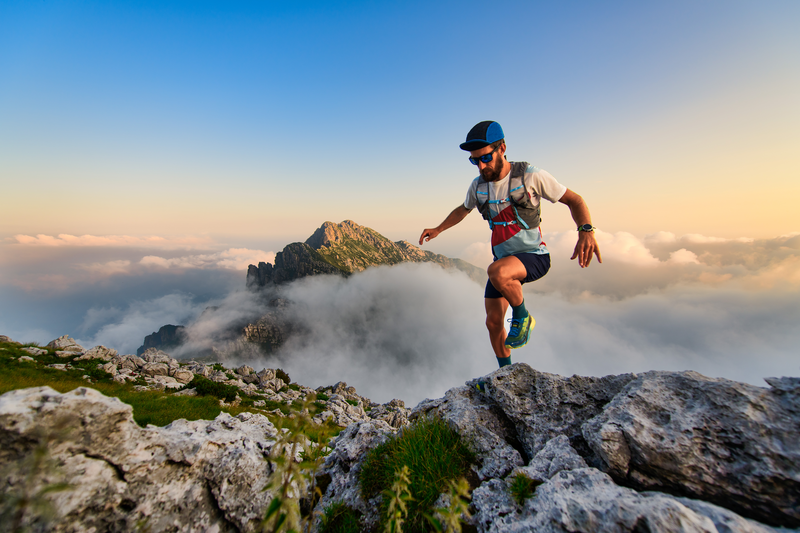
95% of researchers rate our articles as excellent or good
Learn more about the work of our research integrity team to safeguard the quality of each article we publish.
Find out more
ORIGINAL RESEARCH article
Front. Plant Sci. , 03 December 2018
Sec. Crop and Product Physiology
Volume 9 - 2018 | https://doi.org/10.3389/fpls.2018.01749
This article is part of the Research Topic Rediscovering Local Landraces: Shaping Horticulture for the Future View all 13 articles
Shallots are a valuable minor Allium crop, and are propagated vegetatively and maintained in home gardens across generations along the Croatian coast and island areas. Shallot landraces growing along the Croatian coast fall into three genotypes: Allium cepa Aggregatum group (2n = 2x = 16), A. × proliferum (Moench) Schard. (2n = 2x = 16), and A. × cornutum Clementi ex Vis. (2n = 3x = 24), among which A. × cornutum is the most widespread. The aim of this study was to differentiate shallot accessions collected from local farmers using morphological markers. Also, the chemical composition including phenolic content, phenolic profile, total antioxidant capacity, and mineral composition, of shallot accessions was compared with that of the local landraces of common onion, and with market available shallot and common onion cultivars. Based on morphological observations and using multivariate classification, shallot landraces were classified into three distinct groups. Properties, based on which A. × cornutum can be differentiated from A. cepa Aggregatum and A. × proliferum, are stamen morphology, stamen length, leaf and scape vegetative properties, number of bulbs in cluster, cluster mass, and bulb diameter. Flower diameter and flower pedicel length differentiate A. × cornutum and A. × proliferum from A. cepa Aggregatum. Significant variability was observed in the biochemical profiles across tested accessions. Compared with the commercial common onion cultivars, local shallot accessions have higher bulb N, P, and K content. The major phenolic compounds identified in shallots were quercetin-4′-glucoside and quercetin-3,4′-diglucoside. Additionally, several other minor phenolic compounds were also identified. Morphological and biochemical profiles were evaluated using Partial Least Square (PLS) analysis. Specific morphological traits and biochemical markers for possible species identification are proposed.
Allium is a taxonomically complicated genus with more than 750 species, and approximately 60 taxonomic groups at subgenera, sectional, and subsectional ranks (Ohri et al., 1998; Fritsch and Friesen, 2002; Block, 2010). Based on inflorescence morphology, Allium was once classified as Liliaceae and later as Amaryllidaceae (Block, 2010). Recently, molecular data have supported further subdivision into small monophyletic families (Fritsch and Friesen, 2002), and placement of Allium and its close relatives in the Alliaceae family (Takhtajan, 2009).
The origin of the Allium spp. is still somewhat a mystery and many botanists doubt the existence of Allium cepa as a wild plant (Pike, 1986). Domestication of Allium occurred more than 4000 years ago, with spread to Egypt, ancient China, and Persia (Fritsch and Friesen, 2002; Ansari, 2007; Cumo, 2015). Allium is currently widely distributed in Europe, Central Asia, North America, and India and shows complex morphological diversity (Stearn, 1992).
Allium cepa is one of the oldest cultivated vegetables and is currently the second most widely cultivated vegetable in the world after tomato (FAOSTAT, 2018). Other minor Allium species, of less economic importance than onion, are grown sporadically in restricted regions only, and were historically of greater importance (Fritsch and Friesen, 2002). The largest producers of shallots and similar minor Allium species are China and Japan, with more than 500,000 tons of shallot bulbs produced per year, followed by New Zealand, Mexico, Iran, Iraq, Cambodia, and Cameroon (FAOSTAT, 2018).
In Croatia, minor Allium species are cultivated by local farmers and households along the coastal areas of Istria, Kvarner, Dalmatia, and Dalmatian hinterland. They are generally propagated by bulbs and are closely related to common onions. Recently, Puizina (2013) proposed that shallots in Croatia could be divided into three genotypes based on vegetative and generative morphological characteristics: A. cepa Aggregatum (2n = 2x = 16), A. × proliferum (Moench) Schard (2n = 2x = 16), and A. × cornutum Clementi ex Vis. (2n = 3x = 24), among which A. × cornutum is the most widespread in the coastal area. Owing to morphological similarities, it is often difficult to distinguish the species in the field, requiring development of fast and reliable methods for discrimination of landraces to support breeding programs or for commercial exploitation.
Onions are rich in antioxidants, mainly quercetin and its glycosides, and are a major source of dietary flavonoids (Slimestad et al., 2007). In addition, flavonoids are responsible for the yellow or red color of onions (Ferioli and D’Antuono, 2016). Although these health-promoting compounds are ubiquitous in onion bulbs, a detailed chemical profile is required for identification, as the content of specific compounds can vary among Allium species or cultivars (Griffiths et al., 2002; Slimestad et al., 2007; Ferioli and D’Antuono, 2016).
Domesticated cultivars, local landraces, ecotypes, or wild edible hybrids are gaining interest, from both economic and nutritional standpoints. The basis for agricultural research, breeding programs, and crop improvement is assessment of plant genetic diversity (Fowler and Hodgkin, 2004; Govindaraj et al., 2015). In the recent years, effort is allocated toward identification and characterization of local landraces in order to preserve the genetic structure from erosion as well as to protect local agronomic production systems by means of agricultural, biological and chemical multidisciplinary approach (Jump et al., 2009; Siracusa et al., 2013; Ferioli and D’Antuono, 2016).
Minor Allium crops in Croatia belong to three genetically and morphologically different, vegetatively reproduced relatives of the common onion, A. cepa L. (Puizina, 2013). Shallots belonging to A. cepa Aggregatum are no longer considered to be a different species, but are classified in the common onion group, as A. cepa L. species (Fritsch and Friesen, 2002; Rabinowitch and Kamenetsky, 2002; Brickell et al., 2016).
In this study, shallot accessions collected along the Croatian coast and hinterland were evaluated for their morphological properties. Furthermore, chemical composition of these accessions was compared with that of local landraces of common onions and market-available shallot and common onion cultivars. The diversity observed for the tested traits may be useful for preservation of genetic variability in future breeding programs and to protect local agronomic production systems by means of agricultural, biological, and chemical multidisciplinary approach (Jump et al., 2009; Siracusa et al., 2013; Ferioli and D’Antuono, 2016).
Shallot landraces were collected from 2014 to 2017 across Croatia (Figure 1) as part of the National Program of Conservation and Sustainable Use of Plant Genetic Resources. Thirteen shallot landraces were collected along Croatian coastal area, from northern and central Istria, Kvarner, Dalmatia, and Dalmatian hinterland areas. The collected landraces were vegetatively propagated by underground bulbs except IPT023 which was propagated by aerial bulbils.
The field trial was established by the end of October 2016 at the Institute of Agriculture and Tourism in Poreč, Croatia (N 45°13′20.30″, E 13°36′6.49″). The shallot clusters consisted of 2–3 bulbs were planted at distance of 20 cm in row and 30 cm between rows. At least 40 clusters of each accession were planted. In addition to shallot landraces, local landraces of common onion were planted as transplants in the same field at the same time at the same planting density (Table 1). Before planting, NPK fertilizer (5:20:30) was incorporated in soil at 500 kg ha-1 and at begging of March N was applied (urea source) at a rate of 45 kg ha-1. The weeds were removed manually. The plants were grown without irrigation and according to common agricultural practices for onion growing (Lešić et al., 2004). The harvest started at begging of July when at least 50% of pseudo stems bent over for each accession.
TABLE 1. Vegetative and generative morphological qualitative descriptors1 of flowering shallot accessions.
Commercial cultivars of common onion were purchased at a local market in July 2017, for comparison of biochemical characteristics with those of the accessions in our collection. The cultivars Redwing (red onion), Legend (yellow onion), and Lang Prince de Bretagne (long bulb shallot) were included in the study.
During the vegetative period, the accessions were evaluated according to descriptors for generative organs provided by (Puizina, 2013) and a list of ECP/GR descriptors for vegetatively propagated Allium species (IPGRI et al., 2001). Of the 13 coastal shallot accessions collected, only 10 entered reproductive phases, with a flowering period from June 10th to 14th, 2017. These 10 accessions were eligible for morphological differentiation analysis based on flower characteristics. In total, we used 16 qualitative and 10 quantitative plant descriptors for characterization of landraces.
Plants were harvested at maturity and sampled for further analyses after a month of curing in the shade.
Shallot bulbs were dried in an oven with circulating air at 70°C for 48 h, then ground for nutrient analysis. Powdered material (0.5 g) was obtained from each sample, subjected to dry washing in a muffle furnace at 550°C for 5 h, and used to extract P, K, Ca, Mg, Zn, Mn, and Cu after dissolving in 2 mL HCl. P concentration was determined by the vanadate-molybdate yellow color method (Chapman and Pratt, 1961) using a spectrophotometer at 420 nm. K concentrations were measured using flame photometry (Model 410; Sherwood Scientific Ltd., Cambridge, United Kingdom), while Ca, Mg, Zn, Mn, and Cu were determined by atomic absorption spectrometry (Spectraa 220; Varian Inc., Palo Alto, CA, United States). Total N concentration was measured by the micro-Kjeldahl digestion system (Kjeltec system 1026, Foss Inc., Hilleroed, Denmark).
Extraction of phenolic compounds was performed by ultrasound-assisted extraction in 80% methanol. Briefly, 2 g of sample was homogenized with a rotary bearing mill (Model HOMEX 6, Bioreba AG, Reinach, Switzerland) in 9.5 mL of 80% methanol and 0.5 mL NaCl. The mixture was sonicated for 30 min and left to macerate for 4 h at 20°C. The mixture was filtered and centrifuged at 6000 × g for 15 min. The resulting supernatant was collected and diluted to a final volume of 10 mL with extraction solvent. The solution was filtered through a 0.45 μm filter prior to analysis.
Total phenolic content (TPC) was evaluated by the Folin-Ciocalteu assay (Singleton and Rossi, 1965). Sample extracts (0.2 mL) were mixed with 1.4 mL of freshly diluted 0.2 M Folin-Ciocalteu reagent in water. Sodium carbonate (1.4 mL, 6% in distilled water) was added after 1 min and the mixture was vortexed. The reaction mixture was incubated at room temperature and the absorbance of the mixture was read at 750 nm on a UV/Vis spectrophotometer (Model UV-1800, Shimadzu Corporation, Kyoto, Japan). TPC was standardized against gallic acid and expressed as mg of gallic acid equivalents per g sample in fresh weight (FW).
Chromatographic separations were performed by reversed-phase HPLC. The HPLC instrument consisted of a solvent delivery module (Model ProStar 230, Varian Inc., Palo Alto, CA, United States), a column valve module (Model CVM 500, Varian Inc., Palo Alto, CA, United States), UV/Vis detector (Model ProStar 310, Varian Inc., Palo Alto, CA, United States), and a 5 μm RP C18 column (250 mm × 4.6 mm) (Chromsep Omnispher, Varian Inc., Palo Alto, CA, United States). Gradient elution with solvent A (0.1% formic acid in water) and solvent B (0.1% formic acid in methanol) was achieved using the following program: 90% to 25% A, 0 to 55 min; 25% to 2% A, 55 to 57 min; 2% A, 57 to 69 min. Column temperature was held at 30°C, injection volume was 20 μL, and flow rate was 1.0 mL/min. Individual phenolic compounds were identified and quantified using authentic reference standards of quercetin-3,4′-glucoside, quercetin, isoquercetin, chlorogenic, vanillic, and ferulic acids. Quercetin-4′-glucoside was identified using previously published data and quantified by comparing its relative area with the relative area of the isoquercetin (quercetin-3-glucoside) standard.
Total antioxidant capacity of various Allium accessions was evaluated spectrophotometrically (Model UV-1800, Shimadzu Corporation, Kyoto, Japan) by Ferric Reducing Ability of Plasma (FRAP) (Benzie and Strain, 1996) and 2,2-diphenyl-1-picrylhydrazyl (DPPH) radical scavenging assays (Brand-Williams et al., 1995). FRAP values were obtained by analyzing a mixture of 1 mL of sample with 2 mL of freshly prepared FRAP reagent at 593 nm after 4 min of reaction time. Results were expressed as mM of Fe2+ equivalents per g sample in FW. DPPH radical scavenging activity was determined by analyzing a mixture of 1 mL of the sample with 2 mL of 0.1 mM DPPH radical at 517 nm after 30 min in darkness. The results were expressed as mM of Trolox equivalents per g sample in FW.
The morphological description of flowering accessions was conducted on 10 plants per accession as recommended by the IPGRI et al. (2001). Analysis of macro- and micro-elements, phenolic content, phenolic compounds, and antioxidant capacity were performed in triplicate. Data were analyzed by analysis of variance (ANOVA) and Partial Least Square (PLS) analysis using Statistica 13.3 (Tibco, Inc). Significant differences were determined at p ≤ 0.05 and homogenous group means were compared by Tukey’s HSD test.
Similarly to Principal Components Regression (PCR), the scope of PLS regression is to form new components that capture most information in the independent variables that is useful for predicting dependent variables, while reducing the dimensionality of the dataset (Garthwaite, 1994). In addition to the information contained in the independent variables, PLS also uses information from dependent variables in the formation of components. As such, PLS is of particular use when there are many independent variables and comparatively little data (Garthwaite, 1994; Helland, 2014). The advantage of PLS regression lies in its exploratory potential. Here the method was applied as an exploratory tool for identification of variables critical in the discrimination between local shallot landraces.
During the growing season, we observed differences in plant habit (Figures 2A–C) and type of inflorescence (Figures 2D–F). Therefore, vegetative and generative plant morphological descriptors were used to describe and group the 10 flowering shallot accessions. Morphological plant descriptors are summarized in Tables 1, 2, and accessions are denoted by their respective species.
FIGURE 2. Flowering plant in the field: (A) Allium × cornutum, (B) Allium × proliferum, (C) Allium cepa Aggregatum. Inflorescence: (D) Allium × cornutum, (E) Allium × proliferum, (F) Allium cepa Aggregatum. Flower: (G,H) Allium × cornutum, (I) Allium × proliferum, (J) Allium cepa Aggregatum. Underground bulbs: (K) Allium × cornutum, (L) Allium × proliferum, (M) Allium cepa Aggregatum.
TABLE 2. Vegetative and generative morphological quantitative descriptors1 of flowering shallot accessions.
The majority of accessions belonged to A. × cornutum and were characterized by yellow–green foliage color, intermediate foliage attitude, narrow leaf diameter, and the presence of fewer than 30 bulbils in the inflorescence (Table 1). Scapes of accessions belonging to the A. × cornutum group were conic, hollow, simple; round inflorescence with bulbils; perianth purple-green, with pistils taller than stamens; and anthers yellow (Figures 2D,G).
A. × proliferum accessions were distinguished from A. × cornutum and A. cepa Aggregatumg by green foliage color, erect foliage attitude, medium broad leaf diameter (Table 1), prizmic inflorescence, campanulate green striped perianth, and green anther color (Figures 2E,I). Scape morphology in A. × proliferum was gigantic in size, carrying bulbils in several levels (Figure 2B), which differed greatly from the other two species.
Allium cepa Aggregatum accessions were characterized by circular to concave leaf cross sections; absence of bulbils in inflorescence; star-like, green striped perianth; pistils lower than stamens; green stamens and anthers; and fertile flowers (Figures 2F,J and Table 1).
Although bulbs should be the main organ used to differentiate accessions, their shapes were variable and ranged from elongated oval, broad elliptic, globose, and broad oval to broad elliptic in each of the described accessions (Figures 2K–M and Table 1).
Quantitative morphological characteristics were significantly different among the accessions for all traits studied, except bulb diameter (Table 2). Quantitative differences among Allium groups were not as clear as qualitative differences. The gigantic nature of A. × proliferum accession (IPT023) was characterized by greater leaf diameter, cluster mass, scape length, and diameter, whereas the bulb number per cluster was generally smaller (Table 2).
Figures 3A,B present PLS analysis of 10 flowering shallot accessions using qualitative and quantitative morphological descriptors presented in Tables 1, 2, respectively. Based on inflorescence (QL11) and perianth (QL12) morphology, all three groups of shallot species could be distinguished from each other (Figures 2D–J, 3A).
FIGURE 3. Partial least square (PLS) analysis of local shallot accessions based on (A) qualitative morphological descriptors (QL1 to QL16), (B) quantitative morphological descriptors (QN1 to QN10), (C) nutrient and mineral profiles (N1 to N8), and (D) phenolic compounds (P1 to P7).
A. × cornutum shallot accessions could be distinguished from A. cepa Aggregatum and A. × proliferum based on the degree of leaf waxiness (QL5), flower number in umbel (QL10), stamen morphology (QL14), and anther color (QL15), as shown in Figure 3A.
The Aggregatum group of shallot accessions could be distinguished from the other two species based on the presence (QL7) and number of bulbils (QL8), shape of mature dry bulbs (QL6), and general fertility (QL16), as seen in Figures 2F,J,M, 3A. Furthermore, A. × proliferum shallot accessions could be distinguished from A. cepa Aggregatum and A. × cornutum (Figure 3A) based on extreme vegetative growth (Figure 2B), foliage color (QL1) and attitude (QL2), leaf diameter (QL3) and cross-section shape (QL4), and scape morphology (QL9).
Based on PLS analyses of quantitative descriptors of shallot accessions, it was seen that higher variability separates A. × proliferum from the other two species in leaf (QN2) and bulb (QN5) diameter, cluster mass (QN4), and scape length (QN6) and diameter (QN7), confirming gigantism in A. × proliferum (Figure 2B). The number of bulbs in clusters (QN3) and leaf length (QN1) are responsible for most of the variability that differentiated A. × cornutum from the other two species (Figures 2A,K, 3B). Furthermore, shorter stamen length (QN10) and flower pedicel length (QN9) are the distinguishing factors of A. × cornutum (Figure 3B). Inflorescence diameter (QN8) can be used to distinguish A. cepa Aggregatum from A. × cornutum (Figure 3B), particularly in the case of accession IPT208, as seen in Table 2.
Mineral profiles of the shallot accessions, commercial onions, and shallot cultivars are shown in Table 3. Based on morphological descriptors, the accessions were assigned to different species. Data showed that A. × cornutum was characterized by significantly higher N, Ca, Mg, and Cu content than those in A. cepa Aggregatum, but not P and K content (Table 3). The A. × proliferum landrace is characterized by significantly lower Mn content than those in A. × cornutum and A. cepa Aggregatum (Table 3).
TABLE 3. Mineral content of accessions of local shallot landraces and commercial Allium cultivars, expressed on FW.
Local shallot accessions had N concentrations from 8.1 ± 0.3 (in IPT022) to 3.7 ± 0.5 g/kg FW (in IPT208), and differed significantly from the values in shallot cultivar Lang Prince (except IPT208) and commercial onion varieties.
The P concentration in commercial shallots did not differ for the majority of shallot accessions, and ranged from 0.5 ± 0.2 g/kg FW (in Lang Prince and IPT214) to more than 0.7 ± 0.1 g/kg FW (in IPT211, IPT216, IPT217, IPT176, and IPT208). Both commercial onion cultivars had much lower P concentrations than that in the local accessions. P concentrations in commercial onions were 2–3 times lower and differed from that in all shallot accessions (local and commercial), where concentrations ranged from 2.34 ± 0.23 g/kg FW (in Lang Prince) to 3.41 ± 0.06 g/kg FW (in IPT022) and 3.36 ± 0.07 g/kg FW (in IPT216). A. cepa Aggregatum accession IPT208 had higher Ca concentrations (0.94 ± 0.28 g/kg FW) than that of all accessions belonging to A. × proliferum and A. cepa Aggregatum. On average, Mg concentrations in fresh bulbs of shallot accessions were twofold higher than in commercial onion varieties. Generally, higher Mg was found in IPT022, and commercial onions had the lowest Mg concentrations when compared with all shallot accessions.
Shallot accessions and commercial samples did not differ significantly in Zn concentrations. The highest Mn in fresh bulbs was found in ‘Legend’ commercial onions, when compared with all other analyzed samples, except IPT218. The highest concentration of Cu was found in IPT022 (1.83 ± 0.19 g/kg) when compared with all other accessions, except IPT211.
Compared with the cultivars of commercial common onions, local shallot accessions had significantly higher N, P, and K levels, while the content of other minerals was not significantly different (Table 3).
The PLS analysis of nutritional and mineral data is shown in Figure 3C. A. × proliferum IPT023 and most A. × cornutum accessions differed from other groups in P (N2), K (N3), and Mn (N7) content (Figure 3C). Ca (N4) and Zn (N6) represented the largest differences between A. cepa Aggregatum and A. × cornutum. Mg (N5) and Cu (N8) levels also contributed to differentiation between A. cepa Aggregatum and A. × cornutum, albeit to a lesser extent owing to comparable levels of these minerals in several shallot accessions from both groups.
The two most abundant phenolic compounds detected in local shallot accessions were quercetin-4′-glucoside and quercetin-3,4′-diglucoside (Table 4 and Figure 4).
TABLE 4. Phenolic profiles of accessions local shallot landraces, common onion landraces, and commercial Allium cultivars expressed in mg/kg FW.
FIGURE 4. Chromatograms of (A) A. × cornutum IPT211, (B) A. × proliferum IPT023, and (C) A. cepa Aggregatum IPT176 at 280 nm. Peaks: (P1) quercetin-4′-glucoside; (P2) quercetin-3,4′-glucoside; (P3) quercetin; (P4) Chlorogenic acid; (P5) Isoquercetin; (P6) Vanillic acid; (P7) Ferulic acid.
Quercetin-4′-glucoside and quercetin-3,4′-diglucoside concentration in A. × cornutum ranged from 845.0 ± 100.9 mg/kg FW (in IPT211) to 133.5 ± 31.0 mg/kg FW (in IPT021) and from 213.5 ± 39.2 (in IPT022) to 129.4 ± 1.1 mg/kg FW (in IPT215), respectively (Table 4). Quercetin-4′-glucoside and quercetin-3,4′-diglucoside concentration in A. cepa Aggregatum ranged from 193.8 ± 22.3 mg/kg FW (in IPT176) to 26.2 ± 7.2 mg/kg FW (in IPT 208) and from 107.0 ± 15.0 mg/kg FW (in IPT176) to 27.0 ± 5.8 mg/kg FW (in IPT208), respectively (Table 4). Quercetin-4′-glucoside and quercetin-3,4′-diglucoside concentration in A. × proliferum (IPT023) were 213.2 ± 52.7 mg/kg FW and 124.4 ± 30.8 mg/kg FW, respectively, which lie between the quercetin-4′-glucoside levels measured in A. × cornutum and A. cepa Aggregatum (Table 4).
Quercetin was detected in A. × cornutum IPT211 and IPT022 and chlorogenic acid was detected in A. × cornutum IPT211 and IPT214 (Table 4). Ferulic acid was detected in A. × cornutum IPT211 and A. cepa Aggregatum IPT176, IPT216, IPT217, and IPT218 (Table 4).
Vanillic acid (P6) was detected in A. cepa Aggregatum IPT176, IPT216, IPT217, and IPT218 (Table 4). In addition, isoquercetin was detected in A. × cornutum IPT211, which contained the most abundant and diverse phenolic compound profile of all tested accessions and cultivars (Figure 4 and Table 4).
Local common onion landraces (IPT003 and IPT004) and the ‘Redwing’ commercial common onion had levels of quercetin-4′-glucoside and quercetin-3,4′-diglucoside comparable to those in the accessions of A. cepa Aggregatum, except IPT176 (Table 4).
Commercial ‘Legend’ yellow common onions and commercial ‘Lang prince de Bretagne’ long bulb shallots had levels of quercetin-3,4′-diglucoside comparable to those in A. × proliferum IPT023, several A. × cornutum accessions (IPT021, IPT213, and IPT215), and several A. cepa Aggregatum (IPT176 and IPT217) (Table 4).
Average species antioxidant capacities were comparable between A. × cornutum accessions and A. × proliferum, but were significantly lower in A. cepa Aggregatum (Table 5). A. × cornutum IPT211 and IPT022 had the highest FRAP and DPPH quenching levels, while the lowest values were measured in local common onion varieties (IPT003 and IPT004) (Table 5).
TABLE 5. Antioxidant capacity and total phenolic content in local shallot landraces, local common onion landraces, and commercial Allium cultivars.
Total phenolic content results reflect phenolic profiles of local accessions (Table 5). In A. × cornutum, TPC ranged from 1.96 ± 0.01 mg GAE/g FW (in IPT211) to 0.99 ± 0.02 mg GAE/g FW (in IPT021) (Table 5). In A. cepa Aggregatum, TPC levels ranged from 0.93 ± 0.03 mg GAE/g FW (in IPT216) to 0.71 ± 0.02 mg GAE/g FW (in IPT218) (Table 5). In A. × proliferum, IPT023 TPC was 1.28 ± 0.04 mg GAE/g FW, which was comparable to TPC levels in A. × cornutum (Table 5). A. cepa Aggregatum had significantly lower TPC levels than that in A. × cornutum and A. × proliferum (Table 5). The commercial shallot cultivar and commercial yellow onion ‘Legend’ had TPC levels similar to that in A. × cornutum accessions; while the commercial red onion cultivar ‘Redwing’ had TPC levels comparable to A. cepa Aggregatum accessions (Table 5). Local common onion varieties (IPT003 and IPT004) had the lowest TPC values (Table 5).
Among local shallot accessions, A. × cornutum IPT211 had the most abundant and diverse phenolic compound profile (Figure 4 and Table 4). IPT211 also demonstrated high antioxidant capacity, as shown by FRAP and radical scavenging ability, making it the most interesting accession for further studies (Table 5).
Phenolic profile data was processed by PLS analysis to further examine the differences between local shallot accessions (Figure 3D). The property responsible for the most variability was quercetin-4′-glucoside (P1) content, followed by quercetin-3,4′-diglucoside (P2) content (Figure 3D).
Based on quercetin-3,4′-diglucoside (P2) levels, local shallot accessions can be divided into three groups, A. × cornutum, A. × proliferum, and A. cepa Aggregatum (Figure 3D). Furthermore, A. cepa Aggregatum accessions were distinguished from other groups by the presence of vanillic acid (P6), with the exception of IPT208 (Figure 3D and Table 4). A. × cornutum had the most variable phenolic profile, as seen with quercetin-4′-glucoside (P1), quercetin (P3), chlorogenic acid (P4), and isoquercetin (P5) content (Figure 3D). These results indicate that local shallot accessions can be discriminated based on their phenolic profiles.
In this study, based on morphological observations of reproductive and vegetative plant traits, the accessions belonging to A. cepa Aggregatum, (2n = 2x = 16), A. × proliferum Moench Schrad. (2n = 2x = 16), and A. × cornutum Clementi ex Vis. (2n = 3x = 24) were characterized. Among the analyzed accessions characterized using EC/PGR plant morphological descriptors, six belong to A. × cornutum (IPT021, IPT022, IPT211, IPT212, IPT213, IPT214, and IPT215), one to A. × proliferum (IPT023), and three to A. cepa Aggregatum (IPT217, IPT281, and IPT208).
The A. × cornutum group is particularly interesting, since it is grown in a relatively narrow coastal region and on islands in Croatia, and has two main common names. In the southern part of the coast (Dalmatia) it is known as ‘Ljutika,’ while in the northern part (Istria) it is known as ‘Škalonja.’ A genetically similar species named ‘Pran,’ can be found in India (Fredotović et al., 2017). Complexity of the triparental origin of allotriploid A. × cornutum was previously studied by Friesen and Klaas (1998). However, Puizina et al. (1999) found evidence that two of three parents of triploid viviparous A. × cornutum were A. cepa and A. roylei. Combined molecular phylogenic and cytogenetic studies by Fredotović et al. (2014) provided evidence that the third putative parent of A. × cornutum was the wild Asian species A. pskemense B. Fedtsch.
Unlike A. × cornutum, A. × proliferum is only occasionally found in home gardens. It is a spontaneous hybrid between A. cepa and A. fistulosum L., and is commonly known as tree onion or Egyptian onion (Puizina and Papeš, 1996; Maass, 1997; Friesen and Klaas, 1998). It is characterized by underdeveloped underground bulbs and very wide diameter of scape, which bares several levels of sprouting bulbils and ends with prizmatic inflorescence.
The shallots of A. cepa Aggregatum are more important in the continental region of Croatia (Figure 1, IPT216) (personal observation), and their morphological diversity will be the subject of a future study. These “onion-like” shallots are cultivated around the world, including in Europe, and the same species are known by different common names. In addition, the same common name is sometimes used for different species. Therefore, simple and fast tools for evaluation at the phenological level to provide quick classification on-site for breeders or for curators of genetic banks is potentially very useful.
Partial least square analysis of qualitative and quantitative plant morphological descriptors used in this study confirmed the importance of several qualitative traits for accession characterization (Figures 3A,B). The accessions in this study were clearly separated by inflorescence and perianth morphology. To distinguish A. × cornutum from A. × proliferum, and A. cepa Aggregatum, the degree of leaf waxiness, flower number in umbel, stamen morphology, and anther color were the most important morphological descriptors. A. × proliferum was distinguished from the other groups by several qualitative traits, such as foliage color and attitude, leaf diameter and cross-section shape, and scape morphology. In contrast, based on A. × proliferum gigantism, quantitative descriptors, such as leaf and bulb diameter, cluster mass, scape length, and diameter might also be employed for discrimination among shallots (Figures 3A,B). A. cepa Aggregatum is characterized by lack of bulbils in inflorescence, shape of dry bulb, and fertility, when compared with A. × cornutum and A. × proliferum. Although the ECP/GR descriptor list is very comprehensive, in the case of a large number of accessions, the shorter list may be utilized for discriminating accessions.
The main minerals found were N, K, and Ca, while P and Zn were also detected at considerable levels. The mineral content of shallots in our study was similar to values suggested by USDA (2018) for raw shallots, although Ca levels were approximately twofold higher than those reported.
The differences in mineral composition among genotypes and species in our study did not result from differences in cultivation practices or environmental factors, as was suggested for onions and garlic by Ariyama et al. (2006), Petropoulos et al. (2015, 2018), and Vadalà et al. (2016). As the shallot plants were grown in the same field (same soil type and farming practices), the results of our study were because of genotypic differences. The observed differences in mineral content among genotypes may be related to mechanisms controlling nutrient uptake, translocation, or utilization. Our results suggest that commercial common onion cultivars are generally less efficient in nutrient metabolism than local shallot accessions.
The content of phenolic compounds in plant tissues are often negatively affected by high N-nutrition (Treutter, 2010), although experiments with onions showed no significant difference in quercetin-4′-glucoside content between unfertilized onions and onions that received nitrogen fertilizers (Mogren et al., 2007). It is interesting that our accession IPT022 had the highest N concentration in fresh tissue and is among the landraces with higher concentrations of main phenolic compounds. Therefore, it seems that genotype is not related to N-metabolism efficiency or phenolic compound accumulation.
The activities of phenolic acids and flavonoids as antioxidants are directly connected to their ability to reduce oxidizing agents, such as free radicals, via functional hydroxyl groups (Wright et al., 2001). Flavonoids are usually present in plants in glycosylated form, resulting in reduced radical scavenging activity, but increased water solubility (Rice-Evans et al., 1997).
As previously reported, the two major phenolic compounds in onion varieties are quercetin-4′-glucoside and quercetin-3,4′-diglucoside (Yang et al., 2004; Bonaccorsi et al., 2005, 2008; Beretta et al., 2017; Fredotović et al., 2017). Soininen et al. (2014) found that long bulb shallot varieties have higher levels of both compounds than round bulb shallot varieties. In our study the content of phenolic compounds was not directly related to bulb shape, since all variety of shapes were found regardless of species. However, when the values for quercetin-4′-glucoside and quercetin-3,4′-diglucoside were averaged for all accessions belonging to same species, we found significantly higher content in A. × cornutum and A. × proliferum than in A. cepa Aggregatum.
Several minor compounds were detected in some of the investigated accessions, which helped in their differentiation (Figure 3D). Vanillic and ferulic acids were detected in all investigated A. cepa Aggregatum accessions, except in IPT208, which has stamen morphology of A. cepa type indicating close genetic similarity. Ferulic acid was also detected in A. × cornutum IPT211 and A. × proliferum IPT023. Beretta et al. (2017) reported the presence of coumaric and ferulic acids in common onions, and ferulic acid in bunching onions, but not in shallots. Prakash et al. (2007) reported the presence of gallic, ferulic, and protocatechuic acids in four varieties of A. cepa. Vanillic, caffeic, ferulic, and chlorogenic acids were detected in addition to the main flavonoids in fresh cut onions, as demonstrated by Chen et al. (2016). In the analyzed samples, chlorogenic acid was detected only in A. × cornutum IPT211 and IPT214. The ability to identify and characterize local landraces by phenolic profile has been reported previously in different species, cultivars (Riggi et al., 2013; Lo Bianco et al., 2017), and local landraces of A. cepa (Riggi et al., 2013). In our study, the phenolic profile proved to be a powerful tool to discriminate among local shallot accession groups, especially with inclusion of minor phenolic compounds. High levels of the main flavonols, as well as great diversity in minor phenolic compounds suggest A. × cornutum IPT211 accession as a prime candidate for further agronomic, genetic, and biochemical studies.
Total phenolic content was determined by the colorimetric Folin–Ciocalteu method, which measures oxidation of phenolic compounds, and the results should correlate well with the estimated antioxidant capacity (Prior et al., 2005). The estimated antioxidant capacity of biological systems should be evaluated using at least two methods to account for interfering compounds (Schlesier et al., 2002; Ozgen et al., 2006). In our study two methods, DPPH free radical quenching and FRAP, were selected. Each rely on electron transfer to determine antioxidant capacity (Prior et al., 2005). In agreement with our study, shallot cultivars commonly have higher levels of flavonoids, TPC, and antioxidant capacity compared with common onion (Yang et al., 2004; Lu et al., 2011; Beretta et al., 2017). Additionally, our results showed that TPC and antioxidant capacity also differ among shallot species found in Croatia, especially A. × cornutum and A. cepa Aggregatum accessions. It is known that TPC and antioxidant activity in plants change with growing conditions (Heimler et al., 2017), and compound extraction methods (Alothman et al., 2009). A study conducted by Pan et al. (2018) showed that quercetin glucosides in Allium species, especially quercetin-4′-glucoside, have great potential as tumor cell growth inhibitors. Higher TPC values in Allium methanolic extracts correlated with higher in vitro radical scavenging ability and stronger inhibition of tumor cell proliferation (Fredotović et al., 2017). Similarly, in our study, shallot accessions or commercial cultivars with higher TPC exhibited stronger in vitro antioxidative effects.
Local landraces are of paramount importance for local agro-economic systems. By providing detailed morphological and chemical characteristics, these landraces can be appropriately preserved and evaluated in addition to the commercial varieties. In this study, shallot accessions important in coastal Croatia were characterized and compared with commercial Allium varieties. Local accessions in our study were differentiated according to inflorescence and perianth morphology. The most important morphological descriptors that separated A. × cornutum from A. × proliferum and A. cepa Aggregatum were degree of leaf waxiness, flower number in umbel, stamen morphology, and anther color. A. × cornutum and A. × proliferum exhibited higher antioxidative capacity and total phenolic content compared with A. cepa Aggregatum. A. × cornutum is characterized by significantly higher N, Ca, Mg, and Cu content compared with A. cepa Aggregatum, while A. × proliferum is characterized by significantly lower Mn content. Our results suggest that the investigated landraces possess excellent nutritional qualities, which rival, or even exceed, the quality of commercially developed varieties, especially in terms of the diversity of minor phenolic compounds. The A. × cornutum accession IPT211 was found to be of particular interest because of its biochemical wealth and diversity. However, further studies are needed to characterize bioactive constituents in greater depth, which may unravel the benefits and potential new applications of the rediscovered local landraces.
DB and SGB designed the experiments. SGB, JP, BU, GD, and NM executed the experiments and analyzed the results. DB, SGB, JP, BU, GD, and NM discussed the results and conclusion of the study. SGB, JP, BU, and NM wrote the manuscript. DB and SGB edited manuscript drafts. All authors approved the manuscript.
This research has been supported by the project KK.01.1.1.01.0005 Biodiversity and Molecular Plant Breeding, Centre of Excellence for Biodiversity and Molecular Plant Breeding (CoE CroP-BioDiv), Zagreb, Croatia.
The authors declare that the research was conducted in the absence of any commercial or financial relationships that could be construed as a potential conflict of interest.
Alothman, M., Bhat, R., and Karim, A. A. (2009). Antioxidant capacity and phenolic content of selected tropical fruits from Malaysia, extracted with different solvents. Food Chem. 115, 785–788. doi: 10.1016/j.foodchem.2008.12.005
Ansari, N. A. (2007). Onion cultivation and production in Iran. Middle East. Russ. J. Plant Sci. Biotechnol. 1, 26–38.
Ariyama, K., Nishida, T., Noda, T., Kadokura, M., and Yasui, A. (2006). Effects of fertilization, crop year, variety, and provenance factors on mineral concentrations in onions. J. Agric. Food Chem. 54, 3341–3350. doi: 10.1021/JF0525481
Benzie, I. F. F., and Strain, J. J. (1996). The ferric reducing ability of plasma (FRAP) as a measure of “antioxidant power”: the FRAP assay. Anal. Biochem. 239, 70–76. doi: 10.1006/abio.1996.0292
Beretta, H. V., Bannoud, F., Insani, M., Berli, F., Hirschegger, P., Galmarini, C. R., et al. (2017). Relationships among bioactive compounds content and the antiplatelet and antioxidant activities of six allium vegetable species. Food Technol. Biotechnol. 55, 266–275. doi: 10.17113/ftb.55.02.17.4722
Block, E. (2010). Garlic and Other Alliums?: The Lore and The Science, 1st Edn. London: Royal Society of Chemistry.
Bonaccorsi, P., Caristi, C., Gargiulli, C., and Leuzzi, U. (2005). Flavonol glucoside profile of Southern Italian red onion (Allium cepa L.). J. Agric. Food Chem. 53, 2733–2740. doi: 10.1021/jf048152r
Bonaccorsi, P., Caristi, C., Gargiulli, C., and Leuzzi, U. (2008). Flavonol glucosides in Allium species: a comparative study by means of HPLC–DAD–ESI-MS–MS. Food Chem. 107, 1668–1673. doi: 10.1016/j.foodchem.2007.09.053
Brand-Williams, W., Cuvelier, M. E., and Berset, C. (1995). Use of a free radical method to evaluate antioxidant activity. LWT Food Sci. Technol. 28, 25–30. doi: 10.1016/S0023-6438(95)80008-5
Brickell, C. D., Alexander, C., Cubey, J. J., David, J. C., Hoffman, M. H. A., Malécot, V., et al. (2016). International Code of Nomenclature for Cultivated Plants (ICNCP or Cultivated Plant Code), 9 th Edn. Leuven: International Society for Horticultural Science.
Chapman, H. D., and Pratt, P. F. (1961). Methods of Analysis for Soils, Plants and Waters, 1st Edn. Berkley, MI: Press.
Chen, C., Hu, W., Zhang, R., Jiang, A., and Zou, Y. (2016). Levels of phenolic compounds, antioxidant capacity, and microbial counts of fresh-cut onions after treatment with a combination of nisin and citric acid. Hortic. Environ. Biotechnol. 57, 266–273. doi: 10.1007/s13580-016-0032-x
Cumo, C. (2015). Foods That Changed History?: How Foods Shaped Civilization From the Ancient World to THE Present. Santa Barbara, CA: ABC-CLIO.
FAOSTAT (2018). Food and Agriculture Organization Corporate Statistical Database. Available at: http://www.fao.org/faostat/en/#data/QC/visualize [Accessed May 4, 2018].
Ferioli, F., and D’Antuono, L. F. (2016). Evaluation of phenolics and cysteine sulfoxides in local onion and shallot germplasm from Italy and Ukraine. Genet. Resour. Crop Evol. 63, 601–614. doi: 10.1007/s10722-015-0270-2
Fowler, C., and Hodgkin, T. (2004). Plant genetic resources for food and agriculture: assessing global availability. Annu. Rev. Environ. Resour. 29, 143–179. doi: 10.1146/annurev.energy.29.062403.102203
Fredotović, Ž, Šamanić, I., Weiss-Schneeweiss, H., Kamenjarin, J., Jang, T.-S., and Puizina, J. (2014). Triparental origin of triploid onion, Allium? × ?cornutum (Clementi ex Visiani, 1842), as evidenced by molecular, phylogenetic and cytogenetic analyses. BMC Plant Biol. 14:24. doi: 10.1186/1471-2229-14-24
Fredotović, Ž, Šprung, M., Soldo, B., Ljubenkov, I., Budić-Leto, I., Bilušić, T., et al. (2017). Chemical composition and biological activity of allium cepa L. and Allium × cornutum (Clementi ex Visiani 1842) methanolic extracts. Molecules 22:448. doi: 10.3390/molecules22030448
Friesen, N., and Klaas, M. (1998). Origin of some minor vegetatively propagated allium crops studied with RAPD and GISH. Genet. Resour. Crop Evol. 45, 511–523. doi: 10.1023/A:1008647700251
Fritsch, R. M., and Friesen, N. (2002). “Evolution, domestication and taxonomy,” in Allium Crop Science: Recent Advances, eds H. D. Rabinowitch and L. Currah (Wallingford: CABI), 5–30. doi: 10.1079/9780851995106.0005
Garthwaite, P. H. (1994). An interpretation of partial least squares. J. Am. Stat. Assoc. 89, 122–127. doi: 10.2307/2291207
Govindaraj, M., Vetriventhan, M., and Srinivasan, M. (2015). Importance of genetic diversity assessment in crop plants and its recent advances: an overview of its analytical perspectives. Genet. Res. Int. 2015, 1–14. doi: 10.1155/2015/431487
Griffiths, G., Trueman, L., Crowther, T., Thomas, B., and Smith, B. (2002). Onions - a global benefit to health. Phyther. Res. 16, 603–615. doi: 10.1002/ptr.1222
Heimler, D., Romani, A., and Ieri, F. (2017). Plant polyphenol content, soil fertilization and agricultural management: a review. Eur. Food Res. Technol. 243, 1107–1115. doi: 10.1007/s00217-016-2826-6
Helland, I. (2014). “Partial least squares regression,” in Encyclopedia of Statistical Sciences, ed. N. Balakrishnan (Chichester: John Wiley & Sons, Ltd.), doi: 10.1002/9781118445112.stat03287
IPGRI, ECP/Gr, and AVRDC (2001). Descriptors for Allium (Allium spp.) International Plant Genetic Resources Institute,Rome,Italy. Taipei: Asian Vegetable Research and Development Center.
Jump, A. S., Marchant, R., and Peñuelas, J. (2009). Environmental change and the option value of genetic diversity. Trends Plant Sci. 14, 51–58. doi: 10.1016/j.tplants.2008.10.002
Lešić, R., Borošić, J., Buturac, I., Ćustić, M. H., Poljak, M., and Romić, D. (2004). Povrcìarstvo. Zrinski. Available at: http://bib.irb.hr/prikazi-rad?rad=183962 [Accessed August 10, 2017].
Lo Bianco, M., Siracusa, L., Dattilo, S., Venora, G., and Ruberto, G. (2017). Phenolic fingerprint of sicilian modern cultivars and durum wheat landraces: a tool to assess biodiversity. Cereal Chem. J. 94, 1045–1051. doi: 10.1094/CCHEM-06-17-0125-R
Lu, X., Wang, J., Al-Qadiri, H. M., Ross, C. F., Powers, J. R., Tang, J., et al. (2011). Determination of total phenolic content and antioxidant capacity of onion (Allium cepa) and shallot (Allium oschaninii) using infrared spectroscopy. Food Chem. 129, 637–644. doi: 10.1016/j.foodchem.2011.04.105
Maass, H. I. (1997). Genetic diversity in the top onion, Allium × proliferum (Alliaceae), analysed by isozymes. Plant Syst. Evol. 208, 35–44. doi: 10.2307/23643317
Mogren, L. M., Olsson, M. E., and Gertsson, U. E. (2007). Effects of cultivar, lifting time and nitrogen fertiliser level on quercetin content in onion (Allium cepa L.) at lifting. J. Sci. Food Agric. 87, 470–476. doi: 10.1002/jsfa.2735
Ohri, D., Fritsch, R. M., and Hanelt, P. (1998). Evolution of genome size in allium (Alliaceae). Plant Syst. Evol. 210, 57–86. doi: 10.1007/BF00984728
Ozgen, M., Reese, R. N., Tulio, A. Z. Jr., Scheerens, J. C., and Miller, A. R. (2006). Modified 2,2-Azino-bis-3-ethylbenzothiazoline-6-sulfonic acid (ABTS) method to measure antioxidant capacity of selected small fruits and comparison to ferric reducing antioxidant power (FRAP) and 2,2‘-Diphenyl-1-picrylhydrazyl (DPPH) methods. J. Agric. Food Chem. 54, 1151–1157. doi: 10.1021/JF051960D
Pan, Y., Zheng, Y. M., and Ho, W. S. (2018). Effect of quercetin glucosides from allium extracts on HepG2, PC-3 and HT-29 cancer cell lines. Oncol. Lett. 15, 4657–4661. doi: 10.3892/ol.2018.7893
Petropoulos, S., Fernandes,Â, Ntatsi, G., Petrotos, K., Barros, L., and Ferreira, I. (2018). Nutritional value, chemical characterization and bulb morphology of greek garlic landraces. Molecules 23:319. doi: 10.3390/molecules23020319
Petropoulos, S. A., Fernandes,Â, Barros, L., Ferreira, I. C., and Ntatsi, G. (2015). Morphological, nutritional and chemical description of “Vatikiotiko”, an onion local landrace from Greece. Food Chem. 182, 156–163. doi: 10.1016/J.FOODCHEM.2015.03.002
Pike, L. (1986). “Onion breeding,” in Breeding Vegetable Crops, ed. M. Bassett (Hartford, CT: AVI Publishing, Inc), 357–394.
Prakash, D., Singh, B. N., and Upadhyay, G. (2007). Antioxidant and free radical scavenging activities of phenols from onion (Allium cepa). Food Chem. 102, 1389–1393. doi: 10.1016/j.foodchem.2006.06.063
Prior, R. L., Wu, X., and Schaich, K. (2005). Standardized methods for the determination of antioxidant capacity and phenolics in foods and dietary supplements. J. Agric. Food Chem. 53, 4290–4302. doi: 10.1021/jf0502698
Puizina, J. (2013). Shallots in Croatia – genetics, morphology and nomenclature. Acta Bot. Croat. 72, 387–398. doi: 10.2478/botcro-2013-0016
Puizina, J., Javornik, B., Bohanec, B., Schweizer, D., Maluszynska, J., and Papeš, D. (1999). Cytogenetical evidences for hybrid structure and origin of diploid and triploid shallots (Allium cepa var.viviparum, Liliaceae) from Dalmatia (Croatia). Genome 42, 1208–1216. doi: 10.1139/g99-023
Puizina, J., and Papeš, D. (1996). Cytogenetical evidences for hybrid structure and origin of diploid and triploid shallots (Allium cepa var.viviparum, Liliaceae) from Dalmatia (Croatia). Plant Syst. Evol. 199, 203–215. doi: 10.1007/BF00984905
Rabinowitch, H. D., and Kamenetsky, R. (2002). “Shallot (Allium cepa, aggregatum group),” in Allium Crop Science: Recent Advances, eds H. D. Rabinowitch and L. Currah (Wallingford: CABI), 409–430. doi: 10.1079/9780851995106.0409
Rice-Evans, C., Miller, N., and Paganga, G. (1997). Antioxidant properties of phenolic compounds. Trends Plant Sci. 2, 152–159. doi: 10.1016/S1360-1385(97)01018-2
Riggi, E., Avola, G., Siracusa, L., and Ruberto, G. (2013). Flavonol content and biometrical traits as a tool for the characterization of “cipolla di giarratana”: a traditional sicilian onion landrace. Food Chem. 140, 810–816. doi: 10.1016/j.foodchem.2012.10.134
Schlesier, K., Harwat, M., Böhm, V., and Bitsch, R. (2002). Assessment of antioxidant activity by using different in vitro methods. Free Radic. Res. 36, 177–187. doi: 10.1080/10715760290006411
Singleton, V. L., and Rossi, J. A. (1965). Colorimetry of total phenolics with phosphomolybdic-phosphotungstic acid reagents. Am. J. Enol. Vitic. 16, 144–158.
Siracusa, L., Avola, G., Patanč, C., Riggi, E., and Ruberto, G. (2013). Re-evaluation of traditional mediterranean foods. The local landraces of “Cipolla di Giarratana” ( Allium cepa L.) and long-storage tomato (Lycopersicon esculentum L.): quality traits and polyphenol content. J. Sci. Food Agric. 93, 3512–3519. doi: 10.1002/jsfa.6199
Slimestad, R., Fossen, T., and Vågen, I. M. (2007). Onions: a source of unique dietary flavonoids. J. Agric. Food Chem. 55, 10067–10080. doi: 10.1021/jf0712503
Soininen, T. H., Jukarainen, N., Auriola, S. O. K., Julkunen-Tiitto, R., Karjalainen, R., and Vepsäläinen, J. J. (2014). Quantitative metabolite profiling of edible onion species by NMR and HPLC-MS. Food Chem. 165, 499–505. doi: 10.1016/j.foodchem.2014.05.132
Stearn, W. T. (1992). How many species of allium are known? Curtis’s Bot. Mag. 9, 180–182. doi: 10.1111/j.1467-8748.1992.tb00096.x
Takhtajan, A. L. (2009). Flowering Plants. 2nd edn. Dordrecht: Springer, doi: 10.1007/978-1-4020-9609-9
Treutter, D. (2010). Managing phenol contents in crop plants by phytochemical farming and breeding—visions and constraints. Int. J. Mol. Sci. 11, 807–857. doi: 10.3390/ijms11030807
USDA (2018). USDA National Nutrient Database for Standard Reference. Available at: https://ndb.nal.usda.gov/ndb/.
Vadalà, R., Mottese, A., Bua, G., Salvo, A., Mallamace, D., Corsaro, C., et al. (2016). Statistical analysis of mineral concentration for the geographic identification of garlic samples from Sicily (Italy), Tunisia and Spain. Foods 5:20. doi: 10.3390/foods5010020
Wright, J. S., Johnson, E. R., and DiLabio, G. A. (2001). Predicting the activity of phenolic antioxidants: theoretical method, analysis of substituent effects, and application to major families of antioxidants. J. Am. Chem. Soc. 123, 1173–1183. doi: 10.1021/ja002455u
Keywords: landrace, mineral composition, morphology, shallot, phenols, PLS
Citation: Major N, Goreta Ban S, Urlić B, Ban D, Dumičić G and Perković J (2018) Morphological and Biochemical Diversity of Shallot Landraces Preserved Along the Croatian Coast. Front. Plant Sci. 9:1749. doi: 10.3389/fpls.2018.01749
Received: 13 July 2018; Accepted: 09 November 2018;
Published: 03 December 2018.
Edited by:
Spyridon Alexandros Petropoulos, University of Thessaly, GreeceReviewed by:
Maria Gonnella, Consiglio Nazionale delle Ricerche, ItalyCopyright © 2018 Major, Goreta Ban, Urlić, Ban, Dumičić and Perković. This is an open-access article distributed under the terms of the Creative Commons Attribution License (CC BY). The use, distribution or reproduction in other forums is permitted, provided the original author(s) and the copyright owner(s) are credited and that the original publication in this journal is cited, in accordance with accepted academic practice. No use, distribution or reproduction is permitted which does not comply with these terms.
*Correspondence: Smiljana Goreta Ban, c21pbGphQGlwdHBvLmhy
Disclaimer: All claims expressed in this article are solely those of the authors and do not necessarily represent those of their affiliated organizations, or those of the publisher, the editors and the reviewers. Any product that may be evaluated in this article or claim that may be made by its manufacturer is not guaranteed or endorsed by the publisher.
Research integrity at Frontiers
Learn more about the work of our research integrity team to safeguard the quality of each article we publish.