- Federal Research Centre for Cultivated Plants, Institute for Breeding Research on Fruit Crops, Julius Kühn-Institut (JKI), Dresden, Germany
The bacterium, Erwinia amylovora, deposits effector proteins such as AvrRpt2EA into hosts through the type III secretion pathogenicity island to cause fire blight in susceptible Malus genotypes. A single nucleotide polymorphism in the AvrRpt2EA effector plays a key role in pathogen virulence on Malus hosts by exchanging one cysteine to serine in the effector protein sequence. Fire blight resistance quantitative trait loci (QTLs) were detected in a few apple cultivars and wild Malus genotypes with the resistance of wild apples generally found to be stronger than their domestic relatives. The only candidate and functionally analyzed fire blight resistance genes proposed are from wild apple genotypes. Nevertheless, the aforementioned AvrRpt2EA SNP and a couple of effector mutants of E. amylovora are responsible for the breakdown of resistance from a few Malus donors including detected QTLs and underlying R-genes. This review summarizes a key finding related to the molecular basis underpinning an aspect of virulence of E. amylovora on Malus genotypes, as well as mechanisms of host recognition and specificity, and their implications on the results of genetic mapping and phenotypic studies within the last 5–6 years. Although the knowledge gained has improved our understanding of the Malus–E. amylovora system, more research is required to fully grasp the resistance mechanisms in this genus especially as they pertain to direct interactions with pathogen effectors.
Introduction
Economic Importance of Fire Blight and Host Immunity
Erwinia amylovora (Burrill) (Winslow et al., 1920) incites fire blight disease in the genus Malus as it does other genera belonging to the Rosaceae family. The bacterium invades hosts primarily through flowers or wounds on vegetative tissues and migrates internally to other infection sites causing blossom, shoot, and rootstock blights (Peil et al., 2009). This erratic disease is the most feared bacterial disease especially for apples and pears, the two most economically important members of Rosaceae. Commercial cultivars of the domesticated apple (Malus domestica Borkh.) are highly susceptible, although some show low susceptibility (Sobiczewski et al., 2011). Resistance sources are found mostly in wild species having unmarketable fruit quality (Kellerhals et al., 2017). The economic damages following fire blight outbreaks are estimated in millions of Dollars wherever they have been reported (Hasler et al., 2002; Norelli et al., 2003). These huge costs stem from the destruction of trees due to the ability of the pathogen to completely destroy entire orchards of pome fruits in a single growing season, as well as antibiotic application as a control measure especially in the United States. In Europe, economic damages from fire blight comprise mostly of the production costs, since antibiotic application as a control measure is either strictly regulated or completely forbidden.
Therefore, fire blight resistance breeding strategy that focuses on the basic molecular mechanisms of host resistance is increasingly important due to the inadequacies of other control strategies.
Erwinia amylovora (Ea) can adopt a biotrophic or necrotrophic lifestyle, since it invades living Malus hosts yet could overwinter in dead apple leaves (Sobiczewski et al., 2014, 2017). Like other plant-pathogen models (See: Dodd and Rathjen, 2010), Ea manipulates host defense mechanisms to cause disease, while Malus host immunity/resistance depends on their capability to recognize Ea elicitors (e.g., effector proteins) through different molecular strategies. In general, surface receptor proteins known as pattern recognition receptors (PRRs) recognize conserved microbial patterns (pathogen-associated molecular patterns; PAMP), e.g., bacterial flagellin, lipopolysaccharide, in PTI—pattern triggered immunity (Couto and Zipfel, 2016). On the other hand, intracellular receptors could recognize pathogen effector proteins during invasion of host cells in effector triggered immunity (ETI; Toruño et al., 2016). In principle, ETI and PTI elicit similar responses in hosts; however, only the former involves hypersensitive response (HR)—a form of localized cell death. HR is controlled by hypersensitive response and pathogenicity genes (hrp) (Khan et al., 2012) of the so-called pathogenicity island (PAI) (Oh and Beer, 2005).
Knowledge of Malus–Ea interaction is crucial for breeding durable resistant apples, and past and current works have been done with regard to characterizing pathogenicity genes of Ea (reviewed in Oh and Beer, 2005; Khan et al., 2012; Malnoy et al., 2012), as well as breeding for resistance (Peil et al., 2009). This review focuses on works that have contributed to our understanding as it pertains to Malus–Ea interaction and host resistance mechanisms since 2013 till date.
Malus Host Resistance: Strains vs. Resistance
Vogt et al. (2013) and other studies (Table 1) provided valuable insights into E. amylovora pathogenicity and Malus resistance. The avrRpt2EA effector gene of several Ea strains was sequenced and detected a single nucleotide polymorphism (SNP), which translated into a single substitution of amino acid cysteine (C-allele) with serine (S-allele) at position 156 of the protein sequence. Meanwhile, resistance to fire blight was thought to be quantitatively controlled in Malus, and prior to 2013, two fire blight resistance quantitative trait loci (QTLs) with minor effects were described in apple cultivars—“Fiesta” (Calenge et al., 2005) and “Florina” (Le Roux et al., 2010) and three QTLs with major effects in wild apple genotypes Malus ×robusta 5 (Mr5) (Peil et al., 2007; Gardiner et al., 2012), M. floribunda 821 (Mf821), and the ornamental cultivar “Evereste” (Durel et al., 2009). However, in Mr5, a single resistance gene was shown to underlie the QTL region. For these studies, Ea strain CFBP1430 from the French collection was used for artificial shoot inoculations; the exception is in Malus ×robusta 5 (Mr5), where Ea222 was used. Vogt et al. (2013) showed that both Ea222 and CFBP1430 encode for the C-allele of the avrRpt2EA effector of E. amylovora. Interestingly, strains bearing the C-allele are avirulent to Mr5, whereas strains bearing the S-allele are not, thus supporting the strain specificity of Mr5 fire blight resistance (Peil et al., 2011).
Vogt and colleagues proved that strains encoding for the S-allele were more aggressive for different apple cultivars, and Mr5 itself - a major source of fire blight resistance, but not on other wild apple accessions of Malus fusca and Malus baccata. Consequently, the suspicion that the fire blight resistance QTL of Mr5 on linkage group 3 (LG3) is broken down by the highly aggressive S-allele encoding Canadian strain Ea3049 (Peil et al., 2011), was confirmed by Wöhner et al. (2014). In addition, minor QTLs contributing to fire blight resistance in Mr5 were detected (Peil et al., 2011; Wöhner et al., 2014), with one in particular on LG7 found to improve resistance of LG3 QTL (Wöhner et al., 2014). Erwinia amylovora strain specificity in Malus begs the question “Can reduced susceptibility of apple cultivars be considered as resistance?” It could be argued that the fire blight QTLs of apple cultivars like “Fiesta” and “Florina” actually control reduced susceptibility to the disease rather than resistance, given that fire blight QTLs in those apple cultivars have been shown to only explain minor effects to Ea strains considered as less aggressive.
A few novel fire blight minor QTLs were identified in a “Royal Gala” × M. sieversii population (Desnoues et al., 2018). However, two QTLs appear to be the same as QTLs of “Florina” (Le Roux et al., 2010) and “Co-op16” × “Co-op17” (Khan et al., 2013) on chromosomes 10 and 15, respectively, which were identified with strains Ea273 and CFBP140, which encode the C-allele of AvrRpt2EA effector protein (Vogt et al., 2013). While strong fire blight resistance of some accessions of M. sieversii was reported (Harshman et al., 2017), it is unsurprising that the resistance QTLs/levels of M. sieversii accession PI 613981 (Desnoues et al., 2018) are similar to that of apple cultivars “Florina” and “Nova Easygro” (Le Roux et al., 2010), since M. sieversii is the primary progenitor of M. domestica. Similarly, the alleles of QTLs in coupling with resistance inherited by the apple cultivar “Enterprise” and a breeding clone X-6398 (van de Weg et al., 2018) were found to be identical to “Fiesta” (Calenge et al., 2005), with both studies finding epistatic effects, suggesting similar mechanism of reduced fire blight susceptibility. Nonetheless, reduced susceptibility mechanism (small effects) of cultivars to E. amylovora is a defense mechanism capable of contributing to the overall goal of durable resistance by slowing down the effect of mutations of the pathogen that may overcome resistance. However, little is known about the reactions of QTL donor cultivars to strains with stronger pathogenicity (Table 1). Moreover, testing populations used to detect QTLs in cultivars (not just donor parents) with AvrRpt2EA-S-allele-encoding strains will confirm the strength of such QTLs and importantly determine if transgressive individuals (i.e., progenies more resistant or significantly less susceptible than the QTL donor parent) could be obtained. Transgressive progenies would be more worthwhile to use in breeding programs.
In the Malus–Ea system, non-strain-specific (i.e. broad spectrum) resistance appears to be the exception rather than the rule. The fire blight resistance of the M. fusca genotype MAL0045 (hereafter referred to as M. fusca, Emeriewen et al., 2014a) appears to be broad spectrum. This genotype is the source of Mfu10—a resistance QTL mapped on LG10 that was detected with strain Ea222 (Emeriewen et al., 2014b). C- and S-allele strains of the pathogen tested on M. fusca were unable to overcome neither its resistance nor Mfu10 (Vogt et al., 2013; Emeriewen et al., 2015). Although the S-allele strain Ea3049 could not overcome Mfu10, the population was adversely affected (Emeriewen et al., 2015), suggesting the possibility of a second putative genetic factor contributing to the resistance of M. fusca. A situation where M. fusca is highly resistant to such an aggressive S-type strain but almost half of its progenies were found to be highly susceptible indicates that such putative factor could be homozygous recessive in this crabapple accession. However, a “less” resistant reaction was elicited by M. fusca, following fire blight disease assessment with two other S-allele strains LA635 and LA637 (Wöhner et al., 2017). Both strains were shown to not only possess the single nucleotide polymorphism in the type III effector AvrRpt2EA at position 156 but also an rpsL chromosomal mutation responsible for a high degree of streptomycin resistance (Smits et al., 2014). It is unclear whether the combination of the unique mechanisms arising from genomic mutations controlling drug resistance and pathogenicity contributes to the aggressive nature of LA635 and LA637 strains. Clearly, the SNP in AvrRpt2EA is not the only contributory virulence factor in S-allele strains.
Another source of strong fire blight resistance is the wild apple genotype Malus ×arnoldiana MAL0004 initially mentioned as M. baccata (Vogt et al., 2013; Peil et al., 2014). This wild apple is the source of a strong and stable fire blight resistance QTL (FB_Mar12) mapped on LG12 (Emeriewen et al., 2017a). FB_Mar12 detected simultaneously with both the C-allele (Ea222) and S-allele (Ea3049) strains of E. amylovora was mapped at a similar position on chromosome 12 like the QTLs of Mf821 and “Evereste” (Durel et al., 2009), suggesting that all three QTLs could be allelic (Figure 1). Although no additional evidence of their allelic nature exists, however, Wöhner et al. (2017) provided strong evidence that the resistance mechanisms of all three wild apples are in fact different. While all three donors of each QTL, i.e., MAL0004, Mf821, “Evereste,” elicit strong resistance reaction to a C-allele strain Ea1189, such resistance reaction in “Evereste” and Mf821 appears to be overcome by Ea3049, LA635, and LA637, bearing the S-allele but not in the M. ×arnoldiana accession (Wöhner et al., 2017). Thus, FB_Mar12 and Mfu10 are the most stable and durable fire blight resistance QTLs in Malus so far.
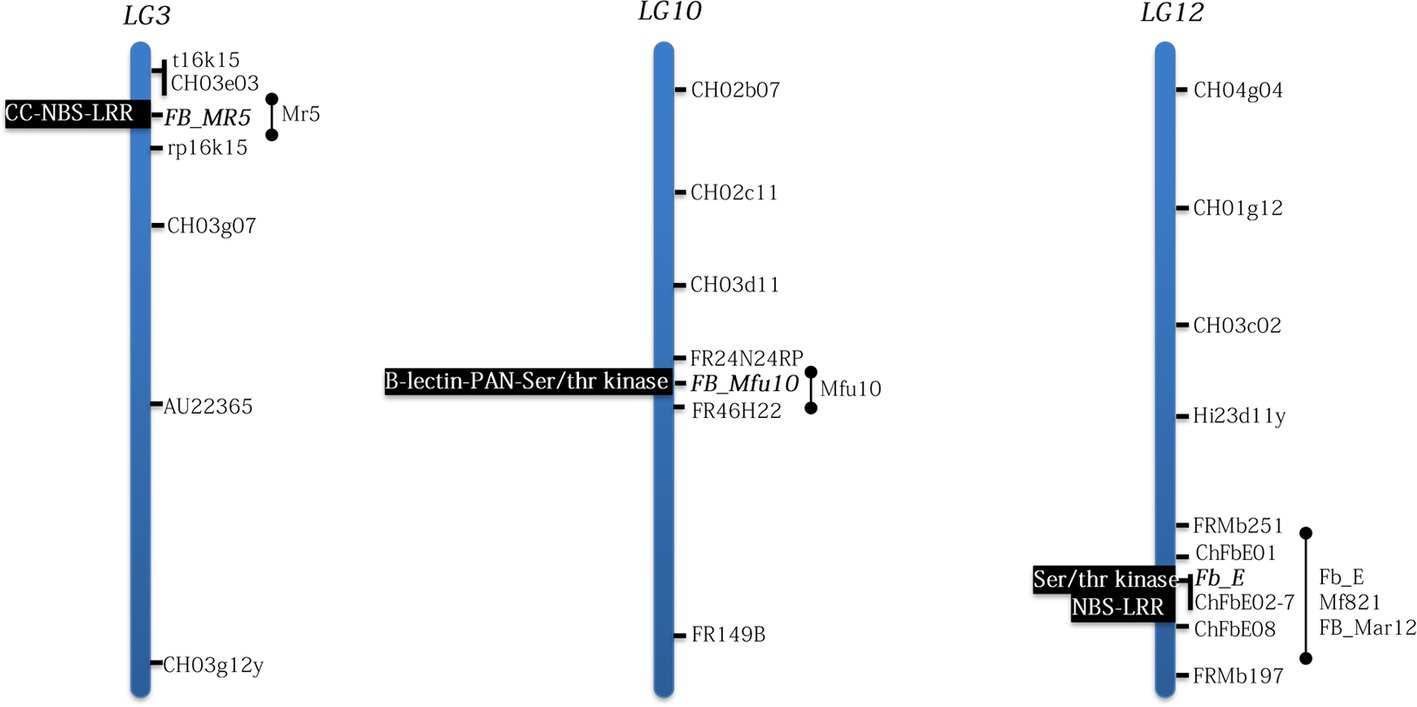
Figure 1. Positions of fire blight (candidate) genes and their domains identified in Malus following positional cloning studies. Bar represents positions of mapped QTLs on the linkage groups. Although no candidate genes have being proposed for M. ×arnoldiana and M. floribunda 821, their respective QTLs, FB_Mar12 and Mf12 map in the region where Fb_E is located on LG12. LG=linkage group.
Malus–Erwinia Amylovora Interactions: The Role of Effector Mutants
Since the Malus–Ea pathosystem is characterized by the ability of the host to activate defense responses upon recognition of effectors secreted by Ea, the use of effector protein knock-out strains is an important tool for deciphering Malus–Ea interactions. The pathogen possesses the hrp-T3SS (hrp type III secretion) system, releasing type III effectors essential for pathogenicity, e.g., DspE, AvrRpt2EA, Eop1, Eop3, and the exopolysaccharide amylovoran (ams), into the host cell. However, T3SS chaperones play critical roles in translocating effector proteins (Castiblanco et al., 2018); other genes (overviewed in McNally et al., 2015) could determine virulence in Ea. Gene-for-gene interactions were shown in Mr5 (Vogt et al., 2013) and partially in Mf821 and “Evereste” (Wöhner et al., 2017). An avrRpt2EA mutant strain ZYRKD3-1 broke down the resistance of Mr5, thus indicating the first gene-for-gene interaction in Malus and the avirulence role of avrRpt2EA in Mr5–Ea pathosystem (Vogt et al., 2013). AvrRpt2EA was further shown to play no such roles in the examined M. fusca, M. ×arnoldiana and Mf821 systems (Vogt et al., 2013; Wöhner et al., 2017; Emeriewen et al., 2017b). Further, both the C- and S-alleles of AvrRpt2EA effector expressed in Nicotiana benthamiana induced cell death as did the Pseudomonas syringae effector AvrRpt2 (Vogt et al., 2013), thus suggesting an interaction between Mr5 and Ea that mimics the RPS2–Arabidopsis thaliana model (Axtell and Staskawicz, 2003). In addition to being an avirulence factor in Mr5, the role of avrRpt2EA as a virulence factor in susceptible hosts was demonstrated by Schröpfer et al. (2018): transgenic apple lines expressing avrRpt2EA developed typical fire blight symptoms. Also, these apple lines resulted in increased expressions of PR-1 gene, thus inducing salicylic acid–dependent defense response (Schröpfer et al., 2018). Deletion of eop1 effector of E. amylovora induced fire blight in Mf821 and “Evereste” but not in M. ×arnoldiana (Wöhner et al., 2017), thus confirming that the resistance mechanism of M. ×arnoldiana is different although their QTLs are closely located on LG12 (Figure 1). Furthermore, that eop1 breaks down the resistance of Mf821 and “Evereste” is strong evidence of another gene-for-gene interaction in Malus (Wöhner et al., 2017). Comparative mapping studies with Eop1 mutant and wild type strains on Mf821 and “Evereste” will confirm the gene-for-gene interactions or otherwise.
Malus Fire Blight Resistance (Candidate) Genes
Identifying fire blight genes and the subsequent functional analyses are crucial in understanding resistance mechanisms in Malus. Only map-based cloning approach has resulted in credible fire blight candidate genes till date (Figure 1). The first set of fire blight resistance candidate genes in Malus (Parravicini et al., 2011) was hypothesized to function in a similar mode as the well-characterized Pto-mediated resistance to bacterial speck in tomato (Pedley and Martin, 2003). Although, the candidate genes from the fire blight QTL region of the ornamental apple cultivar, “Evereste,” showed homology to the Pto/Prf system (Parravicini et al., 2011), it is yet to be determined if these genes actually confer resistance to fire blight in complementing studies.
The guard hypothesis was postulated for FB_MR5 identified in the Mr5 fire blight QTL region (Fahrentrapp et al., 2013). Although multiple genes were suggested in this region to contribute to resistance (Gardiner et al., 2012), FB_MR5 was proposed as the only candidate and is a single R-gene belonging to the class of plant disease R-genes families encoding for nucleotide-binding site (NBS), a C-terminal leucine rich repeat (LRR), and a coiled coil domain (CC). Like RPS2 being triggered on recognizing the disturbance of Arabidopsis thaliana RIN4 protein by AvrRpt2 type III effector avirulence protein from Pseudomonas syringae (Mackey et al., 2003), AvrRpt2EA, an analog in E. amylovora (Zhao et al., 2006), was hypothesized to target the homolog of RIN4 in Mr5 (Fahrentrapp et al., 2013). However, protein-protein interaction analyses via yeast two-hybrid assays failed to detect any interaction between RIN4 from Mr5 and either of the fire blight resistance protein FB_MR5 from Mr5 or the effector protein AvrRpt2EA from E. amylovora (Vogt, 2018). A recent study analyzing the protein structure of AvrRpt2EA, however, suggests a direct interaction with FB_MR5 (Bartho et al., 2019). Importantly, FB_MR5 confers resistance to transgenic lines of an otherwise susceptible cultivar “Gala,” expressing this gene that was inoculated with Ea222 (Broggini et al., 2014). Transgenic lines expressing FB_MR5 inoculated with the mutant strain with disruption of the corresponding avirulence gene (avrRpt2EA) also demonstrated the gene-or-gene relationship found in Mr5 (Broggini et al., 2014).
In M. fusca, a candidate gene (FB_Mfu10) encoding Bulb-lectin, PAN/apple and serine/threonine kinase domains, was shown to underlie Mfu10 (Emeriewen et al., 2018). The resistance model in M. fusca is so far not obvious. If indeed FB_Mfu10 confers broad spectrum resistance, the mechanism could be similar to the proposed zigzag model of PRRs (pathogen recognition receptors) as first line of active defense (Jones and Dangl, 2006). As reviewed elsewhere (Zipfel, 2014), PRRs are either receptor kinases (RKs) or receptor-like proteins (RLPs). Is FB_Mfu10 a potential PRR? What is the corresponding pathogen recognition molecular pattern—ams or effectors? Effector proteins may act as PAMPs (Thomma et al., 2011), and some may target PTI rather than ETI (Jones and Dangl, 2006). Nevertheless, the lack of the AvrRpt2EA effector increasing average disease in M. fusca progeny (Emeriewen et al., 2017b) at least indicates AvrRpt2EA effector triggered response.
Further, the roles of lectin and PAN/apple domains in mediating protein-carbohydrate and protein–protein interactions and defense signaling have been shown (Lannoo and Van Damme, 2014; Kim et al., 2015). To date, no Ea effector mutant able to break the resistance of M. fusca is reported to suggest a gene-for-gene interaction. Functional tests of FB_Mfu10 in transgenic lines will therefore improve our understanding of the fire blight resistance of this crabapple.
Concluding Remarks
Malus–Ea interaction may be governed by a distinct complementary interaction between pathogen avirulence (Avr) effectors and alleles of the corresponding host resistance (R) locus or gene, but other factors and models cannot be excluded. The identification of a SNP in the AvrRpt2EA effector responsible for strain pathogenicity in Mr5, but also virulence in other Malus hosts (Vogt et al., 2013), was a major breakthrough in understanding this system. Molecular breeding for durable and broad spectrum resistance to fire blight should take into cognizance the virulence factors of the pathogen and the reaction of Malus hosts. The use of S-allele strains of E. amylovora should be the benchmark in identifying strong and durable resistance gene sources. It is also imperative to investigate resistance donors from apple cultivars using S-allele strains. However, further studies on host-pathogen interaction are still required to achieve durable resistance. Knockout of genes of the pathogenicity island of different E. amylovora strains and other Ea mutations, which have led to the discovery of more virulence factors (Ramos et al., 2013, 2015; Klee et al., 2018), will improve our understanding of the pathogenic process.
Presently, only one functionally analyzed fire blight resistance gene in Malus from Mr5 (Broggini et al., 2014) is known, although the resistance is overcome. The others are yet to be proven candidate genes from “Evereste” (Parravicini et al., 2011) and M. fusca (Emeriewen et al., 2018). Since the entire resistance gene sources are from wild apples with undesirable fruit qualities, coupled with the ability of the pathogen to overcome resistance over time, the potentials of susceptibility (S) genes have been suggested (Reviewed in van Schie and Takken, 2014). Taking into account, the HrpN-interacting protein of Malus (HIPM) was investigated as a potential fire blight S gene (Campa et al., 2018). These authors showed that HIPM-silenced lines of the apple cultivar Galaxy® showed reduced susceptibility to strain Ea273, a C-allele strain. Similarly, with a C-allele strain, CFBP1430, susceptible response of “Golden Delicious” cultivar, was studied via RNA-seq, leading to the identification of differentially expressed genes (Kamber et al., 2016). It remains unclear what role S-genes will play in the long term especially considering the evolutionary potential of the pathogen. What is clear, however, is the need to functionally analyze other resistance candidate genes, identify more resistance gene sources, and perform more resistance protein and effector protein interaction studies.
Author Contributions
All the authors conceptualized this review and discussed the approach. OFE wrote the manuscript and all authors edited and finally approved the manuscript.
Funding
The Deutsche Forschungsgemeinschaft (DFG) financially supported projects on Malus fusca fire blight resistance (Project number: AOBJ-619970) and AvrRpt2EA studies (Project number: AOBJ-611951).
Conflict of Interest Statement
The authors declare that the research was conducted in the absence of any commercial or financial relationships that could be construed as a potential conflict of interest.
Acknowledgments
We thank past and present collaborators in this area of research and sincerely apologize if some literature were not cited. It is due to the manuscript length limitations.
References
Axtell, M. J., and Staskawicz, B. J. (2003). Initiation of RPS2-specified disease resistance in Arabidopsis is coupled to the AvrRpt2-directed elimination of RIN4. Cell 112, 369–377. doi: 10.1016/S0092-8674(03)00036-9
Bartho, J. D., Demitri, N., Bellini, D., Flachowsky, H., Peil, A., Walsh, M. A., et al. (2019). The structure of Erwinia amylovora AvrRpt2 provides insight into protein maturation and induced resistance to fire blight by Malus × robusta 5. J. Struct. Biol. doi: 10.1016/j.jsb.2019.03.010 (in press).
Broggini, G. A. L., Wöhner, T., Fahrentrapp, J., Kost, T. D., Flachowsky, H., Peil, A., et al. (2014). Engineering fire blight resistance into the apple cultivar ‘Gala’ using the FB_MR5 CC-NBS-LRR resistance gene of Malus × robusta 5. Plant Biotechnol. J. 12, 728–733. doi: 10.1111/pbi.12177
Calenge, F., Drouet, D., Denance, C., Van de Weg, W. E., Brisset, M. N., Paulin, J. P., et al. (2005). Identification of a major QTL together with several minor additive or espistatic QTLs for resistance to fire blight in apple in two related progenies. Theor. Appl. Genet. 111, 128–135. doi: 10.1007/s00122-005-2002-z
Campa, M., Piazza, S., Righetti, L., Oh, C. S., Conterno, L., Borejsza-Wysocka, E., et al. (2018). HIPM is a susceptibility gene of Malus: reduced expression reduces susceptibility to Erwinia amylovora. Mol. Plant-Microbe Interact. 32, 167–175. doi: 10.1094/MPMI-05-18-0120-R
Castiblanco, L. F., Triplett, L. R., and Sundin, G. W. (2018). Regulation of effector delivery by type III secretion chaperone proteins in Erwinia amylovora. Front. Microbiol. 9:146. doi: 10.3389/fmicb.2018.00146
Couto, D., and Zipfel, C. (2016). Regulation of pattern recognition receptor signalling in plants. Nat. Rev. Immunol. 16, 537–552. doi: 10.1038/nri.2016.77
Desnoues, E., Norelli, J. L., Aldwinckle, H. S., Wisniewski, M. E., Evans, K. M., Malnoy, M., et al. (2018). Identification of novel strain-specific and environment-dependent minor QTLs linked to fire blight resistance in apples. Plant Mol. Biol. Report. 36, 247–256. doi: 10.1007/s11105-018-1076-0
Dodd, P. N., and Rathjen, J. P. (2010). Plant immunity: towards an integrated view of plant-pathogen interactions. Nat. Rev. Genet. 11, 539–548. doi: 10.1038/nrg2812
Durel, C. E., Denance, C., and Brisset, M. N. (2009). Two distinct major QTL for resistance to fire blight co-localize on linkage group 12 in apple genotypes ‘Evereste’ and Malus floribunda clone 821. Genome 52, 139–147. doi: 10.1139/G08-111
Emeriewen, O., Malnoy, M., Richter, K., Kilian, A., Hanke, M.-V., and Peil, A. (2014a). Evidence of a major QTL for fire blight resistance in the apple wild species Malus fusca. Acta Hortic. 1056, 289–293. doi: 10.17660/actahortic.2014.1056.49
Emeriewen, O. F., Peil, A., Richter, K., Zini, E., Hanke, M.-V., and Malnoy, M. (2017a). Fire blight resistance of Malus ×arnoldiana is controlled by a quantitative trait locus located at the distal end of linkage group 12. Eur. J. Plant Pathol. 148, 1011–1018. doi: 10.1007/s10658-017-1152-6
Emeriewen, O. F., Richter, K., Hanke, M.-V., Malnoy, M., and Peil, A. (2015). The fire blight resistance QTL of Malus fusca (Mfu10) is affected but not broken down by the highly virulent Canadian Erwinia amylovora strain E2002A. Eur. J. Plant Pathol. 141, 631–635. doi: 10.1007/s10658-014-0565-8
Emeriewen, O. F., Richter, K., Hanke, M.-V., Malnoy, M., and Peil, A. (2017b). Further insights into Malus fusca fire blight resistance. J. Plant Pathol. 99, 45–49. doi: 10.4454/jpp.v99i0.3908
Emeriewen, O., Richter, K., Killian, A., Zini, E., Hanke, M.-V., Malnoy, M., et al. (2014b). Identification of a major quantitative trait locus for resistance to fire blight in the wild apple species Malus fusca. Mol. Breed. 34, 407–419. doi: 10.1007/s11032-014-0043-1
Emeriewen, O. F., Richter, K., Piazza, S., Micheletti, D., Broggini, G. A. L., Berner, T., et al. (2018). Towards map-based cloning of FB_Mfu10: Identification of a receptor-like kinase candidate gene underlying the Malus fusca fire blight resistance locus on linkage group 10. Mol. Breed. 38:106. doi: 10.1007/s11032-018-0863-5
Fahrentrapp, J., Broggini, G. A. L., Kellerhals, M., Peil, A., Richter, K., Zini, E., et al. (2013). A candidate gene for fire blight resistance in Malus × robusta 5 is coding for a CC-NBS-LRR. Tree Genet. Genomes 9, 237–251. doi: 10.1007/s11295-012-0550-3
Gardiner, S. E., Norelli, J. L., de Silva, N., Fazio, G., Peil, A., Malnoy, M., et al. (2012). Putative resistance gene markers associated with quantitative trait loci for fire blight resistance in Malus ‘Robusta 5’ accessions. BMC Genet. 13:25. doi: 10.1186/1471-2156-13-25
Harshman, J. M., Evans, K. M., Allen, H., Potts, R., Flamenco, J., Aldwinckle, H. S., et al. (2017). Fire blight resistance in wild accessions of Malus sieversii. Plant Dis. 101, 1738–1745. doi: 10.1094/PDIS-01-17-0077-RE
Hasler, T., Schaerer, H. J., Holliger, E., Vogelsanger, J., Vignutelli, A., and Schoch, B. (2002). Fire blight situation in Switzerland. Acta Hortic. 590, 73–79. doi: 10.17660/ActaHortic.2002.590.8
Jones, J. D. G., and Dangl, J. L. (2006). The plant immune system. Nature 444, 323–329. doi: 10.1038/nature05286
Kamber, T., Buchmann, J. P., Pothier, J. F., Smiths, T. H. M., Wicker, T., and Duffy, B. (2016). Fire blight disease reactome: RNA-seq transcriptional profile of apple host plant defense responses to Erwinia amylovora pathogen infection. Sci. Rep. 6:21600. doi: 10.1038/srep21600
Kellerhals, M., Schütz, S., and Patocchi, A. (2017). Breeding for host resistance to fire blight. J. Plant Pathol. 99, 37–43. doi: 10.4454/jpp.v99i0.3923
Khan, M. A., Zhao, Y. F., and Korban, S. S. (2012). Molecular mechanisms of pathogenesis and resistance to the bacterial pathogen Erwinia amylovora, causal agent of fire blight disease in Rosaceae. Plant Mol. Biol. Report. 30, 247–260. doi: 10.1007/s11105-011-0334-1
Khan, M. A., Zhao, Y. F., and Korban, S. S. (2013). Identification of genetic loci associated with fire blight resistance in Malus through combined use of QTL and association mapping. Physiol. Plant. 148, 344–353. doi: 10.1111/ppl.12068
Kim, N. H., Lee, D. H., Choi du, S., and Hwang, B. K. (2015). The pepper GNA-related lectin and PAN domain gene, CaGLP1, is required for plant cell death and defense signaling during bacterial infection. Plant Sci. 241, 307–315. doi: 10.1016/j.plantsci.2015.07.003
Klee, S. M., Mostafa, I., Chen, S., Dufresne, C., Lehman, B. L., Sinn, J. P., et al. (2018). An Erwinia amylovora yjeK mutant exhibits reduced virulence, increased chemical sensitivity and numerous environmentally dependent proteomic alterations. Mol. Plant Pathol. 19, 1667–1678. doi: 10.1111/mpp.12650
Lannoo, N., and Van Damme, E. J. M. (2014). Lectin domains at the frontiers of plant defense. Front. Plant Sci. 5:397. doi: 10.3389/fpls.2014.00397
Le Roux, P. M. F., Khan, M. A., Broggini, G. A. L., Duffy, B., Gessler, C., and Patocchi, A. (2010). Mapping of quantitative trait loci for fire blight resistance in the apple cultivars ‘Florina’ and ‘Nova Easygro’. Genome 53, 710–722. doi: 10.1139/G10-047
Mackey, D., Belkhadir, Y., Alonso, J. M., Ecker, J. R., and Dangl, J. L. (2003). Arabidopsis RIN4 is a target of the Type III virulence effector AvrRpt2 and modulates RPS2-mediated resistance. Cell 112, 379–389. doi: 10.1016/S0092-8674(03)00040-0
Malnoy, M., Martens, S., Norelli, J. L., Barny, M., Sundin, G. W., Smiths, T. H. M., et al. (2012). Fire Blight: Applied Genomic Insights of the Pathogen and Host. Annu. Rev. Phytopathol. 50, 475–494. doi: 10.1146/annurev-phyto-081211-172931
McNally, R. R., Zhao, Y., and Sundin, G. W. (2015). “Towards understanding fire blight: virulence mechanisms and their regulation in Erwinia amylovora” in Bacteria-Plant Interactions: Advanced Research and Future Trends. eds. J. Murillo, B. A. Vinatzer, R. W. Jackson, and D. L. Arnold (Norfolk, UK: Caister Academic Press), 61–82. doi: 10.21775/9781908230584
Norelli, J. L., Jones, A. L., and Aldwinkle, H. S. (2003). Fire blight management in the twenty-first century – using new technologies that enhance host resistance in apple. Plant Dis. 87, 756–765. doi: 10.1094/PDIS.2003.87.7.756
Oh, C.-S., and Beer, S. V. (2005). Molecular genetics of Erwinia amylovora involves in the development of fire blight. FEMS Microbio. Lett. 253, 185–192. doi: 10.1016/j.femsle.2005.09.051
Parravicini, G., Gessler, C., Denance, C., Lasserre-Zuber, P., Vergne, E., Brisset, M. N., et al. (2011). Identification of serine/threonine kinase and nucleotide-binding-site-leucine-rich repeat (NBS-LRR) genes in the fire blight resistance quantitative trait locus of apple cultivar ‘Evereste’. Mol. Plant Pathol. 12, 493–505. doi: 10.1111/j.1364-3703.2010.00690.x
Pedley, K. F., and Martin, G. B. (2003). Molecular basis of Pto-mediated resistance to bacterial speck disease in tomato. Annu. Rev. Phytopathol. 41, 215–243. doi: 10.1146/annurev.phyto.41.121602.143032
Peil, A., Bus, V. G. M., Geider, K., Richter, K., Flachowsky, H., and Hanke, M. V. (2009). Improvement of fire blight resistance in apple and pear. Int. J. Plant Breed 3, 1–27.
Peil, A., Flachowsky, H., Hanke, M.-V., Richter, K., and Rode, J. (2011). Inoculation of Malus × robusta 5 progeny with a strain breaking resistance to fire blight reveals a minor QTL on LG5. Acta Hortic. 986, 357–362. doi: 10.17660/ActaHortic.2011.896.49
Peil, A., Garcia-Libreros, T., Richter, K., Trognitz, F. C., Trognitz, B., Hanke, M. V., et al. (2007). Strong evidence for a fire blight resistance gene of Malus robusta located on linkage group 3. Plant Breed. 126, 270–475. doi: 10.1111/j.1439-0523.2007.01408.x
Peil, A., Richter, K., Garcia-Libreros, T., Hanke, M.-V., Flachowsky, H., Celton, J.-M., et al. (2008). Confirmation of the fire blight QTL of Malus × robusta 5 on linkage group 3. Acta Hortic. 793, 297–303.
Peil, A., Wöhner, T., Hanke, M. V., Flachowsky, H., Richter, K., Wensing, A., et al. (2014). Comparative mapping of fire blight resistance in Malus. Acta Hortic. 1056, 47–51. doi: 10.17660/ActaHortic.2014.1056.4
Ramos, L. S., Lehman, B. L., Sinn, J. P., Pfeufer, E. E., Halbrendt, N. O., and McNellis, T. W. (2013). The fire blight pathogen Erwinia amylovora requires the rpoN gene for pathogenicity in apple. Mol. Plant Pathol. 14, 838–843. doi: 10.1111/mpp.12045
Ramos, L. S., Sinn, J. P., Lehman, B. L., Pfeufer, E. E., Peter, K. A., and McNellis, T. W. (2015). Erwinia amylovora pyrC mutant causes fire blight despite pyrimidine auxotrophy. Lett. Appl. Microbiol. 60, 572–573. doi: 10.1111/lam.12417
Schröpfer, S., Böttcher, C., Wöhner, T., Richter, K., Norelli, J., Rikkerink, E. H. A., et al. (2018). A single effector protein, AvrRpt2EA, from Erwinia amylovora can cause fire blight disease symptoms and induces a salicylic acid-dependent defense response. Mol. Plant-Microbe Interact. 31, 1179–1191. doi: 10.1094/MPMI-12-17-0300-R
Smits, T. H. M., Guerrero-Prieto, V. M., Hernández-Escarcega, G., Blom, J., Goesmann, A., Rezzonico, F., et al. (2014). Whole-genome sequencing of Erwinia amylovora strains from Mexico detects single nucleotide polymorphisms in rpsL conferring streptomycin resistance and in the avrRpt2 effector altering host interactions. Genome Announc. 2, e01229–e01213. doi: 10.1128/genomeA.01229-13
Sobiczewski, P., Lakimova, E. T., Mikiciński, A., Węgrzynowicz-Lesiak, E., and Dyki, B. (2017). Necrotrophic behaviour of Erwinia amylovora in apple and tobacco leaf tissue. Plant Pathol. 66, 842–855. doi: 10.1111/ppa.12631
Sobiczewski, P., Mikiciński, A., and Dyki, B. (2014). Survival of Erwinia amylovora in dead apple leaf tissue. Acta Hortic. 1056, 71–75. doi: 10.17660/ActaHortic.2014.1056.8
Sobiczewski, P., Mikiciński, A., Lewandowski, M., Żurawicz, E., Peil, A., Richter, K., et al. (2011). Selection for fire blight resistance of apple genotypes originating from European genetic resources and breeding programs. Acta Hortic. 896, 399–405. doi: 10.17660/ActaHortic.2011.896.57
Thomma, B. P. H. J., Nürnberger, T., and Joosten, M. H. A. J. (2011). Of PAMPs and effectors: The blurred PTI-ETI dichotomy. Plant Cell 23, 4–15. doi: 10.1105/tpc.110.082602
Toruño, T. Y., Stergiopoulos, I., and Coaker, G. (2016). Plant-pathogen effectors: Cellular probes interfering with plant defenses in spatial and temporal manners. Annu. Rev. Phytopathol. 54, 419–441. doi: 10.1146/annurev-phyto-080615-100204
van de Weg, E., Di Guardo, M., Jänsch, M., Socquet-Juglard, D., Cost, F., Baumgartner, I., et al. (2018). Epistatic fire blight resistance QTL alleles in apple cultivar ‘Enterprise’ and selection X-6398 discovered and characterized through pedigree-informed analysis. Mol. Breed. 38:5. doi: 10.1007/s11032-017-0755-0
van Schie, C. C. N., and Takken, F. L. W. (2014). Susceptibility genes 101: How to be a good host. Annu. Rev. Phytopathol. 52, 551–581. doi: 10.1146/annurev-phyto-102313-045854
Vogt, I. (2018). Fire blight: identification, cloning and functional characterization of related genes on Malus × robusta. [dissertation thesis/dissertation aus dem]. Dresden: Julius Kühn-Institut Technischen Universität.
Vogt, I., Wöhner, T., Richter, K., Flachowsky, H., Sundin, G. W., Wensing, A., et al. (2013). Gene-for-gene relationship in the host-pathogen system Malus xrobusta 5-Erwinia amylovora. New Phytol. 197, 1262–1275. doi: 10.1111/nph.12094
Winslow, C. E. A., Broadhurst, J., Buchanan, R. E., Krumwiede, C., Rogers, L. A., and Smith, G. H. (1920). The families and genera of the bacteria. Final report of the Committee of the Society of American Bacteriologists on the characterization and classification of bacterial types. J. Bacteriol. 5, 191–229.
Wöhner, T. W., Flachowsky, H., Richter, K., Garcia-Libreros, T., Trognitz, F., Hanke, M.-V., et al. (2014). QTL mapping of fire blight resistance in Malus × robusta 5 after inoculation with different strains of Erwinia amylovora. Mol. Breed. 34, 217–230. doi: 10.1007/s11032-014-0031-5
Wöhner, T., Richter, K., Sundin, G. W., Zhao, Y., Stockwell, V. O., Sellmann, J., et al. (2017). Inoculation of Malus genotypes with a set of Erwinia amylovora strains indicates a gene-for-gene relationship between the effector gene eop1 and both Malus floribunda 821 and Malus ‘Evereste’. Plant Pathol. 67, 938–947. doi: 10.1111/ppa.12784
Zhao, Y., He, S. Y., and Sundin, G. W. (2006). The Erwinia amylovora avrRpt2EA gene contributes to virulence on pear and AvrRpt2EA ss recognized by Arabidopsis RPS2 when expressed in Pseudomonas syringae. Mol. Plant-Microbe Interact. 19, 644–654. doi: 10.1094/MPMI-19-0644
Keywords: apple, AvrRpt2EA, effectors, SNP, susceptible, virulence, gene-for-gene, wild apple
Citation: Emeriewen OF, Wöhner T, Flachowsky H and Peil A (2019) Malus Hosts–Erwinia amylovora Interactions: Strain Pathogenicity and Resistance Mechanisms. Front. Plant Sci. 10:551. doi: 10.3389/fpls.2019.00551
Edited by:
Jeremy Astier, INRA UMR1347 Agroécologie, FranceReviewed by:
Tim McNellis, Pennsylvania State University, United StatesGennaro Fazio, Plant Genetic Resources Unit (USDA ARS), United States
Copyright © 2019 Emeriewen, Wöhner, Flachowsky and Peil. This is an open-access article distributed under the terms of the Creative Commons Attribution License (CC BY). The use, distribution or reproduction in other forums is permitted, provided the original author(s) and the copyright owner(s) are credited and that the original publication in this journal is cited, in accordance with accepted academic practice. No use, distribution or reproduction is permitted which does not comply with these terms.
*Correspondence: Ofere Francis Emeriewen, b2ZlcmUuZW1lcmlld2VuQGp1bGl1cy1rdWVobi5kZQ==
Andreas Peil, YW5kcmVhcy5wZWlsQGp1bGl1cy1rdWVobi5kZQ==