found.
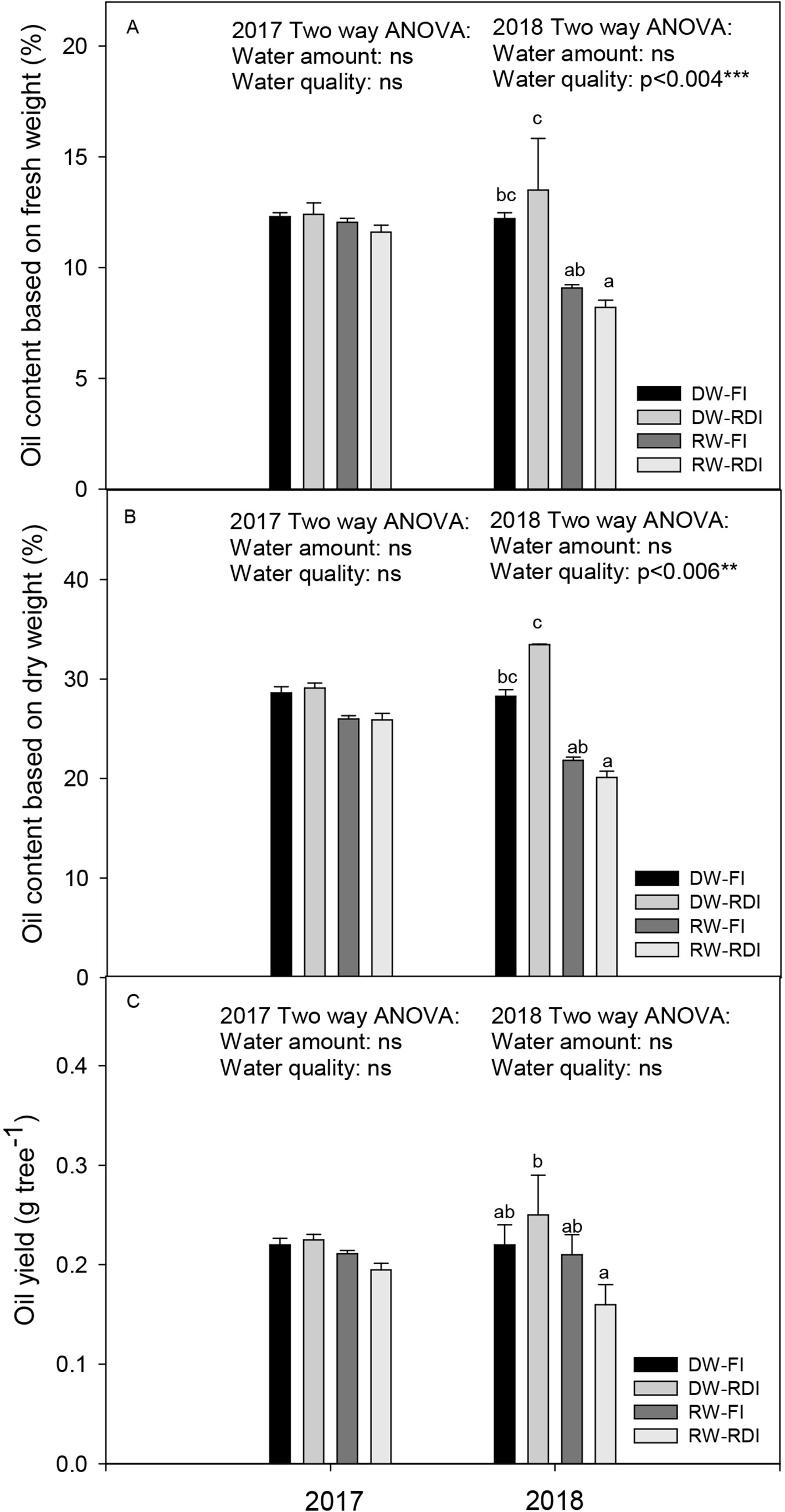
Figure 4 Oil content based on (A) fresh fruit weight (%), (B) oil content based on dry fruit weight (%) and (C) oil yield (g tree−1) for each treatment: DW-FI (DESERT water-full irrigation), DW-RDI (DESERT water-regulated deficit irrigation), RW-FI (reclaimed water-full irrigation) and RW-RDI (reclaimed water-regulated deficit irrigation) in 2017 and 2018.
Oil Quality
The most nutritional and chemical quality parameters accepted in oil evaluation were evaluated (Tables 3 and 4, and Figure 5).
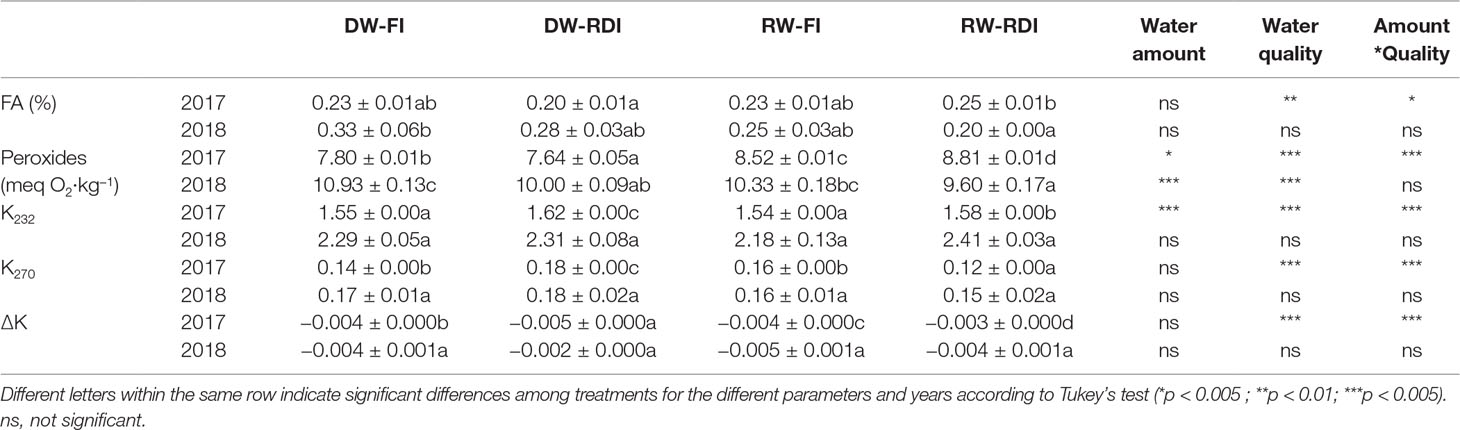
Table 3 Oil quality chemical parameters: free acidity (FA), peroxides, K232, K270 and ΔK for each treatment: DW-FI (DESERT water-full irrigation), DW-RDI (DESERT water-regulated deficit irrigation), RW-FI (reclaimed water-full irrigation) and RW-RDI (reclaimed water-regulated deficit irrigation) in 2017 and 2018.
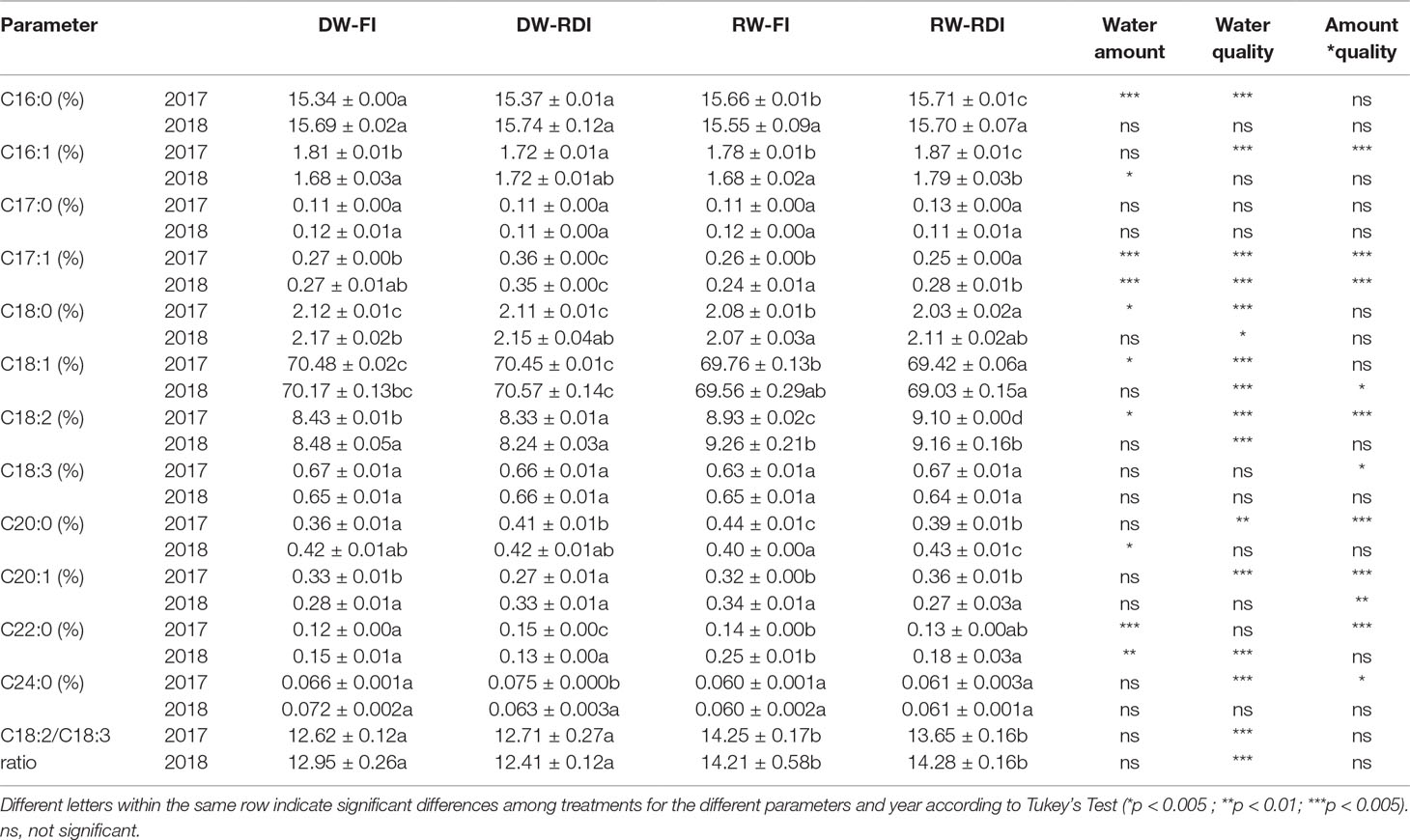
Table 4 Profiles of fatty acids methyl esters in the oil samples of each treatment: DW-FI (DESERT water-full irrigation), DW-RDI (DESERT water-regulated deficit irrigation), RW-FI (reclaimed water-full irrigation) and RW-RDI (reclaimed water-regulated deficit irrigation) in 2017 and 2018.
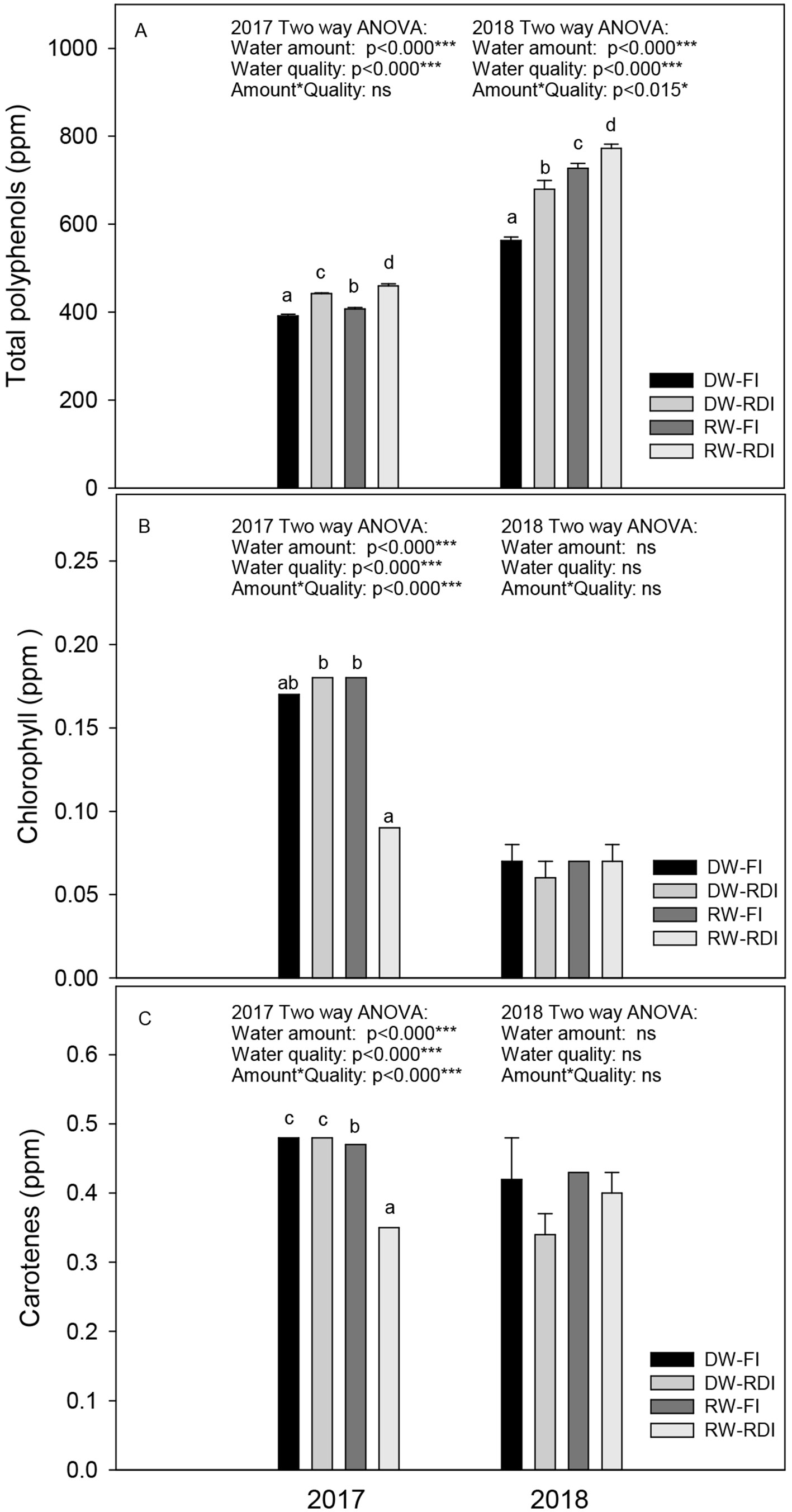
Figure 5 (A) Total polyphenols, (B) chlorophyll and (C) carotenes (ppm) in oil samples of each treatment: DW-FI (DESERT water-full irrigation), DW-RDI (DESERT water-regulated deficit irrigation), RW-FI (reclaimed water-full irrigation), and RW-RDI (reclaimed water-regulated deficit irrigation) in 2017 and 2018.
Taking into account the different irrigation treatments, the FA had no clear tendency (Table 3). We observed that it slightly decreased in DW-RDI in 2 years, similar to the data found for the acidity during industrial extraction (Figure 3). The peroxides decreased in DW-RDI in both years and increased in RW treatments in 2017, with respect to the control trees. The RW-RDI did not show a clear tendency for both years. The K232 and K270 indices are also indicators of the presence of oxidation compounds in oil, other than peroxides. The mean values of the specific extinction coefficients ranged from 1.51 to 2.41 and from 0.12 to 0.18, respectively, for K232 and K270. K232 had the highest values in the RDI treatments, mainly in DW-RDI, but was only significant in 2017. As for K270, it increased in the DW-RDI and decreased in the RW-RDI, being statistically significant only in 2017. The delta K index showed the highest values in RW treatments in 2017. In general, it is also important to highlight, that in the second year, the values of peroxides and K232 were higher than in the first year in all cases.
On the other hand, significant effects among treatments on the fatty acid (FAME) composition of the major fraction of the olive oil, also known as saponifiable fraction, were observed (Table 4). The saturated and monounsaturated acids palmitic (C16:0) and palmitoleic (C16:1) were affected by quality and amount of water. C16:0 increased by RW (FI and RDI) only in 2017 and C16:1 by the combination of water and saline stress (RW-RDI). The saturated stearic acid (C18:0) and monounsaturated oleic acid (C18:1) significantly decreased in the RW treatments, mainly in the RW-RDI, this last one ranging between 69.03% and 70.57%. The polyunsaturated linoleic (C18:2), known as ω6, increased by RW around 9.2% and 7.1% in 2017 and 2018, respectively, being more marked again in the RW-RDI for 2017. However, linolenic acid (C18:3, ω3) was not affected by any treatment, ranging between 0.63% and 0.67%. Consistently, the C18:2/C18:3 ratio increased about 10.3% in both RW treatments (FI and RDI). As for the acids with hydrocarbon chain of 20 or more carbons, C20:0 was higher in all treatments, with respect to the DW-FI in 2017 and only in the RW-RDI in the next year. The acid C20:1 decreased by RDI (DW-RDI) in 2017. C22:0 obtained the higher values in the DW-RDI and the RW-FI. Finally, C24:0 increased in the DW-RDI and tended to decrease by effect of RW in the first year.
The main FAME of olive oil was oleic acid consisting around 70% of the FAMEs found (Table 4). The second most abundant FAME was palmitic, and the third one was the polyunsaturated FAME linoleic. The order from higher to lower concentration of the different acids was as follows:
C18:1>C16:0>C18:2>C18:0>C16:1>C18:3>C20:0>C20:1>C17:1>C22:0>C17:0>C24:0
Within the unsaponifiable minority fraction of olive oil, polyphenols and pigments were evaluated (Figure 5). Total polyphenols content ranged between 390 and 460 ppm and between 562 and 772 ppm in the first and second year, respectively. Their levels significantly improved in all treatments, with respect to the control trees, mainly in RW-RDI. The increases were 12.9%, 4.0%, and 17.5% in 2017 and 20.9%, 29.3%, and 37.3% in 2018 for DW-RDI, RW-FI, and RW-RDI, respectively. The differences among treatments were higher in 2018 than in 2017. This response can be explained by the highest values of 2018 (Figure 5A).
Regardless of the treatments, the chlorophilic and carotenoids levels were lower in the second year than the first one (Figures 5B, C). The RW irrigation increased chlorophyll and decreased carotenoid contents; the combination of both stresses (RW-RDI) strongly decreased the two pigments by in the first experimental year: 47.7% less chlorophyll and 27.0% of carotenoids than the rest of treatments. In 2018, a tendency to decrease both pigments by DW-RDI was observed.
Discussion
Water Quality
Treated RW contain soluble minerals which depend quantitatively and qualitatively on the original source of the water and the type of treatment (Petousi et al., 2015). Our results were roughly in line with what we would expect from a properly performing secondary wastewater treatment plant. Although the EC and SAR values of RW were relatively higher than DW, they met the limits of the D.L. 185/2003. Besides, it is known that olive trees can tolerate irrigation water salinity of up to 5 dS·m−1 with a SAR of 18 (Tattini et al., 1992). The phytotoxic Cl− and Na also were higher in RW, with respect to DW and the Cl− levels exceed the limit. Moreover, RW contained quantities of nutrients as well as essential elements higher than DW source, although it also showed elevated levels of Al and Ni, according to Rosecrance et al. (2015).
Effects of the Water Quality and Amount on the Olive Production Properties
In the first year of our experiment, trees were still under juvenile phase, giving a very low fruit yield response. In the second year, Arbosana trees started to bear, confirming what was well stated in the literature (Camposeo and Vivaldi, 2018). Water quality was key in terms of fruit yield. The higher yield obtained in RW full-irrigated trees was a consequence of the increasing in fruit weight and number due to the presence of nutrient elements. Thus, RW irrigation worked as fertigation. In the RDI treatments, the fruit yield was not affected by water stress although the percent of fruit set was higher and fruit weight was lower than in the control, mainly in DW-RDI, without any significant differences (Figure 2). Our results are in agreement with the finding of other researchers who reported that olive irrigation with treated wastewater significantly increased the fruit yield (Bedbabis et al., 2010; Bourazanis et al., 2016; Ayoub et al., 2016). A long-term experiment of 8 years conducted in Israel with “Barnea” and “Leccino” trees irrigated with saline wastewater of lower EC than ours (ECw∼1.7 dS·m−1) and fresh water reported that fruit yield was not significantly different among treatments in any individual season; however, the highest values of total yield in “Barnea” olives for the entire experiment was found in the wastewater treatment (Erel et al., 2019). . In other experiments carried out in Córdoba (Spain) under field conditions with Picual olive, two saline treatments (ECw = 5 dS·m−1 and ECw = 10 dS·m−1), without using wastewater from treatment plants, did not change annual yield in any of the 9-year studies (Melgar et al., 2009). In contrast, authors Gucci and Tattini, (1997) reported that a significant yield reduction occurs in olives cultivated under high-saline conditions (ECw >7.5 dS·m−1). Ben-Gal et al. (2017) also informed that fruit yield decreased with increasing salinity in “Barnea” trees irrigated with water of different ECw (from 2 to 11 dS·m−1). Other studies, such as Ben Ahmed et al. (2007) and Chartzoulakis (2011), reported that saline waters might reduce yield compared with good quality water. Concerning fruit characteristics like fruit weight, Bourazanis et al. (2016) found that treated wastewater produced heavier fruit than those irrigated with fresh water, as in our results. However, Melgar et al. (2009) showed that salinity did not affect fruit weight.
Regarding water amount, a decrease in biomass production due to deficit irrigation for many fruit trees does not necessarily lead to a parallel reduction in fruit yield because of changes in biomass partitioning between the different organs (Gucci et al., 2019). As a result, no reductions in yield have been reported for peach (Gelly et al., 2003), plum (Intrigliolo and Castel, 2010), almond (Stewart et al., 2011), pear-jujube (Cui et al., 2009), apricot (Perez-Pastor et al., 2014) when the stress applied during the irrigation season was moderate. In olive trees, the water volume can be reduced well below the level of full satisfaction of water needs with limited or no effects on fruit yield (Moriana et al., 2003; Gucci et al., 2007; Lavee et al., 2007; Gómez del Campo, 2013), as in our results.
As for ripening indices, the detachment index and the fruit firmness are usually used to detect the optimal harvesting time for cultivars with a long maturation period, as “Arbosana” (Camposeo et al., 2013). The lowest detachment index was found in RW-FI with values about 2.1 N·g−1, being this really positive for the mechanical harvesting efficiency, which is maximum (90–95%) when the detachment index is around 2 N·g−1 (Farinelli et al., 2012; Camposeo et al., 2013). The RDI strategies did not affect the detachment index, unlike Rosecrance et al. (2015) who reported that the RDI had a lower fruit detachment force, contributing to greater percentage fruit removal. A low fruit firmness was presented by water stress with DW (DW-RDI), contrary to another study that did not observe differences on fruit firmness by deficit irrigation (Rinaldi et al., 2011). In the case of the RW-RDI, a low fruit firmness was not observed likely due to the salinity from RW. Regarding fruit WC, it is known that moisture levels below 50% and about 60% are difficult for the mill to extract the oil (Rosecrance et al., 2015). In our study, all treatments are within that range, although the RDI had the highest values, in contrast with Rosecrance et al. (2015) who found that water stress decreased fruit WC. Finally, crop load could explained the higher PI in 2017 (1.5; very low bearing year), with respect to 2018 (bearing year) since it is known that fruit ripening strongly depends on crop load, among other factors as the climatic conditions (Camposeo et al., 2013).
Effects of the Water Quality and Amount on Oil Industrial Variables
In the process of oil extraction, the irrigation water amount was more decisive than water quality. The treatments under water stress (RDI) presented the lowest fatty yield and oil extractability, which is probably caused by the increase in humidity in the olive paste which hindered the oil extraction, as García et al. (2013) explained. Other researchers have also found that too high fruit humidity content decreases oil extraction (Gómez-del-Campo, 2013). The acidity levels, however, improved with the RDI.
Concerning oil yield, it is known that it is a function of the fruit yield as well as the percent of oil (Ben-Gal et al., 2017). In our study, the water quality was a more determining factor than the amount of water. The lowest values of oil content per dry or fresh weight were found in RW trees. Thus, although the RW-FI had the highest fruit yield, the oil yield did not improve, with respect to the control. So far, nothing has been published accordingly with our results about effects of RW-FI on the oil yield. Ayoub et al. (2016) did not find differences on oil content per dry fruit weight basis among fresh water and RW. Ben-Gal et al. (2017) cited that oil content in fruit is increased with the water EC during two years in Barnea trees irrigated with saline water. Bourazanis et al. (2016) also indicated that Koroneiki trees irrigated during two years with treated water produced more oil per tree than those irrigated with fresh water. Similar results have been also described by Segal et al. (2011), reporting a slight but non-significant increase in olive and oil yield in Barnea and Leccino. With regard both stresses combined (RW-RDI), there is nothing mentioned in the literature.
Moreover, as for water amounts, oil content dry weight-based slightly increased in the treatment with water stress (20% more in DW-RDI respect to the control), leading to the highest oil yield, despite its low oil extractability. Water stress could indirectly increase oil yield in many ways including: (1) improving the light environment for oil accumulation, (2) hastening fruit maturity at harvest, and (3) increasing fruit removal percentages from the trees (Rosecrance et al., 2015). In this work, only the first hypothesis would be possible. Trees growing under water stress (DW-RDI) had lower branches length than control trees (data not shown), and although light environments were not measured, these smaller trees likely had a greater proportion of the fruit exposed to high irradiance which likely contributed to increased oil yields. Other authors already found that to maximize olive oil yield, high irradiance is needed (Gómez-del-Campo et al., 2009; Cherbiy-Hoffmann et al., 2013).
There is dispersion in the literature about the effects of water stress on oil yield. Similar results to ours were found by a number of authors who reported curvilinear relationships between oil yields and water application, indicating that oil yields are maximized at water application rates below 100% of FI (Moriana et al., 2003). This is, the oil percentages can be increased with moderate water stress (Gucci et al., 2009; Caruso et al., 2013; Gómez-del-Campo, 2013; Rosecrance et al., 2015). Other authors cited the water volume can be reduced well below the level of full satisfaction of water needs with limited or no effects on fruit yield and oil yield (Gucci et al., 2007; Gispert et al., 2013; Gómez-del-Campo, 2013; Hernández et al., 2018).
Contrary to our results, in Fernández et al. (2013), a small reduction in oil yield by 26% was observed when the water applications were reduced by 72% in an olive orchard of “Arbequina.”
Effects of the Water Quality and Amount on Oil Quality Parameters
In many parameters, differences between the two experimental years of the study were found as a consequence of the different duration of the RDI period as well as of environmental factors and fruit load, which are known to affect oil quality (Ayoub et al., 2016).
FA, Peroxides, K232, K270, Delta K Index
As for oil quality legal attributes, water quality (RW-FI and RW-RDI) did not affected the FA, according to Bedbabis and Ferrara (2018) who showed that acidity values were not significantly increased by saline water, as also reported by Bourazanis et al. (2016) using wastewater. However, the RW source accelerated the oxidation of the oil causing elevations in the peroxides and ΔK in 2017. Other works affirmed that these parameters were not affected by RW (Bourazanis et al., 2016).
The reduction of water amount combined with DW (DW-RDI), slightly decreased FA and peroxides, improving oil quality; however, it increased the K232 and K270 characteristics compared with the control, although only in the first year. A high value of these coefficients results in a lower resistance to oxidation of the oil and with a greater degree of oxidation. Changes in oil quality due to water deficit have been reported for many olive cultivars (Servili et al., 2007; Gómez-del-Campo and García, 2013; Caruso et al., 2014, Caruso et al., 2017; Hernández et al., 2018). Although most of these studies have shown that the irrigation regime had negligible or no effects on parameters as FA, peroxide values, spectrophotometric indices (K232, K270 and ΔK) (Gucci et al., 2019).
In general, the values of FA, peroxides, and K232 and K270 for all oil samples examined here were lower than the maximum limits established by the cited EU legislation for the extra virgin olive oil (EVOO) category (FA ≤ 0.8; Peroxides Index ≤ 20; K232 ≤ 2.5; K270 ≤ 0.22; ΔK ≤ 0.01).
Fatty Acid Profile
When we evaluated the FAME profile, oleic acid was the dominant acid in the olive oil obtained in all irrigation treatments (ranging from 69% to 71%), followed by palmitic (15.34–15.74%), linoleic (8.24–9.26%), and stearic acid (2.03–2.17%), with their concentration falling within the range of the values for characterizing it as EVOO.
The FAME composition was more affected by water quality than by water amount. Previous studies reported increases in palmitoleic (Ben Brahim et al., 2016) and linoleic (Bedbabis and Ferrara et al., 2018) acids in treatment irrigated with saline water, as in our results. The important increase (7–9%) of the linoleic acid was probably due to the fatty synthase enzymes stimulation as fruit maturity progressed in RW treatments. Since linolenic acid were not affected by RW, according to Bourazanis et al. (2016), the ω6/ω3 ratio of oil from RW treatments increased around 10%, as also Tietel et al. (2019) indicated. Furthermore, other important acids as oleic and stearic reduced their percentages when the olive trees were irrigated with RW.
Moreover, in our experiment the reduction of the water amount (DW-RDI) only affected a few minority fatty acids as C20:1, C22:0, and C24:0 and decreased the linoleic acid respect to the DW-FI, although only in 2017. Accordingly, Hernández et al. (2018) found a decrease in linoleic acid under severe RDI conditions. Moreover, several studies for many cultivars have shown that the water deficit had negligible or no effects on FAME composition (Gómez-del-Campo and García, 2013; Caruso et al., 2014, Caruso et al., 2017).
As for the effects of the combination of both stresses (RW-RDI) on fatty acid, we observed that the reduction of oleic acid and the increase of linoleic were more pronounced than in RW-FI. It is not possible to discuss these results with other work because, so far, nothing has been published about the combination of RW and RDI strategies.
The effects reported here regarding FAME profile did not show very great differences among treatment from an agronomical point of view. However, they have a large nutritional significance, since they reflect a trend of declining nutritional and health quality of olive oil as a result of irrigation with RW. Many of the health-promoting traits of olive oil are ascribed to its monounsaturated fatty acid content, mainly the oleic acid. This has been clinically proven to enhance cardiovascular health and improve blood-lipid profile, in addition to other metabolic-syndrome-related advantages (Tietel et al., 2019). In addition, the low levels of saturated fatty acids and the ω6/ω3 ratio play a key role in the bioactivity of olive oil as a functional food (Tietel et al., 2019). Specifically, higher ω6/ω3 ratios, as occurred in this experiment with the use of RW, increase the risk for obesity (Simopoulos, 2016).
Parameters of the Minority Unsaponificable Fraction
Both water quality and amount affected the polyphenol levels, which play a role in the stress response and defense mechanism of the tree. In our experiment, saline RW (RW-FI) increased oil polyphenols , probably due to stress response to high salt levels, as reported by Chartzoulakis (2011). Salinity stress causes subsequent water deficit, which has been shown to be involved in the activation of phenylalanine ammonia lyase (PAL) (Ben Ahmed et al., 2009), a key enzyme directly involved in polyphenol biosynthesis in fruit, which causes an accumulation of phenolic compounds in the oil (Patumi et al., 2002).
Regarding RDI strategy (DW-RDI), it also improved the polyphenols content in oil, consistent with many studies (Gómez-del-Campo and García, 2013; Rosecrance et al., 2015; Caruso et al., 2017) due to water deficit enhanced synthesis of these compounds in the fruit, according to Alagna et al. (2012). Severe conditions trigger antioxidation mechanisms activated by the plant in response to oxidative stress, and hence accumulate in oil (Tietel et al., 2019). Others findings suggest that the catabolism of phenolic substances in the fruit is likely influenced by water stress too (Cirilli et al., 2017).Moreover, RW-RDI was the treatment that showed the greatest increases in polyphenols both years. As far as we know, it is the first time that data are reported respect to the combination of water and saline stresses and nothing has been published in the literature.
From the point of view of food quality, the irrigation effects on the polyphenols levels are relevant for oil sensory quality and for the health-promoting effects of the oil (Clodoveo et al., 2015; Tietel et al., 2019) as the prevention of the formation of cancer cells, so that European Food Safety Authority (EFSA) launched a specific health claim (EU, 2012). In addition, polyphenols are also key contributors to oxidative stability, mainly 3,4-DHPEA and its secoiridoid derivatives (Servili et al., 2004). Thus, irrigation with RW and RDI and mainly the combination of both might also greatly positively affect oil shelf life and nutraceutical claim.
Finally, the climatic pattern during ripening period could explain the strong increase of 25% of total oil polyphenols contents in 2018 with respect to 2017. Indeed the second year was colder than the former. In the literature, it has been stated that low temperature stimulates polyphenol accumulation in olive oil (Artajo et al., 2006).
Color is a basic attribute for determining the characteristics of olive oil although analysis of the pigmentation is not required in the corresponding EU Regulation. The trees full irrigated with RW increased chlorophyll levels in 2017, being a positive aspect since most consumers associate the presence of chlorophyll with quality. Nevertheless, our results are contradictory with other studies (Ghrab et al., 2014; Bedbabis et al., 2015). As for carotenoids, their levels decreased in RW-FI, as Ghrab et al. (2014) also cited.
In summary, the trees full irrigated with RW improved the fruit yield although it did not increase the oil yield since the oil content dry weight-based was lower than control trees. The changes in oil fatty acid composition of these trees demonstrated tendencies that are undesirable, including increased unsaturated acids, as well as the ratio ω6/ω3. The peroxides also increased. On the contrary, higher levels of the polyphenols in oil were presented. The deficit irrigation, with DW, did not affect the fruit yield, although there was an increase in the number of fruits which showed less weight and firmness during the RDI period. Despite the reduction of the fatty yield and oil extractability due to the high fruit WC, this treatment presented the highest oil yield since oil content fruit dry weight-based improved by 20%. Furthermore, there was a reduction in the acidity and peroxides and an increase in the polyphenols of the oil by water stress. Some negative aspect were also found: an increase in K232 and K270, although within the legal limit, and in some minority acid as C20:0, C22:0, and C24:0. Finally, the combination of RW and RDI neither reduced fruit yield. Besides, its fruits did not lose as much weight or firmness as in DW-RDI. However, although the fatty yield and oil extractability decreased less than in DW-RDI, the oil yield values of these trees under both stresses were the lowest compared with the rest of treatments since the low oil content fruit dry weight-based. As for the oil quality of RW-RDI, similar results as in RW-FI were observed, plus an important decrease of pigments in the first year. It is important to highlight also that the highest levels of polyphenols were displayed in this treatment. These aspects described about the combination of both stresses in this paper are reported in the literature for the first time. These findings could help optimize crop management of cv Arbosana in new olive cropping system, where environmental sustainability represent a key factor.
Data Availability Statement
All datasets generated for this study are included in the manuscript/supplementary files.
Author Contributions
Data curation: GV, CR-T, AP. Formal analysis: GV, AP, CR-T. Investigation: GV, CR-T, EN. Methodology: GV, FS. Project administration: SC, GV. Resources: SC, GV. Supervision: SC, GV, EN. Writing – original draft: CR-T. Writing – review and editing: SC, GV, EN.
Funding
The research involved in this work has been supported by the EU and Water JPI for funding, in the frame of the collaborative international Consortium DESERT, financed under the ERA-NET WaterWorks 2014 Cofunded Call. This ERA-NET is an integral part of the 2015 Joint Activities developed by the Water Challenges for a Changing World Joint Programme Initiative (Water JPI), and “Fondo di Sviluppo e Coesione” 2007-2013 e APQ Ricerca Regione Puglia “Programma regionale a sostegno della specializzazione intelligente e della sostenibilita sociale ed ambientale e FutureInResearch.”
Conflict of Interest
The authors declare that the research was conducted in the absence of any commercial or financial relationships that could be construed as a potential conflict of interest.
Acknowledgments
CR-T acknowledges the financial support for Postdoctoral training and development fellowship (20363/PD/17) of Consejería de Empleo, Universidades y Empresa (CARM) by the Fundación Séneca-Agencia de Ciencia y Tecnología de la Región de Murcia.
References
Alagna, F., Mariotti, R., Panara, F., Caporali, S., Urbani, S., Veneziani, G., et al. (2012). Olive phenolic compounds: metabolic and transcriptional profiling during fruit development. BMC Plant Biol. 12, 162. doi: 10.1186/1471-2229-12-162
Allen, R. G., Pereira, J. S., Raes, D., Smith, M. (1998). “Food and Agriculture Organization of the United Nations,” in Crop Evapotranspiration: Guidelines for Computing Crop Water Requirements (Rome).
Aragüés, R., Puy, J., Royo, A., Espada, J. L. (2005). Three-year field response of young olive trees (Olea europaea L., cv. Arbequina) to soil salinity: trunk growth and leaf ion accumulation. Plant Soil 271, 265–271. doi: 10.1007/s11104-004-2695-9
Arborea, S., Giannoccaro, G., De Gennaro, B., Iacobellis, V., Piccinni, A. F. (2017). Cost–benefit analysis of wastewater reuse in Puglia, Southern Italy. Water 9, 175.
Artajo, L. S., Romero, M. P., Tovar, M. J., Motilva, M. J. (2006). Effect of irrigation applied to olive trees (Olea europaea L.) on phenolic compound transfer during olive oil extraction. Eur. J. Lipid Sci. Technol. 108, 19–27. doi: 10.1002/ejlt.200500227
Ayers, R. S., Westcot, D. W. (1985). Water quality for agriculture. (Rome: FAO Irrigation and Drainage), 174.
Ayoub, S., Al-Ahdiefat, S., Rawashdeh, H., Bahabsheh, I. (2016). Utilization of reclaimed wastewater for olive irrigation: effect on soil properties, tree growth, yield and oil content. Agric. Water Manag. 176, 163–169. doi: 10.1016/j.agwat.2016.05.035
Batarseh, M. I., Rawajfeh, B. A., Kalavrouziotis, I., Prodromos, H. (2011). Treated Municipal Wastewater Irrigation Impact on Olive Trees (Olea Europaea L.) at Al-Tafilah, Jordan. Water Air Soil Pollut. 217, 185–196. doi: 10.1007/s11270-010-0578-7
Bedbabis, S., Clodoveo, M. L., Rouina, B. B., Boukhris, M. (2010). Influence of irrigation with moderate saline water on ‘chemlali’ extra virgin olive oil composition and quality. J Food Qual. 33, 228–247. doi: 10.1111/j.1745-4557.2010.00310.x
Bedbabis, S., Ben Rouina, B., Boukhris, M., Ferrara, G. (2014). Effects of irrigation with treated wastewater on root and fruit mineral elements of Chemlali Olive cultivar. Scien. World J. 2014, Article ID 973638, 8. doi: 10.1155/2014/973638
Bedbabis, S., Trigui, D., Ben Ahmed, C., Clodoveo, M. L., Camposeo, S., Vivaldi, G. A., et al. (2015). Long-terms effects of irrigation with treated municipal wastewater on soil, yield and olive oil quality. Agric. Water Manag. 160, 14–21. doi: 10.1016/j.agwat.2015.06.023
Bedbabis, S., Ferrara, G. (2018). Effects of long term irrigation with treated wastewater on leaf mineral element contents and oil quality in olive cv. Chemlali. J. Hort. Sci. Biotech. 93 (2), 216–223. doi: 10.1080/14620316.2017.1354729
Ben Ahmed, C., Ben Rouina, B., Boukhriss, M. (2007). Effects of water deficit on olive trees cv. Chemlali under field conditions in arid region in Tunisia. Sci. Hort. 133 (3), 267–277. doi: 10.1016/j.scienta.2007.03.020
Ben Ahmed, C., Ben Rouina, B., Sensoy, S., Boukhriss, M. (2009). Saline water irrigation effects on fruit development, quality, and phenolic composition of virgin olive oils, cv. Chemlali. J. Agric. Food Chem. 57, 2803–2811. doi: 10.1021/jf8034379
Ben Brahim, S., Gargouri, B., Marrakchi, F., Bouaziz, M. (2016). The effects of different irrigation treatments on olive oil quality and composition: a comparative study between treated and olive mill wastewater. J. Agric. Food Chem. 64, 1223–1230. doi: 10.1021/acs.jafc.5b05030
Ben-Gal, A., Beiersdorf, I., Yermiyahu, U., Soda, N., Presnov, E., Zipori, I., et al. (2017). Response of young bearing olive trees to irrigation-induced salinity. Irrig. Sci. 35, 99–109. doi: 10.1007/s00271-016-0525-5
Ben Rouina, B., Ben Ahmed, Ch., Bedbabis, S., Baccari, M., Boukhris, M. (2011). “Chapter in Book Environment and Ecology in the Mediterranean Region,” in Effects of long-term irrigation with treated wastewater on soil chemical properties, plant nutrient status, growth and oil quality of olive tree (UK: Cambridge Scholars Publishing), 147–156. Chapter Thirteen.
Bourazanis, G., Roussos, P. A., Argyrokastritis, I., Kosmas, C., Kerkides, P. (2016). Evaluation of the use of treated municipal waste water on the yield, oil quality, free fatty acids’ profile and nutrient levels in olive trees cv Koroneiki, in Greece. Agric. Water Manag. 163, 1–8. doi: 10.1016/j.agwat.2015.08.023
Camposeo, S., Vivaldi, G. A. (2018). Yield, harvesting efficiency and oil chemical quality of cultivars 'Arbequina' and 'Arbosana' harvested by straddle machine in two Apulian growing areas. Proceed. Paper Acta Hortic. of VIII Int. Olive Symp. 1199, 397–402. doi: 10.17660/ActaHortic.2018.1199.63
Camposeo, S., Vivaldi, G. V., Gattull, C. E. (2013). Ripening indices and harvesting times of different olive cultivars for continuous harvest. Sci. Hortic. 151, 1–10. doi: 10.1016/j.scienta.2012.12.019
Caruso, G., Rapoport, H. F., Gucci, R. (2013). Long-term evaluation of yield components of young olive trees during the onset of fruit production under different irrigation regimes. Irrig. Sci. 31, 37–47. doi: 10.1007/s00271-011-0286-0
Caruso, G., Gucci, R., Urbani, S., Esposto, S., Taticchi, A., Di Maio, I., et al. (2014). Effect of different irrigation volumes during fruit development on quality of virgin olive oil of cv Frantoio. Agric. Water. Manag. 134, 94–103. doi: 10.1016/j.agwat.2013.12.003
Caruso, G., Gucci, R., Sifola, M. I., Selvaggini, R., Urbani, S., Esposto, S., et al. (2017). Irrigation and fruit canopy position modify oil quality of olive trees (cv Frantoio). J. Sci. Food Agric. 97, 3530–3539. doi: 10.1002/jsfa.8207
Caponio, F., Squeo, G., Monteleone, J. I., Paradiso, V. M., Pasqualone, A., Summo, C. (2015). First and second centrifugation of olive paste: influence of talc addition on yield, chemical composition and volatile compounds of the oils, LWT. Food Sci.Technol. 64, 439–445. doi: 10.1016/j.lwt.2015.05.007
Chartzoulakis, K. (2005). Salinity and olive: growth, salt tolerance, photosynthesis and yield. Agric. Water Manag. 78, 108–121. doi: 10.1016/j.agwat.2005.04.025
Chartzoulakis, K. (2011). The use of saline water for irrigation of olives: effects on growth, physiology, yield and oil quality. Acta Hortic. 888, 97–108. doi: 10.17660/ActaHortic.2011.888.10
Cherbiy-Hoffmann, S. U., Hall, A. J., Rousseaux, M. C. (2013). Fruit, yield, and vegetative growth responses to photosynthetically active radiation during oil synthesis in olive trees. Sci. Hortic. 150, 110–116. doi: 10.1016/j.scienta.2012.10.027
Cirilli, M., Caruso, G., Gennai, C., Urbani, S., Frioni, E., Ruzzi, M., et al. (2017). The role of polyphenoloxidase peroxidase and β-Glucosidase in phenolics accumulation in Olea europaea L. fruits under different water regimes. Front. Plant Sci. 8, 717. doi: 10.3389/fpls.2017.00717
Clodoveo, M. L., Camposeo, S., Amirante, R., Dugo, G., Cicero, N., Boskou, D. (2015). “Olives and Olive Oil Bioactive Constituents,” in Research and innovative approaches to obtain virgin olive oils with a higher level of bioactive constituents. Ed. Boskou, D. (Urbana, IL – USA: AOCS Press), 179–216. ISBN: 978-1-630670-41-2. 7 cit. doi: 10.1016/B978-1-63067-041-2.50013-6
Cui, N., Du, T., Li, F., Tong, L., Kang, S., Wang, M., et al. (2009). Response of vegetative growth and fruit development to regulated deficit irrigation at different growth stages of pear-jujube tree. Agric. Water Manag. 96, 1237–1246. doi: 10.1016/j.agwat.2009.03.015
D.L. 185/2003. Decreto Ministeriale 12 giugno, and 2003, n. 185. Regolamento recante norme tecniche per il riutilizzo delle acque reflue in attuazione dell’articolo 26, comma 2, del D.Lgs. 11 maggio 1999, n. 152.
EC. (2018). Regulation of the European parliament and of the council on minimum requirements for water reuse. European Community (EC). Brussels, 28.5.2018 COM 337.
EEC. (2011). European Commission Regulation No 61/2011 of 24 January 2011 amending Regulation (EEC) No 2568/91 on the characteristics of olive oil and olive-residue oil and on the relevant methods of analysis. Official Journal of the European Union. L 23/1, 27.1.
Erel, R., Eppel, A., Yermiyahu, U., Ben-Gal, A., Levy, G., Zipori, I., et al. (2019). Long-term irrigation with reclaimed wastewater: Implications on nutrient management, soil chemistry and olive (Olea europaea L.) performance. Agric.Water Manag. 213 (1), 324–335. doi: 10.1016/j.agwat.2018.10.033
EU (2012). Commision regulation (EU) No 432/2012 of 16 May 2012 establishing a list of permitted health claims made on foods, other than those referring to the reduction of disease risk and to children’s development and health. European Commision.
FAO. (2018). “Coping with Water Scarcity in Agriculture: a Global Framework for Action in a Changing Climate,” in Water Scarcity – One of the greatest challenges of our time(Rome, Italy), 4. ISBN: No ISBN. Job Number: I5604E; available online: http://www.fao.org/fao-stories/article/en/c/1185405/?utm_source=facebook&utm_medium=social+media&utm_campaign=fao.
Farinelli, D., Ruffolo, M., Boco, M., Tombesi, A. (2012). Yield efficiency and mechanical harvesting with trunk shaker of some International olive cultivars. Acta Hortic. 949, 379–384. doi: 10.17660/ActaHortic.2012.949.55
Fernández, J., Perez-Martin, A., Torres-Ruiz, J., Cuevas, M., Rodriguez-Dominguez, C., Elsayed-Farag, S., et al. (2013). A regulated deficit irrigation strategy for hedgerow olive orchards with high plant density. Plant Soil 372, 295–297. doi: 10.1007/s11104-013-1704-2
Folin, O., Ciocalteau, V. (1927). On tyrosine and tryptophan determination in protein. J. Biol. Chem. 73, 627–650.
García, J. M., Cuevas, M. V., Fernández, J. E. (2013). Production and oil quality in ‘Arbequina’ olive (Olea eurpoaea L.) trees under two deficit irrigation strategies. Irrig. Sci. 31 (3), 359–370. doi: 10.1007/s00271-011-0315-z
Gelly, M., Recasens, I., Girona, J., Mata, M., Arbones, A., Rufat, J., et al. (2003). Effects of water deficit during stage II of peach fruit development and post harvest on fruit quality and ethylene production. J. Hortic. Sci. Biotechnol. 78, 324–330. doi: 10.1080/14620316.2003.11511626
Ghrab, M., Ayadi, M., Gargouri, K., Chartzoulakis, K., Gharsallaoui, M., Bentaher, H., et al. (2014). Long-term effects of partial root-zone drying (PRD) on yield, oil composition and quality of olive tree (cv. Chemlali) irrigated with saline water in arid land. J. Food Compos. Anal. 36, 90–97. doi: 10.1016/j.jfca.2014.05.005
Gispert, J. R., Ramírez de Cartagena, F., Villar, J. M., Girona, J. (2013). Wet soil volume and strategy effects on drip-irrigated olive trees (cv. ‘Arbequina’). Irrig. Sci. 31, 479–489. doi: 10.1007/s00271-012-0325-5
Global Water Market. (2017). Meeting the world’s water and wastewater needs until 2020. Global Water Intell. 15.
Godini, A., Vivaldi, G. A., Camposeo, S. (2011). Olive cultivars field-tested in super high-density system in southern Italy. Calif. Agric. 65 (1), 39–40. doi: 10.3733/ca.v065n01p39
Gómez-del-Campo, M., Centeno, A., Connor, D. J. (2009). Yield determination in olive hedgerow orchards. I. Yield and profiles of yield components in north–south and east–west oriented hedgerows. Crop Pasture Sci. 60, 434–442. doi: 10.1071/CP08252
Gómez-del-Campo, M. (2013). Summer deficit-irrigation strategies in a hedgerow olive orchard cv ‘Arbequina’: effect on fruit characteristics and yield. Irrig. Sci. 31, 259–269. doi: 10.1007/s00271-011-0299-8
Gómez-del-Campo, M., García, J. M. (2013). Summer deficit-irrigation strategies in a Hedgerow Olive cv. Arbequina orchard: effect on oil quality. J. Agric. Food Chem. 61 (37), 8899–8905. doi: 10.1021/jf402107t
Gucci, R., Tattini, M. (1997). Salinity tolerance in olive. Hortic. Rev. 21, 177–214. doi: 10.1002/9780470650660.ch6
Gucci, R., Lodolini, E. M., Rapoport, H. F. (2007). Productivity of olive trees with different water status and crop load. J. Hortic. Sci. Biotechnol. 82, 648–656. doi: 10.1080/14620316.2007.11512286
Gucci, R., Lodolini, E. M., Rapoport, H. F. (2009). Water deficit-induced changes in mesocarp cellular processes and the relationship between mesocarp and endocarp during olive fruit development. Tree Physiol. 29, 1575–1585. doi: 10.1093/treephys/tpp086
Gucci, R., Caruso, G., Gennai, C., Esposto, S., Urbani, S., Servili, M. (2019). Fruit growth, yield and oil quality changes induced by deficit irrigation at different stages of olive fruit development. Agric. Water Manag. 212, 88–98. 212. doi: 10.1016/j.agwat.2018.08.022
Hernández, M. L., Velázquez-Palermo, D., Sicardo, M. D., Fernández, J. E., Díaz-Espejo, A., Marínez-Rivas, J. M. (2018). Effect of a regulated deficit irrigation strategy in a hedgerow ‘Arbequina’ olive orchard on the mesocarp fatty acid composition and desaturase gene expression with respect to olive oil quality. Agric. Water Manag. 204, 100–106. doi: 10.1016/j.agwat.2018.04.002
Hernandez-Santana, V., Fernandes, R. D. M., Perez-Arcoiza, A., Fernández, J. E., Garcia, J. M., Diaz-Espejo, A. (2018). Relationships between fruit growth and oil accumulation with simulated seasonal dynamics of leaf gas exchange in the olive tree. Agric. For. Meteorol. 256–257, 458–469. doi: 10.1016/j.agrformet.2018.03.019
Intrigliolo, D. S., Castel, J. R. (2010). Response of plum trees to deficit irrigation under two crop levels: tree growth yield and fruit quality. Irrig. Sci. 28, 525–534. doi: 10.1007/s00271-010-0212-x
ISTAT. (2017). Istituto Nazionale di Statistica (http://agri.istat.it).
ISMEA. (2018). Istituto di Servizi per il Mercato Agricolo Alimentare. (http://ismea.it).
Kchaou, H., Larbi, A., Gargouri, K., Chaieb, M., Morales, F., Msallem, M. (2010). Assessment of tolerance to NaCl salinity of five olive cultivars based on growth characteristics and Na+ and Cl– exclusion mechanisms. Sci. Hort. 124 (3), 306–315. doi: 10.1016/j.scienta.2010.01.007
Lavee, S., Hanoch, E., Wodner, M., Abramowitch, H. (2007). The effect of predetermined deficit irrigation on the performance of the cv. Muhasan olive (Olea europaea L.) in the eastern coastal plain of Israel. Sci. Hortic. 112, 156–163. doi: 10.1016/j.scienta.2006.12.017
Melgar, J., Mohamed, Y., Serrano, N., García-Galavís, P., Navarro, C., Parra, M., et al. (2009). Long term responses of olive trees to salinity. Agric. Water Manag. 96, 1105–1113. doi: 10.1016/j.agwat.2009.02.009
Minguez, M. I., Rejano, L., Gandul, B., Higinio, A., Garrido, J. (1991). Color pigment correlation in virgin olive oil. J. Am. Oil Chem. Soc. 68, 332–336. doi: 10.1007/BF02657688
Moriana, A., Orgaz, F., Pastor, M., Fereres, E. (2003). Yield responses of a mature olive orchard to water deficits. J. Am. Soc. Hortic. Sci. 128 (3), 425–431. doi: 10.21273/JASHS.128.3.0425
Patumi, M., D’Andria, R., Marsilio, V., Fontanazza, G., Morelli, G., Lanza, B. (2002). Olive and olive oil quality after intensive monocone olive growing (Oleaeuropaea L., cv. Kalamata) in different irrigation regimes. Food Chem. 77, 27–34.
Petousi, I., Fountoulakis, M., Saru, M., Nikolaidis, N., Fletcher, L., Stentiford, E., et al. (2015). Effects of reclaimed wastewater irrigation on olive (Olea europaea L. cv ‘Koroneiki’) trees. Agric. Water Manag. 160, 33–40. doi: 10.1016/j.agwat.2015.06.003
Perez-Pastor, A., Ruiz-Sanchez, M. C., Domingo, R. (2014). Effects of timing and intensity of deficit irrigation on vegetative and fruit growth of apricot trees. Agric. Water Manag. 134, 110–118. doi: 10.1016/j.agwat.2013.12.007
Rallo, G., Provenzano, G. (2013). Modelling eco-physiological response of table olive trees (Olea europaea L.) to soil water deficit conditions. Agric. Water Manage. 120, 79–88. doi: 10.1016/j.agwat.2012.10.005
Riemenschneider, C., Al-Raggad, M., Moeder, M., Seiwert, B., Salameh, E., Reemtsma, T. (2016). Pharmaceuticals, their metabolites, and other polar pollutants in field-grown vegetables irrigated with treated municipal wastewater. J. Agric. Food Chem. 64 (29), 5784–5792. doi: 10.1021/acs.jafc.6b01696
Rinaldi, R., Amodio, M. L., Colelli, G., Nanos, G. D., Pliakoni, E. (2011). Effect of deficit irrigation on fruit and oil quality of ‘Konservolea’ Olives. Acta Hortic. 924, 445–451. doi: 10.17660/ActaHortic.2011.924.57
Romero-Trigueros, C., Nortes Tortosa, P. A., Alarcon Cabañero, J. J., Nicolas Nicolás, E. (2014). Determination of 15N stable isotope natural abundances for assessing the use of saline reclaimed water in grapefruit. Environ. Eng. Manag. J. 13, 2525–2530. doi: 10.30638/eemj.2014.282
Romero-Trigueros, C., Parra, M., Bayona Gambín, J. M., Nortes Tortosa, P., Alarcon Cabañero, J. J., Nicolás Nicolás, E. (2017). Effect of deficit irrigation and reclaimed water on yield and quality of grapefruits at harvest and postharvest. LWT – Food Sci. Technol. 85, 405–411. doi: 10.1016/j.lwt.2017.05.001
Romero-Trigueros, C., Bayona Gambín, J. M., Nortes Tortosa, P., Alarcón Cabañero, J. J., Nicolás Nicolás, E. (2019). Determination of crop water stress index byi thermometry in grapefruit trees irrigated with saline reclaimed water combined with deficit irrigation. Remote Sens. 11 (7), 757. doi: 10.3390/rs11070757
Rosecrance, R. C., Krueger, W. H., Milliron, L., Bloese, J., Garcia, C., Mori, B. (2015). Moderate regulated deficit irrigation can increase olive oil yields and decrease tree growth in super high density ‘Arbequina’ olive orchards. Sci. Hortic. 190, 75–82. doi: 10.1016/j.scienta.2015.03.045
Saadati, S., Moallemi, N., Mortazavi, S. M. H., Seyyednejad, S. M. (2013). Effects of zinc and boron foliar application on soluble carbohydrate and oil contents of three olive cultivars during fruit ripening. Sci. Hortic. 164, 30–34. doi: 10.1016/j.scienta.2013.08.033
Scholander, P. F., Hammel, H. T., Bradstreet, E. D., Hemmingsen, E. A. (1965). Sap pressure in vascular plants: negative hydrostatic pressure can be measured in plants. Sci. 148, 339–346. doi: 10.1126/science.148.3668.339
Segal, E., Dag, A., Ben-Gal, A., Zipori, I., Erel, R., Suryano, S., et al. (2011). Olive orchard irrigation with reclaimed wastewater: agronomic and environmental considerations. Agric. Ecosyst. Environ. 140, 454–461. doi: 10.1016/j.agee.2011.01.009
Servili, M., Selvaggini, R., Esposto, S., Taticchi, A., Montedoro, G., Morozzi, G. (2004). Health and sensory properties of virgin olive oil hydrophilic phenols: agronomic and technological aspects of production that affect their occurrence in the oil. J. Chromatogr. A. 1054, 113–127. doi: 10.1016/S0021-9673(04)01423-2
Servili, M., Esposto, S., Lodolini, E. M., Selvaggini, R., Taticchi, A., Urbani, S., et al. (2007). Irrigation effects on quality phenolic composition and selected volatiles of virgin olive oil cv Leccino. J. Agric. Food Chem. 55, 6609–6618. doi: 10.1021/jf070599n
Shackel, K. A., Ahmadi, H., Biasi, W., Buchner, R., Goldhamer, D., Gurusinghe, S. (1997). Plant water status as an index of irrigation need in deciduous fruit trees. Horttechnology 7, 23–29. doi: 10.21273/HORTTECH.7.1.23
Simopoulos, A. P. (2016). An increase in the omega-6/omega-3 fatty acid ratio increases the risk for obesity. Nutrients 8, 128. doi: 10.3390/nu8030128
Stewart, W. L., Fulton, A. E., Krueger, W. H., Lampinen, B. D., Shackel, K. A. (2011). Regulated deficit irrigation reduces water use of almonds without affecting yield. Calif. Agric. 65, 90–95. doi: 10.3733/ca.v065n02p90
Tietel, Z., Dag, A., Yermiyahu, U., Zipori, I., Beiersdorf, I., Krispina, S., et al. (2019). Irrigation-induced salinity affects olive oil quality and health-promoting properties. J. Sci. Food Agric. 99, 1180–1189. doi: 10.1002/jsfa.9287
Tattini, M., Bertoni, P., Caselli, S. (1992). Genotypic responses of olive plants to sodium chloride. J. Plant Nutr. 15, 1467–1485. doi: 10.1080/01904169209364412
Vivaldi, G. A., Stripolli, G., Pascuzzi, S., Stellacci, A. M., Camposeo, S. (2015). Olive genotypes cultivated in an adult high-density orchard respond differently to canopy restraining by mechanical and manual pruning. Sci. Hort. 192, 391–399. doi: 10.1016/j.scienta.2015.06.004
Keywords: acidity, Arbosana, fruit weight, oil extractability, oleic acid, polyphenol, peroxide, total production
Citation: Romero-Trigueros C, Vivaldi GA, Nicolás EN, Paduano A, Salcedo FP and Camposeo S (2019) Ripening Indices, Olive Yield and Oil Quality in Response to Irrigation With Saline Reclaimed Water and Deficit Strategies. Front. Plant Sci. 10:1243. doi: 10.3389/fpls.2019.01243
Received: 14 June 2019; Accepted: 06 September 2019;
Published: 09 October 2019.
Edited by:
Antonio Diaz Espejo, Institute of Natural Resources and Agrobiology of Seville (CSIC), SpainReviewed by:
Maria Cecilia Rousseaux, Centro Regional de Investigaciones Científicas y Transferencia Tecnológica de La Rioja (CRILAR CONICET), ArgentinaGregorio Egea, University of Seville, Spain
Copyright © 2019 Romero-Trigueros, Vivaldi, Nicolás, Paduano, Salcedo and Camposeo. This is an open-access article distributed under the terms of the Creative Commons Attribution License (CC BY). The use, distribution or reproduction in other forums is permitted, provided the original author(s) and the copyright owner(s) are credited and that the original publication in this journal is cited, in accordance with accepted academic practice. No use, distribution or reproduction is permitted which does not comply with these terms.
*Correspondence: Emilio Nicolás Nicolás, emilio@cebas.csic.es