- 1Key Laboratory of Agro-ecological Processes in Subtropical Region, Chinese Academy of Sciences, Changsha, China
- 2Dongting Lake Station for Wetland Ecosystem Research, Institute of Subtropical Agriculture Chinese Academy of Sciences, Changsha, China
- 3Key Laboratory of Aquatic Botany and Watershed Ecology, Chinese Academy of Sciences, Wuhan Botanical Garden, Wuhan, China
Phosphorus (P) enrichment as a result of anthropogenic activities can potentially alter plant C:N:P stoichiometry. However, the influence of different P enrichment frequencies on plant C:N:P stoichiometry in P-limited ecosystems is still unclear. In this study, we conducted a P-addition experiment to elucidate the effect of various P enrichment frequencies on the plant C:N:P stoichiometry of Carex brevicuspis in a freshwater wetland at Dongting Lake, China. We used four P enrichment frequencies (treatment A: no P addition; treatment B: three 0.1 g kg−1 additions at 10-day intervals; treatment C: two 0.15 g kg−1 additions at 15-day intervals; and treatment D: one 0.3 g kg−1 addition during the experimental period) in a factorial design with an experimental duration of 30 days. Biomass accumulation was lowest in the treatment A and highest in the treatment C, and increased with decreasing P addition frequency. The shoot:root ratio did not differ significantly between the four treatments. Both foliar and root C concentrations were not significantly different between the treatments. Foliar N concentration was significantly lower in the treatment D than in the other three treatments, while root N concentration did not differ significantly between the treatments. Both foliar and root P concentrations, and foliar C:N were much higher in the treatment B than in the treatment A. However, root C:N did not differ significantly between treatments. Both foliar and root C:P and N:P of C. brevicuspis were lower in the treatment B than in the treatment A. These results indicated that different frequencies of P addition significantly influenced plant growth. Moreover, P enrichment, rather than frequency, significantly influenced plant C:N:P stoichiometry. Our results improve our understanding of the influence of different P enrichment frequencies on plant C:N:P stoichiometry and nutrient cycling in freshwater wetlands.
Introduction
Phosphorus (P) plays an important role in determining plant growth and community structure in both terrestrial and aquatic ecosystems (Elser et al., 2007; He and Dijkstra, 2015; Mao et al., 2016). Recently, anthropogenic discharges of P have doubled the natural P loading due to the intensity of activities such as aquaculture and agriculture (Mao et al., 2015). These large quantities of P are discharged into wetland ecosystems, leading to a series of ecological and environmental problems, such as eutrophication and biodiversity reduction (Rashid and Romshoo, 2013; Bu et al., 2016).
Relative abundances of carbon (C), nitrogen (N), and P, and C:N:P stoichiometry in plants are powerful indicators of ecological processes, e.g., community organization, nutrient limitation, food webs, and decomposition (Elser et al., 2000; Güsewell et al., 2003; Xia et al., 2014). Therefore, studies on plant ecological stoichiometry enhance our understanding of the growth and nutrient-use strategies of plants, and their responses to various environmental stresses. For example, plant C:N:P stoichiometry is significantly affected by external nutrient availability (Elser et al., 2007; Mao et al., 2016). To date, the influence of increased P loading on plant C:N:P stoichiometry has been studied in various wetland ecosystems (Rejmánková et al., 2008; Mao et al., 2016). Most of these studies confirmed that increasing P loading enhanced plant P concentration and decreased C:P, while results regarding the influence of increasing P loading on plant N concentration, C:N, and N:P were inconsistent across studies (Mao et al., 2016). In P-limited ecosystems, P enrichment would promote plant growth and reduce plant N concentrations and N:P ratios, mainly due to the dilution effect (Feller et al., 2007; Mao et al., 2016). However, in N-limited ecosystems, the response of plant N concentration, C:N ratio and N:P ratio might be determined by the plant’s nutrient use strategies (Yuan and Chen, 2015; Mao et al., 2016). Therefore, more studies are needed to investigate the general influence of P enrichment on plant C:N:P stoichiometry.
Nutrient concentrations can increase at different rates over different spatiotemporal scales, which influences plant growth performance (Xie et al., 2004; Zhang et al., 2018). For instance, biomass allocation patterns and P allocation ratios in Eichhornia crassipes differed significantly with different modes of nutrient increase (Xie et al., 2004). Different nutrient enrichment rates would alter soil nitrogen-phosphorus imbalances, affecting the structure, function, and diversity of ecosystems and organisms (Peñuelas et al., 2013). Sun et al. (2018) also confirmed that different N and P input ratios significantly changed the levels of N, P, and other elements in plants, and that the influence differed among different plant organs. While many studies have investigated the influence of P enrichment on plant C:N:P stoichiometry in different types of wetlands (Newman et al., 2004; Feller et al., 2007; Mao et al., 2016), the effects of different P enrichment frequencies on plant stoichiometry remain unclear.
This study investigated the effects of different P enrichment frequencies on wetland plant stoichiometry at Dongting Lake wetland, China. This lake is the second largest freshwater lake in China and has the largest water exchange capacity with the Yangtze River (Xie and Chen, 2008). Our previous studies confirmed that plants were limited by P in this lake (Li et al., 2017, 2018), but it has received increasing inputs of P mainly due to the use of agricultural fertilizer in the local area. The quantity of P input into the lake is about 4.1 × 104 t annually (He et al., 2009). Here, we report the changes in plant C:N:P stoichiometry and growth performance of Carex brevicuspis after four different P enrichment frequency treatments. Based on the above statement, we hypothesized that (1) P enrichment would promote the growth of C. brevicuspis and the influence differ with different P enrichment treatments; (2) P enrichment would increase plant P concentration and C:N ratio, but reduce plant N concentration, C:P and N:P ratios. Moreover, these influences would differ with different P enrichment frequencies.
Materials and Methods
Study Site
Dongting Lake (28°30′–30°20′ N, 111°40′–113°10′ E) is located on the south bank of the mid-section of the Yangtze River (Xie and Chen, 2008). The lake receives inflow from four rivers (Xiang, Zi, Yuan, and Li) in Hunan Province and four channels (Songzikou, Taipingkou, Ouchikou, and Tiaoxiankou) connect it to the Yangtze River (Figure 1). The wetlands are characterized by large seasonal fluctuations in water level and are usually completely flooded from May to October and susceptible to drought from November to April. The mean annual temperature is 16.8°C, with hot summers (June to August, 27.3°C) and cold winters (December to February, 5.8°C). Annual precipitation is 1382 mm, with more than 60% falling from April to August (Deng et al., 2015).
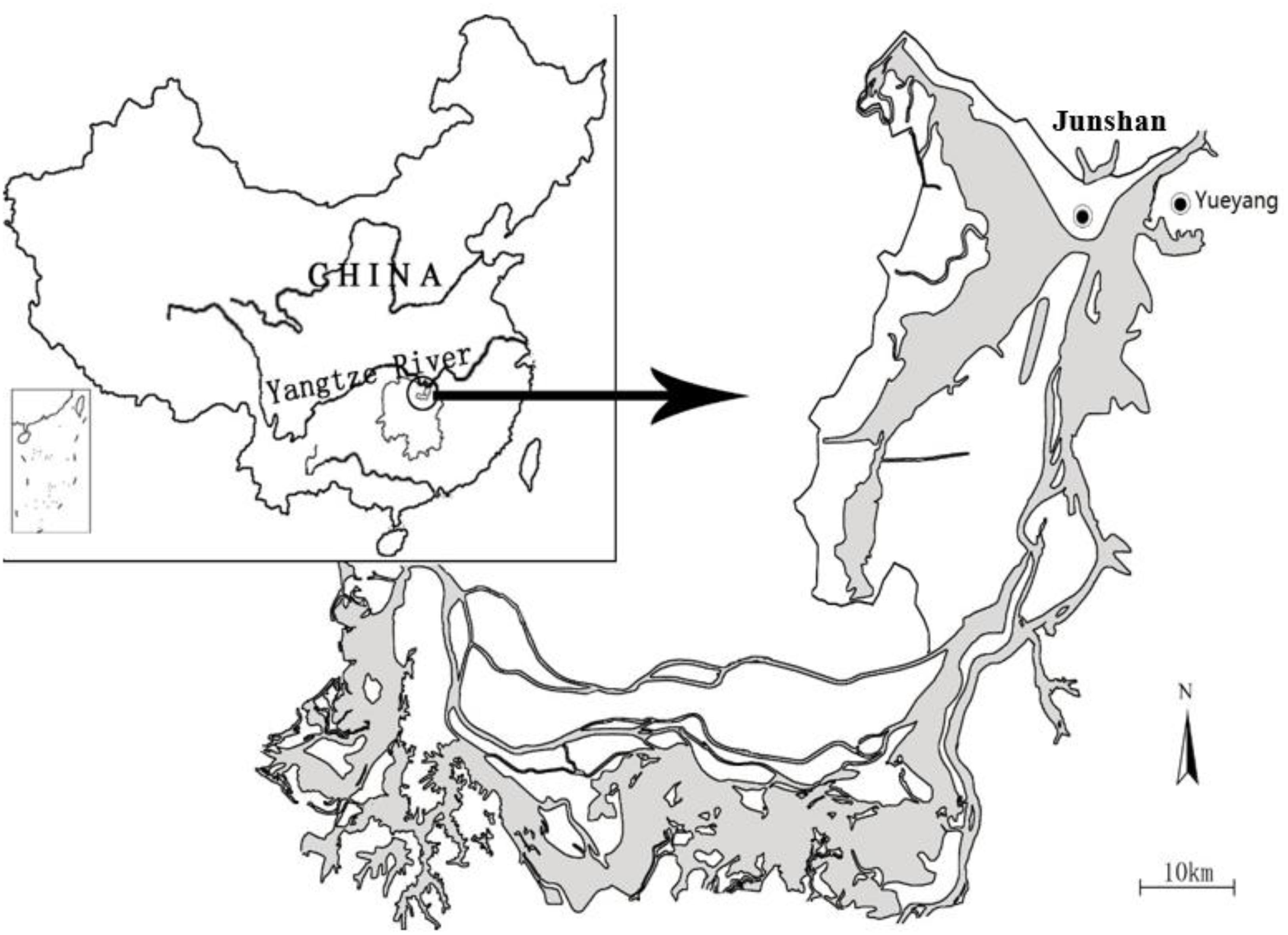
FIGURE 1. Dongting Lake, showing the location of the study site. The shaded areas represent the wetlands.
Study Species
Carex brevicuspis is widely distributed in Taiwan and eastern mainland China (Dai et al., 2010). At Dongting Lake, this species can form mono-dominant communities or co-exist with other species e.g., Miscanthus sacchariflorus and Polygonum hydropiper. C. brevicuspis usually has two growing phases which are related to changes in the water rhythm in Dongting Lake, usually flowering and fruiting in April or May before the flooding, and remaining completely submerged during the flooding season. After flooding, the shoots emerge immediately (November) and grow until January. In January, the above-ground plant parts wither. Subsequently, new ramets emerge and grow rapidly in February and/or March (Deng et al., 2015). In the Dongting Lake, the C. brevicuspis community plays an important role in biodiversity maintenance owing to its multiple ecological functions, for example as a food resource for migratory birds and a spawning ground for migratory fish.
Plants were collected in May 2017 from Junshan county (29°22′ N, 112°59′ E), East Dongting Lake wetland. Small blocks (25 × 25 cm) of C. brevicuspis vegetation were cut and transported to an experimental field at the Dongting Lake Station for Wetland Ecosystem Research, Chinese Academy of Sciences. The blocks were placed in plastic buckets (87 × 65 × 62 cm), which contained 20 cm soil (7.07 mg g−1 organic matter, 0.87 mg g−1 total N, and 0.73 mg g−1 total P; Table 1) to germinate new ramets. Soil was also collected from the area (0–20 cm depth) in which the C. brevicuspis plants were collected. The plants were watered once weekly with tap water (0.511 μg L−1 NH4+−N, 1.760 μg L−1 NO3−N, 0.527 μg L−1 PO43+−P, pH = 7.2).

TABLE 1. Soil characteristics (mean ± SE) after different P addition frequency treatments (treatments A–D represent: no P addition treatment; three – time P addition treatment; two-time P addition treatment; and one-time P addition treatment, respectively).
Experimental Design
Four P enrichment treatments were applied: treatment A – no P addition; treatment B – three 0.1 g kg−1 P additions at 10-day intervals; treatment C – two 0.15 g kg−1 P additions at 15-day intervals; and treatment D – one 0.3 g kg−1 P addition during the experimental period.
On July 11, 2017, 840 seedlings of similar size (3–4 leaves, 20 ± 3 cm in height) were transplanted into 56 pots (30 cm height, 23 cm diameter, and 15 seedlings per pot), which were filled with 7 kg soil (same soil as for seedling cultivation). All pots were placed into 1 of 7 cement ponds (100 × 100 × 100 cm, two pots per treatment per pond) in a random block design. Experimental treatments began on July 18, 2017. Phosphorus was added as NaH2PO4. Firstly, the required mass of NaH2PO4 was dissolved in 150 ml tap water and then sprayed uniformly into the pot. For each P addition treatment, the pots that did not receive NaH2PO4 were leached using 150 ml tap water. Each pot was supplied 500 mL tap water once weekly and exposed to natural sunlight.
Harvest
The plants were harvested 30 days after the first P treatment, before they flowered. Plant roots were carefully dug out by hand and rinsed using tap water to remove sediment. Then, plant parts were separated into leaves and roots (root and rhizome), due to the rhizome is difficult to separated. All parts were oven dried at 80°C for 48 h and weighed. Biomass accumulation was calculated as the collective mass of all tissues. Shoot:root ratio was defined as the ratio of leaf mass to root mass. After plant harvest, we collected soil samples at each pot for soil analysis.
Laboratory Analysis
Dry foliar and root samples were ground for further analysis. Total N and C concentrations were measured using an elemental analyzer (Vario MAX CN, Elementar, Germany), and total P concentration was measured using the molybdenum blue colorimetric method after digesting the samples in a solution of H2SO4 and H2O2 (Zhang et al., 2015).
Soil samples were air-dried and sieved through a 0.15 mm sieve before analysis. Soil organic C content was measured using wet oxidation of organic matter with a solution of KCr2O7 and H2SO4, followed by back-titration using FeSO4. Soil N concentration was measured using the Kjeldahl method and soil P concentration was measured using acid digestion with a solution of H2SO4 and HClO4 (Zhang et al., 2015).
Data Analysis
One-way analysis of variance (ANOVA) was performed in conjunction with Duncan’s test to determine the effect of P addition frequency on biomass accumulation, shoot:root ratio, and plant stoichiometry of C. brevicuspis, as well as soil characteristics (total N, total P, organic carbon content, C:N, C:P, and N : P). Tukey’s post hoc tests were used for multiple comparisons. Data were log10-transformed where necessary to reduce the heterogeneity of variance. Liljefor’s test and Levene’s test were used to test the normality and homogeneity of data, respectively. All statistical analyses were conducted using SPSS ver. 15.0 (SPSS Inc., Chicago, IL, United States).
Results
Biomass Accumulation and Shoot:Root Ratio
The P enrichment frequency had a significant influence on the biomass accumulation of C. brevicuspis (F = 9.562; df = 6; and P < 0.001; Figure 2A), which was lowest in the treatment A and highest in the treatment C. Moreover, biomass accumulation increased with decreasing P enrichment frequency. In contrast, shoot:root ratio did not differ significantly between the four frequency treatments (F = 0.103; df = 6; and P > 0.05; Figure 2B).
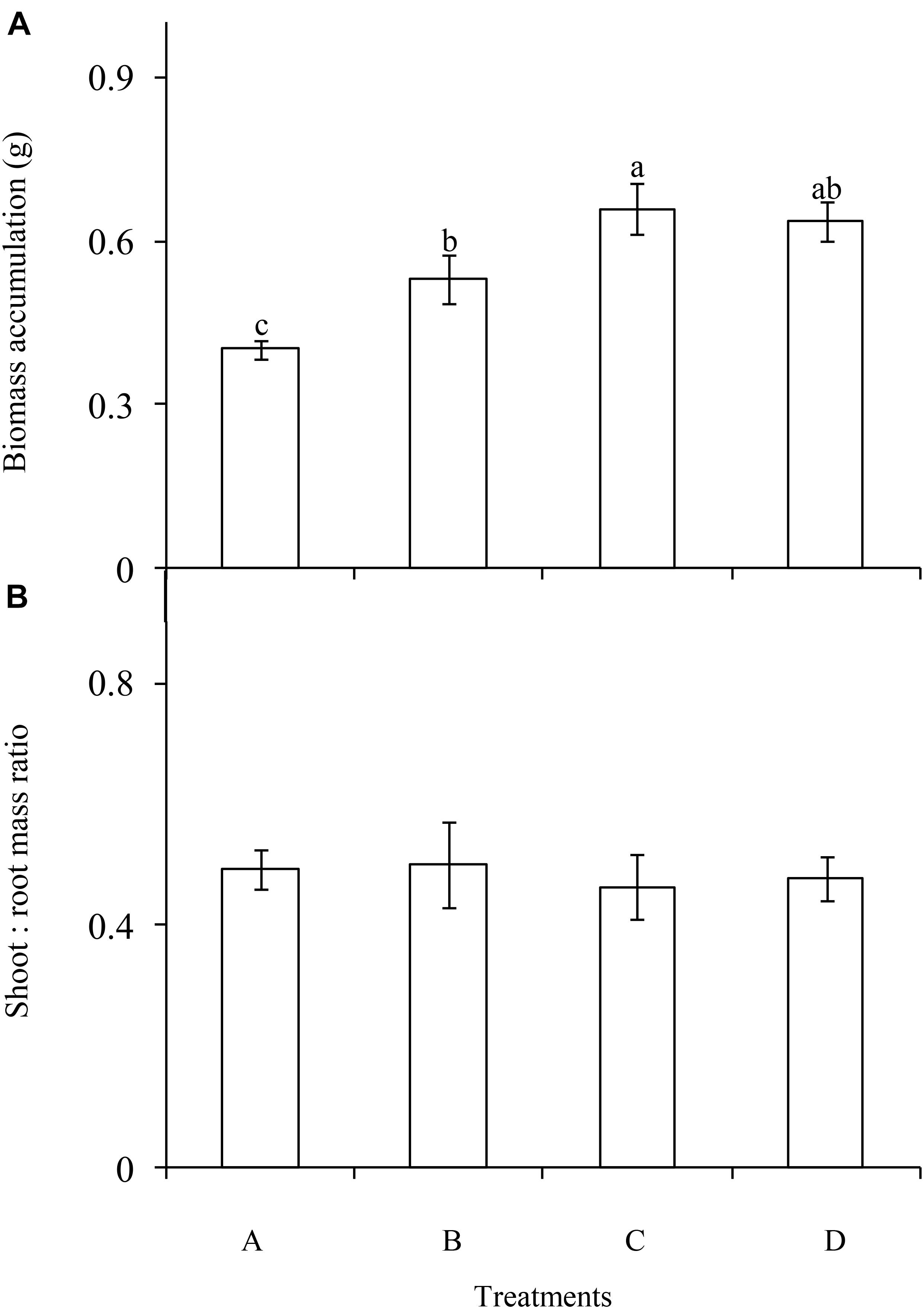
FIGURE 2. Biomass accumulation (A) and allocation (B) of Carex brevicuspis (means ± standard errors, n = 7) under different P addition frequencies (treatments A–D represent: no P addition treatment; three - time P addition treatment; two-time P addition treatment; and one-time P addition treatment, respectively). Different letters indicate significant differences between treatments at the 0.05 significance level.
Foliar Stoichiometry
The frequency of P addition had no significant influence on the foliar total C concentration of C. brevicuspis (F = 2.041; df = 6; and P > 0.05; Figure 3A), although it was lower in the treatment D than in the other treatments. Foliar total N was significantly affected by P addition frequency (F = 3.465; df = 6; and P < 0.05; Figure 3B), which was much lower in the treatment D than in the other three treatments. Foliar total P concentration was much higher in the three P addition treatments than in the control (F = 30.219; df = 6; and P < 0.05; Figure 3C), but did not differ significantly between the three P addition treatments.
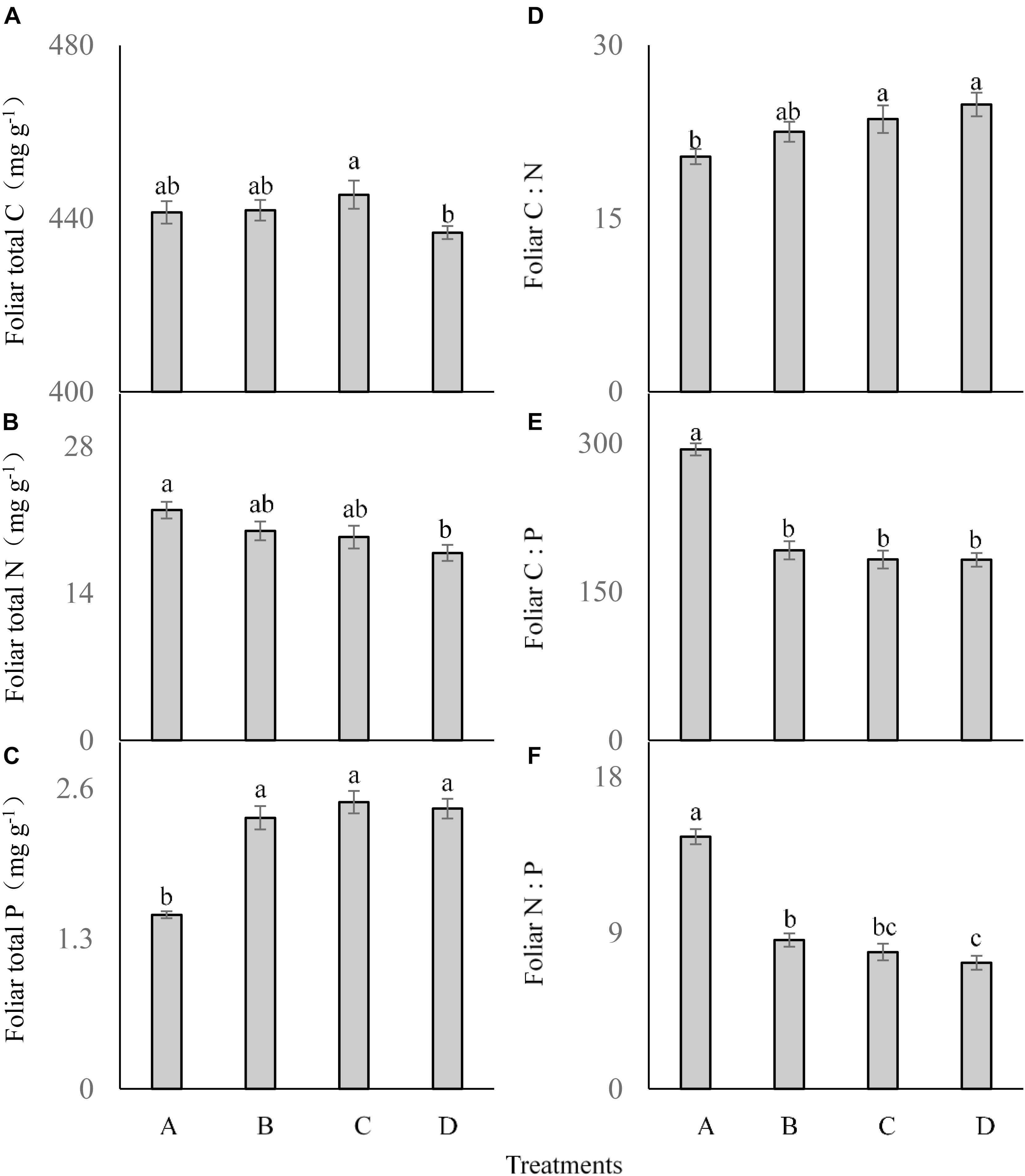
FIGURE 3. Foliar ecological stoichiometry (A–F) of Carex brevicuspis (means ± standard errors, n = 7) under different P addition frequencies (treatments A–D represent: no P addition treatment; three - time P addition treatment; two-time P addition treatment; and one-time P addition treatment, respectively). Different letters indicate significant differences between treatments at the 0.05 significance level.
Foliar C:N showed a similar trend as foliar P concentration, which was much higher in the three addition treatments than in the control (F = 4.009; df = 6; and P < 0.05; Figure 3D), but did not differ significantly among the three P addition treatments. Both foliar C:P (F = 47.787; df = 6; and P < 0.05; Figure 3E) and foliar N:P (F = 61.053; df = 6; and P < 0.05; Figure 3F) showed similar trends, and were much lower in the three P addition treatments than in the control.
Root Stoichiometry
Root total C (F = 2.223; df = 6; and P > 0.05; Figure 4A) and total N (F = 1.299; df = 6; and P > 0.05; Figure 4B) were not significantly different between the treatments. Different P addition treatments significantly influenced the root P content (F = 15.931; df = 6; and P < 0.05; Figure 4C), which was much higher in the three P addition treatments than in the control, and the highest root P content occurred in the treatment C.
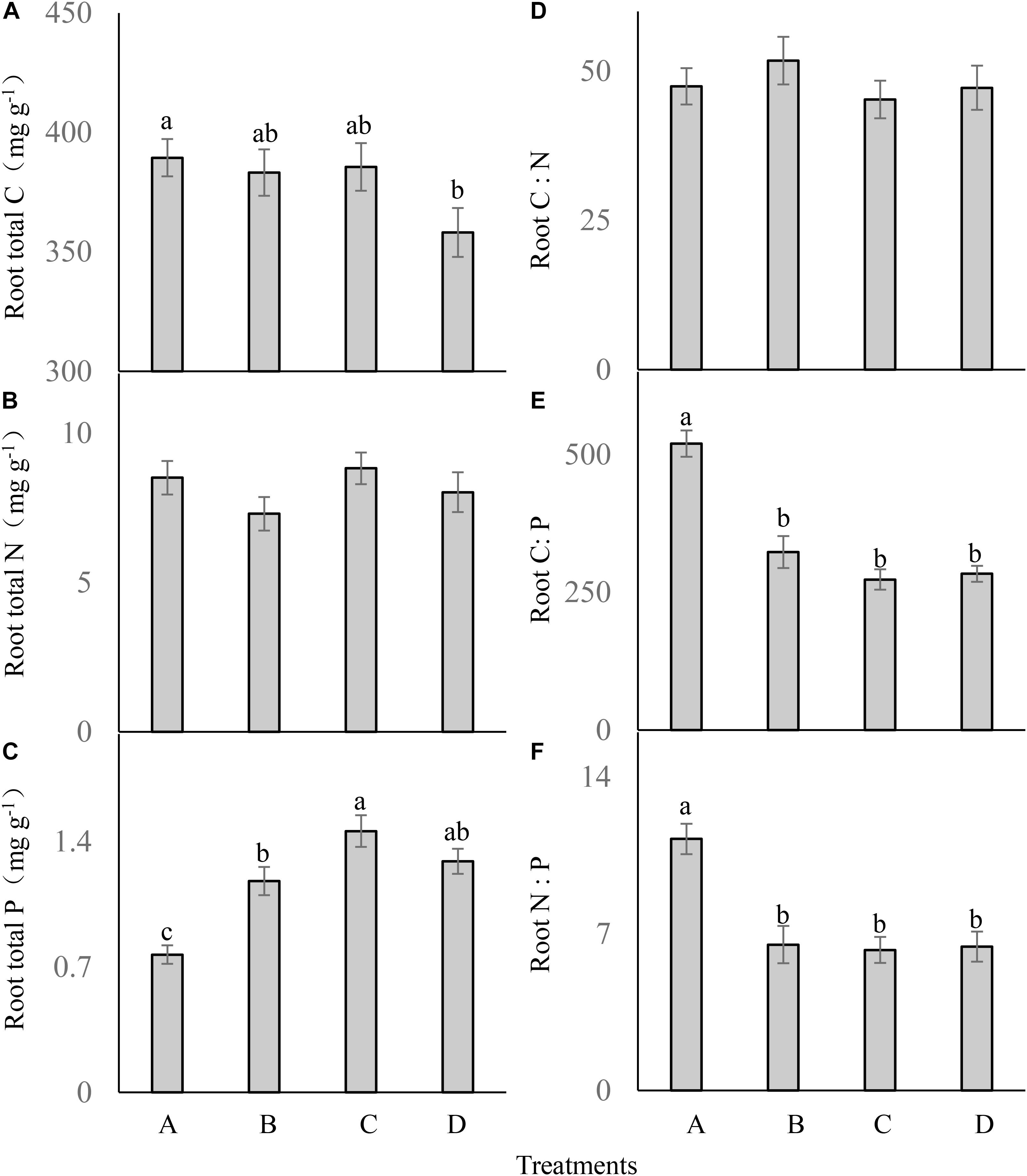
FIGURE 4. Root ecological stoichiometry (A–F) of Carex brevicuspis (means ± standard errors, n = 7) under different P addition frequencies (treatments A–D represent: no P addition treatment; three - time P addition treatment; two-time P addition treatment; and one-time P addition treatment, respectively). Different letters indicate significant differences between treatments at the 0.05 significance level.
Root C:N did not differ significantly between the treatments (F = 0.541; df = 6; and P > 0.05; Figure 4D). Both root C:P (F = 31.954; df = 6; and P < 0.05; Figure 4E) and N:P (F = 12.001; df = 6; and P < 0.05; Figure 4F) were significantly lower in the three P addition treatments than in the control, but were not significantly different between the three P addition treatments.
Discussion
Our study confirmed that biomass accumulation in C. brevicuspis was much higher in the three P addition treatments than in the control, and much higher in the two-time P addition treatment than in the three-time P addition treatment. These results were consistent with hypothesis 1 and suggested that both P addition and addition frequency significantly influenced growth of C. brevicuspis. Moreover, our results confirmed that P enrichment frequency did not significantly influence the biomass allocation of C. brevicuspis, indicating that this was not an effective way for plants to acclimate to different P enrichment frequencies.
The stimulation of plant growth by additional P has been widely reported in other studies (Chiang et al., 2000; McCormick et al., 2001; Mao et al., 2016). For instance, addition of P resulted in a two-fold increase in the biomass of sawgrass and mixed sawgrass-cattail communities in the Everglades Wetland, United States (Chiang et al., 2000). Studies have also confirmed that water lily leaf size was enhanced in response to P enrichment (McCormick et al., 2001; Newman et al., 2004). However, our results contradict those of some other studies (Song et al., 2011; Gao et al., 2016). For instance, Song et al. (2011) found that increased P input had no effect on aboveground biomass of Calamagrostis angustifolia in the Sanjiang Plain Wetland, China, which might have been because plants were adapted to earlier soil P conditions and responded slowly to the addition of P (Macek and Rejmánková, 2007). Another possibility is that P was not a limiting nutrient in the Sanjiang Plain Wetland (Mao et al., 2016). However, our previous study showed that C. brevicuspis was limited by P at Dongting Lake (Li et al., 2017, 2018); this might explain why the addition of P promoted C. brevicuspis growth.
Both foliar and root P concentrations in C. brevicuspis were significantly increased in the three P addition treatments, which might explain the decrease in C:P and N:P in these treatments. These results were partially consistent with our hypothesis 2. Increased plant P concentration following P enrichment has been widely reported (Ostertag, 2010; Song et al., 2011; Mao et al., 2016). However, study of Eleocharis spp. showed that leaf tissue P content decreased with the addition of P, and caused higher C:P and N:P in enriched plots (Daoust and Childers, 2004). These results indicate that plant P concentration responses to P enrichment are species-specific and might be related to plant absorption efficiency and nutrient use strategies, as well as soil microorganism and enzyme activity (Rejmánková et al., 2008; Song et al., 2011; Yuan and Chen, 2015). Moreover, our study confirmed that both foliar and root P concentrations, C:P, and N:P in C. brevicuspis were not significantly different between the three P addition treatments. However, foliar N:P and root P concentration were significantly different, indicating that the influence of P addition frequency on plant stoichiometry was relatively weak. These results contradict our hypothesis 2 and the findings of other studies (Sun et al., 2018). A possible reason is that the amount of P in the three–phase frequency addition treatment was sufficient to support the growth of C. brevicuspis. Another possible reason is that C. brevicuspis has a relatively high stoichiometric homeostasis, which may have maintained stoichiometric stability under different P addition treatments (Han et al., 2014).
Our results suggest that P enrichment decreased foliar N content and increased C:N, which is consistent with the results of other studies (Feller et al., 2007; Yuan and Chen, 2015). The main reason for this finding might be that increased P availability generally stimulates plant growth in P-limited ecosystems, leading to a decline in plant N concentration owing to the dilution effect (Yuan and Chen, 2015; Mao et al., 2016). Another possible reason might be the decrease in soil total N content in the one–time P addition treatment (Table 1). He and Dijkstra (2015) also confirmed that P addition can result in considerable losses of gaseous N from P-poor soils, most likely via direct stimulation of nitrification and denitrification. However, our results differ from those of some other studies (Newman et al., 2004; Mao et al., 2016). In a northern Everglades slough wetland, N concentrations in water lily generally increased in response to increased P loads (Newman et al., 2004), which might have been due to increased soil pore water NH4-N concentrations, mainly from the increased decomposition of resultant nutrient regeneration (Newman et al., 2001). These results suggested that the influence of P enrichment on plant stoichiometry may vary with wetland type and is possibly related to nutrient-limited conditions (Mao et al., 2016).
In conclusion, our results showed that P enrichment promoted plant growth and that this effect increased with decreasing addition frequency. Moreover, we also confirmed that P enrichment, irrespective of the frequency, increased plant C:N and P concentration, but decreased plant N concentration, C:N, and N:P. In recent years, increasing amounts of P have been discharged into Dongting Lake, due to the high intensity of anthropogenic activities. Our results improve our understanding of the influence of P enrichment on wetland nutrient cycling in this lake. However, P input usually occurs with other nutrient elements e.g., N and K. Therefore, the influences of other elements, as well as their interactive effects on plant stoichiometry should be studied to better understand the influence of exogenous nutrient input on nutrient cycling in Dongting Lake.
Author Contributions
FL and CH wrote the manuscript and conducted the technical assays and statistical analysis. YX and WL designed the experiment and edited the manuscript. FL, CH, XC, ZD, and ZH contributed to data collection and interpretation. All authors reviewed the manuscript.
Funding
This study was supported by the National Natural Science Foundation of China (31570431, 41601106), the Open Funding Project of the Key Laboratory of Aquatic Botany and Watershed Ecology, Chinese Academy of Sciences (Y752701s02), the Key Research Program of the Chinese Academy of Sciences (KFZD-SW-318), the Hunan Provincial Natural Science Foundation of China (2017JJ3374), and the Youth Innovation Promotion Association of CAS (2014337).
Conflict of Interest Statement
The authors declare that the research was conducted in the absence of any commercial or financial relationships that could be construed as a potential conflict of interest.
References
Bu, H. M., Liu, W. Z., Song, X. F., and Zhang, Q. F. (2016). Quantitative impacts of population on river water quality in the Jinshui River basin of the South Qinling Mts., China. Environ. Earth Sci. 75:292. doi: 10.1007/s12665-015-5138-4
Chiang, C., Craft, C. B., Rogers, D. W., and Richardson, C. J. (2000). Effects of 4 years of nitrogen and phosphorous additions on Everglades plant communities. Aquat. Bot. 68, 61–78. doi: 10.1016/S0304-3770(00)00098-X
Dai, L. K., Liang, S. Y., Zhang, S. R., Tang, Y. C., Koyama, T., Tucker, G. C., et al. (2010). “Cyperaceae,” in Flora of China, Vol. 23, eds C. Y. Wu, P. H. Raven, and D. Y. Hong (Beijing: Science Press), 164–461.
Daoust, R. J., and Childers, D. L. (2004). Ecological effects of low-level phosphorus additions on two plant communities in a neotropical freshwater wetland ecosystem. Oecologia 141, 672–686. doi: 10.1007/s00442-004-1675-3
Deng, Z. M., Chen, X. S., Xie, Y. H., Xie, Y. J., Hou, Z. Y., and Li, F. (2015). The role of seedling recruitment from juvenile populations of Carex brevicuspis (Cyperaceae) at the Dongting Lake wetlands, China. Sci. Rep. 5:8646. doi: 10.1038/srep08646
Elser, J. J., Bracken, M. E. S., Cleland, E. E., Gruner, D. S., Harpole, W. S., Hillebrand, H., et al. (2007). Global analysis of nitrogen and phosphorus limitation of primary producers in freshwater, marine and terrestrial ecosystems. Ecol. Lett. 10, 1135–1142. doi: 10.1111/j.1461-0248.2007.01113.x
Elser, J. J., Fagan, W. F., Denno, R. F., Dobberfuhl, D. R., Folarin, A., Huberty, A., et al. (2000). Nutritional constraints in terrestrial and freshwater food webs. Nature 408, 578–580. doi: 10.1038/35046058
Feller, I. C., Lovelock, C. E., and McKee, K. L. (2007). Nutrient addition differentially affects ecological processes of Avicennia germinans in nitrogen versus phosphorus limited mangrove ecosystems. Ecosystems 10, 347–359. doi: 10.1007/s10021-007-9025-z
Gao, Y. H., Cooper, D. J., and Ma, X. X. (2016). Phosphorus additions have no impact on plant biomass or soil nitrogen in an alpine meadow on the Qinghai-Tibetan Plateau, China. Appl. Soil Ecol. 106, 18–23. doi: 10.1016/j.apsoil.2016.04.020
Güsewell, S., Koerselman, W., and Verhoeven, J. T. A. (2003). Biomass N:P ratios as indicators of nutrient limitation for plant populations in wetlands. Ecol. Appl. 13, 372–384. doi: 10.1890/1051-0761
Han, X., Sistla, S. A., Zhang, Y. H., Lü, X. T., and Han, X. G. (2014). Hierarchical responses of plant stoichiometry to nitrogen depositionand mowing in a temperate steppe. Plant Soil 382, 175–187. doi: 10.1007/s11104-014-2154-1
He, J. N., Kang, W. X., and Yuan, Z. K. (2009). Analysis of the pollutant source in the Dongting Lake. Chin. Agr. Sci. Bull. 25, 239–244. doi: 10.1371/journal.pone.0170993
He, M. Z., and Dijkstra, F. A. (2015). Phosphorus addition enhances loss of nitrogen in a phosphorous – poor soil. Soil Biol. Biochem. 82, 99–106. doi: 10.1016/j.soilbio.2014.12.015
Li, F., Gao, H., Zhu, L. L., Xie, Y. H., Yang, G. S., Hu, C., et al. (2017). Foliar nitrogen and phosphorus stoichiometry of three wetland plants distributed along an elevation gradient in Dongting Lake, China. Sci. Rep. 7:2820. doi: 10.1038/s41598-017-03126-9
Li, F., Hu, J. Y., Xie, Y. H., Yang, G. S., Hu, C., Chen, X. S., et al. (2018). Foliar carbon, nitrogen and phosphorus stoichiometry of Carex brevicuspis along a small-scale elevation gradient. Ecol. Indic. 92, 322–329. doi: 10.1016/j.ecolind.2017.04.059
Macek, P., and Rejmánková, E. (2007). Response of emergent macrophytes to experimental nutrient and salinity additions. Funct. Ecol. 21, 478–488. doi: 10.1111/j.1365-2435.2007.01266.x
Mao, R., Chen, H. M., Zhang, X. H., Shi, F. X., and Song, C. C. (2016). Effects of P addition on plant C:N:P stoichiometry in a N-limited temperature wetland of Northeast China. Sci. Total Environ. 559, 1–6. doi: 10.1016/j.scitotenv.2016.03.158
Mao, R., Zeng, D. H., Zhang, X. H., and Song, C. C. (2015). Responses of plant nutrient resorption to phosphorus addition in freshwater marsh of Northeast China. Sci. Rep. 5:8097. doi: 10.1038/srep08097
McCormick, P. V., Newman, S., Miao, S. L., Gawlik, D. E., Marley, D., Reddy, K. R., et al. (2001). “Effects of anthropogenic phosphorus inputs on the Everglades,” in The Everglades, Florida Bay, and Coral Reefs of the Florida Keys: An Ecosystem Sourcebook, eds J. W. Porter and K. G. Porter (Boca Raton, FL: CRC Press), 83–126.
Newman, S., Kumpf, H., Laing, J. A., and Kennedy, W. C. (2001). Decomposition responses to phosphorus enrichment in an Everglades (USA) slough. Biogeochemistry 54, 229–250. doi: 10.1023/A:1010659016876
Newman, S., McCormick, P. V., Miao, S. L., Laing, J. A., Kennedy, W. C., and O’Dell, M. B. (2004). The effect of phosphorus enrichment on the nutrient status of a northern Everglades slough. Wetl. Ecol. Manag. 12, 63–79. doi: 10.1023/B:WETL.0000021664.32137.dd
Ostertag, R. (2010). Foliar nitrogen and phosphorus accumulation responses after fertilization: an example from nutrient-limited Hawaiian forests. Plant Soil 334, 85–98. doi: 10.1007/s11104-010-0281-x
Peñuelas, J., Poulter, B., Sardans, J., Ciais, P., van der Velde, M., Bopp, L., et al. (2013). Human-induced nitrogen-phosphorus imbalances alter natural and managed ecosystems across the globe. Nat. Commun. 4:2934. doi: 10.1038/ncomms3934
Rashid, I., and Romshoo, S. A. (2013). Impact of anthropogenic activities on water quality of Lidder River in Kashmir Himalayas. Environ. Monit. Assess. 185, 4705–4719. doi: 10.1007/s10661-012-2898-0
Rejmánková, E., Macek, P., and Epps, K. (2008). Wetland ecosystem changes after three years of phosphorus addition. Wetlands 28, 914–927. doi: 10.1672/07-150.1
Song, C. C., Liu, D. Y., Song, Y. Y., Yang, G. S., Wan, Z. M., Li, Y. C., et al. (2011). Effects of exogenous phosphorus addition on soil respiration in Calamagrostis angustifolia freshwater marshes of Northeast China. Atmos. Environ. 45, 1402–1406. doi: 10.1016/j.atmosenv.2010.12.030
Sun, X., Shen, Y., Schuster, M. J., Searle, E. B., Chen, J. H., Yang, G. W., et al. (2018). Initial responses of grass litter tissue chemistry and N:P stoichiometry to varied N and P input rates and ratios in Inner Mongolia. Agr. Ecosyst. Environ. 252, 114–125. doi: 10.1016/j.agee.2017.10.007
Xia, C. X., Yu, D., Wang, Z., and Xie, D. (2014). Stoichiometry patterns of leaf carbon, nitrogen and phosphorous in aquatic macrophytes in eastern China. Ecol. Eng. 70, 406–413. doi: 10.1016/j.ecoleng.2014.06.018
Xie, Y. H., and Chen, X. S. (2008). Effects of three-gorge project on succession of wetland vegetation in Dongting Lake. Res. Agri. Mod. 29, 684–687.
Xie, Y. H., Wen, M. Z., Yu, D., and Li, Y. K. (2004). Growth and resource allocation of water hyacinth as affected by gradually increasing nutrient. Aquat. Bot. 79, 257–266. doi: 10.1016/j.aquabot.2004.04.002
Yuan, Z. Y., and Chen, H. Y. (2015). Negative effects of fertilization on plant nutrient resorption. Ecology 96, 373–380. doi: 10.1890/14-0140.1
Zhang, W., Zhao, J., Pan, F. J., Chen, H. S., and Wang, K. L. (2015). Changes in nitrogen and phosphorus limitation during secondary succession in a karst region in southwest China. Plant Soil 391, 77–91. doi: 10.1007/s11104-015-2406-8
Keywords: Carex brevicuspis, plant C:N:P stoichiometry, P enrichment frequency, biomass accumulation, biomass allocation
Citation: Li F, Hu C, Xie Y, Liu W, Chen X, Deng Z and Hou Z (2018) Influence of Differ P Enrichment Frequency on Plant Growth and Plant C:N:P in a P-Limited Subtropical Lake Wetland, China. Front. Plant Sci. 9:1608. doi: 10.3389/fpls.2018.01608
Received: 31 March 2018; Accepted: 17 October 2018;
Published: 05 November 2018.
Edited by:
Chunhua Liu, Wuhan University, ChinaReviewed by:
Fuzhong Wu, Sichuan Agricultural University, ChinaXinhou Zhang, Northeast Institute of Geography and Agroecology (CAS), China
Copyright © 2018 Li, Hu, Xie, Liu, Chen, Deng and Hou. This is an open-access article distributed under the terms of the Creative Commons Attribution License (CC BY). The use, distribution or reproduction in other forums is permitted, provided the original author(s) and the copyright owner(s) are credited and that the original publication in this journal is cited, in accordance with accepted academic practice. No use, distribution or reproduction is permitted which does not comply with these terms.
*Correspondence: Feng Li, changshalifeng@163.com Yonghong Xie, yonghongxie@163.com Wenzhi Liu, liuwz@wbgcas.cn
†These authors have contributed equally to this work