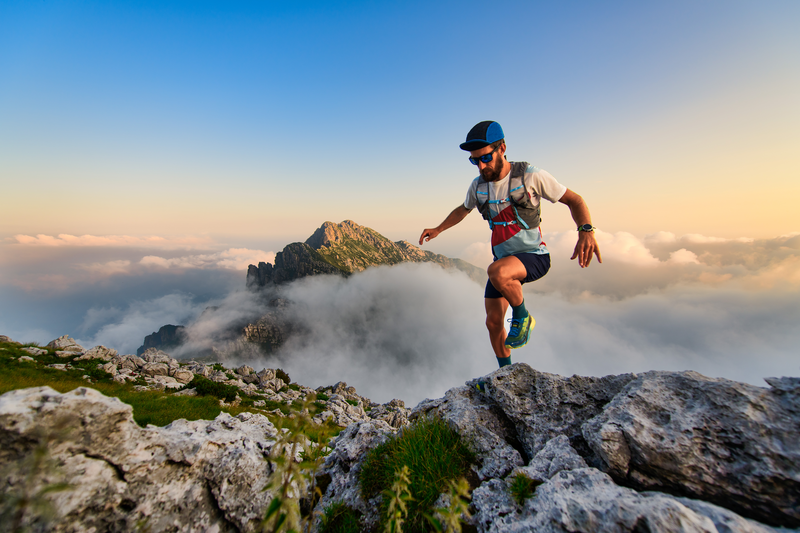
94% of researchers rate our articles as excellent or good
Learn more about the work of our research integrity team to safeguard the quality of each article we publish.
Find out more
ORIGINAL RESEARCH article
Front. Plant Sci. , 20 February 2019
Sec. Functional Plant Ecology
Volume 10 - 2019 | https://doi.org/10.3389/fpls.2019.00169
This article is part of the Research Topic Diversity and Eco-Physiological Responses of Aquatic Plants View all 23 articles
Light is a major limiting resource in aquatic ecosystems and numerous studies have investigated the response of submerged macrophytes to low light conditions. However, few studies have tested whether different light response strategies can also have consequences for macrophyte distribution along different littoral slopes in lakes, which are known to affect macrophyte biomass due to differences in drag forces and sediment characteristic. In this study, we tested (1) whether two macrophyte species of different growth forms (canopy-forming: Potamogeton maackianus, rosette-type: Vallisneria natans) differ in their response strategies to low light conditions and (2) how these responses influence their distribution along different basin slopes in the mesotrophic Lake Erhai, China. We hypothesized that the canopy-forming species responds to low light conditions at deeper sites by stem elongation while the rosette-type species increases its shoot chlorophyll content. As a consequence, P. maackianus should have a higher susceptibility to drag forces and thus prevail at sites with lower slopes. Sites with higher slopes should offer a niche for rosette-type species like V. natans that can better withstand drag forces. We surveyed the distribution and abundance of the two macrophyte species at 527 sampling points along 97 transects in Lake Erhai and measured their height, leaf and stem/rhizome biomass, and leaf chlorophyll a content at different water depths. Our results confirmed stem elongation as a strategy to low light conditions by the canopy-forming species P. maackianus, while V. natans produced more chlorophyll a per shoot biomass at deeper sites to tolerate shading. As hypothesized, these alternative response strategies to low light conditions resulted in a trade-off regarding the plants ability to grow at different basin slopes. P. maackianus was dominant at sites with low-moderate slope (0–4%) and low-moderate water depth (2–4 m), while sites with high basin slope (4–7%) combined with moderate-high water depth (3–5 m) were dominantly colonized by V. natans. The latter habitat thus represents a potential refuge for rosette-type macrophyte species that are often outcompeted when shading increases during eutrophication.
Light limitation has driven the evolution of highly plastic adaptive strategies in plants to either tolerance or avoidance of shading by neighboring vegetation (Franklin, 2008). In freshwater ecosystems, roughly 10% of the global radiation is reflected at the air-water interface and a significant portion is attenuated with depth resulting in low light conditions in most deep aquatic habitats (Spence, 1981). Consequently, numerous aquatic plants have evolved a high plasticity in morphological and physiological traits in response to low light conditions (Maberly, 1993; Olesen et al., 2002). Previous studies showed that the response strategy of submerged macrophytes to low light conditions largely depends on their growth form (Chambers and Kalff, 1987; Fu et al., 2012). Canopy-forming species, such as Myriophyllum spicatum and Potamogeton wrightii, tend to allocate more biomass to stems, grow taller and form dense canopies to counter light attenuation in the water column (Strand and Weisner, 2001; Fu et al., 2012). In contrast, rosette-type macrophytes such as Vallisneria species can increase their plant height in deeper sites as well (Fu et al., 2012), but mainly tolerate shading through a lower light compensation point and a higher leaf mass ratio of total plant mass compared to canopy-forming macrophytes (Su et al., 2004; Chen et al., 2016).
Increasing eutrophication of lakes leads to increased shading of submerged macrophytes by phytoplankton and periphyton. As a consequence, small species such as charophytes and rosette-type angiosperms disappear and tall, canopy-forming macrophytes that can escape low light conditions become dominant (Sand-Jensen et al., 2008; He et al., 2015; Hilt et al., 2018). However, other factors such as morphometric characteristics of the littoral area additionally may influence the biomass and community structure of submerged macrophytes in lakes (Kolada, 2014). Littoral slope for example was suggested as a good predictor of the maximum biomass of submerged macrophyte communities (Duarte and Kalff, 1986). Low slope areas usually have fine, nutrient-rich sediments and little water movement, while coarse, nutrient-poor sediments and stronger water movement characterize high slope areas in temperate lakes (Håkanson, 1977; Duarte and Kalff, 1986). In aquatic environments, hydrodynamic forces caused by water movement can be many times the drag forces produced by wind on land (Puijalon et al., 2011). Currents and waves can cause strong damage to or even uproot submerged plants (Bornette and Puijalon, 2011). However, the degree of damage depends on the sediment characteristic (Barko and Smart, 1986; Schutten et al., 2005; Spierenburg et al., 2013) and the macrophyte’s ability to resist breakage and uprooting, which is closely related to its size and shape (Schutten and Davy, 2000; Schutten et al., 2004). Generally, short rosettes with linear soft leaves are better at resisting drag forces compared to tall canopy-forming submerged macrophytes at a given water velocity (Puijalon et al., 2011). Hence, short rosette-type species may be better adapted to disturbance by water movement. In addition, canopy-forming submerged macrophytes need more nutrients to support their high growth rates, while short rosette-type species grow slowly and can survive under lower nutrient supply (Chambers, 1987). Therefore, the canopy-forming species may dominate in low slope areas of mesotrophic lakes, while rosette-type species can better tolerate the stress of higher slopes.
Although numerous studies have investigated the response of macrophytes to low light conditions, only a few of them have tested whether these response strategies to shading conditions affect the distribution of macrophytes along other environmental gradients such as sediment characteristic and drag forces (Spierenburg et al., 2013). In this study, we measured different morphological and physiological parameters and the abundance of two dominant macrophyte species, the canopy-forming P. maackianus and the rosette-type species V. natans at different water depths (determining light availability) and littoral slopes (determining sediment characteristic and drag forces) in the mesotrophic Lake Erhai, China. We tested (1) whether the two species show the response strategies to low light conditions typical for canopy- and rosette-type macrophytes and (2) whether these responses influence their distribution along different basin slopes. We hypothesized that the canopy-forming species P. maackianus responds to low light conditions in deeper water by stem elongation while V. natans increases its shoot chlorophyll content. As a consequence, P. maackianus should have a higher susceptibility to drag forces and thus prevail at sites with lower slopes. In contrast, the rosette-type species V. natans should tolerate higher drag forces and thus be prevalent at sites with higher slopes.
The study was carried out in the mesotrophic Lake Erhai (25°52′N, 100°06′E), located in the Yunnan province of China (Figure 1). The lake has a total area of 249 km2, a moderate water depth (maximum depth 20.5 m, mean depth 10.5 m) and a large variation in littoral slopes. Macrophytes show a zonation along the water depth gradient, with most of the 12 submerged species inhabiting shallow water (0–3.0 m depth), and only a few species extending to deeper water (Fu et al., 2014). Maximum colonization depth of submerged macrophytes was around 5.5 m and about 20 km2 of the lake littoral area was covered by submerged macrophytes (Figure 1) and the community was dominated by canopy-forming P. maackianus and rosette-type V. natans in 2013. P. maackianus is a clonal and perennial submerged macrophyte widely distributed in East Asia (Sun, 1992), and forms dense canopies and monocultures in many mesotrophic shallow lakes (Ni, 2001). V. natans is a common rosette-type submerged macrophyte species in China. It mainly spreads by clonal reproduction and its aboveground part can overwinter in Lake Erhai.
Figure 1. Macrophyte coverage in Lake Erhai (left), location of the lake in China (below) and sampling scheme at each transect (above). The small rectangle at the bottom right shows the South China Sea Islands.
Submerged macrophyte biomass was collected at 97 transects uniformly distributed perpendicular to the shoreline around Lake Erhai from 16 July to 6 August 2013. Samples were taken at intervals of 1 m water depths along each transect from the shore to the deepest site of macrophyte occurrence (i.e., from around 0.5 m to 5.5 m water depth) (Figure 1). Depending on the macrophyte colonization depth, this resulted in 5 to 6 samples per transect, and in total 527 sampling points. A reaping hook covering a bottom area of 0.2 m2 was used to collect submerged macrophytes, with 3 replicates at each sampling point (Figure 1). Plants were washed, sorted by species, and weighed as fresh biomass (FW). GPS coordinates of each sampling point were recorded by a GPS receiver (GPS map 60CSx).
We randomly sampled around 20 full-grown healthy individuals of P. maackianus or V. natans at each sampling point when the community was dominated by one of these two species and all plant samples were kept in dark containers with ice bags during field work. Three individuals from each sampling point were immediately put into a freezer at −20°C when returning to the laboratory for measuring leaf chlorophyll (chl) a content. Part of the mixed freeze-dried leaves from each individual were ground in a mortar and extracted in 96% ethanol for 24 h at 4°C in the dark. The solution was then centrifuged at 4000 × g for 10 min, and the chl a content determined spectrophotometrically at 665 nm and 649 nm. Plant heights of the remaining individuals were measured and plants were separated into leaves, stems (for P. maackianus) or rhizomes (for V. natans) and dried at 80°C for 48 h to determine the dry weight (DW).
Shoot (leaf+stem/rhizome) chl a content is an important indicator for photosynthetic capacity of submerged macrophytes (Nielsen and Sand-Jensen, 1989). We determined leaf chl a contents and then related it to the entire shoot biomass by calculating (leaf chl a content) × (leaf dry weight)/(leaf dry weight + stem or rhizome dry weight). We used t-tests to compare the trait values between the two species and linear regression analysis to evaluate the response of different plant traits to water depth. Plant trait values at each sampling point were represented by the average of all the individuals in that sampling point (i.e., 20 individuals for shoot mass, leaf mass, stem mass, shoot height and 3 individuals for leaf chl a and shoot chl a content). When plant traits were significantly correlated with water depth for both species, analysis of covariance (ANCOVA) was used to test for differences in the slope of the regression line between the two species. For traits that were correlated with plant size (i.e., leaf biomass, stem biomass, and shoot height), we additionally analyzed biomass-corrected values. To remove differences in size, we conducted a linear regression of each log-transformed trait on log-transformed shoot biomass. The residual values from this regression were saved because they represent size-independent measures of normalized traits (McCoy et al., 2006). We then used linear regression analysis to evaluate the relationship between the residual values and water depth. In this case, the individual trait values were used for the analysis.
GPS coordinates of each sampling point were used to calculate the distance from the shallowest sampling point to the other sampling points in each transect. Then, the littoral slope of each transect was calculated as follows:
Where D is the water depth of the sampling point (except for the shallowest sampling point); D1 is the water depth of the shallowest sampling point; L is the distance from the sampling point to shallowest sampling point in each transect and a is the coefficient of Equation 1. The proportion of the two target species in the total macrophyte biomass (sum of all species) was calculated for each sampling point. In order to compare our results with an earlier study by Duarte and Kalff (1986) on the influence of littoral slopes on biomass of submerged macrophyte communities, we averaged the total macrophyte biomass of all the sampling points in each transect and then examined the relationship between the averaged biomass and the littoral slope. We used the GAM (Generalized Additive Model) to fit the relationship between slope, water depth and total macrophyte biomass as well as proportion of the target species in total macrophyte biomass. GAM is a semi-parametric extension of generalized linear models that enables the user to fit complex non-linear relationships and handle different types of error distributions (Wood, 2006). The models were built with function “gam” in package “mgcv” using penalized regression splines as the smoothing function, Gaussian error distribution, and automatic calculation of smoothing parameters. The main effects and the interaction of the slope and water depth were included in the models. All analyses were performed in R (R Core Team, 2017).
Most of the plant traits measured were significantly different between P. maackianus and V. natans (Table 1). Shoot biomass of P. maackianus (0.54 ± 0.22 g) was lower than that of V. natans (0.73 ± 0.36 g), while plant height of P. macckianus (187 ± 52 cm) was much higher than that of V. natans (115 ± 28 cm). Leaf biomass of P. maackianus (0.20 ± 0.14 g) was much lower than that of V. natans (0.63 ± 0.2 g). Inversely, the stem biomass of the P. maackianus (0.34 ± 0.12 g) was much higher than rhizome biomass of V. natans (0.09 g). The leaf chl a content of the two species was similar (V. natans 7.1 ± 2.0 mg g−1, P. maackianus 7.2 ± 2.2 mg g−1); however, the chl a content per shoot dry weight of P. maackianus (2.5 ± 0.9 mg g−1) was much lower than that of V. natans (6.3 ± 2.0 mg g−1) (Table 1).
Table 1. Comparison between traits values (means ± SD) of Potamogeton maackianus and Vallisneria natans from Lake Erhai.
Plant height of P. maackianus were higher than that of V. natans at a given water depth (Figure 2). Plant height of both species increased linearly with water depth, but the slope of the regression line of P. maackianus was steeper than that of V. natans (t = −2.94, p < 0.01). Leaf chl a contents were significantly positively correlated with water depth for both P. maackianus and V. natans (Table 2). Shoot chl a content of P. maackianus was stable with increasing water depth, while shoot chl a content of V. natans increased strongly. P. maackianus allocated less biomass into leaves with increasing water depth while the opposite was true for V. natans (Table 2).
Figure 2. Relationship between plant height (A) and shoot chlorophyll a content (B) of Potamogeton maackianus and Vallisneria natans and water depth in Lake Erhai. The lines are predicted by linear models.
Table 2. Relationship between plant traits of Potamogeton maackianus and Vallisneria natans and water depth in Lake Erhai based on linear models.
The corrected stem biomass and plant height of P. maackianus increased with water depth and the reverse was found for corrected leaf biomass (Table 2). While corrected rhizome biomass of V. natans decreased with water depth, the reverse was found for leaf mass and height (Table 2).
Littoral slope explained about 53% of the variation of the averaged total macrophyte biomass of transect in Lake Erhai (Table 3). The averaged total macrophyte biomass of transect decreased with basin slope when slopes were lower than 2% (Figure 3), but was relatively stable with increasing slopes above 2%. The combination of littoral slope, water depth and their interaction explained 40% of the variation of the total macrophyte biomass of sampling point (Table 3). The highest biomass of submerged macrophytes appeared at a low slope (0–1%) and moderate water depth (2–4 m) (Figure 4). The GAM model explained 21% and 27% of the variation of the proportion of P. maackianus and V. natans in the total macrophyte biomass, respectively (Table 3). The proportion of P. maackianus in the total macrophyte biomass decreased with increasing littoral slope (Figure 5A), whereas the proportion of V. natans increased with increasing littoral slope (Figure 5B).
Figure 3. Total biomass of submerged macrophytes (FW: fresh weight) along littoral slopes of Lake Erhai. The line is predicted by Generalized Additive Model (n = 97).
Figure 4. Total biomass of submerged macrophytes (kg fresh weight m−2) depending on water depth and littoral slope of Lake Erhai based on a Generalized Additive Model (n = 527).
Figure 5. Proportion of the submerged macrophyte species P. maackianus (A) and V. natans (B) in the total macrophyte biomass along the water depth and littoral slope of Lake Erhai based on a Generalized Additive Model (n = 527).
Our data on morphological and physiological traits and distribution of two abundant macrophyte species with different growth forms in Lake Erhai confirmed both initial hypotheses. Both tested species show response strategies to low light conditions typical for canopy-forming (P. maackianus) and rosette-type (V. natans) macrophyte species (Table 4) and these response strategies significantly influenced their distribution along different water depth and basin slopes.
Table 4. Response strategies of canopy-forming and rosette-type submerged macrophytes to low light conditions.
Stem elongation was found as a major response strategy to low light conditions by the canopy-forming species P. maackianus, while the rosette-type species V. natans produced more chl a per shoot biomass at deeper sites to tolerate light attenuation. These findings are consistent with a similar study by Chen et al. (2016) assessing the response of P. maackianus and V. natans to low light conditions in Lake Donghu, China. In our study, V. natans increased leaf chl a content and allocated more biomass to leaves at deep sampling points, resulting in a strong increase of chl a content per shoot biomass. Such strategy combined with a low light compensation point for photosynthesis (Chen et al., 2016), allows V. natans to successfully survive at sites with low light conditions in Lake Erhai and colonize deep areas with high slopes. Other rosette-type species such as V. americana have shown a similar morphological and physiological response to low light conditions (French and Moore, 2003). This indicates that rosette-type species may mainly adopt a tolerance strategy towards stress in low light conditions (Table 4). In contrast, P. maackianus responds to low light conditions mainly through allocating more shoot biomass into stems to concentrate leaves closer to the water surface. In our study, leaf chl a content of P. maackianus slightly increased with water depth, but the chl a content per shoot biomass did not increase with water depth as less leaf biomass formed at deeper sites. This shade avoidance strategy has also been found in terrestrial plants (Franklin, 2008) and seems common among canopy-forming submerged species (Table 4).
The alternative response strategies to low light conditions of P. maackianus and V. natans affected their ability to grow at different basin slopes and water depths. The canopy-forming species P. maackianus was dominant at sites with low-moderate water depth (2–4 m) and low-moderate basin slopes (0–4%). In contrast, the rosette-type V. natans prevailed at deeper sites (3–5 m) with higher slopes (4%–7%). The potential mechanism allowing V. natans growing at deeper waters than P. maackianus is that photosynthetic adjustments would become more important in determining plant abundance in deep water due to the lower carbon requirements compared with shoot elongation (Chen et al., 2016).
Previous studies suggested the plant communities may be governed by a dominance–tolerance trade-off, where most species perform best in benign, productive sites (i.e., undisturbed sites with a high availability of resources); however, there is often a trade-off between the ability to dominate at productive sites or to sequester high-quality resources and the ability to persist on low-quality resources or to tolerate harsh conditions (Wisheu and Keddy, 1992; McGill et al., 2006). Lake eutrophication enhances the availability of nutrients both in sediment and water column which initially increases the competition for light between the submerged macrophytes. As a result, short-growing species (e.g., charophytes and rosette-type angiosperms) are commonly replaced by canopy-forming species (Hilt et al., 2018), which can form dense monocultures in mesotrophic lakes (He et al., 2015). However, high slope areas in mesotrophic lakes may provide a refuge for short-growing species due to a trade-off between the ability to compete for light and the ability to tolerate harsh conditions like strong drag forces and nutrient-poor sediment at high slope areas. As a consequence of the different response strategies to low light conditions, P. maackianus plants are taller than V. natans in a given water depth and therefore have an advantage in competing for light. However, this strategy and their thin stems result in a lower resistance of P. maackianus to drag forces by currents and waves (Puijalon et al., 2011; Fu et al., 2014). In contrast, V. natans has ribbon-like leaves growing close to the bottom, which are more resistant to drag than P. maackianus in a given flow velocity (Puijalon et al., 2011). The nutrient and organic matter content in sediment in low slope areas are not assumed to limit the growth of V. natans since this species has been shown to grow on a wide range of sediment types in this lake (He et al., 2017). For instance, the total nitrogen, total phosphorus and organic matter contents in sediments were around 3, 0.7, and 100 mg g−1, respectively in Haichao bay of Lake Erhai (unpublished data), all of which are suitable for growth of V. natans (Xiao et al., 2007). However, the macrophyte community was dominated by P. maackianus rather than V. natans in this area, which indicated the V. natans might be excluded by competition other than sediment nutrient content in low slope areas. Consequently, this trade-off between the response strategy to low light conditions and hydrodynamic disturbance resistance likely determines the distribution and abundance of the two major macrophyte species with different growth forms in Lake Erhai. Still, other mechanisms such as wind or other sediment characteristics may contribute to the observed distribution of the two species (Schutten et al., 2004, 2005).
Our finding of a significantly decreased total macrophyte biomass with increasing basin slope is consistent with previous observations made in temperate lakes by Duarte and Kalff (1986). Total macrophyte biomass in Lake Erhai decreased significantly at littoral slopes above 2%. Our data suggest that the different response of macrophyte species to low light conditions may contributes to this pattern. In the 1970s and 1980s, submerged macrophytes covered around 40% of Lake Erhai, and the dominant species were Hydrilla verticillata and V. natans. Macrophyte coverage decreased from 40% to 8% from 1980s to 2012 due to eutrophication. During this period, most of the short species such as charophytes and V. natans were lost in deeper areas and H. verticillata was replaced by P. maackianus (Fu et al., 2013). However, V. natans still occupies large areas of the littoral zone in this lake, especially in high slope areas, confirming our finding that these habitat conditions may provide a niche for survival of this species. Similar displacements of rosette species have been found in temperate lakes, where Isoetes tend to grow deep under oligotrophic conditions, but are similarly displaced as V. natans to the littoral zone with strong disturbance during eutrophication (Arts, 2002).
We conclude that the different response strategies of submerged macrophytes with different growth forms (rosette-type versus canopy-forming) to low light conditions might significantly affect their distribution and abundance in lakes along gradients of other stressors such as physical forces. Morphological heterogeneity of lakes may thus contribute to the maintenance of a high diversity of submerged macrophytes, especially under mesotrophic conditions where competition for light between macrophytes is particularly relevant (Salgado et al., 2017).
TC and LN conceived the idea and proposed the method. LH, TZ, YW, WL, HZ, and XZ contributed to conduct the sampling and traits measurements. LH, TC, XZ, and SH wrote the manuscript. All authors read and approved the final manuscript.
This work was supported by State Key Laboratory of Freshwater Ecology and Biotechnology (Grant No. 2016FBZ08), National Science Foundation of China (Grant Nos. 31700402 and 31870446), and Natural Science Foundation of Jiangxi Province (Grant No. 2015ZBBF6008).
The authors declare that the research was conducted in the absence of any commercial or financial relationships that could be construed as a potential conflict of interest.
The handling Editor is currently organizing a Research Topic with one of the authors TC and confirms the absence of any other collaboration.
We thank Spencer Froelick for checking the language.
Arts, G. H. (2002). Deterioration of Atlantic soft water macrophyte communities by acidification, eutrophication and alkalinisation. Aquat. Bot. 73, 373–393. doi: 10.1016/S0304-3770(02)00031-1
Barko, J. W., and Smart, R. M. (1986). Sediment-related mechanisms of growth limitation in submersed macrophytes. Ecology 67, 1328–1340. doi: 10.2307/1938689
Bornette, G., and Puijalon, S. (2011). Response of aquatic plants to abiotic factors: a review. Aquat. Sci. 73, 1–14. doi: 10.1007/s00027-010-0162-7
Chambers, P. A. (1987). Light and nutrients in the control of aquatic plant community structure. II. In situ observations. J. Ecol. 75, 621–628. doi: 10.2307/2260194
Chambers, P. A., and Kalff, J. (1987). Light and nutrients in the control of aquatic plant community structure. I. In situ experiments. J. Ecol. 75, 621–628. doi: 10.2307/2260193
Chen, J., Cao, T., Zhang, X., Xi, Y., Ni, L., and Jeppesen, E. (2016). Differential photosynthetic and morphological adaptations to low light affect depth distribution of two submersed macrophytes in lakes. Sci. Rep. 6:34028. doi: 10.1038/srep34028
Duarte, C. M., and Kalff, J. (1986). Littoral slope as a predictor of the maximum biomass of submerged macrophyte communities. Limnol. Oceanogr. 31, 1072–1080. doi: 10.4319/lo.1986.31.5.1072
Franklin, K. A. (2008). Shade avoidance. New Phytol. 179, 930–944. doi: 10.1111/j.1469-8137.2008.02507.x
French, G. T., and Moore, K. A. (2003). Interactive effects of light and salinity stress on the growth, reproduction, and photosynthetic capabilities of Vallisneria americana (wild celery). Estuaries 26, 1255–1268. doi: 10.1007/BF02803628
Fu, H., Yuan, G., Cao, T., Ni, L., Zhang, M., and Wang, S. (2012). An alternative mechanism for shade adaptation: implication of allometric responses of three submersed macrophytes to water depth. Ecol. Res. 27, 1087–1094. doi: 10.1007/s11284-012-0991-z
Fu, H., Yuan, G., Cao, T., Zhong, J., Zhang, X., Guo, L., et al. (2013). Succession of submerged macrophyte communities in relation to environmental change in Lake Erhai over the past 50 years. J. Lake Sci. 25, 854–861. doi: 10.18307/2013.0609
Fu, H., Zhong, J., Yuan, G., Xie, P., Guo, L., Zhang, X., et al. (2014). Trait-based community assembly of aquatic macrophytes along a water depth gradient in a freshwater lake. Freshwater Biol. 59, 2462–2471. doi: 10.1111/fwb.12443
Håkanson, L. (1977). The influence of wind, fetch, and water depth on the distribution of sediments in Lake Vänern, Sweden. Can. J. Earth Sci. 14, 397–412. doi: 10.1139/e77-040
He, L., Zhu, T., Cao, T., Li, W., Zhang, M., Zhang, X., et al. (2015). Characteristics of early eutrophication encoded in submerged vegetation beyond water quality: a case study in Lake Erhai, China. Environ. Earth Sci. 74, 3701–3708. doi: 10.1007/s12665-015-4202-4
He, W., Cao, T., Ni, L., and Song, B. (2017). Growth of seven submerged macrophytes cultured on five sediment mixtures from the Lake Erhai. Acta Hydrobiol. Sin. 41, 428–436.
Hilt, S., Alirangues Nunez, M. M., Bakker, E. S., Blindow, I., Davidson, T., Gillefalk, M., et al. (2018). Response of submerged macrophytes to external and internal restoration measures of temperate shallow lakes. Front. Plant Sci. 9:194. doi: 10.3389/fpls.2018.00194
Kolada, A. (2014). The effect of lake morphology on aquatic vegetation development and changes under the influence of eutrophication. Ecol. Indicat. 38, 282–293. doi: 10.1016/j.ecolind.2013.11.015
Maberly, S. (1993). Morphological and photosynthetic characteristics of Potamogeton obtusifolius from different depths. J. Aquat. Plant Manag. 31, 34–39.
McCoy, M. W., Bolker, B. M., Osenberg, C. W., Miner, B. G., and Vonesh, J. R. (2006). Size correction: comparing morphological traits among populations and environments. Oecologia 148, 547–554. doi: 10.1007/s00442-006-0403-6
McGill, B. J., Enquist, B. J., Weiher, E., and Westoby, M. (2006). Rebuilding community ecology from functional traits. Trends Ecol. Evol. 21, 178–185. doi: 10.1016/j.tree.2006.02.002
Ni, L. (2001). Growth of Potamageton maackianus under low-light stress in eutrophic water. J. Freshwater Ecol. 16, 249–256. doi: 10.1080/02705060.2001.9663809
Nielsen, S. L., and Sand-Jensen, K. (1989). Regulation of photosynthetic rates of submerged rooted macrophytes. Oecologia 81, 364–368. doi: 10.1007/BF00377085
Olesen, B., Enriquez, S., Duarte, C. M., and Sand-Jensen, K. (2002). Depth-acclimation of photosynthesis, morphology and demography of Posidonia oceanica and Cymodocea nodosa in the Spanish Mediterranean Sea. Mar. Ecol. Prog. Ser. 236, 89–97. doi: 10.3354/meps236089
Puijalon, S., Bouma, T. J., Douady, C. J., van Groenendael, J., Anten, N. P. R., Martel, E., et al. (2011). Plant resistance to mechanical stress: evidence of an avoidance-tolerance trade-off. New Phytol. 191, 1141–1149. doi: 10.1111/j.1469-8137.2011.03763.x
R Core Team (2017). R: A Language and Environment for Statistical Computing. Version 3.1.2. Vienna: R Foundation for statistical computing.
Salgado, J., Sayer, C. D., Brooks, S. J., Davidson, T. A., and Okamura, B. (2017). Eutrophication erodes inter-basin variation in macrophytes and co-occurring invertebrates in a shallow lake: combining ecology and palaeoecology. J. Paleolimnol. 60, 311–328. doi: 10.1007/s10933-017-9950-6
Sand-Jensen, K., Pedersen, N. L., Thorsgaard, I., Moeslund, B., Borum, J., and Brodersen, K. P. (2008). 100 years of vegetation decline and recovery in Lake Fure, Denmark. J. Ecol. 96, 260–271. doi: 10.1111/j.1365-2745.2007.01339.x
Schutten, J., Dainty, J., and Davy, A. J. (2004). Wave-induced hydraulic forces on submerged aquatic plants in shallow lakes. Ann. Bot. 93, 333–341. doi: 10.1093/aob/mch043
Schutten, J., Dainty, J., and Davy, A. J. (2005). Root anchorage and its significance for submerged plants in shallow lakes. J. Ecol. 93, 556–571. doi: 10.1111/j.1365-2745.2005.00980.x
Schutten, J., and Davy, A. J. (2000). Predicting the hydraulic forces on submerged macrophytes from current velocity, biomass and morphology. Oecologia 123, 445–452. doi: 10.1007/s004420000348
Spence, D. (1981). “Light quality and plant responses underwater,” in Plants and the Daylight Spectrum, ed. H. Smith (London: Academic Press),245–275.
Spierenburg, P., Lucassen, E. C. H. E. T., Pulido, C., Smolders, A. J. P., and Roelofs, J. G. M. (2013). Massive uprooting of Littorella uniflora (L.) A sch. during a storm event and its relation to sediment and plant characteristics. Plant Biol. 15, 955–962. doi: 10.1111/j.1438-8677.2012.00707.x
Strand, J. A., and Weisner, S. E. (2001). Morphological plastic responses to water depth and wave exposure in an aquatic plant (Myriophyllum spicatum). J. Ecol. 89, 166–175. doi: 10.1046/j.1365-2745.2001.00530.x
Su, W., Zhang, G., Zhang, Y., Xiao, H., and Xia, F. (2004). The photosynthetic characteristics of five submerged aquatic plants. Acta Hydrobiol. Sin. 28,395–400.
Wisheu, I. C., and Keddy, P. A. (1992). Competition and centrifugal organization of plant communities: theory and tests. J. Veg. Sci. 3, 147–156. doi: 10.2307/3235675
Wood, S. N. (2006). Generalized Additive Models: An Introduction With R. London: CRC Press. doi: 10.1201/9781420010404
Keywords: submerged macrophyte, littoral slope, water depth, response strategies, species distribution
Citation: He L, Zhu T, Wu Y, Li W, Zhang H, Zhang X, Cao T, Ni L and Hilt S (2019) Littoral Slope, Water Depth and Alternative Response Strategies to Light Attenuation Shape the Distribution of Submerged Macrophytes in a Mesotrophic Lake. Front. Plant Sci. 10:169. doi: 10.3389/fpls.2019.00169
Received: 29 June 2018; Accepted: 31 January 2019;
Published: 20 February 2019.
Edited by:
Chunhua Liu, Wuhan University, ChinaReviewed by:
Jorge Salgado Bonnet, University of Los Andes, ColombiaCopyright © 2019 He, Zhu, Wu, Li, Zhang, Zhang, Cao, Ni and Hilt. This is an open-access article distributed under the terms of the Creative Commons Attribution License (CC BY). The use, distribution or reproduction in other forums is permitted, provided the original author(s) and the copyright owner(s) are credited and that the original publication in this journal is cited, in accordance with accepted academic practice. No use, distribution or reproduction is permitted which does not comply with these terms.
*Correspondence: Xiaolin Zhang, emhhbmd4bEBpaGIuYWMuY24= Te Cao, Y2FvdGVAaWhiLmFjLmNu
Disclaimer: All claims expressed in this article are solely those of the authors and do not necessarily represent those of their affiliated organizations, or those of the publisher, the editors and the reviewers. Any product that may be evaluated in this article or claim that may be made by its manufacturer is not guaranteed or endorsed by the publisher.
Research integrity at Frontiers
Learn more about the work of our research integrity team to safeguard the quality of each article we publish.