- School of Biological Sciences, The University of Queensland, St Lucia, QLD, Australia
Research on insect–plant interactions has highlighted the intricacies of constitutive and induced plant defenses. Of particular interest has been the relationship of natural enemies (especially parasitic hymenoptera) to herbivore induced changes to plants, especially their responses to herbivore induced plant volatiles (HIPVs). In recent decades this has been a fertile area for research, with elegant experiments showing that HIPVs are important in attracting natural enemies to plants. We critically appraise the application of work on HIPVs in plant–insect–natural enemy interactions. The promise of applications to improve pest management has not been forthcoming. We attribute this to a failure to include the multifaceted aspects of natural enemy–prey interactions – attraction, location, subjugation and experience. Attraction in an olfactometer by naïve parasitoids has not been translated to methodologically sound field-based estimates of higher parasitism rates. We highlight what needs to be done to better understand the information that HIPVs convey, how this is utilized by parasitoids and how a greater understanding of these interactions might lead to the development of new strategies so that this knowledge can be effectively deployed for improved pest management.
Introduction
A wide range of pathogens and sucking, chewing and boring herbivores assail all parts of plants in nature. Recent research has revealed the intricacies and sophistication of a Pandora’s box of chemical interactions that mediate plant responses to this attack (see Kaplan, 2012; Schuman and Baldwin, 2016). Plants have a range of constitutive (e.g., Zalucki et al., 2001; Steppuhn et al., 2004) and induced chemical defenses (Kessler and Baldwin, 2002) that can act either directly or indirectly on herbivores. Induced defenses represent a sophisticated, layered set of responses that are modulated by a complex phytohormone system (Wu and Baldwin, 2010; Schuman and Baldwin, 2016; Howe et al., 2018). For example, necrotrophic microbes, some phloem-feeding insects and chewing herbivores induce the jasmonic acid (JA) pathway (Howe and Jander, 2008), whereas some phloem-feeding insects and biotrophic pathogens induce the salicylic acid (SA) pathway (Spoel et al., 2007). Cross-talk between these pathways, enables plants to regulate their defense responses according to the type of attacker (Koornneef and Pieterse, 2008; Thaler et al., 2012).
Following plant damage, induced direct defenses lead to localized and systemic elevation of toxic secondary compounds above constitutive levels, which affect the preference, performance and feeding behavior of herbivores (Wittstock and Gershenzon, 2002; Perkins et al., 2013; Zalucki et al., 2017). The increased release of volatiles from a plant after attack by a herbivore is considered to be an induced indirect defenze, communicating the location of herbivores on infested plants to parasitoids and predators of the attackers (Vet and Dicke, 1992; Dicke et al., 2003a,b; Turlings and Erb, 2018). Numerous studies have shown the importance of multi–trophic relationships in plant-insect-natural enemy “attack-defenze” systems. These may be as a result of aboveground (van Dam et al., 2003) or belowground herbivory (van Dam and Oomen, 2008; Qiu et al., 2009) and they can interact in complex ways (Soler et al., 2005, 2007; van Dam and Heil, 2011).
In natural environments it is very likely that plants suffer sequential and/ or simultaneous attack by numerous herbivores and pathogens. Plant pathogens can suppress the host plant defenses that they induce by leveraging SA-JA crosstalk using chemical toxins and the virulent effector proteins they secret (Xin and He, 2013; Kaloshian and Walling, 2016). In this context, the distinction between plant pathogens and insect symbionts can become blurred. For example, co-infection of tobacco plants with a begomovirus and its betasatellite represses JA-regulated defenses, allowing whitefly populations to increase (Zhang et al., 2012). In this system the compatibility of the tobacco–begomovirus interaction means that SA levels are unaffected, and the suppression of the JA-regulated defenses is independent of SA (Zhang et al., 2012). Similarly, herbivore manipulation of plant defenses has been associated with the chemical compounds and specific proteins in the saliva of chewing and phloem-feeding insect herbivores (Will et al., 2007; Elzinga et al., 2014; Villarroel et al., 2016), making the precise outcome of interactions difficult to predict. Thus, in any given system, simultaneous interactions, each of which can have positive or negative effects of varying degrees of magnitude at the individual and population level, likely manifest as an indeterminate orgy of multipartite interactions. This, coupled with an incomplete understanding of specific relationships, makes reliable predictions of outcomes problematic. This at least partly accounts for the near absence of successful field applications of technologies based on the deployment of herbivore induced plant volatiles (HIPVs) or the chemical manipulation of plants to suppress herbivore pest populations (Turlings and Erb, 2018).
Exploiting Host Plant Odors for Pest Suppression
This is not to suggest that host plant odors have not been successfully manipulated for improved pest management. They provide the mechanism underpinning push- pull strategies (Cook et al., 2007), which rely on plant odors to repel pest insects from crops and attract them to non-crop plants at the margins of fields. The most prominent example of the successful deployment of this strategy involves the companion cropping of maize with the forage grass Melinis minutiflora or the forage legume Desmodium sp. and the planting of other grasses, e.g., Pennisetum purpureum or Sorghum vulgare sudanaense at the field margins (Pickett et al., 2014; Pickett and Khan, 2016). Volatiles produced by M. minutiflora or Desmodium sp. repel ovipositing stem borers from the maize crop (Khan et al., 2000), thereby providing the “push,” while other volatiles produced by the specific forage grasses planted on the margins attract the ovipositing females, thereby producing the “pull” away from the crop (Khan et al., 2000). This strategy, and well researched variants, has been adopted by approximately 120,000 small holders in sub-Saharan East Africa (Pickett and Khan, 2016). Despite this undoubted success, further claims that the attractive properties of volatiles emitted by M. minutiflora themselves result in meaningful greater parasitism of stem borers on maize (Khan et al., 1997; Midega et al., 2009) need to be treated with caution. These compounds clearly invoke strong behavioral responses in parasitoids in the laboratory (Khan et al., 1997; Tamiru et al., 2012), but their impact on pest mortality in the field requires further investigation. Reported parasitism rates are typically derived from small or unreported sample sizes and, even when statistically significant changes in parasitism rates are reported, increases are small and not contextualized with respect to other mortality factors (see Van Driesche et al., 1991). Similarly, although stemborer oviposition on maize has been demonstrated to increase attraction of egg and larval parasitoids in the laboratory (Tamiru et al., 2011), evidence demonstrating increased egg mortality in the field as a result is lacking. Further, the interpretation of increased larval parasitoid attraction as early recruitment of natural enemies in anticipation of egg hatching (Tamiru et al., 2011) is teleological. Indeed, Cotesia sesamiae (Cameron) (Hymenoptera: Braconidae) attacks late larvae of Chilo partellus (Swinhoe) (Lepidoptera: Crambidae) (Chinwada et al., 2003) and naïve females of this species are attracted to, but do not discriminate between, maize plants infested by C. partellus and the non-host Busseola fusca (Fuller) (Lepidoptera: Noctuidae) (Ngi-Song et al., 1995, 1996).
Parasitoid Responses to Herbivore Induced Plant Volatiles
Although naïve parasitoids can be attracted to the volatiles produced by plants that are attacked by their host herbivores, our work shows that they can equally be attracted to volatiles emitted by those plants when they are attacked by non-host herbivores, or even when they are induced by a chemical elicitor, such as exogenous JA (Figure 1A). These responses are typically modulated by parasitoid experience, such that experienced parasitoids orientate preferentially toward plants upon which they have previously successfully located and parasitized a host (Figure 1A). Thus, although parasitoids might exhibit an innate preference for the volatiles produced by certain plants (Allison and Hare, 2009), such preferences can be overridden by experience (Figure 1A). Parasitoid orientation to a plant emitting volatiles that might be associated with the presence of host larvae is merely the first step in host location. Upon alighting on the plant surface, parasitoids typically become arrested (Figures 1B, 2), before increasing activity following the perception of post-alighting cues (which might include volatiles released as a result of herbivore feeding damage, non-volatile plant metabolites or herbivore frass), intensifying their search around sites of herbivore feeding damage before locating, sampling and then parasitizing their hosts (Wang and Keller, 2002; Figures 1C, 2). The vast majority of studies that seek to manipulate the responses of plants to herbivory in order increase biological control of pests rely on the application of a plant defense response elicitor (e.g., JA, SA or their derivatives) with the express purpose of attracting parasitoids to treated plants. The importance of subsequent parasitoid behaviors (see Figure 2 for description of post-alighting fraging behavior) is then not considered, ignoring factors that are critical to successful host location and subsequent attack. For example, although the diamondback moth parasitoid, Diadegma semiclausum (Hellén) (Hymenoptera: Ichneumonidae), orientates toward and alights on intact, host-free cabbage plants treated with exogenous applications of JA, its post-alighting behavior is inhibited. Our work shows that the residency time of individuals alighting on JA-treated plants is the same as that of individuals landing and foraging on plants infested with host larvae (Figure 1B). Furthermore, experienced individuals locate hosts much more quickly than naïve parasitoids, demonstrating the importance of post-alighting cues in successful host location (Figure 1C). The lack of consideration of the subtlety and sophistication of the post-alighting chemical ecology of host–parasitoid interactions likely contributes to the dearth of examples where these strategies have been successfully deployed in the field. Further research to better understand these behaviors in parasitoids that are targeted for field manipulation by exploiting host–plant interactions for improved pest management are encouraged.
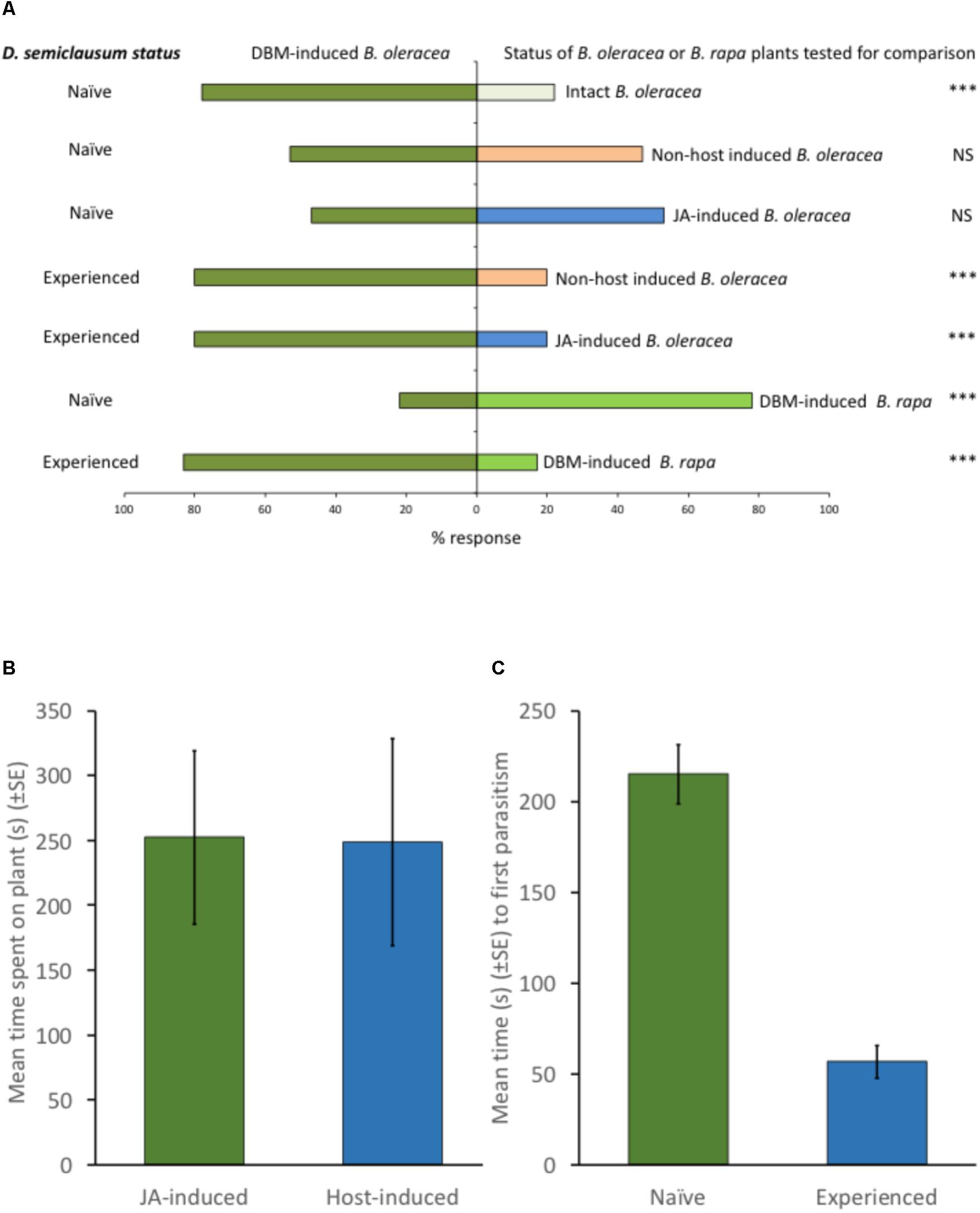
FIGURE 1. (A) Effect of previous experience on the responses of Diadegma semiclausum Hellén (Hymenoptera: Ichneumonidae) to cabbage (Brassica oleracea capitata cv sugarloaf) and Chinese cabbage (Brassica rapa pekinensis cv Wombok) host plants (6-leaf stage) in olfactometer bioassays. Methods: The olfactometer consisted of a glass Y-tube (0.8 cm internal diameter, 7 cm stem) with two 9.5 cm arms at a 60° angle leading to sealed glass chambers, each containing a single plant. Clean air (filtered through activated charcoal filters before entry into the apparatus) was drawn through the system with a vacuum pump at a rate of 1 L min-1. Test insects were introduced to the end of the Y-tube stem 6 cm away from the Y-split. A choice was considered made once a test insect breached the 2.5 cm mark up an arm of the Y-tube. Each test insect was given up to 10 min to respond, and each insect was used only once. For each pairwise combination of plant treatments, fresh female parasitoids were used until 30 individuals had responded. The Y-tube was rotated between each replicate, and between every five consecutive replicates, odor sources were replaced, and all glassware was washed in 95% ethanol, rinsed with distilled water and then dried at 75°C for 1 h. All tests were performed in a draught-free room at 24 ± 1°C under an artificial light. Statistical tests: Preferences of parasitoids in the various pairwise tests were analyzed using a X2-test. NSP > 0.05 (X2(df = 1) < 3.841); ∗∗∗P < 0.001 (X2(df =1)> 10.828). Results: Naïve parasitoids [= parasitoids (1–2 days post-eclosion)] that had not foraged for or oviposited into a host [Plutella xylostella L. (Lepidoptera: Plutellidae) ( = diamondback moth, DBM)] larva on a cabbage plant were more attracted to host-damaged plants (feeding by 10 early 3rd instar DBM larvae for 24 h immediately prior to test, larvae removed prior to olfactometer bioassay) than to (intact plants (P < 0.001), but did not discriminate between DBM-damaged plants and non-host [Crocidolomia pavonana (F) (Lepidoptera: Crambidae) ( = large cabbage moth, LCM)] damaged plants (feeding by 10 2nd instar LCM larvae for 24 h immediately prior to test, larvae removed prior to olfactometer bioassay) (P > 0.05) or DBM-damaged plants and plants treated with jasmonic acid (JA) (aqueous solution of 0.1 μmolL-1 JA applied to plants 24 h prior to test; see Lu et al., 2004 for methods). Experienced parasitoids (= parasitoids that had oviposited into a host DBM larva on a cabbage plant) were more attracted to DBM-damaged plants than LCM-damaged plants (P < 0.001) or plants treated with JA (P < 0.001). Although naïve parasitoids were more attracted to DBM-damaged Chinese cabbage plants than DBM-damaged cabbage plants (P < 0.001), parasitoids previously experienced on DBM on cabbage plants were more attracted to DBM-damaged cabbage plants than DBM-damaged Chinese cabbage plants in subsequent olfactometer assays (P < 0.001). (B) Effect of DBM larvae (damage and presence) and jasmonic acid (JA) treatment on D. semiclausum post-alighting residency time on cabbage plants. Methods: Experiments were conducted in a wind tunnel with a Perspex flight chamber (160 cm × 65 cm × 65 cm). A fan circulated clean air (passed over an activated charcoal filter) through the chamber at 0.7 ms-1. Laminar airflow was obtained by passing air through a honeycomb of soda straws and a fine stainless-steel screen (1.25 mm aperture) before it entered the chamber. A single potted cabbage test plant (6-leaf stage) was placed in the centre of the chamber 45 cm from the screen and a single female D. semiclausum released 50 cm downwind. The responses of parasitoids to JA-treated plants (aqueous solution of 0.1 μmolL-1 JA applied to plants 24 h prior to test; see Lu et al., 2004 for methods) and to DBM-infested (10 early 3rd instar larvae for 24 h) plants were compared by alternating JA-treated and DBM-infested plants in the chamber and recording the D. semiclausum response; each parasitoid was given up to 5 min to respond, and each insect was used only once. When a parasitoid alighted on the test plant it was watched carefully and the time it spent on the plant (= residency time) recorded. The responses of single parasitoids to 9 plants of each treatment were recorded. Statistical tests: Data were tested for normality (D’Agostino and Pearson test) and then subject to an unpaired t-test (Prism 7, Graphpad Software, Inc., 2017). Results: Parasitoids spent as long on JA-treated plants (n = 9) as they did on DBM-infested (10 early 3rd instar larvae) plants (n = 9) [t = 0.036 (df = 16), P = 0.972]. (C) Effect of host experience on post-alighting parasitoid foraging efficiency. Methods: Single D. semiclausum cocoons were transferred to clean glass tubes (0.8 cm × 4 cm). Upon eclosion female parasitoids were fed (10% honey solution) and some parasitoids were then experienced by allowing a single wasp to forage on cabbage plants (6-leaf stage) infested with third instar DBM larvae in a mesh cage (45 cm × 45 cm × 45 cm). Once a parasitoid attacked a host larva it was removed and held in a labeled glass tube. Cabbage plants (6-leaf stage; n = 20) were infested with 20 late instar larvae and transferred singly to mesh cages for 24 h. A single naïve or a single experienced D. semclausum was then introduced to each cage, carefully observed and the time taken to attack the first host larva was recorded. Statistical tests: Data were tested for normality (D’Agostino and Pearson test) and then subject to an unpaired t-test (Prism 7, Graphpad Software, Inc., 2017). Results: Naïve parasitoids (n = 10) took significantly longer to locate and parasitize a larva after alighting on a DBM-infested cabbage plant than previously experienced parasitoids (n = 10) [t = 7.834 (df = 18), P < 0.0001].)
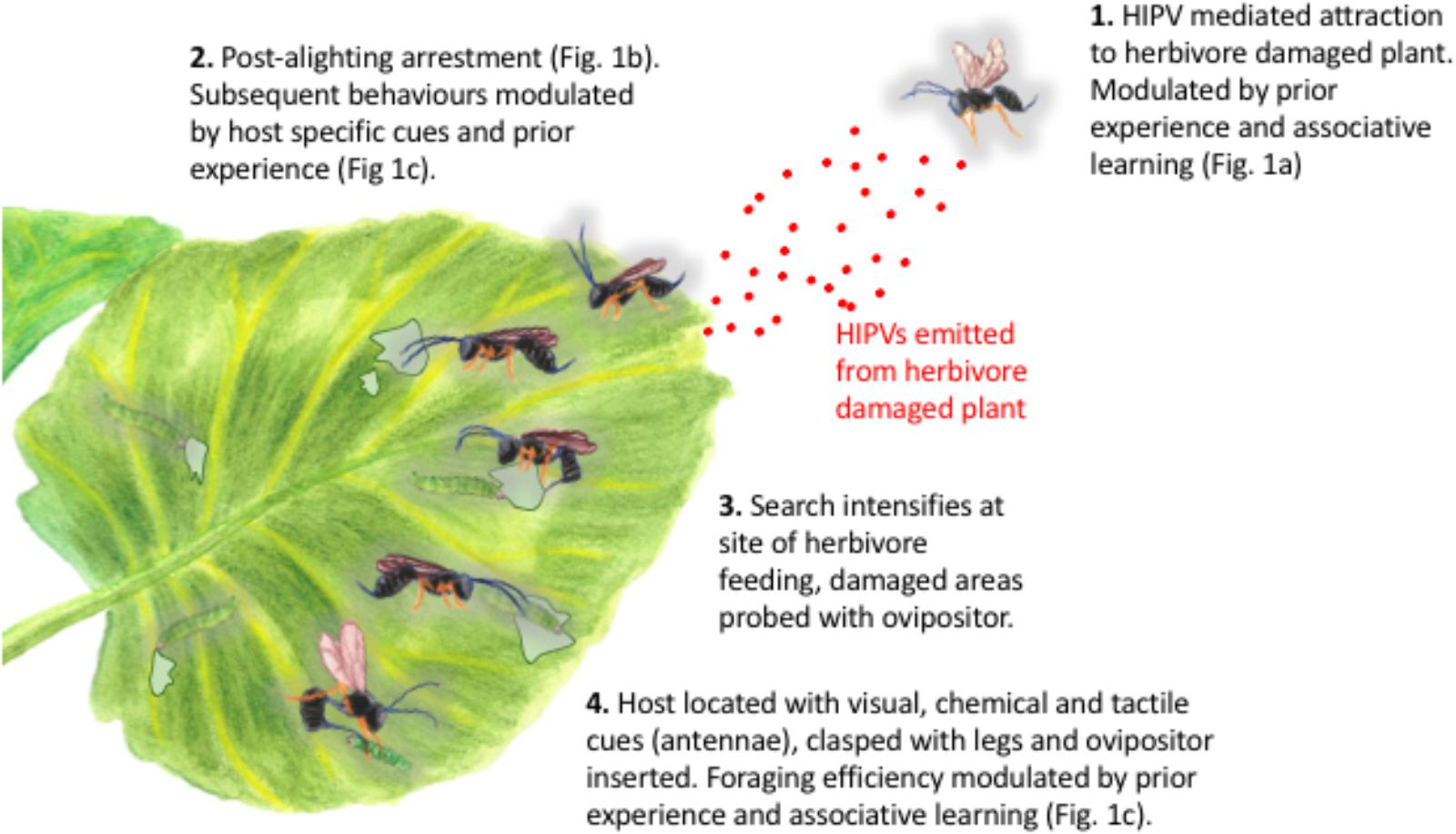
FIGURE 2. Attraction, orientation and post-alighting behavior of parasitoid wasps in response to host-herbivore infested plants. Post-alighting behavioral sequence based on Diadegma semiclausum Hellén (Hymenoptera: Ichneumonidae) as described by Wang and Keller (2002).
Other examples of “field” experiments that demonstrate changes in parasitoid attack rates are small-scale and typically lack adequate controls. Invariably the design consists of a single field into which “test” plants are placed to assess parasitism, usually with both treatments juxtaposed (Lou et al., 2005, 2006; de Lange et al., 2018). Apart from the issues of pseudoreplication associated with such an approach, the key problem is that any change in parasitism rate reflects either a change in parasitoid searching efficiency or a change in number of parasitoids foraging due to a combination of short (within field) or longer-range attraction. If the mechanism responsible for increased parasitism is an increase in parasitoid foraging efficiency then the lower parasitism of non-treatment plants within the experimental field reflects the confounding influence of the more attractive plants, which results in decreased attacks on non-treatment plants within the field. What is required is the deployment of independent control blocks, without treatment, that allow background parasitism rates to be assessed and then compared with parasitism rates in independent treated blocks. Without this approach, the apparent changes in parasitism in treated plants cannot be quantified in appropriate context as such changes clearly do not necessarily reflect increased parasitism at the population scale, which must be the goal for improved pest management. The use of multiple fields (blocks) with treatments in a randomized design is a better approach but even when this is done, experimental units are small, e.g., 7 × 7 plants (Poelman et al., 2009) or 4–10 plants (Simpson et al., 2011) and not independent. Although such experiments can provide important and useful preliminary information, their relevance to actual farm fields is limited, except perhaps in situations where fields are very small, and they require larger scale follow-up studies.
Utilizing Herbivore Induced Plant Volatiles for Improved Biological Control and Pest Management
Herbivore induced plant volatiles can be used by parasitoids and predators to locate plants upon which their arthropod hosts or prey are feeding (e.g., Reddy, 2002; Sobhy et al., 2014). However, the role of these compounds in effecting arthropod herbivore population suppression at the field scale is still to be demonstrated. Synthetic volatiles have been deployed as lures within crops (Rodriguez-Saona et al., 2011), and although some success has been demonstrated with respect to the attraction of arthropod predators and parasitoids (James, 2003; James and Grasswitz, 2005), other studies show that HIPVs can have repellent effects on natural enemies (Braasch et al., 2012). The scale over which parasitoids and predators can be manipulated by synthetic HIPVs is typically small (<10 m) (Lee, 2010; Braasch and Kaplan, 2012), meaning that the major effect of their deployment is likely to be the localized redistribution of natural enemies within crops. This has been shown for some parasitic hymenoptera where the attraction to lures affects local abundances by depleting parasitoid numbers elsewhere in the crop (Braasch and Kaplan, 2012). The redistribution and retention of natural enemies in areas of the crop from which their prey might be absent (Figure 1B), and where their foraging behavior is further disrupted by the absence of appropriate post-alighting cues (Figure 2), is likely to disrupt rather than facilitate the conservation biological control strategies into which HIPVs are integrated. Given these effects perhaps the ways in which HIPVs can be best deployed for improved pest management should be re-evaluated; rather than attractants to support conservation biological control, they might be best utilized as arrestants to complement augmentative or even inundative releases of natural enemies (Kaplan, 2012).
The introduction of synthetic HIPVs into crops can induce natural defense responses in nearby plants (Kaplan, 2012) and the application of chemical elicitors (Sobhy et al., 2014) or the genetic manipulation of plants (Beale et al., 2006) to make then more attractive to natural enemies has been advocated. Various elicitors have been shown to change the volatile profiles of maize plants infested by Spodoptera littoralis (Boisduval) (Lepidoptera: Noctuidae), such that they become more attractive to parasitic hymenoptera (Rostas and Turlings, 2008; Sobhy et al., 2014) in laboratory assays. When the strategy was field tested, despite the elicitors changing plant volatile profiles in ways consistent with previous work in the laboratory, reliable increases in parasitism of Spodoptera frugiperda (J.E. Smith) could not be demonstrated (von Mérey et al., 2012). Similarly, genetic manipulation of Arabidopsis thaliana and wheat plants so that they constitutively produced aphid alarm pheromone was successful at repelling aphids and attracting aphid parasitoids in the laboratory (Beale et al., 2006; Bruce et al., 2015). However, when tested in the field wheat plants became infested with aphids and these showed no increased levels of parasitism (Bruce et al., 2015). In addition to changing the responses of parasitoids to induced plants, HIPVs also affect herbivore–plant interactions. In the Brassica oleracea–Plutella xylostella interaction, herbivore and JA-induced plants are more attractive to ovipositing P. xylostella (Lu et al., 2004), D. semiclausum (Figure 1) and predatory lacewing larvae (Furlong, unpublished data). The increased attraction is temporary, and plants revert to their original level of attractiveness 3–4 days after the application of JA or a feeding event (Ang et al., 2016). Selective application of JA to B. oleracea plants in the field can manipulate the spatial patterns of P. xylostella oviposition (Ang, 2018), raising the possibility of utilizing JA to temporarily induce parts of the crop as a “temporal trap-crop,” where P. xylostella eggs can be concentrated for destruction.
Conclusion
Understanding of the molecular basis of plant responses to herbivore attack has increased markedly in recent years, but the technologies that have been developed have not led to more effective use of natural enemies for pest management. This is at least in part due to an under appreciation of how natural enemies utilize and respond to the cues they perceive from herbivore damaged plants under field conditions. Simply deploying HIPVS to attract natural enemies has not worked, however, developing a better understanding of the ecology of these interactions will inform how HIPVs and other components of plant–herbivore–natural enemy interactions might be more effectively used in as yet untested ways.
Author Contributions
MF and MZ conceived and designed the overall study. GA, RS, and MF designed the experiments. GA and RS conducted the experiments. MF and MZ wrote the paper. GA did the artwork for Figure 2.
Conflict of Interest Statement
The authors declare that the research was conducted in the absence of any commercial or financial relationships that could be construed as a potential conflict of interest.
Acknowledgments
We thank Daniel Vassão for the invitation to submit this manuscript and the Australian Centre for International Agricultural Research (ACIAR) (HORT/2010/090) for the research funding which enabled this work.
References
Allison, J. D., and Hare, J. D. (2009). Learned and naïve natural enemy responses and the interpretation of volatile organic compounds as cues or signals. New Phytol. 184, 768–782. doi: 10.1111/j.1469-8137.2009.03046.x
Ang, G. C. K. (2018). Insect-Plant Interactions: Oviposition and Larval-Feeding Behaviour of Diamondback Moth, School of Biological Sciences. Ph.D. Thesis, The University of Queensland, Brisbane.
Ang, G. C. K., Zalucki, M. P., and Furlong, M. J. (2016). Temporal changes in olfactory and oviposition responses of the diamondback moth to herbivore-induced host plants. Entomol. Exp. Appl. 160, 28–39. doi: 10.1111/eea.12458
Beale, M. H., Birkett, M. A., Bruce, T. J. A., Chamberlain, K., Field, L. M., Huttly, A. K., et al. (2006). Aphid alarm pheromone produced by transgenic plants affects aphid and parasitoid behavior. Proc. Natl. Acad. Sci. U.S.A. 103, 10509–10513. doi: 10.1073/pnas.0603998103
Braasch, J., and Kaplan, I. (2012). Over what distance are plant volatiles bioactive? Estimating the spatial dimensions of attraction in an arthropod assemblage. Entomol. Exp. Appl. 145, 115–123. doi: 10.1111/j.1570-7458.2012.01317.x
Braasch, J., Wimp, G. M., and Kaplan, I. (2012). Testing for phytochemical synergism: arthropod community responses to induced plant volatile blends across crops. J. Chem. Ecol. 38, 1264–1275. doi: 10.1007/s10886-012-0202-y
Bruce, T. J. A., Aradottir, G. I., Smart, L. E., Martin, J. L., Caulfield, J. C., Doherty, A., et al. (2015). The first crop plant genetically engineered to release an insect pheromone for defence. Sci. Rep. 5:11183. doi: 10.1038/srep11183
Chinwada, P., Overholt, W. A., Omwega, C. O., and Mueke, J. M. (2003). Geographic differences in host acceptance and suitability of two Cotesia sesamiae populations in Zimbabwe. Biol. Control 28, 354–359. doi: 10.1016/S1049-9644(03)00094-X
Cook, S. M., Khan, Z. R., and Pickett, J. A. (2007). The use of push-pull strategies in integrated pest management. Annu. Rev. Entomol. 52, 375–400. doi: 10.1146/annurev.ento.52.110405.091407
de Lange, E. S., Farnier, K., Degen, T., Gaudillat, B., Aguilar-Romero, R., Bahena-Juárez, F., et al. (2018). Parasitic wasps can reduce mortality of teosinte plants infested with fall armyworm: support for a defensive function of herbivore-induced plant volatiles. Front. Ecol. Evol. 6:55. doi: 10.3389/fevo.2018.00055
Dicke, M., de Boer, J. G., Hofte, M., and Rocha-Granados, M. C. (2003a). Mixed blends of herbivore-induced plant volatiles and foraging success of carnivorous arthropods. Oikos 101, 38–48. doi: 10.1007/s10886-011-9906-7
Dicke, M., van Poecke, R. M. P., and de Boer, J. G. (2003b). Inducible indirect defence of plants: from mechanisms to ecological functions. Basic Appl. Ecol. 4, 27–42. doi: 10.1078/1439-1791-00131
Elzinga, D. A., De Vos, M., and Jander, G. (2014). Suppression of plant defenses by a Myzus persicae (green peach aphid) salivary effector protein. Mol. Plant Microbe Interact. 27, 747–756. doi: 10.1094/MPMI-01-14-0018-R
Howe, G. A., and Jander, G. (2008). Plant immunity to insect herbivores. Annu. Rev. Plant Biol. 59, 41–66. doi: 10.1146/annurev.arplant.59.032607.092825
Howe, G. A., Major, I. T., and Koo, A. J. (2018). Modularity in jasmonate signallingfor multistress resilience. Annu. Rev. Plant Biol. 69, 387–415. doi: 10.1146/annurev-arplant-042817-040047
James, D. G. (2003). Synthetic herbivore-induced plant volatiles as field attractants for beneficial insects. Environ. Entomol. 32, 977–982. doi: 10.1603/0046-225X-32.5.977
James, D. G., and Grasswitz, T. R. (2005). Synthetic herbivore-induced plant volatiles increase field captures of parasitic wasps. BioControl 50, 871–880. doi: 10.1007/s10526-005-3313-3
Kaloshian, I., and Walling, L. L. (2016). Hemipteran and dipteran pests: effectors and plant host immune regulators. J. Integr. Plant Biol. 58, 350–361. doi: 10.1111/jipb.12438
Kaplan, I. (2012). Attracting carnivorous arthropods with plant volatiles: the future of biocontrol or playing with fire? Biol. Control 60, 77–89. doi: 10.1016/j.biocontrol.2011.10.017
Kessler, A., and Baldwin, I. T. (2002). Plant responses to insect herbivory: the emerging molecular analysis. Annu. Rev. Plant Biol. 53, 299–328. doi: 10.1146/annurev.arplant.53.100301.135207
Khan, Z. R., Ampong-Nyarko, K., Chiliswa, P., Hassanali, A., Kimani, S., Lwande, W., et al. (1997). Intercropping increases parasitism of pests. Nature 388, 631–632. doi: 10.1038/41681
Khan, Z. R., Pickett, J. A., van den Berg, J., Wadhams, L. J., and Woodcock, C. M. (2000). Exploiting chemical ecology and species diversity: stem borer and striga control for maize and sorghum in Africa. Pest Manag. Sci. 56, 957–962. doi: 10.1002/1526-4998(200011)56:11<957::AID-PS236>3.0.CO;2-T
Koornneef, A., and Pieterse, C. M. (2008). Cross talk in defense signaling. Plant Physiol. 146, 839–844. doi: 10.1104/pp.107.112029
Lee, J. C. (2010). Effect of methyl salicylate-based lures on beneficial and pest arthropods in strawberry. Environ. Entomol. 39, 653–660. doi: 10.1603/EN09279
Lou, Y. G., Du, M. H., Turlings, T. C. J., Cheng, J. A., and Shan, W. F. (2005). Exogenous application of jasmonic acid induces volatile emissions in rice and enhances parasitism of Nilaparvata lugens eggs by the parasitoid Anagrus nilaparvatae. J. Chem. Ecol. 31, 1985–2002. doi: 10.1007/s10886-005-6072-9
Lou, Y. G., Hua, X. Y., Turlings, T. C. J., Cheng, J. A., Chen, X. X., and Ye, G. Y. (2006). Differences in induced volatile emissions among rice varieties result in differential attraction and parasitism of Nilaparvata lugens eggs by the parasitoid Anagrus nilaparvatae in the field. J. Chem. Ecol. 32, 2375–2387. doi: 10.1007/s10886-006-9151-7
Lu, Y. B., Liu, S. S., Liu, Y. Q., Furlong, M. J., and Zalucki, M. P. (2004). Contrary effects of jasmonate treatment of two closely related plant species on attraction of and oviposition by a specialist herbivore. Ecol. Lett. 7, 337–345. doi: 10.1111/j.1461-0248.2004.00582.x
Midega, C. A. O., Khan, Z. R., Van den Berg, J., Ogol, C. P. K. O., Bruce, T. J., and Pickett, J. A. (2009). Non-target effects of the ‘push–pull’ habitat management strategy: parasitoid activity and soil fauna abundance. Crop Prot. 28, 1045–1051. doi: 10.1016/j.cropro.2009.08.005
Ngi-Song, A. J., Overholt, W., and Ayertey, J. N. (1995). Suitability of african gramineous stemborers for development of Cotesia flavipes and C. sesamiae (Hymenoptera, Braconidae). Environ. Entomol. 24, 978–984. doi: 10.1093/ee/24.4.978
Ngi-Song, A. J., Overholt, W. A., Njagi, P. G. N., Dicke, M., Ayertey, J. N., and Lwande, W. (1996). Volatile infochemicals used in host and host habitat location by Cotesia flavipes Cameron and Cotesia sesamiae (Cameron) (Hymenoptera: Braconidae), larval parasitoids of stemborers on graminae. J. Chem. Ecol. 22, 307–323. doi: 10.1007/BF02055101
Perkins, L. E., Cribb, B. W., Brewer, P. B., Hanan, J., Grant, M., de Torres, M., et al. (2013). Generalist insects behave in a jasmonate dependent manner on their host plants, leaving induced areas quickly and staying longer on distant parts. Proc. R. Soc. B Boil. Sci. 280:20122646. doi: 10.1098/rspb.2012.2646
Pickett, J. A., and Khan, Z. R. (2016). Plant volatile-mediated signalling and its application in agriculture: successes and challenges. New Phytol. 212, 856–870. doi: 10.1111/nph.14274
Pickett, J. A., Woodcock, C. M., Midega, C. A. O., and Khan, Z. R. (2014). Push-pull farming systems. Curr. Opin. Biotechnol. 26, 125–132. doi: 10.1016/j.copbio.2013.12.006
Poelman, E. H., Oduor, A. M. O., Broekgaarden, C., Hordijk, C. A., Jansen, J. J., Van Loon, J. J. A., et al. (2009). Field parasitism rates of caterpillars on Brassica oleracea plants are reliably predicted by differential attraction of Cotesia parasitoids. Funct. Ecol. 23, 951–962. doi: 10.1111/j.1365-2435.2009.01570.x
Qiu, B. L., Harvey, J. A., Raaijmakers, C. E., Vet, L. E. M., and van Dam, N. M. (2009). Nonlinear effects of plant root and shoot jasmonic acid application on the performance of Pieris brassicae and its parasitoid Cotesia glomerata. Funct. Ecol. 23, 496–505. doi: 10.1111/j.1365-2435.2008.01516.x
Reddy, G. V. P. (2002). Plant volatiles mediate orientation and plant preference by the predator Chrysoperla carnea Stephens (Neuroptera: Chrysopidae). Biol. Control 25, 49–55. doi: 10.1016/S1049-9644(02)00038-5
Rodriguez-Saona, C., Kaplan, I., Braasch, J., Chinnasamy, D., and Williams, L. (2011). Field responses of predaceous arthropods to methyl salicylate: a meta-analysis and case study in cranberries. Biol. Control 59, 294–303. doi: 10.1016/j.biocontrol.2011.06.017
Rostas, M., and Turlings, T. C. J. (2008). Induction of systemic acquired resistance in Zea mays also enhances the plant’s attractiveness to parasitoids. Biol. Control 46, 178–186. doi: 10.1016/j.biocontrol.2008.04.012
Schuman, M. C., and Baldwin, I. T. (2016). The layers of plant responses to insect herbivores. Annu. Rev. Entomol. 61, 373–394. doi: 10.1146/annurev-ento-010715-023851
Simpson, M., Gurr, G. M., Simmons, A. T., Wratten, S. D., James, D. G., Leeson, G., et al. (2011). Attract and reward: combining chemical ecology and habitat manipulation to enhance biological control in field crops. J. Appl. Ecol. 48, 580–590. doi: 10.1111/j.1365-2664.2010.01946.x
Sobhy, I. S., Erb, M., Lou, Y., and Turlings, T. C. J. (2014). The prospect of applying chemical elicitors and plant strengtheners to enhance the biological control of crop pests. Philos. Trans. R. Soc. Lond. B Biol. Sci. 369:20120283. doi: 10.1098/rstb.2012.0283
Soler, R., Bezemer, T. M., Cortesero, A. M., van der Putten, W. H., Vet, L. E. M., and Harvey, J. A. (2007). Impact of foliar herbivory on the development of a root-feeding insect and its parasitoid. Oecologia 152, 257–264. doi: 10.1007/s00442-006-0649-z
Soler, R., Bezemer, T. M., van der Putten, W. H., Vet, L. E. M., and Harvey, J. A. (2005). Root herbivore effects on above-ground herbivore, parasitoid and hyperparasitoid performance via changes in plant quality. J. Anim. Ecol. 74, 1121–1130. doi: 10.1111/j.1365-2656.2005.01006.x
Spoel, S. H., Johnson, J. S., and Dong, X. (2007). Regulation of tradeoffs between plant defenses against pathogens with different lifestyles. Proc. Natl. Acad. Sci. U.S.A. 104, 18842–18847. doi: 10.1073/pnas.0708139104
Steppuhn, A., Gase, K., Krock, B., Halitschke, R., and Baldwin, I. T. (2004). Nicotine’s defensive function in nature. PLoS Biol. 2:e217. doi: 10.1371/journal.pbio.0020217
Tamiru, A., Bruce, T., Woodcock, C., Caulfield, J., Midega, C., Ogol, C. P. K. O., et al. (2011). Maize landraces recruit egg and larval parasitoids in response to egg deposition by a herbivore. Ecol. Lett. 14, 1075–1083. doi: 10.1111/j.1461-0248.2011.01674.x
Tamiru, A., Bruce, T. J. A., Midega, C. A. O., Woodcock, C. M., Birkett, M. A., Pickett, J. A., et al. (2012). Oviposition induced volatile emissions from African smallholder farmers’ maize varieties. J. Chem. Ecol. 38, 231–234. doi: 10.1007/s10886-012-0082-1
Thaler, J. S., Humphrey, P. T., and Whiteman, N. K. (2012). Evolution of jasmonate and salicylate signal crosstalk. Trends Plant Sci. 17, 260–270. doi: 10.1016/j.tplants.2012.02.010
Turlings, T. C. J., and Erb, M. (2018). Tritrophic interactions mediated by herbivore-induced plant volatiles: mechanisms, ecological relevance, and application. Annu. Rev. Entomol. 63, 433–452. doi: 10.1146/annurev-ento-020117-043507
van Dam, N. M., Harvey, J. A., Wackers, F. L., Bezemer, T. M., vander Putten, W. H., and Vet, L. E. M. (2003). Interactions between above ground and belowground induced responses against phytophages. Basic Appl. Ecol. 4, 63–77. doi: 10.1078/1439-1791-00133
van Dam, N. M., and Heil, M. (2011). Multitrophic interactions below and above ground: en route to the next level. J. Ecol. 99, 77–88. doi: 10.1111/j.1365-2745.2010.01761.x
van Dam, N. M., and Oomen, W. A. T. (2008). Root and shoot jasmonic acid applications differentially affect leaf chemistry and herbivore growth. Plant Signal. Behav. 3, 91–98. doi: 10.4161/psb.3.2.5220
Van Driesche, R. G., Bellows, T. S., Elkinton, J. S., Gould, J. R., and Ferro, D. N. (1991). The meaning of percentage parasitism revisited - solutions to the problem of accurately estimating total losses from parasitism. Environ. Entomol. 20, 1–7. doi: 10.1093/ee/20.1.1
Vet, L. E. M., and Dicke, M. (1992). Ecology of infochemical use by natural enemies in a tritrophic context. Annu. Rev. Entomol. 37, 141–172. doi: 10.1146/annurev.en.37.010192.001041
Villarroel, C. A., Jonckheere, W., Alba, J. M., Glas, J. J., Dermauw, W., Haring, M. A., et al. (2016). Salivary proteins of spider mites suppress defenses in Nicotiana benthamiana and promote mite reproduction. Plant J. 86, 119–131. doi: 10.1111/tpj.13152
von Mérey, G. E., Veyrat, N., de Lange, E., Degen, T., Mahuku, G., Lopez Valdez, R., et al. (2012). Minor effects of two elicitors of insect and pathogen resistance on volatile emissions and parasitism of Spodoptera frugiperda in Mexican maize fields. Biol. Control 60, 7–15. doi: 10.1016/j.biocontrol.2011.09.010
Wang, X. G., and Keller, M. A. (2002). A comparison of the host-searching efficiency of two larval parasitoids of Plutella xylostella. Ecol. Entomol. 27, 105–114. doi: 10.1046/j.1365-2311.2002.0374a.x
Will, T., Tjallingii, W. F., Thönnessen, A., and van Bel, A. J. (2007). Molecular sabotage of plant defense by aphid saliva. Proc. Natl. Acad. Sci. U.S.A. 104, 10536–10541. doi: 10.1073/pnas.0703535104
Wittstock, U., and Gershenzon, J. (2002). Constitutive plant toxins and their role in defense against herbivores and pathogens. Curr. Opin. Plant Biol. 5, 300–307. doi: 10.1016/S1369-5266(02)00264-9
Wu, J. Q., and Baldwin, I. T. (2010). New insights into plant responses to the attack from insect herbivores. Annu. Rev. Genet. 44, 1–24. doi: 10.1146/annurev-genet-102209-163500
Xin, X. F., and He, S. Y. (2013). Pseudomonas syringae pv. tomato DC3000: a model pathogen for probing disease susceptibility and hormone signaling in plants. Annu. Rev. Phytopathol. 51, 473–498. doi: 10.1146/annurev-phyto-082712-102321
Zalucki, M. P., Brower, L. P., and Alonso, A. (2001). Detrimental effects of latex and cardiac glycosides on survival and growth of first-instar monarch butterfly larvae Danaus plexippus feeding on the sandhill milkweed Asclepias humistrata. Ecol. Entomol. 26, 212–224. doi: 10.1046/j.1365-2311.2001.00313.x
Zalucki, M. P., Zalucki, J. M., Perkins, L. E., Schramm, K., Vassão, D. G., Gershenzon, J., et al. (2017). A generalist feeder copes with a specialized plant defence: the effects of induction and feeding on intact Arabidopsis by Helicoverpa armigera (Lepidoptera: Noctuidae). J. Chem. Ecol. 43, 608–616. doi: 10.1007/s10886-017-0855-7
Keywords: herbivore induced plant volatiles, jasmonic acid, salicylic acid, indirect defense, parasitoid, chemical ecology, integrated pest management
Citation: Furlong MJ, Ang GCK, Silva R and Zalucki MP (2018) Bringing Ecology Back: How Can the Chemistry of Indirect Plant Defenses Against Herbivory Be Manipulated to Improve Pest Management? Front. Plant Sci. 9:1436. doi: 10.3389/fpls.2018.01436
Received: 26 April 2018; Accepted: 10 September 2018;
Published: 27 September 2018.
Edited by:
Mariana Alves Stanton, Universidade de São Paulo, BrazilReviewed by:
Maria Fernanda Gomes Villalba, Universidade Federal de Lavras, BrazilAndrea McCormick, Massey University, New Zealand
Copyright © 2018 Furlong, Ang, Silva and Zalucki. This is an open-access article distributed under the terms of the Creative Commons Attribution License (CC BY). The use, distribution or reproduction in other forums is permitted, provided the original author(s) and the copyright owner(s) are credited and that the original publication in this journal is cited, in accordance with accepted academic practice. No use, distribution or reproduction is permitted which does not comply with these terms.
*Correspondence: Michael J. Furlong, m.furlong@uq.edu.au Myron P. Zalucki, m.zalucki@uq.edu.au