- 1Biotechnology Research Institute, Chinese Academy of Agricultural Sciences, Beijing, China
- 2National Key Facility for Crop Gene Resources and Genetic Improvement, Beijing, China
Salt stress causes retarded plant growth and reduced crop yield. A complicated regulation network to response to salt stress has been evolved in plants under high salinity conditions. Ethylene is one of the most important phytohormones, playing a major role in salt stress response. An increasing number of studies have demonstrated that ethylene modulates salt tolerance through reactive oxygen species (ROS) homeostasis. Ascorbic acid (AsA) is a non-enzymatic antioxidant, contributing to ROS-scavenging and salt tolerance. Here, we mainly focus on the advances in understanding the modulation of ethylene and AsA on ROS-scavenging under salinity stress. We also review the regulators involved in the ethylene signaling pathway and AsA biosynthesis that respond to salt stress. Moreover, the AsA pool is affected by many environmental conditions, and the potential role of ethylene in AsA production is also extensively discussed. Novel insights into the roles and mechanisms of ethylene in AsA-mediated ROS homeostasis will provide critical information for improving crop salt tolerance.
Introduction
According to the report from the Food and Agricultural Organization of the United Nations, there will be challenges related to the productivity of crops to supply more food for an additional 2.3 billion people by 2050. Crop yield is greatly affected by various abiotic stresses like drought, high salinity, cold, and heat (Zhu, 2016). For instance, high salinity stress disturbs plant physiological processes through osmotic stress and ionic toxicity, causing reductions in both crop growth and yield (Yang and Guo, 2018a,b). For better utilization of salt-affected lands, it is of great help to develop crops with improved salt tolerance through molecular-assistant breeding to reveal the underlying mechanisms involved in plant response to salt stress.
Salt stress responses generally correlate with the regulations of phytohormones, including abscisic acid (ABA), jasmonic acid, gibberellin, and ethylene (Julkowska and Testerink, 2015). Although there exists opposite modulation between monocot and dicot plants (Peng et al., 2014; Yang et al., 2015), increasing investigations have revealed that ethylene-conferred salt tolerance is mediated by deterring reactive oxygen species (ROS) homeostasis (Jiang et al., 2013; Li et al., 2014; Peng et al., 2014; Yang and Guo, 2018b). Under salt stress, ROS, including hydrogen peroxide, superoxide anions and hydroxyl radicals, accumulate and damage cellular structure (Ahanger et al., 2017). ROS plays a dual role in response to stresses as toxic by products and major signal (Qi et al., 2018), and the excess ROS could be scavenged through enzymatic and non-enzymatic antioxidant defense systems (You and Chan, 2015). Accumulating investigations have revealed that ascorbic acid (AsA) is an essential compound of non-enzymatic antioxidant in plants, functioning in plant growth, hormone signaling, and stress response (Bulley and Laing, 2016; Mellidou and Kanellis, 2017; Vidal-Meireles et al., 2017). AsA plays especially critical roles in the fine control of ROS homeostasis to improve salt tolerance (Smirnoff, 2000; Shalata and Neumann, 2001; Zhang et al., 2012; Wang J. et al., 2013), implying that AsA has an essential modulation in salt response. Considering there are some reviews about ethylene-modulated salt response (Zhang M. et al., 2016), this mini review will focus on the advances in understanding the modulation of ethylene on AsA biosynthesis and ROS-scavenging under salinity stress.
The Regulation of Ethylene on ROS Homeostasis Is Tightly Associated With the Plant Response to Salt Stress
Gaseous phytohormone ethylene plays an important role in mediating numerous specific growth and development processes (Wang et al., 2018), especially in response to various stress conditions (Wang F. et al., 2013; Dubois et al., 2018). The biosynthesis and signaling pathway of ethylene have been well established (Guo and Ecker, 2004). After recognition of ethylene by endoplasmic reticulum membrane-associated receptors, the interaction of ethylene receptors with CONSTITUTIVE TRIPLE RESPONSE1 (CTR1) will be released, and the phosphorylation of CTR1 on ETHYLENE INSENSITIVE 2 (EIN2) will be liberated. Then, the C-terminal of EIN2 is generated by an unknown mechanism and is transported to cytoplasmic processing-body (P-body) to repress translation of EIN3 BINDING F-BOX1/2 (EBF1/2), which mediates the proteasomal degradation of EIN3 and EIN3-LIKE 1 (EIL1), resulting in the stability of EIN3/EIL1 proteins and promotion of ethylene response (Li et al., 2015). APETALA2/ETHYLENE RESPONSE FACTORS (AP2/ERFs) are one of the most important transcription factor families, regulating multiple developmental and stress response processes (Phukan et al., 2017), most of which are downstream targets of ethylene signaling (Liu et al., 2016).
Ethylene has long been known for modulating salt stress response (Cao et al., 2007). For instance, blocked ethylene signaling confers reduced salt tolerance to Arabidopsis (Achard et al., 2006; Peng et al., 2014). ein3 eil1 double mutants and other ethylene signaling-related mutants showed enhanced sensitivity to salt stress. In contrast to this modulation, ethylene displays a negative role in rice (Yang et al., 2015). OsEIL1 and OsEIL2 RNAi transgenic plants displayed increased salt tolerance. The regulation of ethylene biosynthesis also plays different roles in salt tolerance between Arabidopsis and rice (Jiang et al., 2013; Li et al., 2014). Ethylene Overproducer 1 (ETO1) plays a positive role in salt response through promoting ROS generation, followed with Na+/K+ homeostasis modulation in Arabidopsis. However, SALT INTOLERANCE 1 (SIT1) negatively regulates salt response due to activation on MITOGEN-ACTIVATED PROTEIN KINASE 3/6 (MPK3/6) in rice, which promotes ethylene and ROS overproduction (Table 1). Thus, the different mechanisms of ethylene-directed salt response between monocot and dicot plants remain in need of research. Subsequent advances indicate that ROS homeostasis is essential for ethylene regulation of plant growth and stress response (Steffens, 2014; Zhong et al., 2014; Yang et al., 2017). ROS is a double-edged sword during salt stress response. On the one hand, ROS act as important signal molecules to activate downstream metabolic pathways. Previous studies demonstrate that ROS burst via RESPIRATORY BURST OXIDASE HOMOLOG D (RbohD) and RbohF is essential for the Na+/K+ homeostasis in Arabidopsis (Ma et al., 2012), and ethylene-induced ROS production through transcriptional regulation on AtRbohF confers enhanced salt tolerance to the ethylene overproduced mutant eto1 (Jiang et al., 2013). On the other hand, ethylene signaling component EIN3/EIL1 activates ROS-scavenging gene expression to deter excess ROS accumulation and to increase salt tolerance (Peng et al., 2014). Similarly, the effects of ethylene signaling downstream factors on ROS are inconsistent during different stages of various stresses. For example, ERF74 promotes ROS burst in the early stages of various stresses through the regulation of gene expression of RbohD, followed with induction of ROS-scavenging-related genes (Yao et al., 2017). However, ethylene inducible factor TERF1 improves stress tolerance through reduced ROS content (Zhang H. et al., 2016). Therefore, fine-tuning of ethylene biosynthesis and signaling on ROS homeostasis are critical for salt tolerance.
Additionally, our previous studies verified several downstream regulators of ethylene signaling in salt response and ROS homeostasis. For example, ETHYLENE AND SALT INDUCIBLE ERF GENE 1 (ESE1), a direct target gene of EIN3, positively regulates salt tolerance and coordinates with EIN3 to activate downstream salt-related gene expression in Arabidopsis (Zhang et al., 2011); and JERF3, an ethylene-induced gene, enhances salt tolerance via direct modulation on the gene expressions of SUPEROXIDE DISMUTASE (SOD) and CARBONIC ANHYDRASE (CA) in tomato to eliminate ROS, which also confers drought and osmotic stress tolerance to transgenic rice with heterologous expression of JERF3 (Wu et al., 2008; Zhang et al., 2010; Table 1). Thus, identification of more ethylene signaling downstream regulators participating in ROS homeostasis under salt stress is necessary for elucidating the regulation of ethylene on ROS and salt response.
The Scavenging Role of AsA on ROS Homeostasis Contributes to Salt Tolerance
AsA, also known as vitamin C, is a low molecular weight antioxidant, functioning as a component of non-enzymatic scavenging of ROS in plant growth and stress tolerance (Smirnoff, 2000; Conklin, 2004; Akram et al., 2017). It has been reported that AsA improves salt tolerance in various species, including rice, potato, tomato, and citrus (Shalata and Neumann, 2001; Hemavathi et al., 2010; Kostopoulou et al., 2015; Qin et al., 2016). The L-galactose pathway is the main pathway of AsA biosynthesis in plants, and most of the genes in this pathway have been identified (Bulley and Laing, 2016). Investigations also have elucidated the regulation via the L-galactose pathway of AsA biosynthesis, including the modulations on the AsA biosynthesis enzyme activities and stabilities at transcriptional and translational levels (Laing et al., 2015). One of these regulators is calmodulins-like 10, which interacts with AsA biosynthesis enzyme phosphomannomutase (PMM) to modulate enzyme activities and AsA pool (Cho et al., 2016), which suggested the role of calcium (Ca2+) in AsA biosynthesis. It has been known that Ca2+ signaling is triggered by ROS accumulation (Rentel and Knight, 2004) and Ca2+ wave is induced under salt stress (Choi et al., 2014; Liu et al., 2018). A chloroplast protein, QUASIMODO1 (QUA1), functions upstream of a thylakoid-localized Ca2+ sensor, CAS, to mediate Ca2+ signaling under salt stress (Zheng et al., 2017). Additionally, AsA could trigger increase of cytosolic Ca2+ in Arabidopsis as a signaling molecule (Makavitskaya et al., 2018), suggesting the association between Ca2+ sensor and AsA-mediated ROS scavenging during salt responses, and feedback regulation of Ca2+ signaling and ROS homeostasis.
The regulation factors of AsA biosynthesis also play a role in salt response. Our previous investigations have found that ethylene-induced factor AtERF98 enhances salt tolerance due to transcriptional activation on gene expressions of AsA biosynthesis enzymes, especially direct binding to the promoter of a key enzyme of AsA biosynthesis encoding gene VTC1 (Zhang et al., 2012). Meanwhile, we also identified the post-transcriptional modulation of COP9 SIGNALOSOME SUBUNIT 5B (CSN5B) on VTC1 in Arabidopsis (Wang J. et al., 2013; Li et al., 2016), elucidating a mechanism of light/dark effects on AsA contents. Loss-of-function mutant csn5b, with more AsA content and less ROS pool, displays increased salt tolerance, suggesting the positive regulation of AsA on salt response. Recent studies showed that salt induced zinc-finger protein SIZF3, which interferes with the interaction between CSN5B and VTC1, simultaneously promotes AsA accumulation and enhances salt tolerance (Li et al., 2018; Table 1). Thus, increasing AsA content is a potential approach for improving plant salt tolerance.
The Integration of Ethylene in AsA Production Finetunes ROS Homeostasis Under Salt Stress
As discussed above, both ethylene and AsA could enhance salt tolerance via regulation of ROS homeostasis. Previous reports have indicated that ethylene in many cases maintains a low level of ROS contents under salt stress through the enzymatic pathway (Wu et al., 2008; Peng et al., 2014; Zhang W. et al., 2016). Moreover, the non-enzymatic pathway of scavenging ROS also participates in ethylene-mediated salt response, such as AtERF98, suggesting that the modulation of ethylene on ROS elimination is alternatively dependent on non-enzymatic antioxidant (Zhang et al., 2012).
There are many environmental factors affecting AsA biosynthesis, such as light (Fukunaga et al., 2010), circadian rhythm (Dowdle et al., 2007), and high temperature (Richardson, 2004). CSN5B, identified in our previous studies (Wang J. et al., 2013), is a subunit of photomorphogenic COP9 signalosome (Gusmaroli et al., 2004), which acts together with COP1, COP10, and DET1 to repress photomorphogenesis (Yanagawa et al., 2004). This research suggest that CSN5B-regulated AsA biosynthesis is a part of photomorphogenesis. Intriguingly, ethylene has functions in COP1 nucleocytoplasmic partitioning (Yu et al., 2013, 2016), indicating a possible link between ethylene and light-regulated AsA biosynthesis. It was reported that ABA-INSENSITIVE 4 (ABI4) mediates AsA-regulated plant growth (Kerchev et al., 2011) and ethylene production via transcriptional repression of ACS in Arabidopsis (Dong et al., 2016). In this regard, ethylene seems to have crosstalk with ABA to modulate AsA production (Figure 1). However, the mechanisms for these modulations are yet to be elucidated.
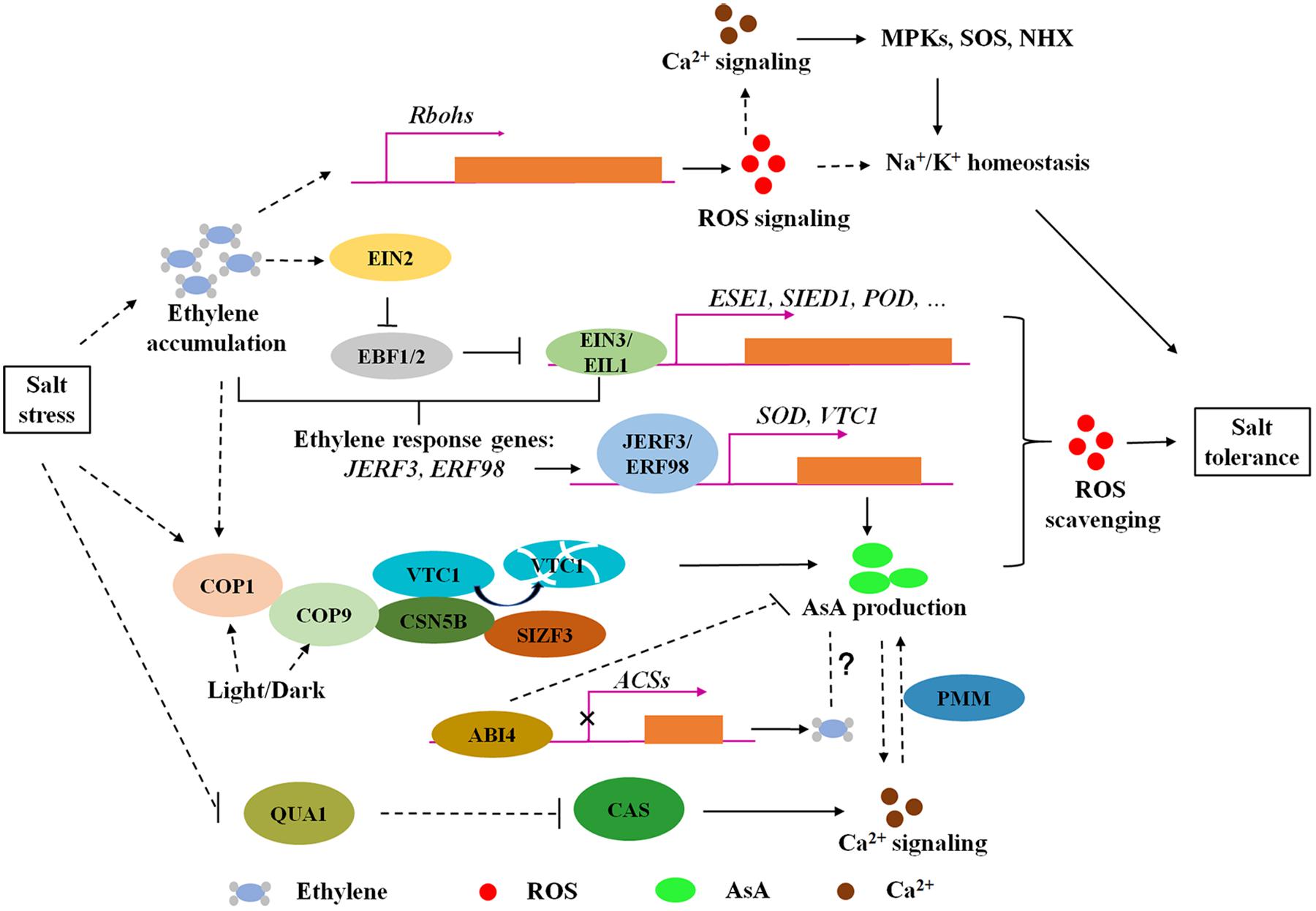
Figure 1. The modulation of ethylene signaling and AsA biosynthesis regulators on ROS homeostasis in response to salt stress. Ethylene is accumulated and plays dual roles in ROS homeostasis under salt stress. On one hand, ethylene promotes ROS production to active Na+ and K+ transport through upregulating Rbohs gene expression. In the other hand, salt stress enhances EBF1/EBF2 degradation through EIN2C-dependant translational regulation to increase EIN3/EIL1 protein levels, activating gene expression of EIN3 direct binding targets (ESE1, SIED1, and POD) and ethylene response factors (JERF3 and ERF98) to regulate salt tolerance via ROS scavenging. ERF98 positively regulates salt tolerance via transcriptional activation of AsA biosynthesis gene VTC1. Moreover, CSN5B, a subunit of photomorphogenic COP9 signalosome, contributes to AsA biosynthesis and salt responses due to modulation on VTC1 degradation. SIZF3 also confers salt tolerance through mediating the interaction between CSN5B and VTC1. This research indicates that ROS accumulation under salt stress could be eliminated through enzymatic and non-enzymatic pathways, in both of which ethylene signaling is involved. However, the understanding of ethylene roles in AsA biosynthesis is yet limited. ABI4 negatively regulates ethylene synthesis and AsA production, which supply a possible mechanism coordinating ABA and ethylene to regulate AsA biosynthesis under salt stress. Ca2+ signaling could be induced by both ROS signaling and participates in AsA biosynthesis modulation through PMM. Arrows and lines with bars indicate activation and inhibition, respectively. Dotted lines indicate indirect regulations.
Conclusion and Perspectives
Emerging evidence provides the understanding of the roles of ethylene and AsA in salt tolerance through fine-tuning ROS homeostasis. Ethylene biosynthesis could be induced under salt stress, followed with ROS accumulation through transcriptional activation of Rbohs gene expression, in which ROS functions as a signal to regulate Na+/K+ homeostasis. Excessive ROS is toxic to plants, and ethylene also performs a scavenging role on ROS homeostasis under salt stress through signaling pathways, including the stability of EBF1/EBF2 and transcriptional regulation of EIN3/EIL1 on downstream direct or indirect regulators such as ESE1, SIED1, POD, JERF3, and ERF98. Ca2+ signaling, as a second messenger, could be induced by both ROS and AsA-mediated ROS balance, and participates in AsA biosynthesis modulation (Figure 1). The antagonistic effect of ethylene on ROS synthesis and scavenging under salinity stress is due to different functions of ROS at different developmental stages and in different tissues (Jiang et al., 2013; Peng et al., 2014). Additionally, non-enzymatic antioxidant AsA and the modulators involved in AsA biosynthesis confer to salt tolerance through reduced ROS accumulation (Figure 1; Wang J. et al., 2013; Qin et al., 2016; Li et al., 2018). However, the individual or crosstalk of ethylene and AsA regulation mechanisms on salt responses remain in need of further research. For example, although the components of the ethylene signaling pathway are conserved in Arabidopsis and rice (Yin et al., 2017), the underlying mechanisms of ethylene signaling in response to salt stress are different. Similarly, the effect of ethylene on plant growth is opposite in light and dark, such as hypocotyl elongation (Yu and Huang, 2017). Moreover, emerging research demonstrates that light plays a pivotal role in AsA synthesis (Fukunaga et al., 2010; Wang J. et al., 2013). These findings suggest a complex network regulated by ethylene signaling under different growth conditions. Further engagement is needed to determine whether ethylene and light coordinate AsA production to maintain ROS homeostasis during salt response. Furthermore, it is widely recognized that ABA and ethylene are simultaneously involved in stress responses (Kumar et al., 2016). ABA signaling component ABI4 mediates AsA-regulated plant growth (Kerchev et al., 2011) and inhibits ethylene biosynthesis (Dong et al., 2016; Figure 1). Nevertheless, the crosstalk between ethylene and ABA in the control AsA pool is unclear. Proper redox homeostasis is necessary for plant growth under salt stress; thus, making clear the detailed mechanisms of ethylene and AsA in maintaining ROS homeostasis will provide new insights for salt-tolerant genetic improvement.
Author Contributions
RH proposed the concept. JW organized and drafted the manuscript. RH contributed to the editing of the manuscript. Both authors read and approved the manuscript.
Funding
This work was supported by the National Key Research and Development Program of China (2016YFD0100604), the National Natural Science Foundation of China (31470366), Agricultural Science and Technology Innovation Program of the Chinese Academy of Agricultural Sciences, and the Fundamental Research Funds for Central Non-profit Scientific Institution (1610392018003).
Conflict of Interest Statement
The authors declare that the research was conducted in the absence of any commercial or financial relationships that could be construed as a potential conflict of interest.
References
Achard, P., Cheng, H., De Grauwe, L., Decat, J., Schoutteten, H., Moritz, T., et al. (2006). Integration of plant responses to environmentally activated phytohormonal signals. Science 311, 91–94. doi: 10.1126/science.1118642
Ahanger, M. A., Tomar, N. S., Tittal, M., Argal, S., and Agarwal, R. M. (2017). Plant growth under water/salt stress: ROS production; antioxidants and significance of added potassium under such conditions. Physiol. Mol. Biol. Plants 23, 731–744. doi: 10.1007/s12298-017-0462-7
Akram, N. A., Shafiq, F., and Ashraf, M. (2017). Ascorbic Acid-A potential oxidant scavenger and its role in plant development and abiotic stress tolerance. Front. Plant Sci. 8:613. doi: 10.3389/fpls.2017.00613
Bulley, S., and Laing, W. (2016). The regulation of ascorbate biosynthesis. Curr. Opin. Plant Biol. 33, 15–22. doi: 10.1016/j.pbi.2016.04.010
Cao, W. H., Liu, J., He, X. J., Mu, R. L., Zhou, H. L., Chen, S. Y., et al. (2007). Modulation of ethylene responses affects plant salt-stress responses. Plant Physiol. 143, 707–719. doi: 10.1104/pp.106.094292
Cho, K. M., Nguyen, H. T., Kim, S. Y., Shin, J. S., Cho, D. H., Hong, S. B., et al. (2016). CML10, a variant of calmodulin, modulates ascorbic acid synthesis. New Phytol. 209, 664–678. doi: 10.1111/nph.13612
Choi, W. G., Toyota, M., Kim, S. H., Hilleary, R., and Gilroy, S. (2014). Salt stress-induced Ca2+ waves are associated with rapid, long-distance root-to-shoot signaling in plants. Proc. Natl. Acad. Sci. U.S.A. 111, 6497–6502. doi: 10.1073/pnas.1319955111
Conklin, P. L. (2004). Ascorbic acid, a familiar small molecule intertwined in the response of plants to ozone, pathogens, and the onset of senescence. Plant Cell Environ. 27, 959–960. doi: 10.1111/j.1365-3040.2004.01203.x
Dong, Z., Yu, Y., Li, S., Wang, J., Tang, S., and Huang, R. (2016). Abscisic acid antagonizes ethylene production through the ABI4-mediated transcriptional repression of ACS4 and ACS8 in Arabidopsis. Mol. Plant 9, 126–135. doi: 10.1016/j.molp.2015.09.007
Dowdle, J., Ishikawa, T., Gatzek, S., Rolinski, S., and Smirnoff, N. (2007). Two genes in Arabidopsis thaliana encoding GDP-L-galactose phosphorylase are required for ascorbate biosynthesis and seedling viability. Plant J. 52, 673–689. doi: 10.1111/j.1365-313X.2007.03266.x
Dubois, M., Van den Broeck, L., and Inze, D. (2018). The pivotal role of ethylene in plant growth. Trends Plant Sci. 23, 311–323. doi: 10.1016/j.tplants.2018.01.003
Fukunaga, K., Fujikawa, Y., and Esaka, M. (2010). Light regulation of ascorbic acid biosynthesis in Rice via light responsive cis-elements in genes encoding ascorbic acid biosynthetic enzymes. Biosci. Biotechnol. Biochem. 74, 888–891. doi: 10.1271/bbb.90929
Guo, H., and Ecker, J. R. (2004). The ethylene signaling pathway: new insights. Curr. Opin. Plant Biol. 7, 40–49. doi: 10.1016/j.pbi.2003.11.011
Gusmaroli, G., Feng, S., and Deng, X. W. (2004). The Arabidopsis CSN5A and CSN5B subunits are present in distinct COP9 signalosome complexes, and mutations in their JAMM domains exhibit differential dominant negative effects on development. Plant Cell 16, 2984–3001. doi: 10.1105/tpc.104.025999
Hemavathi, Upadhyaya, C. P., Akula, N., Young, K. E., Chun, S. C., Kim, D. H., et al. (2010). Enhanced ascorbic acid accumulation in transgenic potato confers tolerance to various abiotic stresses. Biotechnol. Lett. 32, 321–330. doi: 10.1007/s10529-009-0140-0
Jiang, C., Belfield, E. J., Cao, Y., Smith, J. A., and Harberd, N. P. (2013). An Arabidopsis soil-salinity-tolerance mutation confers ethylene-mediated enhancement of sodium/potassium homeostasis. Plant Cell 25, 3535–3552. doi: 10.1105/tpc.113.115659
Julkowska, M. M., and Testerink, C. (2015). Tuning plant signaling and growth to survive salt. Trends Plant Sci. 20, 586–594. doi: 10.1016/j.tplants.2015.06.008
Kerchev, P. I., Pellny, T. K., Vivancos, P. D., Kiddle, G., Hedden, P., Driscoll, S., et al. (2011). The transcription factor ABI4 Is required for the ascorbic acid-dependent regulation of growth and regulation of jasmonate-dependent defense signaling pathways in Arabidopsis. Plant Cell 23, 3319–3334. doi: 10.1105/tpc.111.090100
Kostopoulou, Z., Therios, I., Roumeliotis, E., Kanellis, A. K., and Molassiotis, A. (2015). Melatonin combined with ascorbic acid provides salt adaptation in Citrus aurantium L. seedlings. Plant Physiol. Biochem. 86, 155–165. doi: 10.1016/j.plaphy.2014.11.021
Kumar, D., Hazra, S., Datta, R., and Chattopadhyay, S. (2016). Transcriptome analysis of Arabidopsis mutants suggests a crosstalk between ABA, ethylene and GSH against combined cold and osmotic stress. Sci. Rep. 6:36867. doi: 10.1038/srep36867
Laing, W. A., Martinez-Sanchez, M., Wright, M. A., Bulley, S. M., Brewster, D., Dare, A. P., et al. (2015). An upstream open reading frame is essential for feedback regulation of ascorbate biosynthesis in Arabidopsis. Plant Cell 27, 772–786. doi: 10.1105/tpc.114.133777
Li, C. H., Wang, G., Zhao, J. L., Zhang, L. Q., Ai, L. F., Han, Y. F., et al. (2014). The receptor-like kinase SIT1 mediates salt sensitivity by activating MAPK3/6 and regulating ethylene homeostasis in Rice. Plant Cell 26, 2538–2553. doi: 10.1105/tpc.114.125187
Li, S., Wang, J., Yu, Y., Wang, F., Dong, J., and Huang, R. (2016). D27E mutation of VTC1 impairs the interaction with CSN5B and enhances ascorbic acid biosynthesis and seedling growth in Arabidopsis. Plant Mol. Biol. 92, 473–482. doi: 10.1007/s11103-016-0525-0
Li, W., Ma, M., Feng, Y., Li, H., Wang, Y., Ma, Y., et al. (2015). EIN2-directed translational regulation of ethylene signaling in Arabidopsis. Cell 163, 670–683. doi: 10.1016/j.cell.2015.09.037
Li, Y., Chu, Z., Luo, J., Zhou, Y., Cai, Y., Lu, Y., et al. (2018). The C2H2 zinc-finger protein SlZF3 regulates AsA synthesis and salt tolerance by interacting with CSN5B. Plant Biotechnol. J. 16, 1201–1213. doi: 10.1111/pbi.12863
Liu, L., Jiang, Z., Zhang, S., Zhao, H., Yang, W., Siedow, J. N., et al. (2018). Both NaCl and H2O2 long-term stresses affect basal cytosolic Ca(2+) levels but only NaCl alters cytosolic Ca(2+) signatures in Arabidopsis. Front. Plant Sci. 9:1390. doi: 10.3389/fpls.2018.01390
Liu, M., Gomes, B. L., Mila, I., Purgatto, E., Peres, L. E., Frasse, P., et al. (2016). Comprehensive profiling of ethylene response factor expression identifies ripening-associated ERF genes and their link to key regulators of fruit ripening in tomato. Plant Physiol. 170, 1732–1744. doi: 10.1104/pp.15.01859
Ma, L., Zhang, H., Sun, L., Jiao, Y., Zhang, G., Miao, C., et al. (2012). NADPH oxidase AtrbohD and AtrbohF function in ROS-dependent regulation of Na(+)/K(+)homeostasis in Arabidopsis under salt stress. J. Exp. Bot. 63, 305–317. doi: 10.1093/jxb/err280
Makavitskaya, M., Svistunenko, D., Navaselsky, I., Hryvusevich, P., Mackievic, V., Rabadanova, C., et al. (2018). Novel roles of ascorbate in plants: induction of cytosolic Ca2+ signals and efflux from cells via anion channels. J. Exp. Bot. 69, 3477–3489. doi: 10.1093/jxb/ery056
Mellidou, I., and Kanellis, A. K. (2017). Genetic control of ascorbic acid biosynthesis and recycling in horticultural crops. Front. Chem. 5:50. doi: 10.3389/fchem.2017.00050
Peng, J., Li, Z., Wen, X., Li, W., Shi, H., Yang, L., et al. (2014). Salt-induced stabilization of EIN3/EIL1 confers salinity tolerance by deterring ROS accumulation in Arabidopsis. PLoS Genet. 10:e1004664. doi: 10.1371/journal.pgen.1004664
Phukan, U. J., Jeena, G. S., Tripathi, V., and Shukla, R. K. (2017). Regulation of Apetala2/Ethylene response factors in plants. Front. Plant Sci. 8:150. doi: 10.3389/fpls.2017.00150
Qi, J., Song, C. P., Wang, B., Zhou, J., Kangasjarvi, J., Zhu, J. K., et al. (2018). Reactive oxygen species signaling and stomatal movement in plant responses to drought stress and pathogen attack. J. Integr. Plant Biol. 60, 805–826. doi: 10.1111/jipb.12654
Qin, H., Wang, Y., Wang, J., Liu, H., Zhao, H., Deng, Z., et al. (2016). Knocking down the expression of GMPase gene OsVTC1-1 decreases salt tolerance of rice at seedling and reproductive stages. PLoS One 11:e0168650. doi: 10.1371/journal.pone.0168650
Rentel, M. C., and Knight, M. R. (2004). Oxidative stress-induced calcium signaling in Arabidopsis. Plant Physiol. 135, 1471–1479. doi: 10.1104/pp.104.042663
Richardson, A. C. (2004). High growing temperatures reduce fruit carbohydrate and vitamin C in kiwifruit. Plant Cell Environ. 27, 423–435. doi: 10.1111/j.1365-3040.2003.01161.x
Shalata, A., and Neumann, P. M. (2001). Exogenous ascorbic acid (vitamin C) increases resistance to salt stress and reduces lipid peroxidation. J. Exp. Bot. 52, 2207–2211. doi: 10.1093/jexbot/52.364.2207
Smirnoff, N. (2000). Ascorbic acid: metabolism and functions of a multi-facetted molecule. Curr. Opin. Plant Biol. 3, 229–235. doi: 10.1016/S1369-5266(00)00069-8
Steffens, B. (2014). The role of ethylene and ROS in salinity, heavy metal, and flooding responses in rice. Front. Plant Sci. 5:685. doi: 10.3389/fpls.2014.00685
Vidal-Meireles, A., Neupert, J., Zsigmond, L., Rosado-Souza, L., Kovacs, L., Nagy, V., et al. (2017). Regulation of ascorbate biosynthesis in green algae has evolved to enable rapid stress-induced response via the VTC2 gene encoding GDP-l-galactose phosphorylase. New Phytol. 214, 668–681. doi: 10.1111/nph.14425
Wang, F., Cui, X., Sun, Y., and Dong, C. H. (2013). Ethylene signaling and regulation in plant growth and stress responses. Plant Cell Rep. 32, 1099–1109. doi: 10.1007/s00299-013-1421-6
Wang, J., Yu, Y., Zhang, Z., Quan, R., Zhang, H., Ma, L., et al. (2013). Arabidopsis CSN5B interacts with VTC1 and modulates ascorbic acid synthesis. Plant Cell 25, 625–636. doi: 10.1105/tpc.112.106880
Wang, Y., Ji, Y., Fu, Y., and Guo, H. (2018). Ethylene-induced microtubule reorientation is essential for fast inhibition of root elongation in Arabidopsis. J. Integr. Plant Biol. 60, 864–877. doi: 10.1111/jipb.12666
Wu, L., Zhang, Z., Zhang, H., Wang, X. C., and Huang, R. (2008). Transcriptional modulation of ethylene response factor protein JERF3 in the oxidative stress response enhances tolerance of tobacco seedlings to salt, drought, and freezing. Plant Physiol. 148, 1953–1963. doi: 10.1104/pp.108.126813
Yanagawa, Y., Sullivan, J. A., Komatsu, S., Gusmaroli, G., Suzuki, G., Yin, J., et al. (2004). Arabidopsis COP10 forms a complex with DDB1 and DET1 in vivo and enhances the activity of ubiquitin conjugating enzymes. Genes Dev. 18, 2172–2181. doi: 10.1101/gad.1229504
Yang, C., Li, W., Cao, J., Meng, F., Yu, Y., Huang, J., et al. (2017). Activation of ethylene signaling pathways enhances disease resistance by regulating ROS and phytoalexin production in rice. Plant J. 89, 338–353. doi: 10.1111/tpj.13388
Yang, C., Ma, B., He, S. J., Xiong, Q., Duan, K. X., Yin, C. C., et al. (2015). MAOHUZI6/ETHYLENE INSENSITIVE3-LIKE1 and ETHYLENE INSENSITIVE3-LIKE2 regulate ethylene response of roots and coleoptiles and negatively affect salt tolerance in rice. Plant Physiol. 169, 148–165. doi: 10.1104/pp.15.00353
Yang, Y., and Guo, Y. (2018a). Elucidating the molecular mechanisms mediating plant salt-stress responses. New Phytol. 217, 523–539. doi: 10.1111/nph.14920
Yang, Y., and Guo, Y. (2018b). Unraveling salt stress signaling in plants. J. Integr. Plant Biol. 60, 796–804. doi: 10.1111/jipb.12689
Yao, Y., He, R. J., Xie, Q. L., Zhao, X. H., Deng, X. M., He, J. B., et al. (2017). ETHYLENE RESPONSE FACTOR 74 (ERF74) plays an essential role in controlling a respiratory burst oxidase homolog D (RbohD)-dependent mechanism in response to different stresses in Arabidopsis. New Phytol. 213, 1667–1681. doi: 10.1111/nph.14278
Yin, C. C., Zhao, H., Ma, B., Chen, S. Y., and Zhang, J. S. (2017). Diverse roles of ethylene in regulating agronomic traits in rice. Front. Plant Sci. 8:1676. doi: 10.3389/fpls.2017.01676
You, J., and Chan, Z. (2015). ROS regulation during abiotic stress responses in crop plants. Front. Plant Sci. 6:1092. doi: 10.3389/fpls.2015.01092
Yu, Y., and Huang, R. (2017). Integration of ethylene and light signaling affects hypocotyl growth in Arabidopsis. Front. Plant Sci. 8:57. doi: 10.3389/fpls.2017.00057
Yu, Y., Wang, J., Shi, H., Gu, J., Dong, J., Deng, X. W., et al. (2016). Salt stress and ethylene antagonistically regulate nucleocytoplasmic partitioning of COP1 to control seed germination. Plant Physiol. 170, 2340–2350. doi: 10.1104/pp.15.01724
Yu, Y., Wang, J., Zhang, Z., Quan, R., Zhang, H., Deng, X. W., et al. (2013). Ethylene promotes hypocotyl growth and HY5 degradation by enhancing the movement of COP1 to the nucleus in the light. PLoS Genet. 9:e1004025. doi: 10.1371/journal.pgen.1004025
Zhang, H., Li, A., Zhang, Z., Huang, Z., Lu, P., Zhang, D., et al. (2016). Ethylene response factor TERF1, regulated by ETHYLENE-INSENSITIVE3-like factors, functions in reactive oxygen species (ROS) scavenging in tobacco (Nicotiana tabacum L.). Sci. Rep. 6:29948. doi: 10.1038/srep29948
Zhang, M., Smith, J. A., Harberd, N. P., and Jiang, C. (2016). The regulatory roles of ethylene and reactive oxygen species (ROS) in plant salt stress responses. Plant Mol. Biol. 91, 651–659. doi: 10.1007/s11103-016-0488-1
Zhang, W., Yang, G., Mu, D., Li, H., Zang, D., Xu, H., et al. (2016). An ethylene-responsive factor BpERF11 negatively modulates salt and osmotic tolerance in betula platyphylla. Sci. Rep. 6:23085. doi: 10.1038/srep23085
Zhang, H., Liu, W., Wan, L., Li, F., Dai, L., Li, D., et al. (2010). Functional analyses of ethylene response factor JERF3 with the aim of improving tolerance to drought and osmotic stress in transgenic rice. Transgenic Res. 19, 809–818. doi: 10.1007/s11248-009-9357-x
Zhang, L., Li, Z., Quan, R., Li, G., Wang, R., and Huang, R. (2011). An AP2 domain-containing gene, ESE1, targeted by the ethylene signaling component EIN3 is important for the salt response in Arabidopsis. Plant Physiol. 157, 854–865. doi: 10.1104/pp.111.179028
Zhang, Z., Wang, J., Zhang, R., and Huang, R. (2012). The ethylene response factor AtERF98 enhances tolerance to salt through the transcriptional activation of ascorbic acid synthesis in Arabidopsis. Plant J. 71, 273–287. doi: 10.1111/j.1365-313X.2012.04996.x
Zheng, Y., Liao, C., Zhao, S., Wang, C., and Guo, Y. (2017). The glycosyltransferase QUA1 regulates chloroplast-associated calcium signaling during salt and drought stress in Arabidopsis. Plant Cell Physiol. 58, 329–341. doi: 10.1093/pcp/pcw192
Zhong, S., Shi, H., Xue, C., Wei, N., Guo, H., and Deng, X. W. (2014). Ethylene-orchestrated circuitry coordinates a seedling’s response to soil cover and etiolated growth. Proc. Natl. Acad. Sci. U.S.A. 111, 3913–3920. doi: 10.1073/pnas.1402491111
Keywords: ethylene, AsA, salt stress, ROS scavenging, homeostasis
Citation: Wang J and Huang R (2019) Modulation of Ethylene and Ascorbic Acid on Reactive Oxygen Species Scavenging in Plant Salt Response. Front. Plant Sci. 10:319. doi: 10.3389/fpls.2019.00319
Received: 01 September 2018; Accepted: 27 February 2019;
Published: 18 March 2019.
Edited by:
Yan Guo, China Agricultural University, ChinaReviewed by:
Tse-Min Lee, National Sun Yat-sen University, TaiwanYongqing Yang, China Agricultural University, China
Copyright © 2019 Wang and Huang. This is an open-access article distributed under the terms of the Creative Commons Attribution License (CC BY). The use, distribution or reproduction in other forums is permitted, provided the original author(s) and the copyright owner(s) are credited and that the original publication in this journal is cited, in accordance with accepted academic practice. No use, distribution or reproduction is permitted which does not comply with these terms.
*Correspondence: Rongfeng Huang, rfhuang@caas.cn