- 1Dipartimento Tecnologie Energetiche (DTE), Centro Ricerche Trisaia, ENEA Agenzia nazionale per le nuove tecnologie, l’energia e lo sviluppo economico sostenibile, Rotondella, Italy
- 2Dipartimento di Scienze Bio Agroalimentari (DiSBA), Istituto di Bioscienze e Biorisorse (IBBR), Consiglio Nazionale Delle Ricerche (CNR), Perugia, Italy
- 3Department of Plant Physiology, Umeå Plant Science Centre, Umeå University, Umeå, Sweden
- 4Laboratorio di Genomica, Dipartimento di Agronomia, Animali, Alimenti, Risorse naturali e Ambiente (DAFNAE), Università di Padova, Legnaro, Italy
Olive, representing one of the most important fruit crops of the Mediterranean area, is characterized by a general low fruit yield, due to numerous constraints, including alternate bearing, low flower viability, male-sterility, inter-incompatibility, and self-incompatibility (SI). Early efforts to clarify the genetic control of SI in olive gave conflicting results, and only recently, the genetic control of SI has been disclosed, revealing that olive possesses an unconventional homomorphic sporophytic diallelic system of SI, dissimilar from other described plants. This system, characterized by the presence of two SI groups, prevents self-fertilization and regulates inter-compatibility between cultivars, such that cultivars bearing the same incompatibility group are incompatible. Despite the presence of a functional SI, some varieties, in particular conditions, are able to set seeds following self-fertilization, a mechanism known as pseudo-self-compatibility (PSC), as widely reported in previous literature. Here, we summarize the results of previous works on SI in olive, particularly focusing on the occurrence of self-fertility, and offer a new perspective in view of the recent elucidation of the genetic architecture of the SI system in olive. Recent advances in research aimed at unraveling the molecular bases of SI and its breakdown in olive are also presented. The clarification of these mechanisms may have a huge impact on orchard management and will provide fundamental information for the future of olive breeding programs.
Introduction
Olive (Olea europaea L.) is a perennial diploid species mainly clonally propagated and diffused in the Mediterranean area as one of the oldest tree crops (Loumou and Giourga, 2003; McKey et al., 2010; Zohary et al., 2012; Mousavi et al., 2017). As in many other allochthonous, hermaphrodite, wind-pollinated species, olive is characterized by a plentiful flowering, followed by a poor fruit set, which results in low yields (Cuevas and Polito, 2004; Ben Dhiab et al., 2017; Kassa et al., 2019). Environmental conditions, such as temperature, rainfall, and wind, may strongly affect flowering time, flowering intensity, and fertilization (Fernandez-Escobar et al., 2008; Haberman et al., 2017; Benlloch-González et al., 2018). Efficient pollination depends on many factors, such as the presence of exogenous compatible pollen, the duration of stigma receptivity, the number of pollen grains, pollen-ovule ratio, and stigma morphology (Cruden, 2000; García-Mozo et al., 2004; Pinillos and Cuevas, 2009; Rojo et al., 2016). Despite the importance of these factors, the main constraints responsible for the low fruit setting of olive are undoubtedly self-incompatibility (SI) and a high percentage of ovary abortion of some cultivars (Reale et al., 2009; Seifi et al., 2015).
Self-incompatibility is one of the most effective systems adopted by flowering plants to prevent inbreeding and maintain a high diversity within species (Ferrer and Good, 2012). Within the sporophytic (SSI) and gametophytic (GSI) categories, several incompatibility systems have been reported, but only three of them have been characterized at molecular level, one for SSI (Brassicaceae) and two for GSI (one in Solanaceae, Plantaginaceae, and Rosaceae, and one for Papaveraceae) (Higashiyama, 2010). The recent discovery of the diallelic SSI system in olive and other related genera has provided evidence on the SI system operating in the Oleaceae family. In particular, it has been clarified that the inhibition of pollen tube growth takes place at the stigma, and it has been established that there are only two incompatibility groups, i.e., diallelic SI, such that olive cultivars are incompatible within groups and compatible between groups (Saumitou-Laprade et al., 2017a).
In light of this evidence, it has become even more difficult to explain the self-fertility of some varieties, which has been confirmed by numerous studies (Seifi et al., 2012; Selak et al., 2014a; Breton et al., 2016; Marchese et al., 2016a). It is also now clear that pseudo-self-compatibility (PSC)—the failure to reject self-pollen despite the presence of a functional SI system—may occur in olive, and all previous reports on olive self-fertility need to be re-interpreted or ignored when not confirmed by paternity tests of seeds deriving from selfing. In this review, we hope to shed some light on the complexity of SI system in olive, particularly focusing on the evidence for PSC, providing a synthesis of the fragmented work on this topic and a new perspective on SI in olive, supported by experimental work from our laboratory.
Olive Self-Incompatibility
For a long time, olive has been erroneously classified as a GSI species, based on morphological traits shared with taxa manifesting a GSI system, such as wet-type stigma and bi-nucleate pollen (Serrano et al., 2008); however, only a few cytological studies supported the occurrence of this kind of SI system (Serrano et al., 2010). Recently, it has been demonstrated that the GSI model fails to explain the presence of reciprocal differences in fruit set in one-third of mates (Breton et al., 2014; Farinelli et al., 2018). This evidence, together with others, such as the inhibition of germination at the stigma and the failure to identify genes controlling GSI in olive (Collani et al., 2012), led to the hypothesis that olive may have a SSI system. The wide methodical genetic study carried out by Saumitou-Laprade et al. (2017a,b) definitively confirmed that olive has a sporophytic homomorphic diallelic system, similar to that identified in close species, such as Phillyrea angustifolia and Fraxinus ornus (Saumitou-Laprade et al., 2010; Vernet et al., 2016) and different from any other described in other plant families. The SSI system has been classified as diallelic because controlled by a single locus with only two alleles (S1 and S2), according to the segregation analysis of SI trait in a cross population (Saumitou-Laprade et al., 2017a). Thus, only two SI genotypes have been found so far, and olive cultivars can be classified, based on their belonging to the SI group, as G1 = S1S2 or G2 = S1S1. First evidence on the olive diallelic SSI system indicated that it is not accompanied by heterostyly (Saumitou-Laprade et al., 2017a); however, dedicated studies to confirm this preliminary data were not conducted.
The general behavior of pollen within pistil tissues, under self- or incompatible cross-pollination, is represented in Figure 1. After self-pollination (Figure 1, panels A–G), most of the pollen grains landing on the stigmatic surface do not germinate, and others start germinating but do not penetrate nor grow into the transmitting tissue of the style, indicating that inhibition of pollen tube growth takes place at stigmatic level, according to the sporophytic nature of the olive SI. By contrast, during cross-pollination with compatible pollen (Figure 1, panels H–J), while a high number of pollen grains germinate on the stigma and grow toward the style, a few of them are also able to penetrate the transmitting tissue of the style and reach the ovule. Generally, only one pollen tube (and rarely two or three) grow toward the ovary and reach the carpels to penetrate an ovule (Ateyyeh et al., 2000; Seifi et al., 2011).
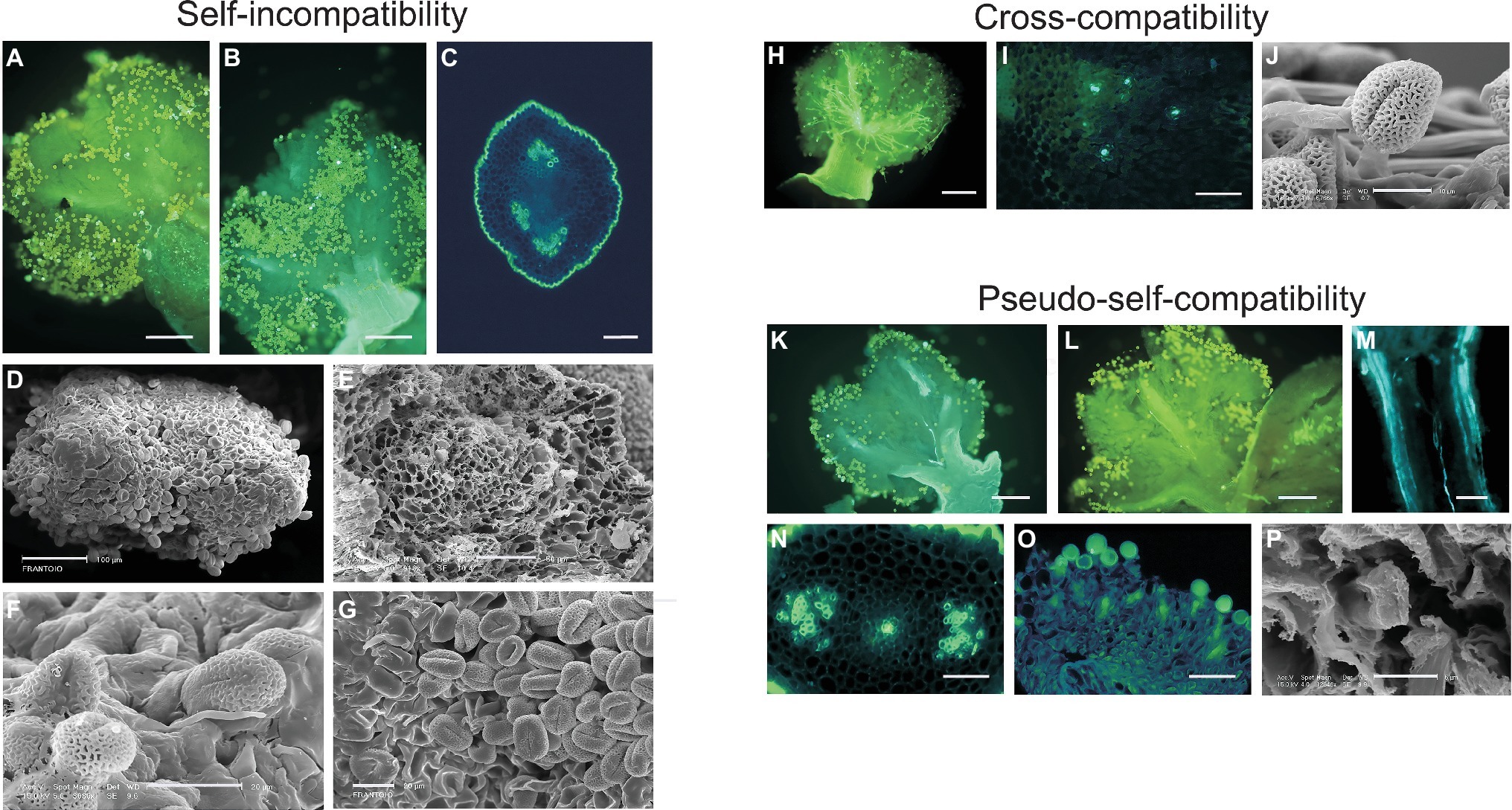
Figure 1. Self-incompatibility, cross-compatibility, and pseudo-self-compatibility in olive. (A–G) Self-incompatibility reaction in flowers of cultivars Frantoio, Leccino, and Dolce Agogia at 6 and 15 days after anthesis, as observed by epifluorescence images after aniline blue staining (A–C) and scanning electron microscope (SEM) images (D–G). Pistils of cv. Frantoio (A,B) and transversal sections of style of cv. Leccino (C) and cv. Dolce Agogia (E) showing the absence of pollen tube growth in the style. Stigma of cv. Frantoio (D,G) and cv. Leccino (F) showing the absence of germinated pollen grains into the style. Bars = 150 μm (A,B), 100 μm (D), 50 μm (C,E), and 20 μm (F,G). (H–J) Cross-compatibility of cv. Frantoio (incompatibility group G1), pollinated with compatible G2 pollen, as observed by epifluorescence images after aniline blue staining (H,I) and SEM image (J). Cross-pollination at 72 h after anthesis showing pollen-pistil compatibility (H). Transversal section of style showing pollen tube growth throughout the pistil (I). Stigma showing germinated pollen grains (J). Bars = 150 μm (H), 50 μm (I), and 10 μm (J). (K–P): Pseudo-self-compatibility in self-pollinated flowers, as observed by epifluorescence images after aniline blue staining (K–O) and SEM image (P). Flowers were collected at 6 (M–P) and 15 (K,L) days after anthesis. Pistils showing pollen tube growth in the style (K–M,O). Transversal sections of style showing pollen tube growth through the stylar tissues (N,P). Bars = 150 μm (K–M), 50 μm (N–O), and 6 μm (P).
It has also been observed that the SI group or the genotype of the pollen recipient may have a significant effect on the length that pollen tubes may reach in the stigma prior to being arrested due to the SI reaction. In general, in G1 plants, pollen grains do not germinate at all or tube growth stops shortly after germination, whereas a higher variability can be observed in G2 plants, characterized by short or long pollen tube growth (Saumitou-Laprade et al., 2017a). This behavior, which probably contributed to the earlier difficulties in the classification of olive as GSI or SSI species, might be explained with a different timing of the SI response after the recognition of self-pollen.
Olive Pseudo-Self-Compatibility
The current knowledge on olive SI system indicates that all olive cultivars are self-incompatible (Saumitou-Laprade et al., 2017a); however, some of them can behave as PSC in particular conditions (probably due to both genetic and environmental factors), being able to overcome SI and produce seeds after self-pollination (Breton et al., 2016; Marchese et al., 2016a; Saumitou-Laprade et al., 2017a). For a long time, olive cultivars were classified into self-compatible and self-incompatible cultivars, with most of them considered as self-incompatible (Wu et al., 2002; Mookerjee et al., 2005; Seifi et al., 2011; Sánchez-Estrada and Cuevas, 2018). Self-compatibility tests carried out in several studies have shown a low but certain rate of self-fertilizing cultivars, that were, consequently, considered to be partially self-compatible (Androulakis and Loupassaki, 1990; Ateyyeh et al., 2000; Moutier, 2002; Kasasbeh et al., 2005; Selak et al., 2011; Taslimpour and Aslmoshtaghi, 2013; Breton et al., 2014; Koubouris et al., 2014; Farinelli et al., 2018). Unfortunately, due to the lack of molecular tests to assess the origin of putatively selfed seeds and the use of unreliable materials and protocols for cross- and self-pollination experiments, some varieties were erroneously considered as self-compatible and were later proved totally self-incompatible. As an example, cv. Arbequina, previously classified as self-compatible (Cuevas, 2005), was then shown as self-incompatible (Díaz et al., 2006; Marchese et al., 2016b). Similarly, as a probable consequence of the multiple factors affecting self-fertilization success, some varieties were considered self-incompatible, as cv. Koroneiki (Mookerjee et al., 2005; Seifi et al., 2011), that later on definitively showed a low but reliable self-fertilization rate (Marchese et al., 2016b). Differences were also found among clones of the same cultivar, as in the case of cv. Leccino, mostly resulting in self-incompatible (de la Rosa et al., 2003), but with some clones partially self-compatible (Solfanelli et al., 2006).
Based on the recent evidence about the SI system operating in olive, the cultivars considered representative of the Mediterranean cultivated olive diversity showed a clear self-incompatibility reaction and inhibition of self-pollen growth (Saumitou-Laprade et al., 2017a). These results definitively support that the genetic architecture of olive SI excludes, in theory, any form of self-fertilization. The contrasting evidence that some cultivars may produce selfed progeny hints that a mechanism of incompatibility breakdown exists and that this mechanism is presently still unknown. In view of these findings, we can now conclude that olive shows PSC. This behavior can explain the contradictory data on the self-compatibility tests, and it is in agreement with previous literature reporting that the percent of successful self-fertilization was significantly lower than the fertilization rate under open- or cross-pollination (Griggs et al., 1975; Ateyyeh et al., 2000; Kasasbeh et al., 2005; Mookerjee et al., 2005). The production of truly self-seedlings has been confirmed by numerous studies where paternity tests have been applied (de la Rosa et al., 2004; Mookerjee et al., 2005; Marchese et al., 2016b).
PSC pollen growth reaction seems completely different from the compatibility response observed under pollination with compatible pollen. In self-pollinated pistils of cv. Frantoio, in fact, in most cases, pollen grains do not germinate at all, even after 6 and 15 days after flower opening and pollen-stigma contact (Figure 1, panels K and L), but in few cases, one or few pollen tubes may grow into the style and likely reach the ovary (Figure 1, panels M–P).
Factors Affecting the Pseudo-Self-Compatibility
Pseudo-self-compatibility has been observed in numerous self-incompatible species in Asteraceae, Brassicaceae, Fabaceae, Poaceae, Ranunculaceae, Solanaceae, and other families (Good-Avila et al., 2008; Crawford et al., 2015; Liao et al., 2016). In these species, numerous external and internal factors seem to affect the ability of plants to overcome SI barrier, including the pollen germination speed, the relative growth rate of self-pollen tubes compared to cross-pollen grains, and the flower aging (Levin, 1996; Stephenson et al., 2000; Good-Avila et al., 2008; Horisaki and Niikura, 2008). The factors affecting the overcome of olive SI system are not yet known, but the available data indicate that both genotypic and environmental factors can play a role in this process. By contrast, flower age does not seem to affect PSC, considering that it has been observed in stigmas of all developmental stages.
Environmental Factors
It is well established that environmental conditions may affect self-fertility of self-incompatible plants. In particular, SI can be overcome by high temperatures (Okazaki and Hinata, 1987; Wilkins and Thorogood, 1992; Horisaki and Niikura, 2008), high humidity (Ockendon, 1978), and chemical treatments (Lao et al., 2014; Yang et al., 2018). Also, different environments and artificial pollination techniques may favor self-fertility (Do Canto et al., 2016).
In accordance with these studies, it has been reported that environmental conditions may affect the SI reaction and fruit set in olive, and, among them, temperature appears to play a key role (Orlandi et al., 2010; Selak et al., 2013; Haberman et al., 2017). It is thought, in fact, that SI in olive is temperature-dependent (Suárez et al., 2012), and generally, high temperatures during flowering may reduce self-fertilization rate (Ayerza and Coates, 2004; Selak et al., 2013). However, the effect of temperature seems strongly genotype-dependent (Griggs et al., 1975; Koubouris et al., 2009; Selak et al., 2013). Furthermore, temperature variations strongly influence the ability of olive pollen to grow and germinate (Koubouris et al., 2009), as well as it influences stigma receptivity and ovule longevity (Selak et al., 2014b), thus affecting the fruit set (Cuevas et al., 1994).
According to the role of environmental factors in the PSC expression, results from studies on self-fertilization of olive cultivars varied particularly among different environmental conditions. For instance, variability in the self-fertilization rate has been observed among different experimental years (Griggs et al., 1975; Cuevas et al., 2001; Lavee et al., 2002; Solfanelli et al., 2006; Selak et al., 2011), orchard location (Selak et al., 2011), or different conditions, as those determined by the use of polyethylene cages (Selak et al., 2013). However, considering the high number of environmental factors changing among location and years, the available data do not allow to identify, with the exception of temperature, other key environmental factors affecting self-fertility.
Genotype-Dependent Factors
In addition to the environmental effects, PSC appears to be strongly influenced by olive genotype, considering that self-fertilization has been exclusively observed in specific olive varieties and never reported in others. For example, successful self-fertilization in cv. Frantoio has been reported in numerous studies (Kasasbeh et al., 2005; Farinelli et al., 2008; Spinardi and Bassi, 2012; Breton et al., 2014), despite a functional SI system is also present in this variety.
Our histological and molecular study confirmed the ability of cv. Frantoio to overcome SI. By contrast, PSC was not observed for other varieties, such as cvs. Leccino and Dolce Agogia (Figure 2A), in agreement with other authors (Spinardi and Bassi, 2012; Farinelli et al., 2018). According to these data, paternity tests with microsatellite markers performed on seeds of cv. Koroneiki, Manzanilla, Cacereña, and Manzanilla de Sevilla obtained by self-pollination (Figure 2B), confirmed the origin of zygotic embryos by effective self-fertilization (Saumitou-Laprade et al., 2017a). These results validated the ability of some olive cultivars to overcome the SI barrier and confirm the occurrence of PSC.
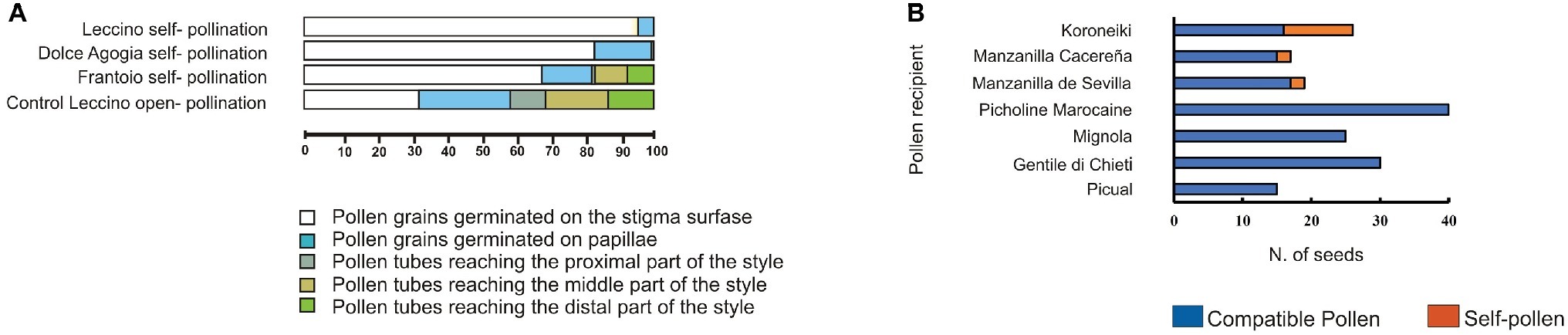
Figure 2. Pseudo-self-compatibility data in olive cultivars. (A) Variation of pseudo-self-compatibility of three olive genotypes observed under field conditions. Percentage of pollen tubes germinated in different pistil tissues of cv. Leccino and Frantoio (G1 incompatibility group) and cv. Dolce Agogia (G2 incompatibility group) was determined analyzing a total of 34.000 pollen grains. Samples were collected at 6 days after anthesis in both self-pollinated and open-pollinated (control) flowers. Pollen grains were divided into five different classes (different colors). About 9.5% of cv. Frantoio self-pollen tubes grew through the transmitting tissues reaching the distal part of the style, indicating pseudo-self-compatibility. The few pollen grains of cv. Dolce Agogia, reaching the proximal part of the style, are presumably due to slight differences in the incompatibility response of the G2 incompatibility group compared to G1. (B) The number of seeds obtained from pollination with compatible and self-pollen in seven cultivars under field conditions. Paternity was validated by using microsatellite markers.
Similar to olive, a significant difference in PSC among genotypes has been reported in other plants (Foster and Wright, 1970; Elgersma et al., 1989; Brennan et al., 2005; Baldwin and Schoen, 2017). According to the quantitative nature of PSC, this trait is typically polygenic (Do Canto et al., 2016). In some Brassicaceae species, variation in PSC has been shown to be caused by genetic variation in genes unlinked to the S-locus but involved in the SI signaling cascade that mediates the rejection of self-pollen (Liu et al., 2007; Baldwin and Schoen, 2017). This pattern has also been confirmed in either GSI or SSI species (Good-Avila and Stephenson, 2002; Mable et al., 2005; Mena-Ali and Stephenson, 2007; Crawford et al., 2015; Liao et al., 2016).
The hypothesized architecture of the S-locus in olive (G1 = S1S2 and G2 = S1S1), which guarantees a perfect 1:1 balance between the two groups (Saumitou-Laprade et al., 2017a), implies that only out-crossing between the two groups could preserve these two unique combinations, while the selfing of the heterozygous group would result in the appearance of the homozygous combination S2S2, which would lead to the imbalance of the populations in favor of one group with respect to the other. Furthermore, first evidence demonstrates that cross-pollination between self-fertile varieties and genotypes belonging to the same SI group never occurs (Saumitou-Laprade et al., 2017a). This complex picture of the paradoxical occurrence of PSC in olive is difficult to explain, also assuming the presence of a “leaky S-allele,” as observed in other plant species (Baldwin and Schoen, 2017).
Toward the Identification of Molecular Determinants of Self-Incompatibility and Pseudo-Self-Compatibility
Further studies should be carried out, either at microscopic, genomic, and genetic levels, in order to understand the mechanisms underpinning the PSC in olive, although, it is also crucial to increase the knowledge of the molecular events occurring during the SI reaction which remain still unexplored. Studies aimed at identifying female and male determinants of the olive SI system, based on gene similarity to other plant species in which SI was molecularly characterized, have been conducted, but results of these studies indicated that olive flowers do not possess or express genes similar to the GSI determinants identified in other plants (Collani, 2012; Collani et al., 2012). On the contrary, candidate genes for female (OeSRK-like and OeSLG-like) and male (OeSCR-like) determinants, as orthologous of the genes that control the SSI system in the Brassicaceae family, have been cloned and characterized in olive varieties (Collani, 2012; Collani et al., 2012), and gene expression studies showed that the OeSRK-like gene is preferentially expressed in pistils at early flowering stages of cv. Leccino and lowly expressed in pistils at later flowering stages of cv. Frantoio, while OeSCR-like was found specifically expressed in dehiscent anthers of both cultivars (Collani, 2012). Despite such positive initial evidences, further genetic and molecular findings clearly demonstrated that these genes do not encode for the genetic determinants of the olive S-locus, as independent segregation of OeSRK-like and OeSCR-like genes was documented by means of SNP-based markers and no interaction between OeSRK-like and OeSCR-like proteins was observed by Yeast-2-Hybrid screens (data not shown). Although the role of these genes remains to be disclosed, our negative results corroborate an olive SI system whose genetic determinism is different from that active in Brassicaceae and from the others molecularly characterized, according to the peculiar features of olive SSI. This result supports the hypothesis that independent evolution of multiple SI systems has occurred in flowering plants (Ferrer and Good, 2012).
Activity and localization of enzymes, such as RNases, putatively involved in pollen rejection mechanism under self-pollination, have been described in olive (Serrano and Olmedilla, 2012), as well as the occurrence of programmed cell death in olive pollen, as a consequence of the SI response and the differential level of reactive oxygen and nitrogen species between self-compatible and self-incompatible pollen grains (Serrano et al., 2012). However, their role in the SI mechanism needs to be further investigated.
A high throughput transcriptomic study of olive self-pollinated flowers identified a wide set of transcripts showing extensive expression differences between pseudo-self-compatible (cv. Frantoio) and self-incompatible (cv. Leccino) cultivars, confirming that biochemical, physiological, and signaling changes occur when incompatibility is broken down (Alagna et al., 2016). These data represent a valuable resource for the identification of genes related to PSC.
In addition, several enzymes putatively involved in the regulation of pollen tube growth and in the modulation of temperature-dependent reproductive processes were identified by studying the proteomic profile of olive stigma exudate (Rejón et al., 2013). The ReprOlive database, built on the transcriptomic information of olive reproductive tissues (Carmona et al., 2015), as well as the availability of the sequence of cultivated and wild olive genomes (Cruz et al., 2016; Unver et al., 2017), provides further high valuable tools for the identification of candidate genes involved in SI signaling and for the discovery of the molecular mechanisms involved in PSC. These studies will be facilitated by the trans-generic functional homology of olive SI, which will allow for the application of discoveries from P. angustifolia and F. ornus species to olive.
Conclusions and Perspectives
The important advances made in the study of the olive SI system do not explain the occurrence of self-fertility in some cultivars, confirmed by many studies and certainly regulated by both genetic and environmental factors. New observations should be carried out in order to clarify how germination of pollen grains and their growth within the transmitting tissue of the style may occur in a context of incompatibility. The availability of genetic materials and microscopic, genomic, and transcriptomic resources for the study of olive reproductive constrains should allow the elucidation of the selfing mechanism, as well as the identification of putative genes involved in the PSC. Understanding the mechanisms regulating SI and PSC will have a huge impact on olive orchard management and breeding programs, offering new tangible opportunities for improving olive and olive oil production.
Author Contributions
LB and GB conceived the study. FA wrote the first draft of the manuscript. MEC and SP performed the histological observations. All the authors contributed to the content of the manuscript and revised the manuscript. All the authors agreed on the final version of this review.
Funding
This work was partially supported by the project BeFOre—“Bioresources for Oliviculture,” H2020-MSCA-RISE (G.A. 645595) and by the project INNO.V.O. – “Development of new varieties to face the challenges of olive growing,” PSR Umbria 2014-2020.
Conflict of Interest Statement
The authors declare that the research was conducted in the absence of any commercial or financial relationships that could be construed as a potential conflict of interest.
References
Alagna, F., Cirilli, M., Galla, G., Carbone, F., Daddiego, L., Facella, P., et al. (2016). Transcript analysis and regulative events during flower development in olive (Olea europaea L.). PLoS One 11:e0152943. doi: 10.1371/journal.pone.0152943
Androulakis, I. I., and Loupassaki, M. H. (1990). Studies on the self-fertility of some olive cultivars in the area of Crete. Acta Hortic. 286, 159–162. doi: 10.17660/ActaHortic.1990.286.30
Ateyyeh, A., Stosser, R., and Qrunfleh, M. (2000). Reproductive biology of the olive (Olea europaea L.) cultivar “Nabali Beladi”. J. Appl. Bot. 270, 255–270.
Ayerza, R., and Coates, W. (2004). Supplemental pollination—increasing olive (Olea europaea) yields in hot, arid environments. Exp. Agric. 40, 481–491. doi: 10.1017/S0014479704002133
Baldwin, S. J., and Schoen, D. J. (2017). Genetic variation for pseudo-self-compatibility in self-incompatible populations of Leavenworthia alabamica (Brassicaceae). New Phytol. 213, 430–439. doi: 10.1111/nph.14109
Ben Dhiab, A., Ben Mimoun, M., Oteros, J., Garcia-Mozo, H., Domínguez-Vilches, E., Galán, C., et al. (2017). Modeling olive-crop forecasting in Tunisia. Theor. Appl. Climatol. 128, 541–549. doi: 10.1007/s00704-015-1726-1
Benlloch-González, M., Sánchez-Lucas, R., Benlloch, M., and Ricardo, F.-E. (2018). An approach to global warming effects on flowering and fruit set of olive trees growing under field conditions. Sci. Hortic. 240, 405–410. doi: 10.1016/j.scienta.2018.06.054
Brennan, A. C., Harris, S. A., and Hiscock, S. J. (2005). Modes and rates of selfing and associated inbreeding depression in the self-incompatible plant Senecio squalidus (Asteraceae): a successful colonizing species in the British Isles. New Phytol. 168, 475–486. doi: 10.1111/j.1469-8137.2005.01517.x
Breton, C. M., Farinelli, D., Koubouris, G., and Bervillé, A. (2016). A model based on S-allele dominance relationships to explain pseudo self-fertility of varieties in the olive tree. Euphytica 210, 105–117. doi: 10.1007/s10681-016-1708-0
Breton, C. M., Farinelli, D., Shafiq, S., Heslop-Harrison, J. S., Sedgley, M., and Bervillé, A. J. (2014). The self-incompatibility mating system of the olive (Olea europaea L.) functions with dominance between S-alleles. Tree Genet. Genomes 10, 1055–1067. doi: 10.1007/s11295-014-0742-0
Carmona, R., Zafra, A., Seoane, P., Castro, A. J., Guerrero-Fernández, D., Castillo-Castillo, T., et al. (2015). ReprOlive: a database with linked data for the olive tree (Olea europaea L.) reproductive transcriptome. Front. Plant Sci. 6:625. doi: 10.3389/fpls.2015.00625
Collani, S. (2012). Reproductive barriers in crop plants: understanding the genetic and molecular bases of self-incompatibility in olive and male-sterility in chicory. PhD thesis. University of Padua. Available at: http://paduaresearch.cab.unipd.it/4615/ (accessed 21 Jan 2019).
Collani, S., Galla, G., Ramina, A., Barcaccia, G., Alagna, F., Càceres, E. M., et al. (2012). Self-incompatibility in olive: a new hypothesis on the S-locus genes controlling pollen-pistil interaction. Acta Hortic. 967, 133–140. doi: 10.17660/ActaHortic.2012.967.15
Crawford, D. J., Anderson, G. J., Silva, L. B., de Sequeira, M. M., Moura, M., Santos-Guerra, A., et al. (2015). Breeding systems in Tolpis (Asteraceae) in the Macaronesian islands: the Azores, Madeira and the Canaries. Plant Syst. Evol. 301, 1981–1993. doi: 10.1007/s00606-015-1210-5
Cruden, R. W. (2000). Pollen grains: why so many? Plant Syst. Evol. 222, 143–165. doi: 10.1007/BF00984100
Cruz, F., Julca, I., Gómez-Garrido, J., Loska, D., Marcet-Houben, M., Cano, E., et al. (2016). Genome sequence of the olive tree, Olea europaea. Gigascience 5:29. doi: 10.1186/s13742-016-0134-5
Cuevas, J. (2005). “Incompatibilidad polen-pistilo” in Las variedades de olivo cultivadas en España (libro II: variabilidad y selección). ed. L. Rallo (Sevilla: Junta de Andalucía, Mundi-Prensa y COI).
Cuevas, J., Diaz Hermoso, A., Galian, D., Hueso, J., Pinillos, V., Prieto, M., et al. (2001). Response to cross pollination and choice of pollinisers for the olive cultivars (Olea europaea L.) “Manzanilla de Sevilla”, “Hojiblanca” and “Picual”. Olivae 85, 26–32.
Cuevas, J., and Polito, V. S. (2004). The role of staminate flowers in the breeding system of Olea europaea (Oleaceae): an andromonoecious, wind-pollinated taxon. Ann. Bot. 93, 547–553. doi: 10.1093/aob/mch079
Cuevas, J., Rallo, L., and Rapoport, H. (1994). Initial fruit set at high temperature in olive, Olea europaea L. J. Hort. Sci. 69, 665–672. doi: 10.1080/14620316.1994.11516498
de la Rosa, R., Angiolillo, A., Guerrero, C., Pellegrini, M., Rallo, L., Besnard, G., et al. (2003). A first linkage map of olive (Olea europaea L.) cultivars using RAPD, AFLP, RFLP and SSR markers. Theor. Appl. Genet. 106, 1273–1282. doi: 10.1007/s00122-002-1189-5
de la Rosa, R., James, C. M., and Tobutt, K. R. (2004). Using microsatellites for paternity testing in olive progenies. HortScience 39, 351–354. doi: 10.21273/HORTSCI.39.2.351
Díaz, A., Martín, A., Rallo, P., Barranco, D., and de la Rosa, R. (2006). Self-incompatibility of Arbequina and Picual olive assessed by SSR markers. J. Am. Soc. Hort. Sci. 131, 250–255. doi: 10.21273/JASHS.131.2.250
Do Canto, J., Studer, B., and Lubberstedt, T. (2016). Overcoming self-incompatibility in grasses: a pathway to hybrid breeding. Theor. Appl. Genet. 129, 1815–1829. doi: 10.1007/s00122-016-2775-2
Elgersma, A., Stephenson, A. G., and den Nijs, A. P. M. (1989). Effects of genotype and temperature on pollen tube growth in perennial ryegrass (Lolium perenne L.). Sex. Plant Reprod. 2, 225–230. doi: 10.1007/BF00195583
Farinelli, D., Breton, C., Koubouris, G., Famiani, F., Villemur, P., and Bervillé, A. (2018). Reply to Saumitou-Laprade et al. (2017) “controlling for genetic identity of varieties, pollen contamination and stigma receptivity is essential to characterize the self-incompatibility system of Olea europaea L”. Evol. Appl. 11, 1465–1470. doi: 10.1111/eva.12633.
Farinelli, D., Tombesi, A., and Hassani, D. (2008). Self-sterility and cross-pollination responses of nine olive cultivars in Central Italy. Acta Hortic. 791, 127–136. doi: 10.17660/ActaHortic.2008.791.16
Fernandez-Escobar, R., Ortiz-Urquiza, A., Prado, M., and Rapoport, H. F. (2008). Nitrogen status influence on olive tree flower quality and ovule longevity. Environ. Exp. Bot. 64, 113–119. doi: 10.1016/j.envexpbot.2008.04.007
Ferrer, M. M., and Good, S. V. (2012). Self-sterility in flowering plants: preventing self-fertilization increases family diversification rates. Ann. Bot. 110, 535–553. doi: 10.1093/aob/mcs124
Foster, C. A., and Wright, C. E. (1970). Variation in the expression of self-fertility in Lolium perenne L. Euphytica 19, 61–70. doi: 10.1007/BF01904667
García-Mozo, H., Comtois, P., and Kuehne, E. (2004). Aerobiological clines: the role of topography as a barrier for establishing dispersal corridors. Aerobiologia 20, 161–172. doi: 10.1023/B:AERO.0000032953.03309.c5
Good-Avila, S. V., Mena-Alí, J. I., and Stephenson, A. G. (2008). “Genetic and environmental causes and evolutionary consequences of variations in self-fertility in self incompatible species” in Self-incompatibility in flowering plants: evolution, diversity, and mechanisms. ed. V. E. Franklin-Tong (Berlin, Heidelberg: Springer), 33–51.
Good-Avila, S. V., and Stephenson, A. G. (2002). The inheritance of modifiers conferring self-fertility in the partially self-incompatible perennial campanula, Campanula rapunculoides L. (CAMPANULACEAE). Evolution 56, 263–272. doi: 10.1111/j.0014-3820.2002.tb01336.x
Griggs, W., Hartmann, H., Bradley, M., Iwakiri, B., and Whisler, J. (1975). Olive pollination in California. Calif. Agric. Exp. Station. Bull. 869, 1–50.
Haberman, A., Bakhshian, O., Cerezo-Medina, S., Paltiel, J., Adler, C., Ben-Ari, G., et al. (2017). A possible role for flowering locus T-encoding genes in interpreting environmental and internal cues affecting olive (Olea europaea L.) flower induction. Plant Cell Environ. 40, 1263–1280. doi: 10.1111/pce.12922
Higashiyama, T. (2010). Peptide signaling in pollen-pistil interactions. Plant Cell Physiol. 51, 177–189. doi: 10.1093/pcp/pcq008
Horisaki, A., and Niikura, S. (2008). Developmental and environmental factors affecting level of self-incompatibility response in Brassica rapa L. Sex. Plant Reprod. 21, 123–132. doi: 10.1007/s00497-008-0071-2
Kasasbeh, M. F. A., Ateyyeh, A. F., and Qrunfleh, M. M. (2005). A study on self- and cross-pollination of three olive cultivars in Jordan. Agric. Sci. 32, 222–228.
Kassa, A., Konrad, H., and Geburek, T. (2019). Molecular diversity and gene flow within and among different subspecies of the wild olive (Olea europaea L.): a review. Flora 250, 18–26. doi: 10.1016/j.flora.2018.11.014
Koubouris, G. C., Breton, C. M., Metzidakis, I. T., and Vasilakakis, M. D. (2014). Self-incompatibility and pollination relationships for four Greek olive cultivars. Sci. Hortic. 176, 91–96. doi: 10.1016/j.scienta.2014.06.043
Koubouris, G. C., Metzidakis, I. T., and Vasilakakis, M. D. (2009). Impact of temperature on olive (Olea europaea L.) pollen performance in relation to relative humidity and genotype. Environ. Exp. Bot. 67, 209–214. doi: 10.1016/j.envexpbot.2009.06.002
Lao, X., Suwabe, K., Niikura, S., Kakita, M., Iwano, M., and Takayama, S. (2014). Physiological and genetic analysis of CO2-induced breakdown of self-incompatibility in Brassica rapa. J. Exp. Bot. 65, 939–951. doi: 10.1093/jxb/ert438
Lavee, S., Taryan, J., Levin, J., and Haskal, A. (2002). The significance of cross-pollination for various olive cultivars under irrigated intensive growing conditions. Olivae 91, 25–36.
Levin, D. (1996). The evolutionary significance of pseudo-self-fertility. Am. Nat. 148, 321–332. doi: 10.1086/285927
Liao, J., Dai, J., Kang, H., Liao, K., Ma, W., Wang, J., et al. (2016). Plasticity in the self-incompatibility system of cultivated Nicotiana alata. Euphytica 208, 129–141. doi: 10.1007/s10681-015-1606-x
Liu, P., Sherman-Broyles, S., Nasrallah, M. E. E., and Nasrallah, J. B. (2007). A cryptic modifier causing transient self-incompatibility in Arabidopsis thaliana. Curr. Biol. 17, 734–740. doi: 10.1016/j.cub.2007.03.022
Loumou, A., and Giourga, C. (2003). Olive groves: the life and identity of the Mediterranean. Agric. Hum. Values 20, 87–95. doi: 10.1023/A:1022444005336
Mable, B., Robertson, A., Dart, S., Di Berardo, C., and Witham, L. (2005). Breakdown of self-incompatibility in the perennial Arabidopsis lyrata (Brassicaceae) and its genetic consequences. Evolution 59, 1437–1448. doi: 10.1111/j.0014-3820.2005.tb01794.x
Marchese, A., Marra, F. P., Caruso, T., Mhelembe, K., Costa, F., Fretto, S., et al. (2016a). The first high-density sequence characterized SNP-based linkage map of olive (Olea europaea L. subsp. europaea) developed using genotyping by sequencing. Aust. J. Crop. Sci. 10, 857–863. doi: 10.21475/ajcs.2016.10.06.p7520
Marchese, A., Marra, F. P., Costa, F., Quartararo, A., Fretto, S., and Caruso, T. (2016b). An investigation of the self- and inter-incompatibility of the olive cultivars “Arbequina” and “Koroneiki” in the Mediterranean climate of Sicily. Aust. J. Crop. Sci. 10, 88–93. doi: 10.1016/j.ccc.2010.06.004
McKey, D., Elias, M., Pujol, M. E., and Duputié, A. (2010). The evolutionary ecology of clonally propagated domesticated plants. New Phytol. 186, 318–332. doi: 10.1111/j.1469-8137.2010.03210.x
Mena-Ali, J. I., and Stephenson, A. G. (2007). Segregation analyses of partial self-incompatibility in self and cross progeny of Solanum carolinense reveal a leaky S-allele. Genetics 177, 501–510. doi: 10.1534/genetics.107.073775
Mookerjee, S., Guerin, J., Collins, G., Ford, C., and Sedgley, M. (2005). Paternity analysis using microsatellite markers to identify pollen donors in an olive grove. Theor. Appl. Genet. 111, 1174–1182. doi: 10.1007/s00122-005-0049-5
Mousavi, S., Arzani, K., Torkzaban, B., Hosseini-Mazinani, M., Cultrera, N. G. M., Mariotti, R., et al. (2017). The eastern part of the Fertile Crescent concealed an unexpected route of olive (Olea europaea L.) differentiation. Ann. Bot. 119, 1305–1318. doi: 10.1093/aob/mcx027
Moutier, N. (2002). Self-fertility and inter-compatibilities of sixteen olive varieties. Acta Hortic. 586, 209–212. doi: 10.17660/ActaHortic.2002.586.38
Ockendon, D. (1978). Effect of hexane and humidity on self-incompatibility in Brassica oleracea. Theor. Appl. Genet. 52, 113–117. doi: 10.1007/BF00264743
Okazaki, K., and Hinata, K. (1987). Repressing the expression of self-incompatibility in crucifers by short-term high temperature treatment. Theor. Appl. Genet. 73, 496–500. doi: 10.1007/BF00289185
Orlandi, F., Sgromo, C., Bonofiglio, T., Ruga, L., Romano, B., and Fornaciari, M. (2010). Spring influences on olive flowering and threshold temperatures related to reproductive structure formation. HortScience 45, 1052–1057. doi: 10.21273/hortsci.45.7.1052
Pinillos, V., and Cuevas, J. (2009). Open-pollination provides sufficient levels of cross-pollen in Spanish monovarietal olive orchards. HortScience 44, 499–502. doi: 10.21273/hortsci.44.2.499
Reale, L., Sgromo, C., Ederli, L., Pasqualini, S., Orlandi, F., Fornaciari, M., et al. (2009). Morphological and cytological development and starch accumulation in hermaphrodite and staminate flowers of olive (Olea europaea L.). Sex. Plant Reprod. 22, 109–119. doi: 10.1007/s00497-009-0096-1
Rejón, J. D., Delalande, F., Schaeffer-Reiss, C., Carapito, C., Zienkiewicz, K., De Dios Alché, J., et al. (2013). Proteomics profiling reveals novel proteins and functions of the plant stigma exudate. J. Exp. Bot. 64, 5695–5705. doi: 10.1093/jxb/ert345
Rojo, J., Orlandi, F., Pérez-Badia, R., Aguilera, F., Ben Dhiab, A., Bouziane, H., et al. (2016). Modeling olive pollen intensity in the Mediterranean region through analysis of emission sources. Sci. Total Environ. 551–552, 73–82. doi: 10.1016/j.scitotenv.2016.01.193
Sánchez-Estrada, A., and Cuevas, J. (2018). ‘Arbequina’ olive is self-incompatible. Sci. Hortic. 230, 50–55. doi: 10.1016/j.scienta.2017.11.018
Saumitou-Laprade, P., Vernet, P., Vassiliadis, C., Hoareau, Y., De Magny, G., Dommée, B., et al. (2010). A self-incompatibility system explains high male frequencies in an Androdioecious plant. Science 327, 1648–1650. doi: 10.1126/science.1186687
Saumitou-Laprade, P., Vernet, P., Vekemans, X., Billiard, S., Gallina, S., Essalouh, L., et al. (2017a). Elucidation of the genetic architecture of self-incompatibility in olive: evolutionary consequences and perspectives for orchard management. Evol. Appl. 10, 867–880. doi: 10.1111/eva.12457
Saumitou-Laprade, P., Vernet, P., Vekemans, X., Castric, V., Barcaccia, G., Khadari, B., et al. (2017b). Controlling for genetic identity of varieties, pollen contamination and stigma receptivity is essential to characterize the self-incompatibility system of Olea europaea L. Evol. Appl. 10, 860–866. doi: 10.1111/eva.12498
Seifi, E., Guerin, J., Kaiser, B., and Sedgley, M. (2011). Sexual compatibility and floral biology of some olive cultivars. New Zeal. J. Crop Hortic. Sci. 39, 141–151. doi: 10.1080/01140671.2011.560165
Seifi, E., Guerin, J., Kaiser, B., and Sedgley, M. (2012). Sexual compatibility of the olive cultivar ‘Kalamata’ assessed by paternity analysis. Sp. J. Agric. Res. 10, 731–740. doi: 10.5424/sjar/2012103-501-11
Seifi, E., Guerin, J., Kaiser, B., and Sedgley, M. (2015). Flowering and fruit set in olive: a review. J. Plant Physiol. 5, 1263–1272. doi: 10.22034/IJPP.2015.539653
Selak, G., Cuevas, J., Goreta Ban, S., and Perica, S. (2014a). Pollen tube performance in assessment of compatibility in olive (Olea europaea L.) cultivars. Sci. Hortic. 165, 36–43. doi: 10.1016/j.scienta.2013.10.041
Selak, G. V., Cuevas, J., Goreta Ban, S., Pinillos, V., Dumicic, G., and Perica, S. (2014b). The effect of temperature on the duration of the effective pollination period in “Oblica” olive (Olea europaea) cultivar. Ann. Appl. Biol. 164, 85–94. doi: 10.1111/aab.12082
Selak, G., Perica, S., Ban, S. G., Radunic, M., and Poljak, M. (2011). Reproductive success after self-pollination and cross-pollination of olive cultivars in Croatia. HortScience 46, 186–191. doi: 10.21273/hortsci.46.2.186
Selak, G. V., Perica, S., Goreta Ban, S., and Poljak, M. (2013). The effect of temperature and genotype on pollen performance in olive (Olea europaea L.). Sci. Hortic. 156, 38–46. doi: 10.1016/j.scienta.2013.03.029
Serrano, I., and Olmedilla, A. (2012). Histochemical location of key enzyme activities involved in receptivity and self-incompatibility in the olive tree (Olea europaea L.). Plant Sci. 197, 40–49. doi: 10.1016/j.plantsci.2012.07.007
Serrano, I., Romero-Puertas, M. C., Rodríguez Serrano, M., Sandalio, L. M., and Olmedilla, A. (2012). Role of peroxynitrite in programmed cell death induced in self-incompatible pollen. Plant Signal. Behav. 7, 779–781. doi: 10.4161/psb.20570
Serrano, I., Salvatore, P., and Adela, O. (2010). Programmed-cell-death hallmarks in incompatible pollen and papillar stigma cells of Olea europaea L. under free pollination. Plant Cell Rep. 29, 561–572. doi: 10.1007/s00299-010-0845-5
Serrano, I., Suárez, C., Olmedilla, A., Rapoport, H. F., and Rodríguez-García, M. I. (2008). Structural organization and cytochemical features of the pistil in olive (Olea europaea L.) cv. Picual at anthesis. Sex. Plant Reprod. 21, 99–111. doi: 10.1007/s00497-008-0075-y
Solfanelli, C., Bartolini, S., Vitagliano, C., and Lorenzi, R. (2006). Immunolocalization and quantification of IAA after self-and free-pollination in Olea europaea L. Sci. Hortic. 110, 345–351. doi: 10.1016/j.scienta.2006.06.026
Spinardi, A., and Bassi, D. (2012). Olive fertility as affected by cross-pollination and boron. Sci. World J. 2012, 1–8. doi: 10.1100/2012/375631
Stephenson, A. G., Good, S. V., and Vogler, D. W. (2000). Interrelationships among inbreeding depression, plasticity in the self-incompatibility system, and the breeding system of Campanula rapunculoides L. (Campanulaceae). Ann. Bot. 85, 211–219. doi: 10.1006/anbo.1999.1033
Suárez, C., Castro, A. J., Rapoport, H. F., and Rodríguez-García, M. I. (2012). Morphological, histological and ultrastructural changes in the olive pistil during flowering. Sex. Plant Reprod. 25, 133–146. doi: 10.1007/s00497-012-0186-3
Taslimpour, M. R., and Aslmoshtaghi, E. (2013). Study of self-incompatibility in some Iranian olive cultivars. Crop Breed. J. 3, 123–127. doi: 10.22092/CBJ.2012.100458
Unver, T., Wu, Z., Sterck, L., Turktas, M., Lohaus, R., Li, Z., et al. (2017). Genome of wild olive and the evolution of oil biosynthesis. Proc. Natl. Acad. Sci. USA 114, E9413–E9422. doi: 10.1073/pnas.1708621114
Vernet, P., Lepercq, P., Billiard, S., Bourceaux, A., Lepart, J., Dommée, B., et al. (2016). Evidence for the long-term maintenance of a rare self-incompatibility system in Oleaceae. New Phytol. 210, 1408–1417. doi: 10.1111/nph.13872
Wilkins, P. W., and Thorogood, D. (1992). Breakdown of self-incompatibility in perennial ryegrass at high temperature and its uses in breeding. Euphytica 64, 65–69. doi: 10.1007/BF00023539
Wu, S.-B., Collins, G., and Sedgley, M. (2002). Sexual compatibility within and between olive cultivars. J. Hortic. Sci. Biotechnol. 77, 665–673. doi: 10.1080/14620316.2002.11511554
Yang, Y., Liu, Z., Zhang, T., Zhou, G., Duan, Z., Li, B., et al. (2018). Mechanism of salt-induced self-compatibility dissected by comparative proteomic analysis in Brassica napus L. Int. J. Mol. Sci. 19:1652. doi: 10.3390/ijms19061652
Keywords: Olea europaea L., pseudo-self-compatibility, pollen-pistil interaction, sporophytic system, self-incompatibility
Citation: Alagna F, Caceres ME, Pandolfi S, Collani S, Mousavi S, Mariotti R, Cultrera NGM, Baldoni L and Barcaccia G (2019) The Paradox of Self-Fertile Varieties in the Context of Self-Incompatible Genotypes in Olive. Front. Plant Sci. 10:725. doi: 10.3389/fpls.2019.00725
Edited by:
Giuseppe Ferrara, University of Bari Aldo Moro, ItalyCopyright © 2019 Alagna, Caceres, Pandolfi, Collani, Mousavi, Mariotti, Cultrera, Baldoni and Barcaccia. This is an open-access article distributed under the terms of the Creative Commons Attribution License (CC BY). The use, distribution or reproduction in other forums is permitted, provided the original author(s) and the copyright owner(s) are credited and that the original publication in this journal is cited, in accordance with accepted academic practice. No use, distribution or reproduction is permitted which does not comply with these terms.
*Correspondence: L. Baldoni, bHVjaWFuYS5iYWxkb25pQGliYnIuY25yLml0
†These authors have contributed equally to this work