- The National Field Station of Freshwater Ecosystem of Liangzi Lake, College of Life Sciences, Wuhan University, Wuhan, China
Clonal plants may face various types of resource heterogeneity in their natural habitats; as such, spatial or temporal resource heterogeneity can affect the growth of clonal plants. Clonal plants can concentrate their organs in a smaller area where resources are high would cause heterogeneity to increase competition between plants. Most studies on resource heterogeneity have investigated the response of plants under a single density or by manipulating a single resource. Few studies have tested the effects of the heterogeneous distribution of two covariable resources on plant growth and intraspecific competition. A greenhouse experiment was therefore conducted to study plant responses to the spatial and temporal heterogeneity of the soil and water supply under a variety of plant densities (one, two, four, or six plants per container). The perennial clonal herb Bolboschoenus yagara was grown under different combinations of water supply patterns, soil nutrient distribution types and plant densities while maintaining the total water and soil nutrient availability per container constant. Compared with that at a relatively high plant density, soil nutrient heterogeneity resulted in significantly less total plant biomass and less-modified morphological traits when the plant density is relative low. At the highest plant density, compared with the homogeneous soil treatments, the heterogeneous soil treatments significantly increased the total biomass and R/S ratio. Water supply patterns also clearly affected plant morphological traits at the highest plant density. Furthermore, soil heterogeneity significantly increased intraspecific competition intensity at low plant densities, but did not significantly affect intraspecific competition intensity at higher plant densities. Water heterogeneity had little impact on intraspecific competition. These results suggest that the growth performance and intraspecific competition of B. yagara are more strongly affected by soil nutrient distribution rather than by water supply patterns and that competition for soil nutrients may increase plant sensitivity to soil heterogeneity.
Introduction
Resources (light, water, and nutrients) exhibit spatial and temporal heterogeneity which is ubiquitous within natural habitats (Jackson and Caldwell, 1993; Gross et al., 1995; Ryel et al., 1996; Farley and Fitter, 1999). Owing to the plasticity of their various plant traits, clonal plants can adapt to changing environments (Sultan, 1987; Hutchings and de Kroon, 1994). For example, plastic foraging, by organism searches or rootlets in areas where nutrient levels are higher than those in low-nutrient quality areas, can lead to more efficient use of heterogeneously distributed resources (Wijesinghe et al., 2001; Hutchings and John, 2004; de Kroon et al., 2005; Guo et al., 2011). In addition, as a physiological response to heterogeneity, organs of foraging plants (such as rhizomes, stolons, and corms) can take up nutrients at greater rates in nutrient-rich areas (Jackson and Caldwell, 1996; Hodge, 2004). The clonal plants can transport resources through connecting spacers among connected ramets in homogeneous or heterogeneous resources habitats (Alpert, 1999; He et al., 2011).
Besides resources heterogeneity, clonal plants are exposed to many other environmental stressors such as plant density (Fransen et al., 2001; Li et al., 2017). Plant density has a significant effect on the growth and reproduction performance of individual, population structure, and competitive relationship (Grace and Tilman, 1990; Stevens and Carson, 1999). The effect of intraspecific competition depends on the plant density (Antonovics and Levin, 1980). In addition, intraspecific competition may be affected by resource heterogeneity (Day et al., 2003b; Wang Y.J. et al., 2016). Several studies reported that the effects of resource heterogeneity on intraspecific competition led to changes in competitive intensity under heterogeneous distribution of a single resource (Day et al., 2003b; Hagiwara et al., 2010). For example, light heterogeneity significantly increased the intraspecific competition intensity of Duchesnea indica (Wang et al., 2012).
The relative nutrient concentrations in different soil areas (hereafter referred to as “soil nutrient heterogeneity”) can determine the extent to which plants concentrate more nutrient-absorbing organs in areas where nutrients are high; in addition, the efficient forage for nutrients in high-nutrient quality areas may lead to increased biomass, ramets and root production in heterogeneous environments compared to homogeneous environments that have the same amount of nutrient supply (Gersani et al., 1998; Fransen et al., 2001; Day et al., 2003b). Therefore, soil nutrient heterogeneity can influence interspecific and intraspecific competition (Day et al., 2003b; van der Waal et al., 2011; Mommer et al., 2012). Because plants prioritize the investment of relatively greater amounts of biomass in areas where nutrients are high in heterogeneous environments, competition between ramets and roots of neighboring plants may increase in intensity in smaller soil areas (Fransen et al., 2001; Day et al., 2003b). However, the results of other? experiments involving Festuca ovina (Day et al., 2003b), Hydrocotyle vulgaris (Dong et al., 2015) and Alternanthera philoxeroides (Zhou et al., 2012) have indicated that competition between plants is not influenced by soil nutrient heterogeneity or that this effect is temporary.
The temporal and spatial heterogeneity of water supplies (hereafter referred to as “heterogeneity of water supply”) clearly affects plant biomass allocation (Fay et al., 2003; Hagiwara et al., 2008), further altering community structure and composition (Maestre and Reynolds, 2007). For the same amount of water input, a stable water supply (hereafter referred to as “homogeneity of water supply”) can promote plant root systems to absorb water more efficiently and thus grow larger (Novoplansky and Goldberg, 2001; Hagiwara et al., 2012). In contrast, many plants exhibit negative biomass growth under conditions of heterogeneous water supply because those plants compensate for periodic water shortages by greater investment in roots, thus they have less to invest in other parts (Novoplansky and Goldberg, 2001; Fay et al., 2003; Hagiwara et al., 2010, 2012). In addition, spatial heterogeneity or temporal variation in water availability can alter intraspecific competition of Perilla frutescens (Hagiwara et al., 2010) and Iris japonica (Wang Y.J. et al., 2016).
Most studies on resource heterogeneity have investigated the response of only one species or the entire community by manipulating a single resource, e.g., nutrients, water, or light (Fransen et al., 2001; Day et al., 2003b; Fay et al., 2003; Moore and Franklin, 2012; Dong et al., 2015). Few studies have tested the heterogeneity of two resources affects intraspecific competition among clonal plants (Wang et al., 2012; Wang Y.J. et al., 2016), as the effects of resource heterogeneity on the relationships between plants may be altered by the supply patterns of other resources (Maestre and Reynolds, 2007).
Thus, we investigated the effects of heterogeneity in soil nutrients and water supply on the growth of both individual plants and the entire population under a variety of plant densities, as a single plant or a population at different densities usually experience both types of resource heterogeneity in their natural habitats. To test the responses of clonal plants to soil heterogeneity and water heterogeneity at different plant densities, we conducted a greenhouse experiment involving clonal plants of the rhizomatous species B. yagara (Ohwi).
Specifically, we addressed the following questions:
(a) Does the soil nutrient heterogeneity and heterogeneity of water supply affect the biomass accumulation in B. yagara?
(b) How do morphological traits of B. yagara respond to resource heterogeneity?
(c) Is the intensity of intraspecific competition of B. yagara affected by resource heterogeneity?
Materials and Methods
The Species
Bolboschoenus yagara (Ohwi) is a perennial clonal herb in the Cyperaceae family; this species develops underground rhizomes that terminate in a globose tuber (Board, 2010; Hroudová et al., 2014). Plants of this species occur in wet habitats such as swamps and wetlands and are distributed mainly in the northeastern, northwestern and southwestern regions of China (Board, 2010).
Experimental Design
On January 5, 2015, corms of B. yagara were obtained from mono-populations in a riparian area of Liangzi Lake, Hubei Province, China (30°05′–30°18′N, 114°21′–114°39′E). The corms were sprouted in sandy clay before the experiment setup. On April 1, 2015, 312 morphologically identical plants (without branches, height: approximately 12 cm; corm diameter: 0.91 ± 0.02 cm) were selected for the experiment described below, and 30 plants were randomly selected to measure their initial dry biomass (initial biomass: mean ±SE, 0.41 ± 0.02 g; corm biomass: 0.29 ± 0.02 g). The experiment involved a three-way factorial design. The first factor involved the pattern of water supply: homogeneous (800 ml of water daily) or heterogeneous (4 L of water every 5 days) water was supplied to each container, and the total amount of water provided was kept constant throughout the experimental period. Eight hundred milliliters equated to soil saturation, as measured by a soil moisture probe (SIN-TN8, Hangzhou, Liance Instrument, China). The environmental parameters of the water were as follows: total nitrogen (TN) concentration = 0.63 ± 0.009 mg.L−1; total phosphorus (TP) concentration = 0.04 ± 0.002 mg.L−1; pH = 8.55 ± 0.013; and salinity (SAL) = 0.09 ± 0.002 ppt [mean ±SE, measured by a YSI Professional Plus water quality meter (YSI Inc., Yellow Springs, OH, United States)]. The second factor involved the following four plant density treatments: one, two, four, or six plants per container. The third factor involved the substrate type. The first substrate represented the heterogeneous soil treatment. For this treatment, containers (70 cm long × 50 cm wide × 47 cm deep) were divided into four areas (35 cm long × 25 cm wide) (Figure 1): two areas were filled with clay (TN = 3.05 ± 0.05 mg.g−1; TP = 1.33 ± 0.03 mg.g−1; organic matter content = 60.67 ± 1.01 mg.g−1), and other two were completely filled with sand (TN = 0.02 ± 0.002 mg.g−1; TP = 0.25 ± 0.011 mg.g−1; organic matter content = 0.75 ± 0.02 mg.g−1) [mean ±SE, measured by a Flash 2000 Organic Elemental Analyzer (Thermo Fisher Scientific Inc., United States), IL500 TP Automatic Analyzer (Hach Corp., Loveland, CO, United States), and a Multiwave 3000 device (Anton Paar Corp., Austria)]. The second substrate represented the homogeneous soil treatment. For this treatment, containers were filled with the same soil type (comprised of equal volumes of clay and sand), after which the soils were completely homogenized. The total concentration of soil nutrients was the same in all treatments. Therefore, 16 treatment combinations (two water supply patterns × two soil nutrition distribution types × four plant densities) existed, and each combination was replicated 8 times. The mean temperature and mean humidity in the greenhouse were 25.34 ± 2.55°C and 64.67 ± 5.02% (mean ±SE), respectively. The experiment lasted for 70 days (duration of the pattern of water supply)—from April 2nd to June 10th 2015.
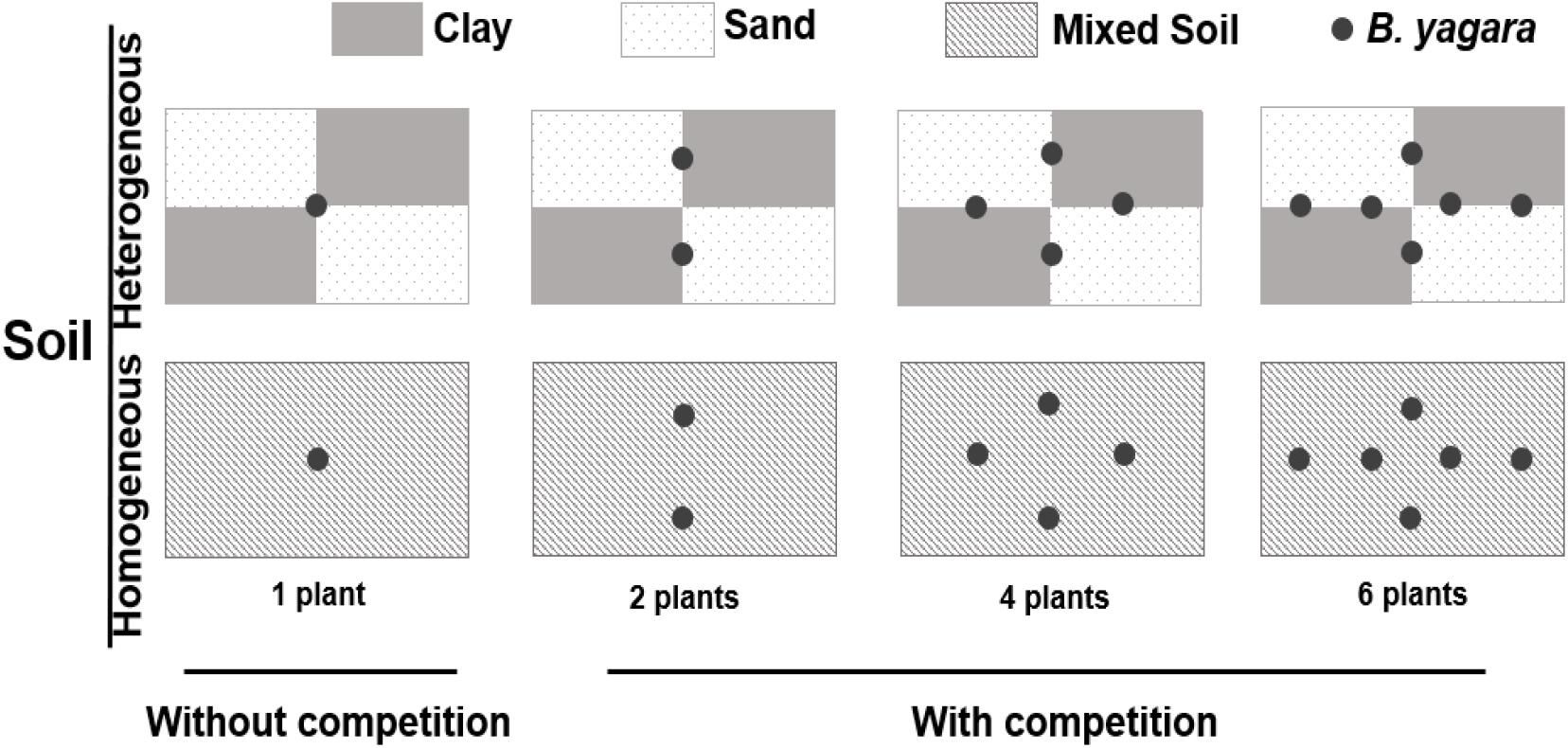
Figure 1. Experimental design. The experiment consisted of three factors. The first factor involved two patterns of water supply: a homogeneous (800 ml of water daily) and a heterogeneous (4 L of water every 5 days) water supply, with the total amount of water provided to each container kept constant throughout the experimental period. The second factor involved the intraspecific competition treatments: without competition (one plant per container) and with competition (two, four, or six plants per container). The third factor involved the substrate type. The first substrate represented the heterogeneous soil treatment, in which the containers were divided into four areas: two areas were filled with clay, and the other two were filled with true sand. The second substrate represented the homogeneous soil treatment, in which the containers were filled with the same completely homogeneous soil type. The total amount of soil nutrients was the same in all treatments.
Harvest and Measurements
The soil moisture (volumetric water content) and temperature were recorded with a soil moisture probe (SIN-TN8, Hangzhou, Liance Instrument, China) during the experimental period. The measurements were carried out daily before watering. To test for differences in the temporal heterogeneity of soil moisture between the two watering heterogeneity treatments, the soil moisture (Mm) values, soil moisture minimum (Mmin) values and soil moisture maximum (Mmax) values were measured and recorded, and the temporal mean value of the relative soil moisture content was calculated (James et al., 2003; Hagiwara et al., 2010).
We used one-way ANOVA to test the effects of the water heterogeneity treatment on both the temporal variability in the relative soil moisture content and the mean variance during the 5-day cycle in different water treatments. The mean value of the relative soil moisture was not affected by water temporal heterogeneity (F = 1.215, P = 0.275); however, the variance in the relative soil moisture during the 5-day cycle was significantly different between the water homogeneity treatments and the water heterogeneity treatments (F = 38.626, P < 0.001).
At harvest, plant height, fresh weight, rhizome length, ramet number, corm number and corm diameter were measured and recorded. The B. yagara material was subsequently divided into aboveground (leaves and stems above the soil surface) and underground parts (roots, corms and rhizomes). All the separated parts were oven-dried at 70°C for at least 3 days to obtain dry weights. To further calculate the performance of B. yagara at different densities, the root-to-shoot (R/S) ratios were calculated (Perez-Harguindeguy et al., 2013) as:
The effects of soil nutrient distribution and watering regimen on the intensity of intraspecific competition were calculated by the log response ratio (LnRR) of biomass (Armas et al., 2004).
Bmono represents the total biomass in the absence of competition (i.e., solitary plant density treatment), and Bmix represents the average biomass of a plant per container in the presence of competition (i.e., multidensity treatment). The LnRR for each plant density treatment (2, 4, and 6) are calculated separately. The LnRR values are symmetrical around zero, and no ceiling is imposed on the maximum possible competition intensity (Goldberg et al., 1999; Weigelt and Jolliffe, 2003).
Data Analysis
We measured biomass and morphological traits and calculated the R/S ratio and LnRR on a per-initial-plant basis for each container. All data was transformed by log10 prior to analysis to meet the requirements for homoscedasticity and normality. The treatment effects on plant height, corm number, corm diameter, rhizome length, ramet number, total mass, the R/S ratio and the LnRR were analyzed via a three-way ANOVA. One-way ANOVA in conjunction with Duncan’s (P < 0.05) test for post hoc comparisons was used to investigate the differences in biomass and morphological traits as well as in the R/S ratio, and the LnRR between the soil nutrient heterogeneity and the heterogeneity of water supply combinations at each plant density. To investigate the treatment effects on the intensity of competition, the LnRR was analyzed via a three-way ANOVA at each density. All of the analyses were conducted using SPSS 22.0 (SPSS, Chicago, IL, United States).
Results
Biomass and Biomass Allocation
Both soil nutrient treatment (P = <0.001) and plant density (P = <0.001) significantly affected biomass, whereas water supply treatment (P = 0.351) did not (Table 1). The interactive effects between soil nutrient treatment and plant density (P = <0.001), and between water supply treatment, soil nutrient treatment and plant density significantly (P = <0.001) affected biomass (Table 1). The biomass was 37.5–55% larger under the homogeneous soil nutrient distribution than under the heterogeneous soil nutrient distribution in the one-, two- and four-plant density treatments, while the six-plant density treatment exhibited opposite results (Figure 2). The R/S ratio was significantly affected only by the density treatment (Table 1). The biomass allocation was not affected by soil nutrient heterogeneity at low plant densities (Figures 2E,F). However, the R/S ratios in the six-plant density treatments were greater under soil nutrient heterogeneity than under the soil nutrient homogeneity (Figures 2G,H). Also, there was no significant effect of water supply heterogeneity on plant biomass (Table 1).
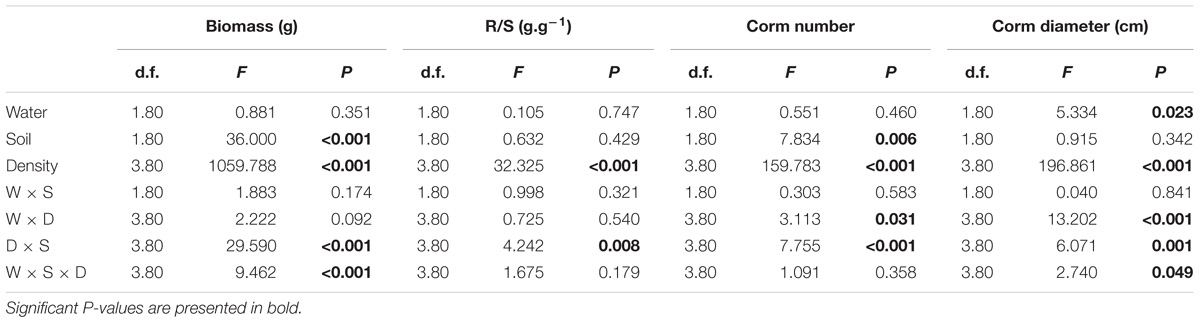
Table 1. Three-way ANOVAs of the effects of water heterogeneity (W), soil heterogeneity (S), and plant density (D) and their interaction on biomass, the R/S ratio, corm number and corm diameter of B. yagara.
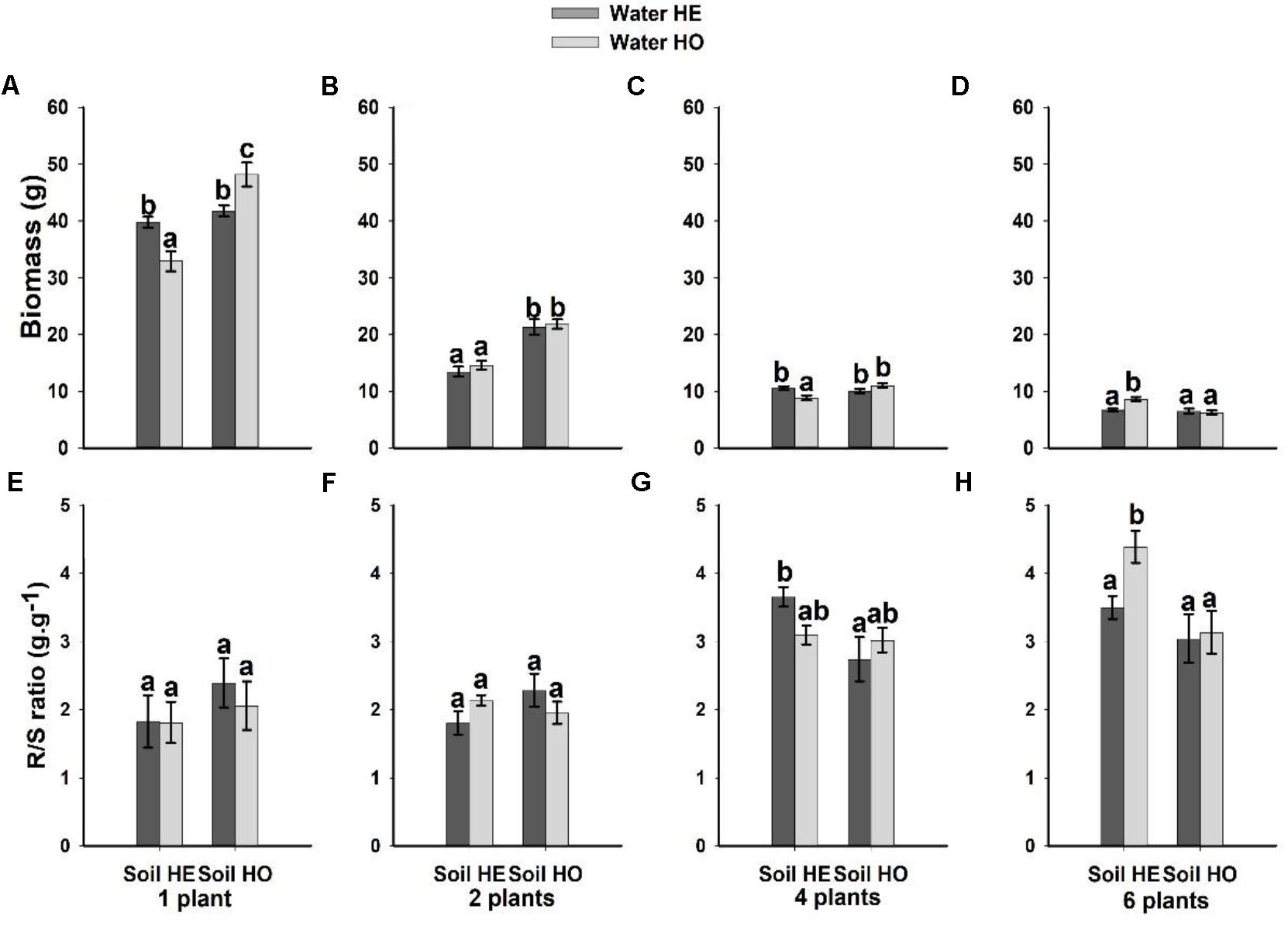
Figure 2. Effects of the heterogeneity of water supply and soil nutrients on the biomass (A–D) and R/S ratio (E–H) (±SE) of Bolboschoenus yagara at each plant density.
Morphological Traits
Compared with the water supply treatment the soil nutrient treatment significantly affected the morphological traits of B. yagara at low plant densities, although plant height and rhizome length were unaffected (Table 2 and Figures 3, 4). There were significant interactive effects between water supply treatment, soil nutrient treatment and plant density on corm diameter (Table 1). For example, compared with the soil nutrient heterogeneity treatment, the soil nutrient homogeneity treatment significantly increased the corm number, corm diameter and ramet number at low plant densities (Figures 3A,B,F, 4E,F). However, compared with the soil nutrient treatment, the water supply treatment significantly affected the morphological traits of B. yagara at high plant densities (Tables 1, 2). For example, with the exception of plant height, compared with the homogeneous water supply treatment, the heterogeneous water supply treatment significantly increased the corm number, corm diameter, rhizome length and ramet number of B. yagara at the four-plant density; however, compared with the heterogeneous water supply treatment, the homogeneous water supply treatment significantly increased the corm number, corm diameter, rhizome length, plant height, and ramet number of B. yagara at the six-plant density (Figures 3C,D,G,H,I, 4D,G,H).
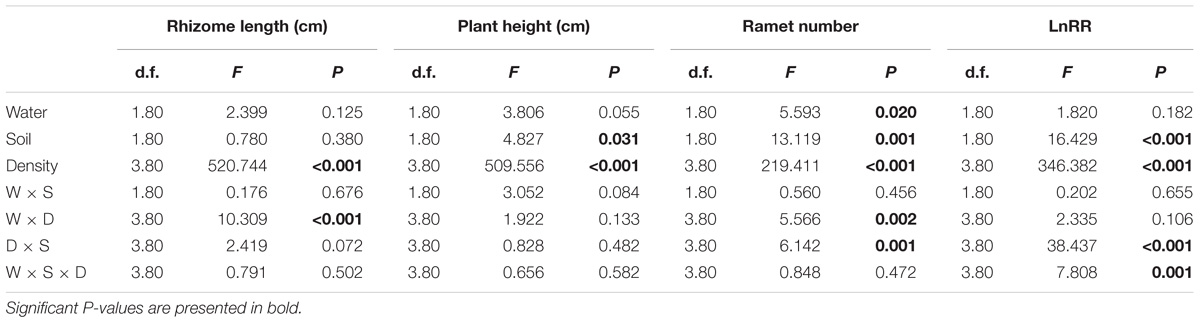
Table 2. Three-way ANOVAs of the effects of water heterogeneity (W), soil heterogeneity (S), and plant density (D) and their interaction on rhizome length, plant height, ramet number, and LnRR of B. yagara.
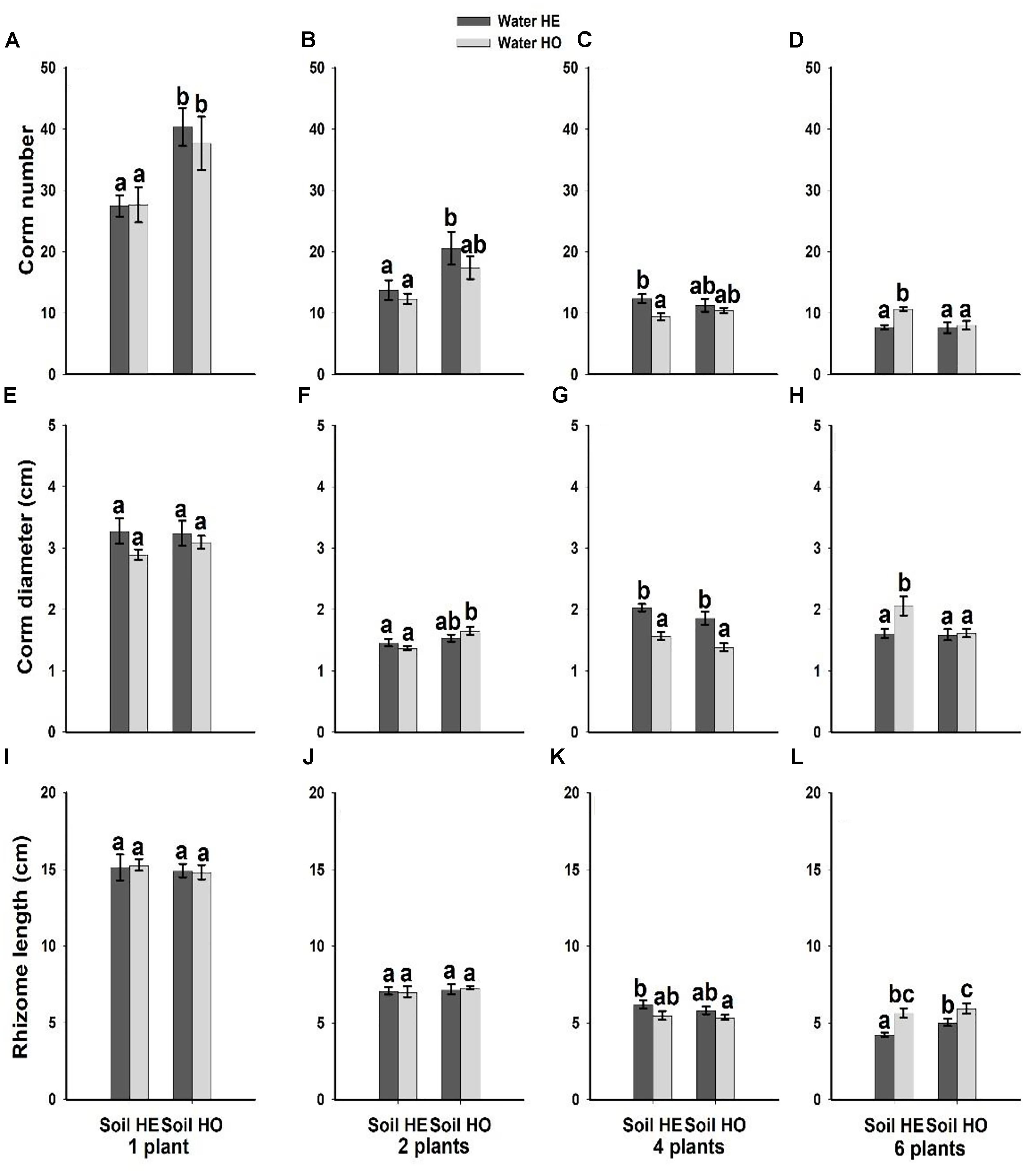
Figure 3. Effects of the heterogeneity of water supply and soil nutrients on the corm number (A–D), corm diameter (E–H) and rhizome length (I–L) (±SE) of B. yagara at each plant density.
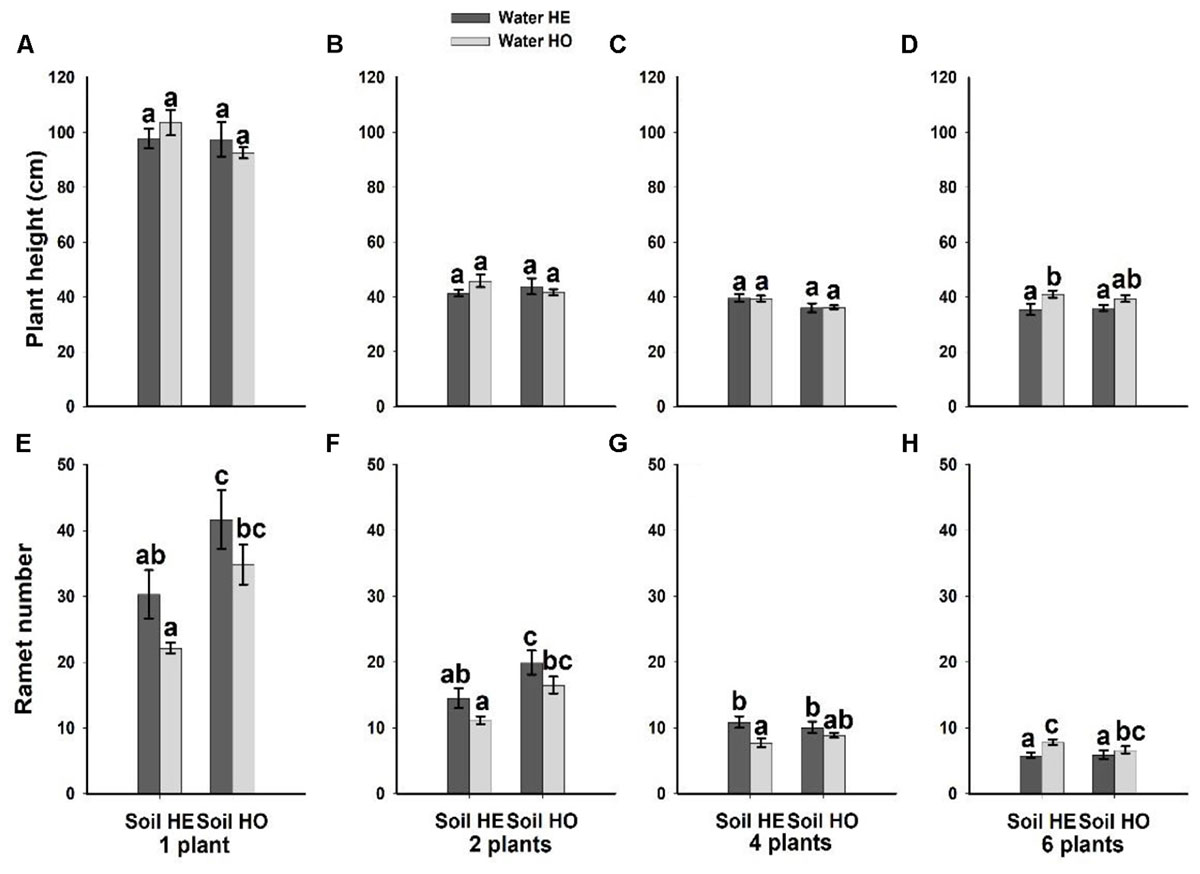
Figure 4. Effects of the heterogeneity of water supply and soil nutrients on the plant height (A–D) and ramet number (E–H) (±SE) of B. yagara at each plant density.
Intensity of Competition
Compared with the water supply treatment, the soil nutrient treatment significantly affected the LnRR (Table 2). The interactive effects between water supply treatment, the soil nutrient treatment and plant density significantly affected the LnRR (Table 2). Compared with the soil nutrient homogeneity treatment, the soil nutrient heterogeneity treatment significantly increased the LnRR of the biomass at the two- and four-plant densities (Figures 5A,B). However, the opposite results occurred at the highest, six-plant density treatment (Figure 5C). These results mean that competition was more severe as plant density increased and was significantly and more strongly affected by the soil substrate heterogeneity than by the water supply heterogeneity.
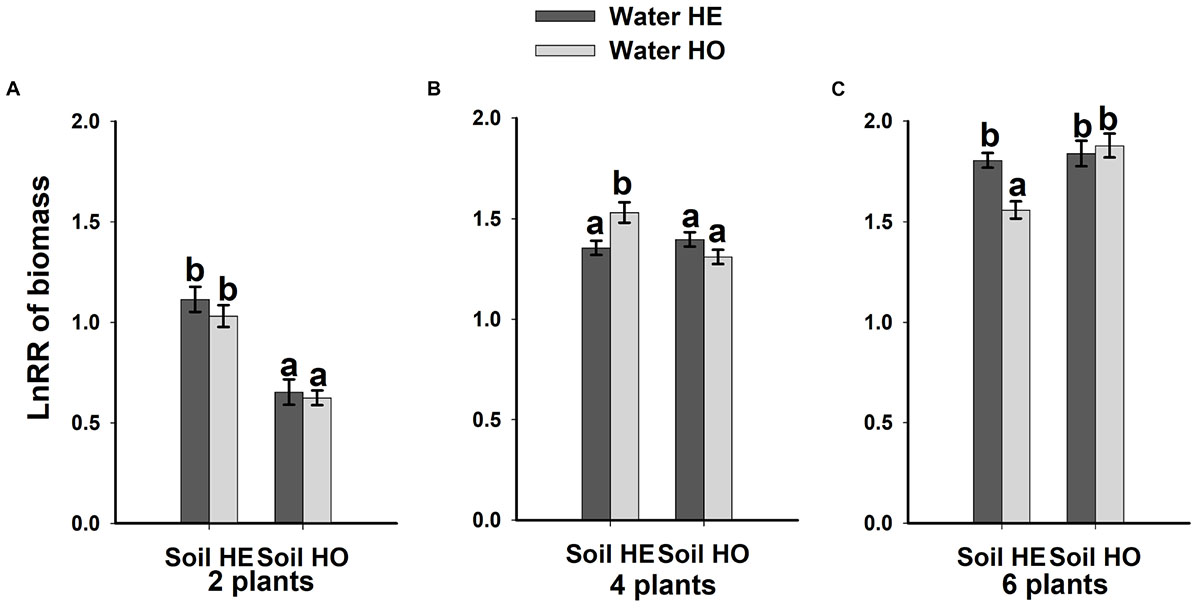
Figure 5. Competitive intensity as measured by the log response ratio (LnRR) of the biomass (A–C) (±SE) of B. yagara in response to heterogeneity of water supply and soil nutrients.
Discussion
Biomass and Biomass Allocation
The results of several previous experiments have shown that some plant species accumulate greater biomass under heterogeneous conditions than under homogeneous conditions, given the same total concentration of available nutrients (Hutchings and Wijesinghe, 2008; García-Palacios et al., 2011). However, in the present experiment, plant biomass was greater in the homogeneous soil nutrient treatment than in the heterogeneous soil nutrient treatment (Figures 2A–H and Table 1). These patterns may result from the homogeneous conditions in which the nutrients are evenly distributed, which is therefore more conducive to plant growth (Maestre and Reynolds, 2007; Dong et al., 2012; Hagiwara et al., 2012), and partly due to physiological integration that allows ramets to share resources with other ramets (Dong et al., 2015). Plant density significantly affected the growth of B. yagara, as the average biomass of the plants gradually decreased as the density increased (Figures 2A–D and Table 1). These findings indicated that both the existence of intraspecific competition among plants and the competition intensity increased as the plant density increased, because of both the density-dependent effect attributable to increased numbers of competitors and the increased effect of competition on individuals in single-species plant populations (Antonovics and Levin, 1980; Watkinson, 1980).
Plants grow larger under a more homogeneous water supply than under a more heterogeneous water supply because they can take up water more consistently under homogeneous conditions that the low variability in resources availability, thereby allowing the plants to increase their growth performance continuously (Novoplansky and Goldberg, 2001; Hagiwara et al., 2010, 2012). In the present study, compared with the heterogeneous conditions, the homogeneous of water supply clearly led to more plant biomass when the plants grew in isolation (Figure 2A). In addition, we found that the heterogeneous of water supply did not affect B. yagara biomass accumulation, which may be because B. yagara is more sensitive to soil nutrients treatment than to water supply treatment.
Previous experiments have shown that some plant species employ morphological specialization and physiological responses to heterogeneity to place more nutrient-absorbing organs (e.g., roots or ramets) in nutrient-rich areas to forage efficiently for heterogeneously distributed nutrients (de Kroon et al., 2005; Gao et al., 2012). However, the R/S ratio of B. yagara increased only at higher plant densities under soil nutrient heterogeneity treatment (Figures 2G,H and Table 1). As the density increased, the plants were more likely to encounter resources deficits. Thus plants invested more in underground part rather than aboveground part to acquire resources (Day et al., 2003b; Hagiwara et al., 2010, 2012; van der Waal et al., 2011).
Morphological Traits
Soil space decreased as the planting density increased, which caused the effect of soil nutrient heterogeneity to gradually diminish. Thus, B. yagara is more sensitive to water deficit at high plant density, and plants can alter their morphological characteristics according to the external environment. For example, plants have been shown to alter the length and angle of their spacers (includes stolon, rhizomes and corms, etc.), and their number and distribution of ramets (Hutchings et al., 2003; de Kroon et al., 2005; Gao et al., 2012).
Owing to high morphological plasticity, clonal plants generally respond positively to resource heterogeneity (Eilts et al., 2011; Zhou et al., 2012; Liu et al., 2017). For example, except at the 4-plant density, the B. yagara plants in the present study responded positively to resource homogeneity. That’s probably because the low variability in resource availability under the homogeneous conditions allowed the plants to absorb resources steadily, effectively improving their growth performance (Saeed and El-Nadi, 1998; Novoplansky and Goldberg, 2001; Maestre and Reynolds, 2007; Dong et al., 2012; Hagiwara et al., 2012). Overall, these results are consistent with other experiments that positive foraging responses to resource heterogeneity may not always be adaptive (Roiloa and Retuerto, 2006; Dong et al., 2015) and may be temporary (Day et al., 2003a,b).
Intensity of Competition
Our results demonstrated that, compared with the heterogeneity of water supply, soil nutrient heterogeneity significantly affected the intraspecific competition of B. yagara. For example, soil nutrient heterogeneity increased the intraspecific competition at the two- and four-plant densities (Figures 5A,B). One explanation is that, to efficiently take up heterogeneously distributed resources, clonal plants place more nutrient-absorbing organs in nutrient-rich areas in heterogeneous environments. Also, the roots of neighboring plants would proliferate in nutrient-rich areas (de Kroon et al., 2005; Gao et al., 2012), thus competition becomes more severe under heterogeneous soil nutrient conditions than under homogeneous ones (Fransen et al., 2001; Day et al., 2003b). However, in the present study, heterogeneous soil nutrient conditions had no effect on the intensity of intraspecific competition at the six-plant density under a heterogeneous water supply. Other experiments have also shown that soil nutrient heterogeneity does not alter intraspecific competition at the container level for Poa pratensis (Maestre et al., 2006), Achillea millefolium (Rajaniemi, 2011), A. philoxeroides (Zhou et al., 2012) or H. vulgaris (Dong et al., 2015). These results may have been observed because resource heterogeneity can significantly affect plant competition when individuals are not genetically identical (Day et al., 2003b; Zhou et al., 2012). Other may be due to high resource depletion rate in the high density population, the nutrient-rich patches might gradually decline to the same level of suitability as the nutrient-poor patches, and then lead to high density population less sensitive response to soil nutrient heterogeneity (Roiloa and Retuerto, 2006; Dong et al., 2015). Thus, heterogeneity in soil nutrient availability has different effects on the intensity of intraspecific competition of B. yagara at different densities.
Conclusion
We found that plants respond differently to environmental heterogeneity with respect to the supply of two covariable resources at different plant densities. The soil nutrient treatment significantly influenced the biomass and intraspecific competition of B. yagara. However, only the water supply treatment influenced the morphological traits of B. yagara at high plant densities, and heterogeneity of water supply had little impact on intraspecific competition. In addition, the interactive effect of soil nutrient heterogeneity and heterogeneity of water supply had no significant effect on the growth performance and competition relationship of B. yagara. Therefore, B. yagara was more sensitive to soil nutrient heterogeneity than to heterogeneity of water supply. Spatial or temporal heterogeneity in soil nutrient distribution and water supply patterns may be highly important with respect to the growth performance and population structure of clonal plants (Hutchings et al., 2003; Wang T. et al., 2016; Wang Y.J. et al., 2016; You et al., 2016). The ecological effects of resource heterogeneity should be investigated further due to various ecological factors (temperature, light, and humidity) that affect the growth performance of clonal plants. In addition, we should investigate how pulses of resource availability influence growth performance at individual, population, and community levels, because resource pulses provides opportunities to understand the dynamics of natural systems.
Author Contributions
CL and DY designed the experiment and edited the manuscript text. HY and NS performed the experiment. HY and NS wrote the manuscript text and executed the technical assays and statistical analysis. All authors reviewed the manuscript.
Funding
The authors gratefully acknowledge funding support from the Special Foundation of National Science and Technology Basic Research (2013FY112300) and the Major Science and Technology Program for Water Pollution Control and Treatment (2015ZX07503-005).
Conflict of Interest Statement
The authors declare that the research was conducted in the absence of any commercial or financial relationships that could be construed as a potential conflict of interest.
The handling Editor is currently co-organizing a Research Topic with one of the authors CL and confirms the absence of any other collaboration.
Acknowledgments
We greatly appreciate the assistance of Y. Wang and L. G. Wang for the valuable work in the field and the laboratory.
References
Alpert, P. (1999). Clonal integration in Fragaria chiloensis differs between populations: ramets from grassland are selfish. Oecologia 120, 69–76. doi: 10.1007/s004420050834
Antonovics, J., and Levin, D. A. (1980). The ecological and genetic consequences of density-dependent regulation in plants. Annu. Rev. Ecol. Syst. 11, 411–452.
Armas, C., Ordiales, R., and Pugnaire, F. I. (2004). Measuring plant interactions: a new comparative index. Ecology 85, 2682–2686. doi: 10.1890/03-0650
Day, K. J., Hutchings, M. J., and John, E. A. (2003a). The effects of spatial pattern of nutrient supply on the early stages of growth in plant populations. J. Ecol. 91, 305–315. doi: 10.1046/j.1365-2745.2003.00763.x
Day, K. J., John, E. A., and Hutchings, M. J. (2003b). The effects of spatially heterogeneous nutrient supply on yield, intensity of competition and root placement patterns in Briza media and Festuca ovina. Funct. Ecol. 17, 454–463. doi: 10.1046/j.1365-2435.2003.00758.x
de Kroon, H., Huber, H., Stuefer, J. F., and Van Groenendael, J. M. (2005). A modular concept of phenotypic plasticity in plants. New Phytol. 166, 73–82. doi: 10.1111/j.1469-8137.2004.01310.x
Dong, B. C., Wang, J. Z., Liu, R. H., Zhang, M. X., Luo, F. L., and Yu, F. H. (2015). Soil heterogeneity affects ramet placement of Hydrocotyle vulgaris. J. Plant Ecol. 8, 91–100. doi: 10.1093/jpe/rtu003
Dong, C. X., Lu, Y. L., Zhu, Y. Y., Zhou, Y., Xu, Y. C., and Shen, Q. R. (2012). Effect of homogeneous and heterogeneous supply of nitrate and ammonium on nitrogen uptake and distribution in tomato seedlings. Plant Growth Regul. 68, 271–280. doi: 10.1007/s10725-012-9715-1
Eilts, J. A., Mittelbach, G. G., Reynolds, H. L., and Gross, K. L. (2011). Resource heterogeneity, soil fertility, and species diversity: effects of clonal species on plant communities. Am. Nat. 177, 574–588. doi: 10.1086/659633
Farley, R., and Fitter, A. (1999). Temporal and spatial variation in soil resources in a deciduous woodland. J. Ecol. 87, 688–696. doi: 10.1046/j.1365-2745.1999.00390.x
Fay, P. A., Carlisle, J. D., Knapp, A. K., Blair, J. M., and Collins, S. L. (2003). Productivity responses to altered rainfall patterns in a C4-dominated grassland. Oecologia 137, 245–251. doi: 10.1007/s00442-003-1331-3
Fransen, B., de Kroon, H., and Berendse, F. (2001). Soil nutrient heterogeneity alters competition between two perennial grass species. Ecology 82,2534–2546.
Gao, Y., Xing, F., Jin, Y. J., Nie, D. D., and Wang, Y. (2012). Foraging responses of clonal plants to multi-patch environmental heterogeneity: spatial preference and temporal reversibility. Plant Soil 359, 137–147. doi: 10.1007/s11104-012-1148-0
García-Palacios, P., Maestre, F. T., and Gallardo, A. (2011). Soil nutrient heterogeneity modulates ecosystem responses to changes in the identity and richness of plant functional groups. J. Ecol. 99, 551–562. doi: 10.1111/j.1365-2745.2010.01765.x
Gersani, M., Abramsky, Z., and Falik, O. (1998). Density-dependent habitat selection in plants. Evol. Ecol. 12, 223–234. doi: 10.1023/a:1006587813950
Goldberg, D. E., Rajaniemi, T., Gurevitch, J., and Stewart-Oaten, A. (1999). Empirical approaches to quantifying interaction intensity: competition and facilitation along productivity gradients. Ecology 80, 1118–1131. doi: 10.2307/177059
Grace, J. B., and Tilman, D. (1990). Perspectives on plant competition. Bioscience 5, 3–7. doi: 10.2307/1311467
Gross, K. L., Pregitzer, K. S., and Burton, A. J. (1995). Spatial variation in nitrogen availability in three successional plant communities. J. Ecol. 83, 357–367. doi: 10.2307/2261590
Guo, W., Song, Y. B., and Yu, F. H. (2011). Heterogeneous light supply affects growth and biomass allocation of the understory fern Diplopterygium glaucum at high patch contrast. PLoS One 6:e27998. doi: 10.1371/journal.pone.0027998
Hagiwara, Y., Kachi, N., and Suzuki, J. I. (2008). Effects of temporal heterogeneity of watering on size of an annual forb, Perilla frutescens (Lamiaceae), depend on soil nutrient levels. Botany 86, 1111–1116. doi: 10.1139/B08-064
Hagiwara, Y., Kachi, N., and Suzuki, J. I. (2010). Effects of temporal heterogeneity of water supply on the growth of Perilla frutescens depend on plant density. Ann. Bot. 106, 173–181. doi: 10.1093/aob/mcq096
Hagiwara, Y., Kachi, N., and Suzuki, J. I. (2012). Effects of t temporal heterogeneity of water supply and nutrient levels on plant biomass growth depend on the plant’s relative size within its population. Ecol. Res. 27, 1079–1086. doi: 10.1007/s11284-012-0989-6
He, W. M., Alpert, P., Yu, F. H., Zhang, L. L., and Dong, M. (2011). Reciprocal and coincident patchiness of multiple resources differentially affect benefits of clonal integration in two perennial plants. J. Ecol. 99, 1202–1210. doi: 10.1111/j.1365-2745.2011.01848.x
Hodge, A. (2004). The plastic plant: root responses to heterogeneous supplies of nutrients. New Phytol. 162, 9–24. doi: 10.1111/j.1469-8137.2004.01015.x
Hroudová, Z., Zákravský, P., and Flegrová, M. (2014). The tolerance to salinity and nutrient supply in four European Bolboschoenus species (B. maritimus, B. laticarpus, B. planiculmis and B. yagara) affects their vulnerability or expansiveness. Aquat. Bot. 112, 66–75. doi: 10.1016/j.aquabot.2013.07.012
Hutchings, M., and de Kroon, H. (1994). Foraging in plants: the role of morphological plasticity in resource acquisition. Adv. Ecol. Res. 25, 159–238.
Hutchings, M. J., and John, E. A. (2004). The effects of environmental heterogeneity on root growth and root/shoot partitioning. Ann. Bot. 94, 1–8. doi: 10.1093/aob/mch111
Hutchings, M. J., John, E. A., and Wijesinghe, D. K. (2003). Toward understanding the consequences of soil heterogeneity for plant populations and communities. Ecology 84, 2322–2334. doi: 10.1890/02-0290
Hutchings, M. J., and Wijesinghe, D. K. (2008). Performance of a clonal species in patchy environments: effects of environmental context on yield at local and whole-plant scales. Evol. Ecol. 22, 313–324. doi: 10.1007/s10682-007-9178-4
Jackson, R., and Caldwell, M. (1993). The scale of nutrient heterogeneity around individual plants and its quantification with geostatistics. Ecology 74, 612–614. doi: 10.2307/1939320
Jackson, R., and Caldwell, M. (1996). Integrating resource heterogeneity and plant plasticity: modelling nitrate and phosphate uptake in a patchy soil environment. J. Ecol. 84, 891–903. doi: 10.2307/2960560
James, S. E., Pärtel, M., Wilson, S. D., and Peltzer, D. A. (2003). Temporal heterogeneity of soil moisture in grassland and forest. J. Ecol. 91, 234–239. doi: 10.1046/j.1365-2745.2003.00758.x
Li, F., Xie, Y., Yang, G., Zhu, L., Hu, C., Chen, X., et al. (2017). Interactive influence of water level, sediment heterogeneity, and plant density on the growth performance and root characteristics of carex brevicuspis. Limnologica 62, 111–117. doi: 10.1016/j.limno.2016.11.007
Liu, L., Alpert, P., Dong, B. C., Li, J. M., and Yu, F. H. (2017). Combined effects of soil heterogeneity, herbivory and detritivory on growth of the clonal plant Hydrocotyle vulgaris. Plant Soil 421, 429–437. doi: 10.1007/s11104-017-3476-6
Maestre, F. T., Bradford, M. A., and Reynolds, J. F. (2006). Soil heterogeneity and community composition jointly influence grassland biomass. J. Veg. Sci. 17, 261–270. doi: 10.1111/j.1654-1103.2006.tb02445.x
Maestre, F. T., and Reynolds, J. F. (2007). Amount or pattern? Grassland responses to the heterogeneity and availability of two key resources. Ecology 88, 501–511. doi: 10.1890/06-0421
Mommer, L., van Ruijven, J., Jansen, C., van de Steeg, H. M., and de Kroon, H. (2012). Interactive effects of nutrient heterogeneity and competition: implications for root foraging theory? Funct. Ecol. 26, 66–73. doi: 10.1111/j.1365-2435.2011.01916.x
Moore, J. E., and Franklin, S. B. (2012). Water stress interacts with early arrival to influence interspecific and intraspecific priority competition: a test using a greenhouse study. J. Veg. Sci. 23, 647–656. doi: 10.1111/j.1654-1103.2012.01388.x
Novoplansky, A., and Goldberg, D. E. (2001). Effects of water pulsing on individual performance and competitive hierarchies in plants. J. Veg. Sci. 12, 199–208. doi: 10.2307/3236604
Perez-Harguindeguy, N., Diaz, S., Garnier, E., Lavorel, S., Poorter, H., Jaureguiberry, P., et al. (2013). New handbook for standardised measurement of plant functional traits worldwide. Aust. J. Bot. 61, 167–234. doi: 10.1071/BT12225
Rajaniemi, T. K. (2011). Competition for patchy soil resources reduces community evenness. Oecologia 165, 169–174. doi: 10.1007/s00442-010-1710-5
Roiloa, S., and Retuerto, R. (2006). Small-scale heterogeneity in soil quality influences photosynthetic efficiency and habitat selection in a clonal plant. Ann. Bot. 98, 1043–1052. doi: 10.1093/aob/mcl185
Ryel, R. J., Caldwell, M., and Manwaring, J. (1996). Temporal dynamics of soil spatial heterogeneity in sagebrush-wheatgrass steppe during a growing season. Plant Soil 184, 299–309. doi: 10.1007/BF00010459
Saeed, I., and El-Nadi, A. (1998). Forage sorghum yield and water use efficiency under variable irrigation. Irrig. Sci. 18, 67–71. doi: 10.1007/s002710050046
Stevens, M. H. H., and Carson, W. P. (1999). Plant density determines species richness along an experimental fertility gradient. Ecology 80, 455–465. doi: 10.2307/176625
Sultan, S. E. (1987). “Evolutionary implications of phenotypic plasticity in plants,” in Evolutionary Biology, eds M. K. Hecht, B. Wallace, and G. T. Prance (Boston, MA: Springer), 127–178.
van der Waal, C., de Kroon, H., Heitkönig, I., Skidmore, A. K., van Langevelde, F., de Boer, W. F., et al. (2011). Scale of nutrient patchiness mediates resource partitioning between trees and grasses in a semi-arid savanna. J. Ecol. 99, 1124–1133. doi: 10.1111/j.1365-2745.2011.01832.x
Wang, P., Lei, J. P., Li, M. H., and Yu, F. H. (2012). Spatial heterogeneity in light supply affects intraspecific competition of a stoloniferous clonal plant. PLoS One 7:e39105. doi: 10.1371/journal.pone.0039105
Wang, T., Hu, J., Miao, L., Yu, D., and Liu, C. (2016). The invasive stoloniferous clonal plant Alternanthera philoxeroides outperforms its co-occurring non-invasive functional counterparts in heterogeneous soil environments–invasion implications. Sci. Rep. 6:38036. doi: 10.1038/srep38036
Wang, Y. J., Shi, X. P., Meng, X. F., Wu, X. J., Luo, F. L., and Yu, F. H. (2016). Effects of spatial patch arrangement and scale of covarying resources on growth and intraspecific competition of a clonal plant. Front. Plant Sci. 7:753. doi: 10.3389/fpls.2016.00753
Watkinson, A. (1980). Density-dependence in single-species populations of plants. J. Theor. Biol. 83, 345–357. doi: 10.1016/0022-5193(80)90297-0
Weigelt, A., and Jolliffe, P. (2003). Indices of plant competition. J. Ecol. 91, 707–720. doi: 10.1046/j.1365-2745.2003.00805.x
Wijesinghe, D. K., John, E. A., Beurskens, S., and Hutchings, M. J. (2001). Root system size and precision in nutrient foraging: responses to spatial pattern of nutrient supply in six herbaceous species. J. Ecol. 89, 972–983. doi: 10.1111/j.1365-2745.2001.00618.x
You, W. H., Han, C. M., Liu, C. H., and Yu, D. (2016). Effects of clonal integration on the invasive clonal plant Alternanthera philoxeroides under heterogeneous and homogeneous water availability. Sci. Rep. 6:29767. doi: 10.1038/srep29767
Keywords: resource heterogeneity, intraspecific competition, growth performance, clonal plant, population density
Citation: Yu H, Shen N, Yu D and Liu C (2019) Effects of Temporal Heterogeneity of Water Supply and Spatial Heterogeneity of Soil Nutrients on the Growth and Intraspecific Competition of Bolboschoenus yagara Depend on Plant Density. Front. Plant Sci. 9:1987. doi: 10.3389/fpls.2018.01987
Received: 12 May 2018; Accepted: 20 December 2018;
Published: 11 January 2019.
Edited by:
Katya E. Kovalenko, University of Minnesota Duluth, United StatesReviewed by:
Peng Yu, Universität Bonn, GermanyFei-Hai Yu, Taizhou University, China
Wei Xue, Netherlands Institute of Ecology (NIOO-KNAW), Netherlands
Copyright © 2019 Yu, Shen, Yu and Liu. This is an open-access article distributed under the terms of the Creative Commons Attribution License (CC BY). The use, distribution or reproduction in other forums is permitted, provided the original author(s) and the copyright owner(s) are credited and that the original publication in this journal is cited, in accordance with accepted academic practice. No use, distribution or reproduction is permitted which does not comply with these terms.
*Correspondence: Chunhua Liu, liuchh@163.com