- 1Department of Mathematics, University of Sussex, Brighton, United Kingdom
- 2Department of Chemistry of Bioactive Nitrogen-Containing Heterocyclic Compounds, Institute of Bioorganic Chemistry and Petrochemistry, National Academy of Sciences of Ukraine, Kyiv, Ukraine
- 3Department of General and Soil Microbiology, Zabolotny Institute of Microbiology and Virology, National Academy of Sciences of Ukraine, Kyiv, Ukraine
- 4Department of Cell Biology and Biotechnology, Institute of Food Biotechnology and Genomics, National Academy of Sciences of Ukraine, Kyiv, Ukraine
- 5Department of Genomics and Molecular Biotechnology, Institute of Food Biotechnology and Genomics, National Academy of Sciences of Ukraine, Kyiv, Ukraine
With the growing global demands on sustainable food production, one of the biggest challenges to agriculture is associated with crop losses due to parasitic nematodes. While chemical pesticides have been quite successful in crop protection and mitigation of damage from parasites, their potential harm to humans and environment, as well as the emergence of nematode resistance, have necessitated the development of viable alternatives to chemical pesticides. One of the most promising and targeted approaches to biocontrol of parasitic nematodes in crops is that of RNA interference (RNAi). In this study we explore the possibility of using biostimulants obtained from metabolites of soil streptomycetes to protect wheat (Triticum aestivum L.) against the cereal cyst nematode Heterodera avenae by means of inducing RNAi in wheat plants. Theoretical models of uptake of organic compounds by plants, and within-plant RNAi dynamics, have provided us with useful insights regarding the choice of routes for delivery of RNAi-inducing biostimulants into plants. We then conducted in planta experiments with several streptomycete-derived biostimulants, which have demonstrated the efficiency of these biostimulants at improving plant growth and development, as well as in providing resistance against the cereal cyst nematode. Using dot blot hybridization we demonstrate that biostimulants trigger a significant increase of the production in plant cells of si/miRNA complementary with plant and nematode mRNA. Wheat germ cell-free experiments show that these si/miRNAs are indeed very effective at silencing the translation of nematode mRNA having complementary sequences, thus reducing the level of nematode infestation and improving plant resistance to nematodes. Thus, we conclude that natural biostimulants produced from metabolites of soil streptomycetes provide an effective tool for biocontrol of wheat nematode.
1. Introduction
One of the biggest challenges to agriculture comes from pests and parasites that cause enormous economic losses and threaten global food security. Plant parasitic nematodes are known to infest almost all cultivated crops, and they result in losses of over 20% of the annual yield (Jung and Wyss, 1999), and estimated economic losses of over $130 billion worldwide (Chitwood, 2003). The largest damage to crops can be attributed to sedentary endoparasitic nematodes of the Tylenchoidea superfamily, which includes root-knot nematodes (Meloidogyne spp.) and cyst nematodes (Globodera and Heterodera spp.) (Tamilarasan and Rajam, 2013). Symptoms of nematode-related plant disease include wilting, stunting, and enhanced susceptibility to other diseases. While cyst nematodes are quite specific in the type of host plants they choose to parasitize on, root-knot nematodes do not have such high host specificity and can infect a very wide range of different crops. Irrespective of the type of nematodes, when their juveniles reach the second stage J2 (infective juveniles), they migrate from the soil into new host roots, or use the same host plant as the one used by their parent. In case of migration through the soil, these juveniles use lipids stored in their gut to sustain them for a short period of time needed to get established in a new host. Inside the plant, the nematodes migrate intra-cellularly (cyst nematodes) or inter-cellularly (root-knot nematode) to become sedentary in the roots and form feeding cells that support further development and reproduction (Bird and Kaloshian, 2003; Caillaud et al., 2008). These feeding cells later transform into either syncytia in the case of cyst nematodes, or multinucleate giant cells for root-knot nematodes, which subsequently result in the formation of galls and plant death after several cycles of nematode reproduction. In the case of wheat (Triticum aestivum L.), its major nematode parasites are cereal cyst nematode, primarily Heterodera avenae (Nicol et al., 2007; Peng et al., 2009), and root lesion nematode Pratylenchus (P. neglectus and P. thornei) (Vanstone et al., 1998). Smiley and Nicol (2009) and Nicol and Rivoal (2008) provide nice overviews of different species of wheat nematodes, including the discussion of their epidemiology, as well as approaches to management.
Over the years various control measures have been proposed to reduce the negative impact of nematodes on the performance of crops in general, and wheat in particular. These include application of chemical nematicides, development and use of organic cultivars, and crop rotation (Tamilarasan and Rajam, 2013). In the case of wheat, management and sanitation can be achieved using rotation with non-cereal, resistant or tolerant cultivars (Smiley and Nicol, 2009). Despite successes of synthetic chemical pesticides in mitigating damage and protecting crop from pests, other options are currently being explored due to the emergence of resistance in pest populations, and concerns over safety to humans and environment associated with ground water contamination and residues in food (Thomason, 1987).
One promising approach for crop protection and control of nematodes is RNA interference (RNAi) (Saurabh et al., 2014; Kamthan et al., 2015; Li et al., 2015; Kanakala and Ghanim, 2016; Rehman et al., 2016; Ali et al., 2017a; Banerjee et al., 2017; Borel, 2017; Majumdar et al., 2017), which is a fundamental biological process, through which eukaryotic cells are able to post-transcriptionally control expression of specific genes. The process starts with either exogenous (in the case of plant viruses) or endogenous (host's own) double-stranded RNA (dsRNA), which is cleaved by the Dicer enzyme into 21–25nt long small interfering RNA (siRNA). These are then unwound, and the passenger strand is discarded, while the guide strand is loaded onto Argonaute protein to form the RNA-induced silencing complex (RISC) that is able to degrade complementary mRNA, thus stopping it from being translated into protein (Hammond et al., 2000; Filipowicz, 2005; Carthew and Sontheimer, 2009). Host cells can also take RNAs from viruses and make them double stranded using RNA-dependent RNA polymerases (Wassenegger and Pelissier, 1998; Lipardi et al., 2001; Makeev and Bamford, 2002). One should also note that sRNAs do not only target mRNAs. In fact, most siRNAs target non-coding RNAs (Hüttenhofer et al., 2005; Chen and Aravin, 2015; Wang et al., 2015), while miRNA target mRNAs, as well as non-coding RNAs, which results in the production of phasiRNA (Ye et al., 2014; Liu et al., 2015). For the purpose of controlling plant infections, RNAi can be used in two different ways: it can protect the plants from infection, and it can be used to target the parasites. Protecting plants from infection relies on the plant possessing appropriate dsRNA, so that it could produce the RISC, which would stop expression of proteins necessary for the successful infection. Targeting parasites is a strategy where upon feeding on plants, insects or nematodes would consume dsRNA that upon entering their gut would trigger the process of RNAi against their own genes, thus reducing their fecundity and causing mortality (Fairbairn et al., 2007; Charlton et al., 2010; Dalzell et al., 2010; Li et al., 2010, 2015; Duan et al., 2012). In both of these approaches the important first step is supplying plants with the appropriate dsRNA. This can be achieved either by developing transgenic plants able to produce necessary dsRNA through their own cell machinery (Zhang et al., 2015), or by providing it externally through spraying, root soaking etc. (Li et al., 2015; Joga et al., 2016; Heidebrecht, 2017). It has been shown recently that it is possible to develop transgenic plants carrying RNAi constructs providing protection against fungal infections of peanut (Arias et al., 2015) and wheat (Chen et al., 2016). One of the biggest challenges with spraying dsRNA on crops on agricultural scale is that it gets washed away with rain and is quite quickly degraded by the soil. A recent work has demonstrated that this problem can be overcome by loading dsRNA on clay nano-particles that in a field experiment stayed on the surface of leaves up to 30 days after application of the spray (Mitter et al., 2017).
In the context of nematode infections of crops, transgenic plants can quite effectively produce dsRNA that targets various nematode housekeeping genes, as well as parasitism or effector genes. For example, tobacco plant has been designed to express dsRNA of target genes of Meloidogyne incognita (Yadav et al., 2006), soybean has been engineered to express dsRNA to target a few house-keeping genes of Heterodera glycines (Klink et al., 2009; Li et al., 2010), and rapeseed plant has been designed to express dsRNA to target an important secretory protein of Heterodera schachtii (Tsygankova et al., 2013). Feeding dsRNA directly to nematode larvae is able to induce RNA silencing in western corn rootworm (Bolognesi et al., 2012) and colorado potato beetle (Baum et al., 2007). Urwin et al. (2002) have shown how octopamine can induce uptake of dsRNA by H. glycines and Globodera pallida.
Another prominent alternative strategy for crop protection is the use of biopesticides, in particular, natural biostimulants that are usually metabolites of plants or soil bacteria (Chandler et al., 2011; Ruiu, 2015; Yakhin et al., 2017). A major source of natural biostimulants are soil actinobacteria, such as Saccharopolyspora and Streptomyces genera. One example is Streptomyces avermitilis that produces Avermectins having insecticidal and nematicidal properties (Putter et al., 1981; Turner and Schaeffer, 1989). These macrocyclic lactone compounds target the GABA receptors in the peripheral nervous system of the insects and nematodes, causing inhibition of neurotransmission and paralysis of the neuromuscular systems (Bloomquist, 1996; Cabrera et al., 2013). Products of different streptomycetes have recently been shown provide effective nematicidal action (Ruanpanun et al., 2010; Rashad et al., 2015; Kaur et al., 2016). Recent work has shown that metabolites produced by some of the streptomycetes have a significant positive effect on plant performance through improved growth and development, and they also yield protection against nematode through stimulating production in plant cells of appropriate si/miRNA (Jailani and Mukherjee, 2017; Tsygankova et al., 2019). More specifically, metabolites and cell culture supernatant of soil streptomycetes S. avermitilis (Biliavska et al., 2012; Iutynska, 2012), S. netropsis (Biliavska et al., 2016a), and S. violaceus (Biliavska et al., 2016b) have been shown to possess strong antagonistic activity against various phytopathogenic micromycetes and bacteria (Iutynska et al., 2017). Earlier work (Biliavska et al., 2012, 2015a; Iutynska et al., 2017) has shown that when the selected streptomycetes are cultivated in liquid nutrient media, they simultaneously produce a number of distinct biologically active compounds, such as antibiotics (heptaene antibiotic candidin for S. netropsis, anthracycline antibiotics rhodilunantsin A and rhodilunantsin B for S. violaceus, and macrocyclic avermectins for S. avermitilis), amino acids, lipids, phytohormones (auxins, cytokinins, gibberellins, abscisic acid), and steroid compounds (cholesterol, ergosterol, sitosterol, stigmasterol, 24-epibrassinolide, squalene). The presence of these different biological products in the cultural liquid supernatant and biomass ethanol extracts means that the resulting biostimulants are actually complex poly-component products, which together provide a significant improvement of plant growth and development (Ponomarenko and Iutynska, 2011). This positive effect of biostimulants on plant growth has been demonstrated through improved callogenesis and organogenesis in wheat (Tsygankova et al., 2016, 2017). Biostimulants developed on the basis of metabolites of these streptomycetes have also shown nematicidal effects on root-knot nematode M. incognita in vitro (Biliavska et al., 2015a), as well as bioprotective RNAi-mediated effects against cyst nematode H. schachtii in rape (Tsygankova et al., 2013, 2014a), Brassica rapa subs. pekinensis (Chinese cabbage) (Biliavska et al., 2016c), and sugar beet (Tsygankova et al., 2012b), as well as on both of these nematodes in sugar beet and cucumber (Tsygankova et al., 2014b). It should be noted that we are using the term “biostimulant” loosely to describe poly-component substances without discriminating them by the mode of their action, such as biopesticides, plant growth regulators etc., hence avoiding the need to abide by one of the more restrictive definitions Calvo et al., 2014; Brown and Saa, 2015; du Jardin, 2015; Nardi et al., 2016.
To facilitate the development of optimal strategies for delivery of biostimulants into the plants, it is essential to understand how complex mixtures of chemical compounds are taken up by plants from the soil, as well as how they are subsequently transported within plants. Experimentally driven mathematical models suggest that equilibrium concentrations of chemical compounds in plants are reached very quickly, when plants are taking up compound from the soil through their roots (Briggs et al., 1983; Fryer and Collins, 2003; Rein et al., 2011). Theoretical models of RNAi (Groenenboom and Hogeweg, 2008; Neofytou et al., 2016a,b, 2017) have shown that the rate at which proliferating cells of meristematic tissue mature into healthy plant cells plays an important role in determining the success of RNAi-based plant response, which suggests that the transport of these products to different parts of the plant during its growth has a knock-on effect on their availability for consumption by nematodes. Furthermore, one should be mindful of the fact that in the case of poly-component mixtures, diffusion and uptake of different chemical components and their subsequent within-plant transport can significantly differ due to their various characteristics, such as lipophilicity, acidity, and electrical charge. Existing studies on the dynamics of germination and associated water uptake suggest that a significant role in this process is played by the seed morphology, which should be important in the case where biostimulants are applied directly to germinating seeds prior to them being sowed, rather than to the soil.
This paper analyses how poly-component biostimulants obtained on the basis of metabolites of soil streptomycetes can be used to protect wheat (Triticum aestivum L. cv. Zimoyarka) against the cereal cyst nematode H. avenae through stimulating production in plant cells of si/miRNA that are complementary with nematode mRNA upon being consumed by nematode from plants. The underlying idea of this methodology is the so-called host-induced gene silencing (HIGS), whereby the transfer of RNA in the form of either dsRNA or si/miRNA generated within host results in silencing essential nematode genes that are involved in nematode multiplication and/or egg production, parasitism and housekeeping (Fairbairn et al., 2007; Lilley et al., 2012; Nunes and Dean, 2012; Koch and Kogel, 2014). A very important point is that biostimulants used in this study are natural products of soil streptomycetes that are able to deliver targeted protection against the nematode, thus providing a safe and effective tool of biocontrol.
One particular problem for efficient plant-induced RNAi is a significant variability that has been observed in the efficiency of RNAi in nematodes in response to differences in sRNA concentrations, sizes of dsRNA constructs, and the duration of exposure (Gheysen and Vanholme, 2007; Maule et al., 2011; Lilley et al., 2012). Another major challenge is identifying specific RNAi compounds that are being transferred between plants and nematodes, i.e., whether it is the unprocessed dsRNA that subsequently has to be processed into siRNA by nematodes, or the siRNA, resulting from processing of these dsRNAs by plants using their DICER enzymes (Gheysen and Vanholme, 2007; Lilley et al., 2012). Even though significant amount of work has been done recently on trying to understand trans-kingdom transfer of small RNAs, and more specifically on the transfer of RNA between plants and their parasitic nematodes, many aspects of such transfer remain poorly understood (Knip et al., 2014; Sarkies and Miska, 2014; Han and Luan, 2015; Zhou et al., 2017; Cai et al., 2018; Baldrich et al., 2019), thus limiting our ability to design effective tools for host-induced RNAi.
Efficiency of plant-induced RNAi relies on the knowledge of appropriate nematode genetic targets for RNAi, and some progress has been made recently on identifying such targets for a number of agriculturally important nematodes (Koch and Kogel, 2014). In one recent study, Liu et al. (2016) have shown the effectiveness of RNAi on silencing the expression of HaEXPB2 in H. avenae by in vitro soaking H. avenae larvae in homologous dsRNA, thus reducing its parasitism on N. benthamiana. Yang et al. (2017) have sequenced mRNA from both pre- and post-parasitic stages of H. avenae, providing an extensive characterization of development, metabolism, and parasitism genes. A recent work by Cui et al. (2018) has identified three specific H. avenae genes c59821_g1, c69968_g1, and c45915_g1 associated with lethal phenotypes, which suggests that they can be used as effective genetic targets for plant-induced RNAi. Similarly, Zheng et al. (2015) have studied transcriptome of second-stage juveniles of H. avenae and shown that silencing unigene38116, unigene102492, and unigene38007 genes, which are homologs of C. elegans genes C18D1.3, F38E11.7, and C06G4.2, respectively, by soaking nematode larvae in the gene-specific siRNA has lethal effect on the nematode. Kumar et al. (2014) have also analyzed transcriptome of second-stage juveniles of H. avenae, showing that it has the highest degree of similarity to a potato cyst nematode G. pallida and identified a number of candidate genes that can be used as targets for RNAi.
As mentioned earlier, one of the biggest challenges to RNAi-based biocontrol is the production in plants of si/miRNA complementary to nematode mRNA, as well as the delivery of necessary RNAi products to nematodes. Using some of the above-mentioned insights from mathematical models of plant uptake of chemical compounds, as well as the dynamics of RNAi in plants, we perform experiments on triggering nematode-specific si/miRNA production in wheat by using several streptomycete-derived biostimulants. The results suggest that these biostimulants are indeed very effective in protecting wheat against the cereal cyst nematode.
2. Materials and Methods
2.1. Biostimulants and Experimental Set-Up
Biostimulants used in this study are products based on metabolites of soil streptomycetes S. avermitilis, S. netropsis, and S. violaceus. Avermectins produced by S. avermitilis have long been known to possess insectidical, acaricidal, and nematicidal properties (Putter et al., 1981; Cabrera et al., 2013). In light of these observations, a strain S. avermitilis IMV Ac-5015 was grown in liquid organic soya media in bioreactors for 7 days at a temperature of +28±1°C (Biliavska et al., 2012, 2015b; Iutynska et al., 2017). After reaching the phase of stationary growth, the streptomycete's biomass was separated by centrifugation. Extraction of the streptomycete's metabolites in the biomass and supernatant of the cultural liquid was then carried out with ethanol at a ratio of 1:1 or 1:3 for one day, and subsequently the biomass was centrifuged. The biostimulant denoted as Avercom was obtained as a mixture of this cultural liquid supernatant extract and biomass extract at a 1:1 ratio, and was then stored at +4°C. Chemical analysis of this biostimulant showed that it contains avermectins at concentration of 100 μg/mL. The second biostimulant, Avercom nova-2, is a derivative of Avercom and contains 50mL of cell culture supernatant added to 50 mL of biomass extract of S. avermitilis IMV Ac-5015, with the addition of 0.01 mM of chitosan, a natural compound with known elicitor effects in plants (Hadrami et al., 2010; Malerba and Cerana, 2016). Exactly the same methodology was used to produce Phytovit from the streptomycete S. netropsis IMV Ac-5025, and Violar from S. violaceus IMV Ac-5027, with the only distinction being that for Phytovit and Violar the ratio of cultural liquid supernatant extract to biomass extract was 4:1. All these biolostimulants were tested in the laboratory setting prior to being used for plant treatment.
To investigate the content of auxins, cytokinins, and abscisic acid in biostimulants, we used quantitative spectrodensitometric thin-layer chromatography (TLC) (Negretskii, 1988; Savinskiy et al., 1991; Zizková et al., 2017), while gibberellins were measured by the spectrophotometric method (Muromtsev and Agnistikova, 1984; Waadt et al., 2015). The analysis of steroid compounds was carried out by gas chromatographic mass spectrometry on the device 6890N/5973inert (Agilent Technologies, USA) (Kamthan et al., 2012).
Working only with virus-free lines of plants, for the first set of experiments, seeds of wheat Triticum aestivum L. cv. Zimoyarka were surface sterilized successively in 1% KMnO4 solution (3 mins), 1% AgNO3 solution (2 mins), and 96% ethanol solution (1 min), after which they were thrice washed in distilled water. With the aim of stimulating seed germination and growth of wheat seedlings, as well as improving resistance to nematodes in the laboratory conditions, we have used the following physiologically optimal concentrations of microbial biostimulants
For lower concentrations, the positive effect was reduced, while concentrations above 3 μL/L resulted in inhibition of seed germination and growth of wheat seedlings, as well as reduced resistance to nematode infestation. The seeds were germinated for 3 days in darkness at temperature 23°C in perlite-filled cuvettes, each containing 20 seeds and either distilled water or a solution of a biostimulant Avercom, Avercom nova-2, Phytovit, and Violar (Tsygankova et al., 2016). Upon germination, seedlings were grown for the next 3 weeks at 22–24°C under a 16/8 h photoperiod (light intensity 3000 lux) with relative humidity 60–80% in perlite-filled cuvettes. The artificial nematode-infested background was created by adding second stage larvae of the H. avenae nematode directly to perlite-filled cuvettes at concentration of 3–5 larvae per 1 mL of suspension at a rate of 5 mL per each wheat plant seed (i.e., 15–25 nematode larvae per each wheat plant seed). Additionally, leaf and stem surfaces of wheat seedlings were inoculated with suspension of nematode H. avenae larvae once per week at weekly intervals. Nematode larvae were obtained from nematode eggs using the method described in detail in our earlier published work (Tsygankova et al., 2012a).
The infection in our experiment occurred from the very start, due to the seeds growing on invasive background. As one of the parameters characterizing the effects of biostimulants on plants, we measured plant viability. With several different approaches being proposed over the years to define and measure viability of seeds (Scharpf, 1970; Rao et al., 2006; Shaban, 2013) and plants (Stergios and Howell, 1973; von Fircks and Verwijst, 1993), in our experiments we measured viability by planting 75 seeds in each trial, and then determining the percentage of surviving wheat plant seedlings at the end of the 3-week observation period. In terms of identifying the effects of biostimulants on plant growth, development, and protection against nematodes, we measured the percentage reduction in the overall level of nematode infestation on the surface of roots, stems, and leaves for experimental wheat seedlings grown on the invasive background in perlite filled cuvettes with biostimulants applied in planta, compared to control wheat seedlings to which no biostimulants were applied. The measurements were performed at the end of the 3-week period from the time the plant seeds were sown to the perlite-filled cuvettes with nematode larvae.
2.2. Isolation of Cytoplasmic mRNA and si/miRNA
To verify that biostimulants do indeed promote the additional production of plant si/miRNA complementary to either plant mRNA or nematode mRNA, we extracted total cytoplasmic RNA from plant cells using standard methods (Aviv and Leder, 1972; Davis et al., 1986; Tsygankova et al., 1998). The polymericity of isolated total RNA preparations was analyzed by electrophoresis in a 1.5% agarose gel stained with ethidium bromide in the presence of 7M urea by the Locker method (Locker, 1979). Separation of cytoplasmic poly(A)+mRNA (that is, mRNA) and poly(A)−mRNA was carried out by chromatography on oligo(dT) cellulose column (Aviv and Leder, 1972; Sambrook et al., 1989), with the purity of isolated cytoplasmic poly(A)+mRNA being analyzed using the Northern-blot method (Sambrook et al., 1989). Plant cytoplasmic si/miRNA was isolated from cytoplasmic RNA using the method we had developed earlier to analyse RNAi in rapeseed (Tsygankova et al., 2012a), and which was subsequently used to study RNAi in sugar beet (Tsygankova et al., 2012b), cucumber and potato (Tsygankova et al., 2014b), and Chinese cabbage (Biliavska et al., 2016c). We then performed dot blot hybridization (Sambrook et al., 1989) between this plant cytoplasmic si/miRNA and cytoplasmic mRNA isolated either from plant cells, or from nematode larvae cells. For the purpose of dot blot hybridization experiments, before isolation from the total plant cytoplasmic RNA population, si/miRNA were labeled in vivo with [33P] using Na2HP33O4 (Tsygankova et al., 2012a, 2014a,b). On the other hand, for experiments on inhibition of protein expression in a wheat germ cell-free system of protein synthesis (Marcus et al., 1974; Sambrook et al., 1989; Tuschl et al., 1999; Spirin and Swartz, 2008; Takai et al., 2010; Tsygankova et al., 2010), the original unlabeled si/miRNA were used (Tsygankova et al., 2014a,b). Purity of isolated si/miRNAs (i.e., the fact that their sizes were 21–25nt) was verified through electrophoresis in a 15% polyacrylamide gel (GE Healthcare Amersham, UK), which was stained with ethidium bromide solution and photographed under UV light (Sambrook et al., 1989). Once the gel was vacuum dried in a thermal gel dryer (LKB, Sweden), a fluorescent agent 2,5-diphenyl-1,3-oxazole (Abakumov et al., 1980) was added, and the gel was exposed for 2 months to X-ray film at -70°C for gel fluorography (Bonner and Laskey, 1974). Total cytoplasmic mRNA from cells of cereal cyst nematode H. avenae was isolated from nematode larvae using the methodology discussed in Tsygankova et al. (2012a).
2.3. Analysis of Synthesis of si/miRNAs Within Plant Cells and Their Silencing Activity
We studied the impact of biostimulants on production within plant cells of si/miRNA that target both plant mRNA associated with plant host genes, whose expression is upregulated during nematode infestation, thus promoting penetration of nematodes into plant roots (Safari et al., 2005; Tsygankova et al., 2012a, 2014a; Kong et al., 2015; Ali et al., 2017a; Chen et al., 2017), and these can be used as target genes for silencing (Koch and Kogel, 2014), and nematode mRNA associated with genes that control nematode life cycle, nematode housekeeping genes, nematode parasitism, or effector genes that are expressed during nematode entrance into plant cells (Tsygankova et al., 2012a, 2014a; Li et al., 2014; Chen et al., 2015, 2017; Xu et al., 2016; Ali et al., 2017b; Yang et al., 2017; Cui et al., 2018). For this purpose we perform dot blot hybridization of [33P]-labeled si/miRNA isolated from experimental plants with mRNA isolated from control plants, i.e., plants uninfested by nematodes and not treated by biostimulants, as well as with mRNA molecules isolated from nematode larvae. si/miRNA is isolated from nematode-infested plants grown in perlite-filled cuvettes from seeds treated by biostimulants in planta. To avoid potential losses of nucleic acids, hybridization was performed on Millipore AP-15 glass fiber filters composed of APT paper Kahl (2015). The filters were then dried, and the radioactivity of hybrid molecules per 20 μg±SD of mRNA was determined in a Beckman LS-100C counter using a toluene-based scintillation fluid (Tishler and Epstein, 1968; Bonner and Laskey, 1974). The level of hybridization provides a quantitative characteristic of the amount of in planta synthesized si/miRNA that is complementary with either plant mRNA, or nematode mRNA. To compare the effects of different biostimulants, levels of hybridization between plant si/miRNA and plant own mRNA were compared to baseline obtained for control plants uninfested by nematode and grown without the use of biostimulants.
To study silencing (inhibitory) activity of plant-derived si/miRNA, i.e., si/miRNA-mediated effects on translation of nematode mRNA, we used a wheat germ cell-free system of protein synthesis, which, together with other heterologous cell-free systems, is widely used to analyse the expression of proteins from viruses (Roberts and Paterson, 1973; Fogeron, 2015), plants (Takai et al., 2010; Harbers, 2014; Mmeka et al., 2014), mammals (Wakiyama et al., 2007; Singh et al., 2012), various prokaryotic and eukaryotic microorganisms (Guild et al., 2011; Harbers, 2014), and nematodes (El-Ansary and Al-Daihan, 2005; Wallace et al., 2010). It has been suggested that the wheat germ and other heterologous cell-free systems can also be used for studying the RNAi machinery or validating siRNAs for various organisms (Tuschl et al., 1999; Katzen et al., 2005; Mathonnet et al., 2007; Shyu et al., 2008). To improve the performance, the standard wheat germ extract system (Roberts and Paterson, 1973) that contains various cellular components essential for protein synthesis, was modified to include an energy generating system based on phosphocreatine kinase and phosphocreatine, magnesium acetate (Roy et al., 2011; Agalarov et al., 2014) and potassium acetate Wolin and Walter (1988) were added for improved translation, while additional stabilization was provided by adding spermidine (Spirin and Swartz, 2008). For the purpose of studying silencing (inhibitory) activity of si/miRNA, we used unlabeled si/miRNA obtained before their isolation from root and shoot tissues of wheat plants. Silencing activity was determined using the index of decreasing of incorporation [35S]-methionine into proteins (Sambrook et al., 1989; Titus, 1991; Spirin and Swartz, 2008). This index was measured as the level of radioactivity of polypeptides (in imp./count per min/1mg of proteins) obtained on a Millipore AP-15 glass fiber filter in toluene scintillator in the Beckman LS 100C scintillation counter (Bonner and Laskey, 1974; Osterman, 1981). The index of silencing activity of si/miRNA (in %) was then computed as a difference in the index of radioactivity of polypeptides synthesized on the template of nematode mRNA, obtained using si/miRNA isolated from experimental plants grown under application of biostimulants in planta, as compared to the same index obtained using si/miRNA isolated from control plants grown without biostimulants.
All experiments were performed in triplicate, and statistical analysis of data was performed using standard methods as described in Bang et al. (2010).
3. Results
In order to understand the effects on biostimulants on plant growth and development, in Figure 1 we show plant hormone content of different biostimulants, including both auxins, cytokinins, gibberellins, and anti-stress (abscisic acid, ABA) hormones. It is worth noting that unlike stimulatory plant hormones, the inhibitory hormone ABA is present in biostimulants at a much lower concentration. All auxins in biostimulants are represented by indole-3-acetic acid (IAA) and indole-3-butyric acid and their derivatives.
As a first step in our experimental studies, we look at the effects of biostimulants on viability of 3-week-old wheat plants, as well as on their ability to withstand nematode infestation. Figure 2A shows that all biostimulants have a positive effect on plant viability in the absence of nematode infestation, but the differences between their influence on improving the viability of plants are very small. In contrast, adding invasive background reduces the viability of plants grown without biostimulants to 32%, while applying biostimulants in planta results in mitigating this effect and restoring plant viability to between 57 and 92%. Besides having a stimulatory effect on plant survivability in the presence of invasive background, biostimulants also appear to possess significant anti-nematode effect, as illustrated in Figure 2B. This Figure suggests that all biostimulants provide a significant anti-nematode effect, reducing the level of nematode infestation by 73–83% compared to plants grown without biostimulants.
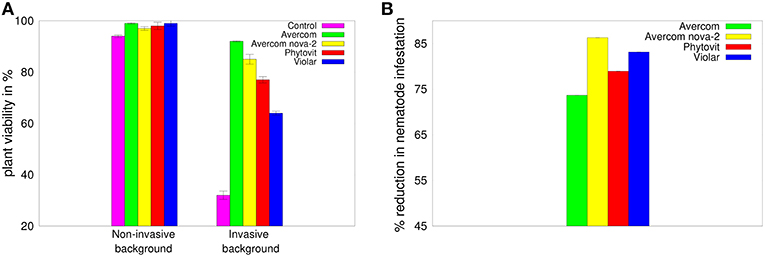
Figure 2. (A) Effects of biostimulants on viability of plants grown on non-invasive or invasive background in perlite-filled cuvettes from seeds treated in planta by biostimulants, as compared to a viability of control plants grown in perlite-filled cuvettes without biostimulants. (B) Reduction in infestation with H. avenae larvae of plants grown in perlite-filled cuvettes from seeds treated in planta by biostimulants, as compared to control plants grown in perlite-filled cuvettes in a nematode-infested background without biostimulants. The error bars in plot (B) are not visible, since they are almost 3 orders of magnitude smaller than the reported values.
Now that it has been established that biostimulants do have an anti-nematode effect, we look into whether this can be attributed to wheat plants producing additional si/miRNA complementary to mRNA of infested plants that is associated with plant genes, whose expression is upregulated during the entry of nematodes into plant cells, thus facilitating infestation of plants with nematodes, or to nematode mRNA associated with nematode genes that control nematode life cycle, nematode housekeeping genes, nematode parasitism or effector genes that are expressed during nematode entry into plant cells (Tsygankova et al., 2012a, 2014b; Li et al., 2014; Chen et al., 2015, 2017; Xu et al., 2016; Ali et al., 2017b; Yang et al., 2017; Cui et al., 2018).
The results of the dot blot hybridization between the si/miRNA isolated from plant cells grown without and with biostimulants with the mRNA extracted from either control plants, i.e., plants uninfested by nematodes and not treated by biostimulants, or from nematode larvae, are presented, respectively, in Figures 3A,B. In the case of hybridization with the plant mRNA, as shown in Figure 3A, we observe that biostimulants enhance the synthesis of si/miRNA complementary with plant mRNA by a factor of 1.7−3.09. Similar result is observed in Figure 3B for the experiment on dot blot hybridization between si/miRNA from nematode-infested plants and nematode mRNA, namely, application of biostimulants results in a significant enhancement of synthesis of si/miRNA complementary with nematode mRNA compared to plants grown from seeds without any biostimulants (Tsygankova et al., 2016). Interestingly, for Avercom and Avercom nova-2, the level of enhancement of synthesis of si/miRNA complementary with nematode mRNA is higher than analogous levels for si/miRNA homologous with plant mRNA, while for Phytovit the level of synthesis of si/miRNA complementary with nematode mRNA is smaller than the respective level for plant mRNA. Comparison with Figure 2 indicates that while the synthesis of complementary si/miRNA can be enhanced very significantly, the difference between levels of reduction in nematode infestation is much smaller, suggesting that out of the total si/miRNA produced in the plant, only a part of it contained in the feeding cells formed on plant roots can enter nematode through ingestion using a stylet (Maule et al., 2011; Tsygankova et al., 2012a; Cui et al., 2018), possibly resulting in the subsequent nematode death.
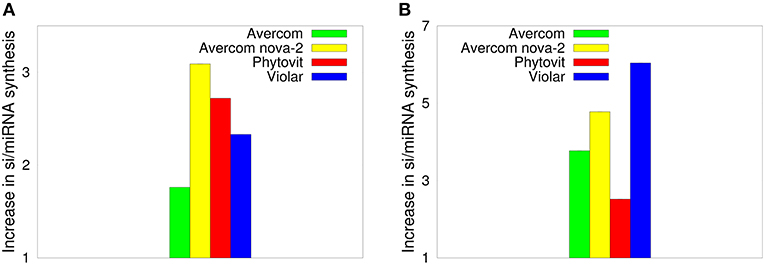
Figure 3. Effects of biostimulants on increasing the synthesis of si/miRNA in nematode-infested plants grown using biostimulants in planta that are complementary with mRNA from control plants (A), and with nematode mRNA (B), scaled by the amount of si/miRNA isolated from nematode-infested plants grown under the same conditions but without the use of biostimulants. The error bars are not visible, since they are three orders of magnitude smaller than the reported values.
To better understand how effective are the si/miRNA at suppressing nematode activity and causing their mortality, in Figure 4 we compare silencing activity of si/miRNA isolated from the plants grown without or with biostimulants, as measured in a wheat germ cell-free system. One observes a significant increase in silencing activity of si/miRNA on reducing the translation of proteins on the template of nematode mRNA (Tsygankova et al., 2016, 2019). Comparison of the results on silencing efficiency with Figure 3B suggests that it is not so much the actual amount of the additional si/miRNA produced in plant cells, but rather its specificity as determined by the level of silencing activity on the template of nematode mRNA that determines the degree to which biostimulants are effective in reducing nematode infestation.
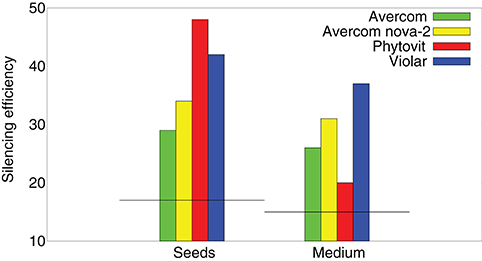
Figure 4. Effects of biostimulants on silencing activity of si/miRNA isolated from nematode-infested plants grown using biostimulants in planta, i.e., a percentage of inhibition of protein synthesis on the template of nematode mRNA in a wheat germ cell-free system, measured per 1μg of protein. Horizontal black lines denote the respective levels of silencing activity achieved by si/miRNA isolated from nematode-infested plants grown without the use of biostimulants.
These results show that biostimulants do indeed result in the production within wheat plants of si/miRNA complementary with nematode mRNA, and we have also observed a significant reduction in the overall protein production, as well as a marked increase in nematode mortality. Despite recent identification of some candidate genetic targets in H. avenae (Kumar et al., 2014; Cui et al., 2018), so far practical efforts have focused mostly on demonstrating the feasibility of RNAi by soaking nematode larvae either in dsRNA or gene-specific siRNA (Zheng et al., 2015; Liu et al., 2016), while plant-induced RNA silencing has remained elusive due to a significant variation in RNAi responses and poor understanding of the precise mechanisms of how RNAi products are transferred from plants into H. avenae (Maule et al., 2011; Lilley et al., 2012; Ali et al., 2019). In the absence of comprehensive understanding of genetic targets and the mechanisms of RNAi transfer between plant and nematode, we hypothesize that the observed reduction in the level of nematode infestation can be attributed to one of the genes, that are targeted by plant-produced RNAi products, being directly involved in protein synthesis, so silencing that particular gene results in the reduction of the overall protein level. Another possibility is that among the genes, whose expression is affected by the plant-produced sRNAs, there is one or several genes, whose silencing directly results in nematode death. With the recent work on splicing (Mackereth et al., 2011; Yang et al., 2016) demonstrating the possibility of multiple protein isoforms being encoded by the same genes, identifying the precise matching between sRNAs, produced in wheat plants under the influence of biostimulants, and specific H. avenae genes remains a challenging open problem.
4. Discussion
In this paper we have demonstrated the feasibility of using poly-component biostimulants derived from metabolites of various soil streptomycetes for protecting wheat plants against the cereal cyst nematode, which is known to be one of the main wheat parasites. Based on the guidance provided by theoretical models regarding how various chemical compounds are taken up by the plants through the seeds, we have subsequently performed experiments with biostimulants to investigate their efficiency in terms of plant uptake and subsequent nematicidal action.
Analysis of dot blot hybridization between the population of cytoplasmic si/miRNA from plants with mRNA from plants and nematodes suggests that application of biostimulants to germinating seeds results in the higher level of synthesis of si/miRNA in plant cells. These additional si/miRNAs are complementary to sequences present in the plant genes whose upregulated expression facilitates infestation of plants with nematodes, as well as to evolutionarily conserved sequences present in the nematode genes that control nematode life cycle, nematode housekeeping genes, nematode parasitism, or effector genes, and which are expressed during nematode entrance into plant cells (Tsygankova et al., 2012a, 2014a; Li et al., 2014; Chen et al., 2015, 2017; Xu et al., 2016; Yang et al., 2017; Cui et al., 2018). On the other hand, wheat germ cell-free experiments on silencing activity provide further evidence that biostimulants enhance the production in plant cells of si/miRNA that are capable of inhibiting (silencing) the translation of nematode mRNA having homologous evolutionarily conserved sequences, thus reducing the level of nematode infestation and improving plant resistance to nematodes. Taken together, these experiments demonstrate that as a method of nematode control, plant-delivered RNA interference is a complex process affected by a number of factors that include the mode of application of biostimulant, within-cell RNAi dynamics, specific properties of plant growth and development, as well as the uptake by nematodes and subsequent within-nematode dynamics. Although our results clearly indicate that streptomycete-derived biostimulants are indeed able to significantly reduce nematode infestation through plant-induced nematode mortality, the specific mechanism of genetic interactions between plant-produced RNAs and nematode mRNAs remains to be explored. At the same time, from a practical perspective these natural biostimulants do provide effective and safe means of nematode control for major staple crops.
There are several directions in which the work presented in this paper could be extended. Developing better theoretical models for uptake of biostimulants by seeds during their germination, as well as understanding more specific details of the transfer of biostimulants from the soil into plant roots, and the transfer of sRNAs from plants into nematode would provide important practical insights for optimizing the performance of biostimulants. Our future work will focus on identifying specific genes in H. avenae that are silenced by si/miRNAs produced in wheat plants under the action of biostimulants, which would provide a more targeted and effective approach to improving the performance of biostimulants as a means of nematode control.
Author Contributions
KB developed theoretical models, and together with FF prepared a summary of theoretical results. VT, GI, and YB designed the experiments. VT, LB, GI, AY, and YB performed the experiments. The manuscript was written by KB and FF, and edited by all authors. All authors read and approved the final manuscript.
Funding
FF has been sponsored by the Chancellor's Award from Sussex University. KB was supported by the interdisciplinary Data Intensive Science Centre at the University of Sussex (DISCUS). The work of VT, LB, GI, AY, and YB was financially supported through the interdisciplinary program of the National Academy of Sciences of Ukraine on “Molecular and cellular biotechnologies for medicine, industry and agriculture for 2015-2019”, within the project “Production of cell lines of agricultural plants with increased resistance to pathogenic and parasitic organisms by inducing RNA interference using bioregulators of microbial origin”.
Conflict of Interest Statement
The authors declare that the research was conducted in the absence of any commercial or financial relationships that could be construed as a potential conflict of interest.
References
Abakumov, G. A., Anisimov, Y. M., Polyakov, B. I., and Simonov, A. P. (1980). Spectral, fluorescent, photochemical and laser properties of some organic compound vapors. Appl. Phys. 23, 83–87.
Agalarov, S. C., Sakharov, P. A., Fattakhova, D. K., Sogorin, E. A., and Spirin, A. S. (2014). Internal translation initiation and eIF4F/ATP-independent scanning of mRNA by eukaryotic ribosomal particles. Sci. Rep. 4:4438. doi: 10.1038/srep04438
Ali, M. A., Azeem, F., Abbas, A., Joyia, F. A., Li, H., and Dababat, A. A. (2017a). Transgenic strategies for enhancement of nematode resistance in plants. Front. Plant Sci. 8:750. doi: 10.3389/fpls.2017.00750
Ali, M. A., Azeem, F., Li, H., and Bohlmann, H. (2017b). Smart parasitic nematodes use multifaceted strategies to parasitize plants. Front. Plant Sci. 8:1699. doi: 10.3389/fpls.2017.01699
Ali, M. A., Shahzadi, M., Zahoor, A., Dababat, A. A., Toktay, H., Baksh, A., et al. (2019). Resistance to cereal cyst nematodes in wheat and barley: an emphasis on classical and modern approaches. Int. J. Mol. Sci. 20:432. doi: 10.3390/ijms20020432
Arias, R. S., Dang, P. M., and Sobolev, V. S. (2015). RNAi-mediated control of aflatoxins in peanut: method to analyze mycotoxin production and transgene expression in the peanut/Aspergillus pathosystem. J. Vis. Exp. 106:53398. doi: 10.3791/53398
Aviv, H., and Leder, P. (1972). Purification of biologically active globin messenger RNA by chromatography on oligothymidylic acid-cellulose. Proc. Natl. Acad. U.S.A. 69, 1408–1412.
Baldrich, P., Rutter, B. D., Karimi, H. Z., Podicheti, R., Meyers, B. C., and Innes, R. W. (2019). Plant extracellular vesicles contain diverse small RNA species and are enriched in 10 to 17 nucleotide “tiny” RNAs. Plant Cell 31, 315–324. doi: 10.1105/tpc.18.00872
Banerjee, S., Banerjee, A., Gill, S. S., Gupta, O. P., Dhuja, A., Jain, P. K., et al. (2017). RNA interference: a novel source of resistance to combat plant parasitic nematodes. Front. Plant Sci. 8:834. doi: 10.3389/fpls.2017.00834
Bang, H., Zhou, X. K., Epps, H. L. V., and Mazumdar, M. (2010). Statistical Methods in Molecular Biology Series: Methods in Molecular Biology. New York, NY: Springer.
Baum, J. A., Bogaert, T., Clinton, W., Heck, G. R., Feldmann, P., Ilagan, O, et al. (2007). Control of coleopteran insect pests through RNA interference. Nat. Biotechnol. 25, 1322–1326. doi: 10.1038/nbt1359
Biliavska, L. A., Efimenko, T. A., Efremenkova, O. V., Koziritska, V. Y., and Iutynska, G. A. (2016a). Identification and antagonistic properties of the soil streptomycete Streptomyces sp. 100. Mikrobiol. Z. 78, 61–73. doi: 10.15407/microbiolj78.02.061
Biliavska, L. A., Pidlypska, V. A., Kozyritska, V. Y., and Iutsynska, G. A. (2015a). “Biosynthetic activity of soil streptomycetes - antagonists of plant-parasitic nematodes and phytopathogens,” in Proceedings of the 4th European Conference on Biology and Medical Sciences (Vienna:“East West" Association for Advanced Studies and Higher Education GmbH), 10–16.
Biliavska, L. O., Galagan, T. O., and Iutynska, G. O. (2016b). Antinematicidal activity of metabolites produced by soil streptomycetes. Mikrobiol. Z. 78, 34–47. doi: 10.15407/microbiolj78.04.034
Biliavska, L. O., Kozyritska, V. E., Kolomiets, Y. V., Babich, A. G., and Iutynska, G. O. (2015b). Phytoprotective and growth-regulatory properties of bioformulations on the base of soil streptomycetes metabolites. Dop. NANU 1, 131–137. Available online at: http://dspace.nbuv.gov.ua/handle/123456789/95709
Biliavska, L. O., Kozyritska, V. E., Valagurova, O. V., and lutynska, G. O. (2012). Biologically active substances of new microbial preparation Avercom. Mikrobiol. Z. 7, 10–15. Available online at: http://www.irbis-nbuv.gov.ua/cgi-bin/irbis_nbuv/cgiirbis_64.exe?C21COM=2&I21DBN=UJRN&P21DBN=UJRN&IMAGE_FILE_DOWNLOAD=1&Image_file_name=PDF/MicroBiol_2012_74_3_3.pdf
Biliavska, L. O., Tsygankova, V. A., Kozyritska, V. E., Iutynska, G. O., Andrusevich, Y. A., Babich, O. A., et al. (2016c). Application of new microbial plant resistance/plant growth protection inducers for increasing Chinese cabbage plant tolerance against parasitic nematode Heterodera schachtii Schmidt. Int. J. Res. Biosci. 5, 64–82. Available online at: https://www.ijrbs.in/abstract.php?article_id=1126
Bird, D. M., and Kaloshian, I. (2003). Are roots special? Nematodes have their say. Physiol. Mol. Plant Pathol. 62, 115–123. doi: 10.1016/S0885-5765(03)00045-6
Bloomquist, J. R. (1996). Ion channels as targets for insecticides. Annu. Rev. Entomol. 41, 163–190.
Bolognesi, R., Ramaseshadri, P., Anderson, J., Bachman, P., Clinton, W., Flannagan, R., et al. (2012). Characterizing the mechanism of action of double-stranded RNA activity against western corn rootworm (Diabrotica virgifera virgifera LeConte). PLoS ONE 7:e47534. doi: 10.1371/journal.pone.0047534
Bonner, W. M., and Laskey, R. A. (1974). A film detection method for tritium-labelled proteins and nucleic acids in polyacrylamide gels. Eur. J. Biochem. 46, 83–88.
Borel, B. (2017). CRISPR, microbes and more are joining the war against crop killers. Nature 543, 302–304. doi: 10.1038/543302a
Briggs, G. G., Bromilow, R. H., Evans, A. A., and Williams, M. (1983). Relationships between lipophilicity and the distribution of non-ionised chemicals in barley shoots following uptake by the roots. Pest Manage. Sci. 14, 492–500.
Brown, P., and Saa, S. (2015). Biostimulants in agriculture. Front. Plant Sci. 6:671. doi: 10.3389/fpls.2015.00671
Cabrera, J. A., Menjivar, R. D., Dababat, A. A., and Sikora, R. A. (2013). Properties and nematicide performance of Avermectins. J. Phytopathol. 161, 65–69. doi: 10.1111/jph.12014
Cai, Q., Qiao, L., Wang, M., He, B., Lin, F. M., Palmquist, J., et al. (2018). Plants send small RNAs in extracellular vesicles to fungal pathogen to silence virulence genes. Science 360, 1126–1129. doi: 10.1126/science.aar4142
Caillaud, M. C., Dubreuil, G., Quentin, M., Perfus-Barbeoch, L., Lecomte, P., de Almeida Engler, J., et al. (2008). Root-knot nematodes manipulate plant cell functions during a compatible interaction. J. Plant Physiol. 165, 104–113. doi: 10.1016/j.jplph.2007.05.007
Calvo, P., Nelson, L., and Kloepper, J. W. (2014). Agricultural uses of plant biostimulants. Plant Soil 383, 3–41. doi: 10.1007/s11104-014-2131-8
Carthew, R., and Sontheimer, E. (2009). Origins and mechanisms of miRNAs and siRNAs. Cell 136, 642–655. doi: 10.1016/j.cell.2009.01.035
Chandler, D., Bailey, A. S., Tatchell, G. M., Davidson, G., Greaves, J., and Grant, W. P. (2011). The development, regulation and use of biopesticides for integrated pest management. Philos. Trans. R. Soc. B 366, 1987–1998. doi: 10.1098/rstb.2010.0390
Charlton, W. L., Harel, H. Y., Bakhetia, M., Hibbard, J. K., Atkinson, H. J., and McPherson, M. J. (2010). Additive effects of plant expressed double-stranded RNAs on root-knot nematode development. Int. J. Parasitol. 40, 855–864. doi: 10.1016/j.ijpara.2010.01.003
Chen, C., Cui, L., Chen, Y., Zhang, H., Liu, P., Wu, P., et al. (2017). Transcriptional responses of wheat and the cereal cyst nematode Heterodera avenae during their early contact stage. Sci. Rep. 7:14471. doi: 10.1038/s41598-017-14047-y
Chen, C., Liu, S., Liu, Q., Niu, J., Liu, P., Zhao, J., et al. (2015). An ANNEXIN-like protein from the cereal cyst nematode Heterodera avenae suppresses plant defense. PLoS ONE 10:e0122256. doi: 10.1371/journal.pone.0122256
Chen, W., Kastner, C., Nowara, D., Oliveira-Garcia, E., Rutten, T., Zhao, Y., et al. (2016). Host-induced silencing of Fusarium culmorum genes protects wheat from infection. J. Exp. Bot. 67, 4979–4991. doi: 10.1093/jxb/erw263
Chen, Y.-C. A., and Aravin, A. (2015). Non-coding RNAs in transcriptional regulation. Curr. Mol. Biol. Rep. 1, 10–18. doi: 10.1007/s40610-015-0002-6
Chitwood, D. J. (2003). Research on plant-parasitic nematode biology conducted by the United States Department of Agriculture - Agricultural Research Service. Pest Manage. Sci. 59, 748–753. doi: 10.1002/ps.684
Cui, J.-K., Peng, H., Qiao, F., Wang, G. F., Huang, W. K., Wu, D. Q., et al. (2018). Characterization of putative effectors from the cereal cyst nematode Heterodera avenae. Phytopathology 108, 264–274. doi: 10.1094/PHYTO-07-17-0226-R
Dalzell, J. J., Warnock, N. D., Stevenson, M. A., Mousley, A., Fleming, C. C., and Maule, A. G. (2010). Short interfering rna-mediated knockdown of drosha and pasha in undifferentiated Meloidogyne incognita eggs leads to irregular growth and embryonic lethality. Int. J. Parasitol. 40, 1303–1310. doi: 10.1016/j.ijpara.2010.03.010
Davis, L. G., Dibner, M. D., and Battey, J. F. (1986). Basic Methods in Molecular Biology. New York, NY: Elsevier.
du Jardin, P. (2015). Plant biostimulants: definition, concept, main categories and regulation. Sci. Hortic. 196, 3–14. doi: 10.1016/j.scienta.2015.09.021
Duan, C.-G., Wang, C.-H., and Guo, H.-S. (2012). Application of RNA silencing to plant disease resistance. Silence 3:5. doi: 10.1186/1758-907X-3-5
El-Ansary, A., and Al-Daihan, S. (2005). Stage-specifically expressed schistosome proteins as potential chemotherapeutic targets. Med. Sci. Monit. 11, RA94–RA103. Available online at: http://www.medscimonit.com/abstract/index/idArt/15402
Fairbairn, D. J., Cavallaro, A. S., Bernard, M., and Mahalinga-Iyer, J. (2007). Host-delivered RNAi: an effective strategy to silence genes in plant parasitic nematodes. Planta 226, 1525–1533. doi: 10.1007/s00425-007-0588-x
Filipowicz, W. (2005). RNAi: the nuts and bolts of the RISC machine. Cell 122, 17–20. doi: 10.1016/j.cell.2005.06.023
Fogeron, M.-L. (2015). Development of a Wheat Germ Cell-Free Expression System for the Production, the Purification and the Structural and Functional Characterization of Eukaryotic Membrane Proteins : Application to the Preparation of Hepatitis C Viral Proteins. PhD thesis, Université Claude Bernard - Lyon I.
Fryer, M. E., and Collins, C. D. (2003). Model intercomparison for the uptake of organic chemicals by plants. Environ. Sci. Technol. 37, 1617–1624. doi: 10.1021/es026079k
Gheysen, G., and Vanholme, B. (2007). RNAi from plants to nematodes. Trends Biotech. 25, 89–92. doi: 10.1016/j.tibtech.2007.01.007
Groenenboom, M. A. C., and Hogeweg, P. (2008). RNA silencing can explain chlorotic infection patterns on plant leaves. BMC Syst. Biol. 2:105. doi: 10.1186/1752-0509-2-105
Guild, K., Zhang, Y., Stacy, R., Mundt, E., Benbow, S., Green, A., et al. (2011). Wheat germ cell-free expression system as a pathway to improve protein yield and solubility for the SSGCID pipeline. Acta Cryst. F67, 1027–1031. doi: 10.1107/S1744309111032143
Hadrami, A. E., Adam, L. R., Hadrami, I. E., and Daayf, F. (2010). Chitosan in plant protection. Mar. Drugs 8, 968–987. doi: 10.3390/md8040968
Hammond, S. M., Bernstein, E., Beach, D., and Hannon, G. J. (2000). An RNA-directed nuclease mediates post-transcriptional gene silencing in Drosophila cells. Nature 404, 293–296. doi: 10.1038/35005107
Han, L., and Luan, Y.-S. (2015). Horizontal transfer of small RNAs to and from plants. Front. Plant Sci. 6:1113. doi: 10.3389/fpls.2015.01113
Harbers, M. (2014). Wheat germ systems for cell-free protein expression. FEBS Lett. 588, 2762–2773. doi: 10.1016/j.febslet.2014.05.061
Heidebrecht, W. (2017). Delivery strategies: RNA interference in agriculture and human health. Pest Manage. Sci. 73, 686–691. doi: 10.1002/ps.4341
Hüttenhofer, A., Schattner, P., and Polacek, N. (2005). Non-coding RNAs: hope or hype? Trends Genet. 21, 289–297. doi: 10.1016/j.tig.2005.03.007
Iutynska, G. O. (2012). Elaboration of natural polyfunctional preparations with antiparasitic and biostimulating properties for plant growth. Mikrobiol. Z. 74, 3–12. Available online at: http://nbuv.gov.ua/UJRN/MicroBiol_2012_74_4_2
Iutynska, G. O., Biliavska, L. O., and Kozyritska, V. Y. (2017). Development strategy for the new environmentally friendly multifunctional bioformulations based on soil streptomycetes. Mikrobiol. Z 79, 22–33. doi: 10.15407/microbiolj79.01.022
Jailani, A. A. K., and Mukherjee, S. K. (2017). Does endogenous RNAi have antipathogenic features? Ind. J. Plant Physiol. 22, 506–513. doi: 10.1007/s40502-017-0336-6
Joga, M. R., Zotti, M. J., Smagghe, G., and Christiaens, O. (2016). RNAi efficiency, systemic properties, and novel delivery methods for pest insect control: what we know so far. Front. Physiol. 7:553. doi: 10.3389/fphys.2016.00553
Jung, C., and Wyss, U. (1999). New approaches to control plant parasitic nematodes. Appl. Microbiol. Biotechnol. 51, 439–446.
Kamthan, A., Chaudhuri, A., Kamthan, M., and Datta, A. (2015). Small RNAs in plants: recent development and application for crop improvement. Front. Plant Sci., 6:208. doi: 10.3389/fpls.2015.00208
Kamthan, A., Kamthan, M., Chakraborty, M., Chakraborty, S., and Datta, A. (2012). A simple protocol for extraction, derivatization, and analysis of tomato leaf and fruit lipophilic metabolites using gc-ms. Protocol Exchange 2012. doi: 10.1038/protex.2012.061
Kanakala, S., and Ghanim, M. (2016). RNA interference in insect vectors for plant viruses. Viruses 8:329. doi: 10.3390/v8120329
Katzen, F., Chang, G., and Kudlicki, W. (2005). The past, present and future of cell-free protein synthesis. Trends Biotech. 23, 150–156. doi: 10.1016/j.tibtech.2005.01.003
Kaur, T., Jasrotia, S., Ohri, P., and Manhas, R. K. (2016). Evaluation of in vitro and in vivo nematicidal potential of a multifunctional streptomycete, Streptomyces hydrogenans strain DH16 against Meloidogyne incognita. Microbiol. Res. 192, 247–252. doi: 10.1016/j.micres.2016.07.009
Klink, V. P., Kim, K. H., Martins, V., Macdonald, M. H., Beard, H. S., Alkharouf, N. W., et al. (2009). A correlation between host-mediated expression of parasite genes as tandem inverted repeats and abrogation of development of female Heterodera glycines cyst formation during infection of Glycine max. Planta 230, 53–71. doi: 10.1007/s00425-009-0926-2
Knip, M., Constantin, M. E., and Thordal-Christensen, H. (2014). Trans-kingdom cross-talk: small RNAs on the move. PLoS Genet. 10:e1004602. doi: 10.1371/journal.pgen.1004602
Koch, A., and Kogel, K. (2014). New wind in the sails: improving the agronomic value of crop plants through RNAi-mediated gene silencing. Plant Biotech. J. 12, 821–831. doi: 10.1111/pbi.12226
Kong, L., Wu, D.-Q., Huang, W.-K., Peng, H., Wang, G.-F., Cui, J.-K., et al. (2015). Large-scale identification of wheat genes resistant to cereal cyst nematode Heterodera avenae using comparative transcriptomic analysis. BMC Genomics 16:801. doi: 10.1186/s12864-015-2037-8
Kumar, M., Gantasala, N. P., Roychowdhury, T., Thakur, P. K., Banakar, P., Shukla, R. N., et al. (2014). De novo transcriptome sequencing and analysis of the cereal cyst nematode, Heterodera avenae. PLoS ONE 9:e96311. doi: 10.1371/journal.pone.0096311
Li, H., Guan, R., Guo, H., and Miao, X. (2015). New insights into an RNAi approach for plant defence against piercing-sucking and stem-borer insect pests. Plant Cell Environ. 38, 2277–2285. doi: 10.1111/pce.12546
Li, J., Todd, T. C., and Trick, H. N. (2010). Rapid in planta evaluation of root transgenes in chimeric soybean plants. Plant Cell Rep. 29, 113–123. doi: 10.1007/s00299-009-0803-2
Li, R., Rashotte, A. M., Singh, N. K., Weaver, D. B., Lawrence, K. S., and Locy, R. D. (2014). Integrated signaling networks in plant responses to sedentary endoparasitic nematodes: a perspective. Plant Cell Rep. 34, 1–18. doi: 10.1007/s00299-014-1676-6
Lilley, C. J., Davies, L. J., and Urwin, P. E. (2012). RNA interference in plant parasitic nematodes: a summary of the current status. Parasitology 139, 630–640. doi: 10.1017/S0031182011002071
Lipardi, C., Wei, Q., and Paterson, B. M. (2001). RNAi as random degradative PCR: siRNA primers convert mRNA into dsRNAs that are degraded to generate new siRNAs. Cell 107, 297–307. doi: 10.1016/S0092-8674(01)00537-2
Liu, J., Peng, H., Cui, J., Huang, W., Kong, L., Clarke, J. L., et al. (2016). Molecular characterization of a novel effector expansin-like protein from Heterodera avenae that induces cell death in Nicotiana benthamiana. Sci. Rep. 6:35677. doi: 10.1038/srep35677
Liu, X., Hao, L., Li, D., Zhu, L., and Hu, S. (2015). Long non-coding RNAs and their biological roles in plants. Genomics Proteomics Bioinformatics 13, 137–147. doi: 10.1016/j.gpb.2015.02.003
Locker, J. (1979). Analytical and preparative electrophoresis of RNA in agarose-urea. Anal. Biochem. 98, 358–367.
Mackereth, C. D., Madl, T., Bonnal, S., Simon, B., Zanier, K., Gasch, A., et al. (2011). Multi-domain conformational selection underlies pre-mRNA splicing regulation by U2AF. Nature 475, 358–367. doi: 10.1038/nature10171
Majumdar, R., Rajasekaran, K., and Cary, J. W. (2017). RNA interference (RNAi) as a potential tool for control of mycotoxin contamination in crop plants: concepts and considerations. Front. Plant Sci. 8:200. doi: 10.3389/fpls.2017.00200
Makeev, E. V., and Bamford, D. H. (2002). Cellular RNA-dependent RNA polymerase involved in posttranscriptional gene silencing has two distinct activity modes. Mol. Cell 10, 1417–1427. doi: 10.1016/S1097-2765(02)00780-3
Malerba, M., and Cerana, R. (2016). Chitosan effects on plant systems. Int. J. Mol. Sci. 17:996. doi: 10.3390/ijms17070996
Marcus, A., Efron, D., and Weeks, D. P. (1974). The wheat embryo cell-free system. Methods Enzymol. 30, 749–754.
Mathonnet, G., Fabian, M. R., Svitkin, Y. V., Parsyan, A., Huck, L., Murata, T., et al. (2007). MicroRNA inhibition of translation initiation in vitro by targeting the cap-binding complex eIF4F. Science 317, 1764–1767. doi: 10.1126/science.1146067
Maule, A. G., McVeigh, P., Dalzell, J. J., Atkinson, L., Mousley, A., and Marks, N. J. (2011). An eye on RNAi in nematode parasites. Trends Parasitol. 27, 505–513. doi: 10.1016/j.pt.2011.07.004
Mitter, N., Worrall, E. A., Robinson, K. E., Li, P., Jain, R. G., Taochy, C., et al. (2017). Clay nanosheets for topical delivery of RNAi for sustained protection against plant viruses. Nat. Plants 3:16207. doi: 10.1038/nplants.2016.207
Mmeka, E. C., Adesoye, A., Ubaoji, K. I., and Nwokoye, A. B. (2014). Gene silencing technologies in creating resistance to plant diseases. Int. J. Plant Breed. Genet. 8, 100–120. doi: 10.3923/ijpbg.2014.100.120
Nardi, S., Pizzeghello, D., Schiavon, M., and Erthani, A. (2016). Plant biostimulants: physiological responses induced by protein hydrolyzed-based products and humic substances in plant metabolism. Sci. Agric. 73, 18–23. doi: 10.1590/0103-9016-2015-0006
Negretskii, V. A. (1988). Methodical Recommendations for Determination of Phytohormones. Kiev: Inst. Bot. Akad. Nauk UkrSSR.
Neofytou, G., Kyrychko, Y. N., and Blyuss, K. B. (2016a). Mathematical model of plant-virus interactions mediated by RNA interference. J. Theor. Biol. 403, 129–142. doi: 10.1016/j.jtbi.2016.05.018
Neofytou, G., Kyrychko, Y. N., and Blyuss, K. B. (2016b). Time-delayed model of immune response in plants. J. Theor. Biol. 389, 28–39. doi: 10.1016/j.jtbi.2015.10.020
Neofytou, G., Kyrychko, Y. N., and Blyuss, K. B. (2017). Time-delayed model of RNA interference. Ecol. Complex. 30, 11–25. doi: 10.1016/j.ecocom.2016.12.003
Nicol, J. M., Elekçioğlu, I. H., Bolat, N., and Rivoal, R. (2007). The global importance of the cereal cyst nematode (Heterodera spp.) on wheat and international approaches to its control. Comm. Agric. Appl. Biol. Sci. 72, 677–686. Available online at: https://www.tib.eu/en/search/id/BLSE%3ARN225133668/
Nicol, J. M., and Rivoal, R. (2008). “Global knowledge and its application for the integrated control and management of nematodes on wheat,” in Integrated Management and Biocontrol of Vegetable and Grain Crops Nematodes, eds A. Ciancio and K. G. Mukerji (Dordrecht: Springer), 251–294.
Nunes, C. C., and Dean, R. A. (2012). Host-induced gene silencing: a tool for understanding fungal host interactions and for developing novel disease control strategies. Mol. Plant Pathol. 13, 519–529. doi: 10.1111/j.1364-3703.2011.00766.x
Peng, D. L., Nicol, J. M., Li, H. M., Hou, S. Y., Li, H. X., Chen, S. L., et al. (2009). “Current knowledge of cereal cyst nematode (Heterodera avenae) on wheat in China,” in Cereal cyst nematodes: status, research and outlook, eds I. T. Riley, J. M. Nicol, and A. A. Dababat (Ankara: CIMMYT), 29–34.
Ponomarenko, S. P., and Iutynska, G. O. (2011). New Plant Growth Regulators: Basic Research and Technologies of Application. Kyiv: Nichlava.
Putter, I., Connell, J. G. M., Preiser, F. A., Haidri, A. A., Ristich, S. S., and Dybas, R. A. (1981). Avermectins: novel insecticides, acaricides and nematicides from a soil microorganism. Experientia 37, 963–964.
Rao, N. K., Hanson, J., Dulloo, M. E., Nowell, K. G., and Larinde, M. (2006). Manual of Seed Handling in Genebanks. Handbooks for Genebanks No. 8. Rome: Biodiversity Internation.
Rashad, F. M., Fathy, H. M., El-Zayata, A. S., and Elghonaimy, A. M. (2015). Isolation and characterization of multifunctional Streptomyces species with antimicrobial, nematicidal and phytohormone activities from marine environments in Egypt. Microbiol. Res. 175, 34–47. doi: 10.1016/j.micres.2015.03.002
Rehman, S., Gupta, V. K., and Goyal, A. K. (2016). Identification and functional analysis of secreted effectors from phytoparasitic nematodes. BMC Microbiol. 16:48. doi: 10.1186/s12866-016-0632-8
Rein, A., Legind, C. N., and Trapp, S. (2011). New concepts for dynamics plant uptake models. SAR QSAR Environ. Res. 22, 191–215. doi: 10.1080/1062936X.2010.548829
Roberts, B. E., and Paterson, B. M. (1973). Efficient translation of tobacco mosaic virus RNA and rabbit Globin 9S RNA in a cell-free system from commercial wheat germ. Proc. Natl. Acad. Sci. U.S.A. 70, 2330–2334.
Roy, S. M., Bajad, S., Green, E., and Heinsohn, H. (2011). Method for Enhancing Recombinant Protein Production by Cell-Free Protein Expression System. US9040253B2. Available online at: https://patents.google.com/patent/US9040253B2/en
Ruanpanun, P., Tangchitsomkid, N., Hyde, K. D., and Lumyong, S. (2010). Actinomycetes and fungi isolated from plant-parasitic nematode infested soils: screening of the effective biocontrol potential, indole-3-acetic acid and siderophore production. World J. Microbiol. Biotechnol. 26, 1569–1578. doi: 10.1007/s11274-010-0332-8
Ruiu, L. (2015). Insect pathogenic bacteria in integrated pest management. Insects 6, 352–367. doi: 10.3390/insects6020352
Safari, E., Gororo, N. N., Eastwood, R. F., Lewis, J., Eagles, H. A., and Ogbonnaya, F. C. (2005). Impact of Cre1, Cre8 and Cre3 genes on cereal cyst nematode resistance in wheat. Theor. Appl. Genet. 110, 567–572. doi: 10.1007/s00122-004-1873-8
Sambrook, J., Fritsch, E. F., and Maniatis, T. (1989). Molecular Cloning: A Laboratory Manual. New York, NY: Cold Spring Harbor Laboratory Press.
Sarkies, P., and Miska, E. A. (2014). Small RNS break out: the molecular cell biology of mobile small RNAs. Nat. Genet. 15, 525–572. doi: 10.1038/nrm3840
Saurabh, S., Vidyarthi, A. S., and Prasad, D. (2014). RNA interference: concept to reality in crop improvement. Planta 239, 543–564. doi: 10.1007/s00425-013-2019-5
Savinskiy, S. V., Drogovoz, I. V., and Pedchenko, V. K. (1991). Determination of zeatin, indole-3-acetic and abscisic acids in one plant sample by high performance liquid chromatography. Physiol. Biochem. Cult. Plants 23, 611–619.
Scharpf, R. F. (1970). Seed Viability, Germination and Radicle Growth of Dwarf Mistletoe in California. USDA Paper PSW-59. Berkeley, CA: USDA; Forest Service Research.
Shaban, M. (2013). Study on some aspects of seed viability and vigor. Int. J. Adv. Biol. Biomed. Res. 1, 1692–1697. Available online at: http://www.ijabbr.com/article_7964.html
Shyu, A.-B., Wilkinson, M. F., and van Hoof, A. (2008). Messenger RNA regulation: to translate or to degrade. EMBO J. 27, 471–481. doi: 10.1038/sj.emboj.7601977
Singh, H., Makino, S., Endo, Y., Li, Y., Stephens, A. N., and Nie, G. (2012). Application of the wheat-germ cell-free translation system to produce high temperature requirement A3 (HtrA3) proteases. Biotechniques 52, 23–28. doi: 10.2144/000113798
Smiley, R. W., and Nicol, J. M. (2009). “Nematodes which challenge global wheat production,” inWheat Science and Trade, ed B. F. Carver (Ames, IA: Wiley-Blackwell), 171–187.
Stergios, B. G., and Howell, G. S. (1973). Evaluation of viability tests for cold stressed plants. J. Am. Soc. Hortic. Sci. 98, 325–330.
Takai, K., Sawasaki, T., and Endo, Y. (2010). Practical cell-free protein synthesis system using purified wheat embryos. Nat. Protoc. 5, 227–238. doi: 10.1038/nprot.2009.207
Tamilarasan, S., and Rajam, M. V. (2013). Engineering crop plants for nematode resistance through host-derived RNA interference. Cell Dev. Biol. 2:1000114. doi: 10.4172/2168-9296.1000114
Thomason, I. A. (1987). “Challenges facing nematology: Environmental risks with nematicides and the need for new approaches,” in Vistas on Nematology, eds J. A. Weech and D. W. Dickson (Hyattsville, MD: Society of Nematologists), 469–476.
Tishler, P. V., and Epstein, C. J. (1968). A convenient method of preparing polyacrylamide gels for liquid scintillation spectrometry. Anal. Biochem. 22, 89–98.
Tsygankova, V. A., Andrusevich, Y., Ponomarenko, S. P., Galkin, A. P., and Blume, Y. B. (2012a). Isolation and amplification of cDNA from the conserved region of the nematode Heterodera schachtii 8H07 gene with a close similarity to its homolog in rape plants. Cytol. Genet. 46, 335–341. doi: 10.3103/S0095452712060114
Tsygankova, V. A., Andrusevich, Y. V., Galkin, A. P., Biliavska, L. O., Galagan, T. O., Yemets, A. I., et al. (2019). RNAi-mediated resistance against plant parasitic nematodes of wheat plants obtained in vitro using bioregulators of microbiological origin. Curr. Chem. Biol. 13, 73–89. doi: 10.2174/2212796812666180507130017
Tsygankova, V. A., Biliavska, L. O., Andrusevich, Y. V., Bondarenko, O. N., Galkin, A. P., Babich, O. A., et al. (2014a). Impact of new microbial PR/PGW inducers on increase of resistance to parasitic nematode of wild and RNAi transgenic rape plants. Adv. Biosci. Bioeng. 2, 66–103. Available online at: https://www.infinitypress.info/index.php/abb/article/view/887/405
Tsygankova, V. A., Iutynska, G. A., Galkin, A. P., and Blume, Y. (2014b). Impact of new natural biostimulants on increasing synthesis in plant cells of small regulatory si/miRNA with high anti-nematodic activity. Int. J. Biol. 6, 48–64. doi: 10.5539/ijb.v6n1p48
Tsygankova, V. A., Musatenko, L. I., Ponomarenko, S. P., Galkina, L. O., Andrusevich, Ja. V., and Galkin, A. P. (2010). Change of functionally active cytoplasmic mRNA populations in plant cells under growth regulators action and biological perspectives of cell-free systems of protein synthesis. Biotekhnologiya 3, 19–32.
Tsygankova, V. A., Shysha, E., Andrusevich, Y., Galkin, A., Iutynska, G., Yemets, A., et al. (2016). Using of new microbial biostimulants for obtaining in vitro new lines of Triticum aestivum L. cells resistant to nematode H. avenae. Eur. J. Biotech. Biosci. 4, 39–53. Available online at: http://www.biosciencejournals.com/archives/2016/vol4/issue4/4-4-26.1
Tsygankova, V. A., Shysha, E., Galkin, A. P., Biliavska, L., Iutynska, G., Yemets, A., et al. (2017). Impact of microbial biostimulants on induction of callusogenesis and organogenesis in the isolated tissue culture of wheat in vitro. J. Med. Plant Stud. 5, 155–164. Available online at: http://www.plantsjournal.com/archives/?year=2017&vol=5&issue=3&part=C&ArticleId=614
Tsygankova, V. A., Stefanovska, T. R., Galkin, A. P., Ponomarenko, S. P., and Blume, Y. B. (2012b). Inducing effect of PGRs on small regulatory si/miRNA in resistance to sugar beet cyst nematode. Comm. Appl. Biol. Sci. 77, 779–788.
Tsygankova, V. A., Yemets, A. I., Iutynska, H. O., Beljavska, L. O., Galkin, A. P., and Blume, Y. (2013). Increasing the resistance of rape plants to the parasitic nematode Heterodera schachtii using RNAi technology. Cytol. Genet. 47, 222–230. doi: 10.3103/S0095452713040105
Tsygankova, V. A., Zayets, N. N., Galkina, L. A., Prikazchikova, L. P., and Blume, Y. B. (1998). An unusual minor protein appearing in embryonic axis cells of haricot bean seeds following germination process stimulated by 6-methylthiouracil. Biopolym. Cell 14, 438–448.
Turner, M. J., and Schaeffer, J. M. (1989). “Mode of action of ivermectin,” in Ivermectin and Abamectin, ed W. C. Cambell (New York, NY: Springer Verlag), 73–88.
Tuschl, T., Zamore, P. D., Lehmann, R., Bartel, D. P., and Sharp, P. A. (1999). Targeted mRNA degradation by double-stranded RNA in vitro. Genes Dev. 13, 3191–3197.
Urwin, P. E., Lilley, C. J., and Atkinson, H. J. (2002). Ingestion of double-stranded RNA by preparasitic juvenile cyst nematodes leads to RNA interference. Mol. Plant Microbe Interact. 15, 747–752. doi: 10.1094/MPMI.2002.15.8.747
Vanstone, V. A., Rathjen, A. J., Ware, A. H., and Wheeler, R. D. (1998). Relationship between root lesion nematodes (Pratylenchus neglectus and P. thornei) and performance of wheat varieties. Austr. J. Exp. Agric. 38, 181–188.
von Fircks, H. A., and Verwijst, R. (1993). Plant viability as a function of temperature stress. Plant Physiol. 103, 125–130.
Waadt, R., Hsu, P.-K., and Schroeder, J. I. (2015). Abscisic acid and other plant hormones: methods to visualize distribution and signaling. Bioessays 37, 1338–1349. doi: 10.1002/bies.201500115
Wakiyama, M., Takimoto, K., Ohara, O., and Yokohama, S. (2007). Let-7 microRNA-mediated mRNA deadenylation and translational repression in a mammalian cell-free system. Genes Dev. 21, 1857–1862. doi: 10.1101/gad.1566707
Wallace, A., Filbin, M. E., Veo, B., McFarland, C., Stepinski, J., Jankowska-Anyszka, M., et al. (2010). The nematode eukaryotic translation initiation factor 4E/G complex works with a trans-spliced leader stem-loop to enable efficient translation of trimethylguanosine-capped RNAs. Mol. Cell. Biol. 30, 1958–1970. doi: 10.1128/MCB.01437-09
Wang, F., Polydore, S., and Axtell, M. J. (2015). More than meets the eye? Factors that affect target selection by plant miRNAs and heterochromatic siRNAs. Curr. Opin. Plant Biol. 27, 118–124. doi: 10.1016/j.pbi.2015.06.012
Wassenegger, M., and Pelissier, T. (1998). A model for RNA-mediated gene silencing in higher plants. Plant Mol. Biol. 37, 349–362.
Wolin, S. L., and Walter, P. (1988). Ribosome pausing and stacking during translation of a eukaryotic mRNA. EMBO J. 7, 3559–3569.
Xu, L., Tang, Y., Gao, S., Su, S., Hong, L., Wang, W., et al. (2016). Comprehensive analyses of the annexin gene family in wheat. BMC Genomics 17:415. doi: 10.1186/s12864-016-2750-y
Yadav, B. C., Veluthambi, K., and Subramaniam, K. (2006). Host-generated double stranded RNA induces RNAi in plant-parasitic nematodes and protects the host from infection. Mol. Biochem. Parasitol. 148, 219–222. doi: 10.1016/j.molbiopara.2006.03.013
Yakhin, O. I., Lubyanov, A. A., Yakhin, I. A., and Brown, P. H. (2017). Biostimulants in plant science: a global perspective. Front. Plant Sci. 7:2049. doi: 10.3389/fpls.2016.02049
Yang, D., Chen, C., Liu, Q., and Kian, H. (2017). Comparative analysis of pre- and post-parasitic transcriptomes and mining pioneer effectors of Heterodera avenae. Cell Biosci. 7:11. doi: 10.1186/s13578-017-0138-6
Yang, X., Coulombe-Huntington, J., Kang, S., Sheynkman, G. M., Hao, T., Richardson, A., et al. (2016). Widespread expansion of protein interaction capabilities by alternative splicing. Cell 164, 805–817. doi: 10.1016/j.cell.2016.01.029
Ye, C. Y., Xu, H., Shen, E., Liu, Y., Wang, Y., Shen, Y., et al. (2014). Genome-wide identification of non-coding RNAs interacted with microRNAs in soybean. Front. Plant Sci. 5:743. doi: 10.3389/fpls.2014.00743
Zhang, J., Khan, S. A., Hasse, C., Ruf, S., Heckel, D. G., and Bock, R. (2015). Full crop protection from an insect pest by expression of long double-stranded RNAs in plastids. Science 347, 991–994. doi: 10.1126/science.1261680
Zheng, M., Long, H., Zhao, Y., Li, L., Xu, D., Zhang, H., et al. (2015). RNA-Seq based identification of candidate parasitism genes of cereal cyst nematode (Heterodera avenae) during incompatible infection to Aegilops variabilis. PLoS ONE 10:e0141095. doi: 10.1371/journal.pone.0141095
Zhou, G., Zhou, Y., and Chen, X. (2017). New insight into inter-kingdom communication: horizontal transfer of mobile small RNAs. Front. Microbiol. 8:768. doi: 10.3389/fmicb.2017.00768
Keywords: crop protection against nematodes, streptomycete-derived biostimulants, RNA interference, wheat (Triticum aestivum L.), cereal cyst nematode (Heterodera avenae)
Citation: Blyuss KB, Fatehi F, Tsygankova VA, Biliavska LO, Iutynska GO, Yemets AI and Blume YB (2019) RNAi-Based Biocontrol of Wheat Nematodes Using Natural Poly-Component Biostimulants. Front. Plant Sci. 10:483. doi: 10.3389/fpls.2019.00483
Received: 21 October 2018; Accepted: 28 March 2019;
Published: 17 April 2019.
Edited by:
Goetz Hensel, Leibniz-Institut für Pflanzengenetik und Kulturpflanzenforschung (IPK), GermanyReviewed by:
Matthew R. Willmann, Cornell University, United StatesHuipeng Pan, South China Agricultural University, China
Copyright © 2019 Blyuss, Fatehi, Tsygankova, Biliavska, Iutynska, Yemets and Blume. This is an open-access article distributed under the terms of the Creative Commons Attribution License (CC BY). The use, distribution or reproduction in other forums is permitted, provided the original author(s) and the copyright owner(s) are credited and that the original publication in this journal is cited, in accordance with accepted academic practice. No use, distribution or reproduction is permitted which does not comply with these terms.
*Correspondence: Konstantin B. Blyuss, ay5ibHl1c3NAc3Vzc2V4LmFjLnVr