- 1Department of Horticultural Science, Kyungpook National University, Daegu, South Korea
- 2School of Agriculture and Aquaculture, Tra Vinh University, Trà Vinh, Vietnam
- 3School of Applied Biosciences, Kyungpook National University, Daegu, South Korea
- 4National Institute of Agricultural Science, RDA, Jeonju, South Korea
The RsMYB1 transcription factor (TF) controls the regulation of anthocyanin in radishes (Raphanus sativus), and its overexpression in tobacco and petunias strongly enhances anthocyanin production. However, there are no data on the involvement of RsMYB1 in the mechanisms underlying abiotic stress tolerance, despite strong sequence similarity with other MYBs that confer such tolerance. In this study, we used the anthocyanin-enriched transgenic petunia lines PM6 and PM2, which overexpress RsMYB1. The tolerance of these lines to heavy metal stress was investigated by examining several physiological and biochemical factors, and the transcript levels of genes related to metal detoxification and antioxidant activity were quantified. Under normal conditions (control conditions), transgenic petunia plants (T2-PM6 and T2-PM2) expressing RsMYB1, as well as wild-type (WT) plants, were able to thrive by producing well-developed broad leaves and regular roots. In contrast, a reduction in plant growth was observed when these plants were exposed to heavy metals (CuSO4, ZnSO4, MnSO4, or K2Cr2O7). However, T2-PM6 and T2-PM2 were found to be more stress tolerant than the WT plants, as indicated by superior results in all analyzed parameters. In addition, RsMYB1 overexpression enhanced the expression of genes related to metal detoxification [glutathione S-transferase (GST) and phytochelatin synthase (PCS)] and antioxidant activity [superoxide dismutase (SOD), catalase (CAT), and peroxidase (POX)]. These results suggest that enhanced expression levels of the above genes can improve metal detoxification activities and antioxidant activity, which are the main components of defense mechanism included in abiotic stress tolerance of petunia. Our findings demonstrate that RsMYB1 has potential as a dual-function gene that can have an impact on the improvement of anthocyanin production and heavy metal stress tolerance in horticultural crops.
Introduction
Heavy metals occur naturally in the earth’s crust. However, excess levels of heavy metals produced by natural or anthropogenic activities are detrimental to living organisms. Over the past few decades, advances in industrialization and modern agricultural practices worldwide have led to contamination of cultivatable land with the heavy metals released from agro-chemicals and industrial activities (Yang et al., 2005). Generally, heavy metals, such as Zn, Cu, and Mn, play important roles in plant physiological and biochemical processes, such as chlorophyll biosynthesis, photosynthesis, and DNA synthesis (reviewed by Singh et al., 2015). For example, Zn contributes to the maintenance of membrane integrity, auxin metabolism, and reproduction because it interacts with enzymes and transcription factors (TFs) underlying these processes (Williams and Pittman, 2010; Prasad, 2012; Ricachenevsky et al., 2013). However, the toxic effects of heavy metals at high concentrations have also been well-documented (Fontes and Cox, 1998; Lewis et al., 2001; Warne et al., 2008; Ai et al., 2018). Zn at elevated concentrations can cease plant metabolic functions, causing growth retardation and senescence (Fontes and Cox, 1998; Warne et al., 2008). It has been reported that high Cu concentrations cause a similar range of symptoms (Lewis et al., 2001; Ai et al., 2018). In addition, elevated Mn concentrations are toxic to many plant species (Izaguirre-Mayoral and Sinclair, 2005; Rezai and Farboodnia, 2008), and high Cr levels negatively affect cell division and root and stem growth in many plants (Shanker et al., 2005; Zou et al., 2006; Fozia et al., 2008). Overall, elevated concentrations of these metals lower biomass accumulation and crop productivity by inhibiting several plant mechanisms. Theoretically, the presence of excess heavy metals limits CO2 fixation and reduces photosynthetic electron transport chains in chloroplasts and mitochondria. This leads to the overproduction of reactive oxygen species (ROS), which damage plant cells and inhibit plant growth, thereby reducing crop yields (Davidson and Schiestl, 2001; Mittler et al., 2004; Keunen et al., 2011). Therefore, it is important to understand how plants respond to heavy metal stress at physiological and molecular levels, and to develop plants that can resist stress-induced ROS overproduction and maintain crop productivity.
The roles of glutathione (GSH) and the phytochelatin synthase (PCS) gene in reducing heavy metal stress and ROS scavenging have been documented (Millar et al., 2003; Freeman et al., 2004; Foyer and Noctor, 2005; Hirata et al., 2005; Shao et al., 2008; Ai et al., 2018). The roles of antioxidants in scavenging ROS and reducing the oxidative stress caused by heavy metals have also been investigated (Hirschi et al., 2000; Tseng et al., 2007). As anthocyanin-enriched plants contain higher levels of antioxidants, which can effectively scavenge ROS, these plants are able to survive abiotic and biotic stress conditions (Winkel-Shirley, 2002; Dixon et al., 2005; Agati et al., 2011; Fini et al., 2011; Dehghan et al., 2014; Nakabayashi et al., 2014). Transgenic potato plants overexpressing IbMYB1 (Cheng et al., 2013) and transgenic tobacco plants overexpressing the snapdragon Delila (Del) gene (Naing et al., 2017) showed enhanced anthocyanin production and improved abiotic tolerance. The results of previous studies have emphasized the importance of producing anthocyanin-enriched plants, which can provide antioxidants responsible for scavenging ROS to overcome abiotic stress conditions. Lim et al. (2016) indicated that overexpression of RsMYB1 enhanced anthocyanin levels and antioxidant activity. Certain studies have demonstrated the role of MYB, GSH, and PCS in heavy metal tolerance in maize and walnut plants (Li et al., 2017; Xu et al., 2018); however, the yield penalty caused by the specific heavy metals was not described. Recently, Ai et al. (2017) showed that overexpression of RsMYB1 enhanced anthocyanin accumulation in petunias. However, they did not investigate whether the anthocyanin-enriched transgenic plants expressing RsMYB1 could tolerate heavy metal stress. Therefore, we aimed to investigate the stress tolerance of anthocyanin-enriched transgenic plants, which has not been adequately addressed to date.
In the present study, we used anthocyanin-enriched T2 transgenic petunia lines (PM2 and PM6) expressing RsMYB1, which were developed by successive pollination of the T0 transgenic lines reported previously (Ai et al., 2017), in order to investigate whether they are able to tolerate heavy metal stress. The tolerance of PM2 and PM6 to heavy metal stress relative to that of the wild-type (WT) plants was investigated by examining several physiological and biochemical factors. In addition, the transcript levels of genes related to metal detoxification and antioxidant activity were investigated.
Materials and Methods
Plant Materials
The transgenic petunia lines, PM6 and PM2, expressing RsMYB1, which were developed in our previous work (Ai et al., 2017), showed visible anthocyanin pigmentation in the whole plant; therefore, we selected these lines to be examined for heavy metal stress tolerance.
Production of the T2 Generation
First, the T0-PM6 and T0-PM2 lines grown in a greenhouse were self-pollinated to obtain T1 lines, which were then screened visually for the anthocyanin phenotype. Second, the screened T1 lines were self-pollinated to obtain T2 seeds, and these seeds were used as the source plant material for experimentation.
Detection of Anthocyanin Content and ROS-Scavenging Activity
To determine the anthocyanin content of the T2-PM6, T2-PM2, and WT plants, their seeds were grown in a greenhouse for 6 weeks. Six-week-old T2-PM6 and T2-PM2 plants, which showed the anthocyanin phenotype, and WT seedlings were selected for the analysis of total anthocyanin content and ROS-scavenging activity.
Total anthocyanin extraction was performed following our previously published procedure (Ai et al., 2017). Briefly, fresh leaves (fifth top leaves, ∼500 mg) were collected and ground to a fine powder, which was transferred to the extraction solution. The mixture was incubated at 4°C for 24 h and then centrifuged at 13,000 rpm and 4°C for 20 min. The anthocyanin content of the supernatant was quantified with a spectrophotometer (Shimadzu, Kyoto, Japan). For determining ROS-scavenging activity, fresh leaves (fifth top leaves, 2.0 g) were collected from T2-PM6, T2-PM2, and WT seedlings, and the activity was measured using 2,2′-azino-bis(3-ethylbenzothiazoline-6-sulfonic acid) diammonium salt (ABTS) and 1,1-diphenyl-2-picrylhydrazyl (DPPH) assays (Kim et al., 2014; Lim et al., 2016). Three biological samples were used for each of the T2-PM6, T2-PM2, and WT seedlings, and each measurement was repeated thrice.
Phylogenetic Analysis of RsMYB1
The amino acid sequence of RsMYB1 was aligned with those of 35 MYB TFs involved in tolerance to different abiotic stresses (e.g., cold, salt, drought, and heavy metal stress) in several plant species using MEGA7 software; a phylogenetic tree was constructed using the maximum likelihood method. Initial trees for the heuristic search were obtained automatically by applying the neighbor-joining and BioNJ algorithms to a matrix of pairwise distances estimated using a Jones–Taylor–Thornton (JTT) model.
In vitro Seed Germination and Heavy Metal Treatments
For in vitro seed germination, seeds of the T2-PM6, T2-PM2, and WT plants were soaked in 0.05% sodium hypochlorite (Yuhan Co., Ltd., Seoul, South Korea) containing 0.01% Tween 20 (Duchefa, Haarlem, Netherlands) for 10 min and then rinsed with sterile distilled water at least thrice. The sterilized T2-PM6 and T2-PM2 seeds were sown in Murashige and Skoog (MS) basal medium containing 3% sucrose, 1 mg⋅L-1 phosphinothricin (PPT), and 0.8% agar to obtain only the seedlings expressing RsMYB1; WT seeds were cultured on the same medium without PPT. The cultures were incubated at 25 ± 2°C with a 16 h photoperiod and a light intensity of 50 μmol m-2 s-1 for 30 days.
The T2-PM6 and T2-PM2 seedlings that were red and uniformly sized were selected for the heavy metal stress experiment. The T2-PM6, T2-PM2, and WT seedlings were then stressed by continuous culturing in MS liquid medium containing increasing concentrations of CuSO4, ZnSO4, K2Cr2O7 (25, 50, and 100 μM for each salt), or MnSO4 (100, 250, and 500 μM) for 10 days per concentration on a rotary shaker set to 50 rpm. The concentrations were chosen based on those used in previous studies (Baek et al., 2012; Liu et al., 2015). MS liquid medium without heavy metals was used as the control. The culture conditions were the same as described above. Each treatment contained 20 seedlings, and there were three replicates.
Effects of Different Heavy Metals
After the plants were treated with the final concentrations of CuSO4, ZnSO4, K2Cr2O7 (100 μM), and MnSO4 (500 μM) (see section 2.5), the total time taken for the treatment periods was 30 days (from initial to final concentration treatment). At the end of the stress period, 15 plants each of T2-PM6, T2-PM2, and WT cultured in heavy metal-free media (control condition) and heavy metal-containing media (stress conditions) were randomly selected for physiological, biochemical, and molecular analyses. These analyses included plant height, fresh weight, stomatal density, fluorescence, photosynthetic pigment content, relative water content (RWC), heavy metal uptake, and gene expression. The measurements were taken thrice, and the data represent the means of three replicates.
Measurement of RWC
Relative water content was measured using the seventh leaf from the top of T2-PM6, T2-PM2, and WT plants subjected to 30 days of heavy metal stress or control conditions. Fresh leaf weight was immediately recorded after excision from the plants. The leaves were then floated in deionized water at 4°C overnight, and their rehydrated weights were recorded. Finally, the leaves were oven-dried at 70°C overnight, and their dry leaf weight was recorded. The formula for determining RWC was as follows: RWC = (fresh weight-dry weight)/(rehydrated weight-dry weight). Five leaves each from the T2-PM6 and WT plants were used to determine RWC, and the analysis was repeated three times.
Determination of Chlorophyll Content
Following the stress, chlorophyll content was measured using the fifth leaf from the top of the T2-PM6, T2-PM2, and WT plants, according to the method of Baek et al. (2012). Briefly, the leaves were homogenized in 15 mL methanol, and the homogenate was filtered through two layers of cheesecloth. This was followed by centrifugation at 3,000 × g for 10 min. The total chlorophyll content in the supernatant was measured and calculated using the formula described by Wellburn (1994).
Detection of Anthocyanin Content and ROS-Scavenging Activity
Following the stress, anthocyanin content and ROS-scavenging activity (the latter using DPPH and ABTS assays) were measured using the seventh leaf from the top from the T2-PM6, T2-PM2, and WT plants. The protocols were identical to those used in the above experiment (Kim et al., 2014; Lim et al., 2016; Ai et al., 2017). Three biological samples were used for each of the T2-PM6, T2-PM2, and WT plants, and each measurement was repeated thrice.
Determination of Stomatal Density
To determine whether the heavy metal treatments affected stomatal density in the T2-PM6, T2-PM2, and WT plants, 2-cm-long leaf segments (middle part) from the fifth leaves from the top were excised with scalpel blades. The excised leaf segments were immediately fixed in formalin–acetic acid–alcohol and kept overnight according to the protocol used by Naing et al. (2015). The samples were then dehydrated for 10 min using serial ethanol concentrations (25, 50, 70, 85, and 100%). The dehydrated samples were dried to their critical point at room temperature and then coated with gold-palladium on a Quick Cool Coater (Sanyu-Denshi, Japan). The stomatal density of each sample was examined using a scanning electron microscope (SEM; JEOL Ltd., Tokyo, Japan). Investigations were performed on three samples per treatment with three replicates.
RNA Extraction and Gene Expression Analysis by Quantitative Reverse Transcription PCR (qRT-PCR)
The transcript levels of genes related to metal detoxification [glutathione S-transferase (GST) and PCS] and antioxidant activity [superoxide dismutase (SOD), catalase (CAT), and peroxidase (POX)] in the T2-PM6, T2-PM2, and WT plants with and without heavy metal stress were investigated. Total RNA was isolated from 100 mg of leaf tissue per treatment using the TRI ReagentTM (Ambion, United States). Exactly 1 μg of total RNA and an oligo (dT)20 primer were used for reverse transcription (ReverTra Ace-α®, Toyobo, Japan). Then, the transcript levels of the genes (GST, PCS, SOD, CAT, POX, and actin) were measured using a StepOnePlusTM Real-Time PCR system (Thermo Fisher Scientific, Waltham, MA, United States) (Naing et al., 2017). Relative gene expression was calculated using the quantitative-comparative CT (ΔΔCT) method. The primers and PCR conditions for the detected genes are listed in Table 1. Three samples per plant line were used, and the analysis was repeated three times.
Uptake of Heavy Metals
Exactly 1 g of dried leaf tissue per treatment was collected from the T2-PM6, T2-PM2, and WT plants to determine heavy metal uptake (Cu, Zn, Mn, and Cr). The analysis was carried out as described by Cataldi et al. (2003). There were three samples per treatment and three replicates.
Statistical Analysis
Data were collected on day 30 of the experiment and statistically analyzed using SPSS version 11.09 (IBM Corporation, Armonk, NY, United States). The results are presented as the means ± SE. Least significant difference tests were used to compare the means, and the significance was set at P < 0.05.
Results
Detection of Anthocyanin Content and ROS-Scavenging Activity
All of the homozygous T2-PM6 and T2-PM2 plants, which were obtained by successive self-pollination of T0 and T1 plants, displayed anthocyanin-containing phenotypes; the total leaf anthocyanin content in T2-PM6 was significantly higher than that in T2-PM2, followed by that in the WT plants (Figure 1A). To determine the association between anthocyanin content and ROS-scavenging activity, we measured the latter in the T2-PM6, T2-PM2, and WT plants using ABTS and DPPH assays. As shown in Figures 1B,C, the ABTS and DPPH activities were highest in the T2-PM6 plants, followed by T2-PM2 and WT plants, indicating that higher ROS-scavenging activity depends on the anthocyanin content of the plants.
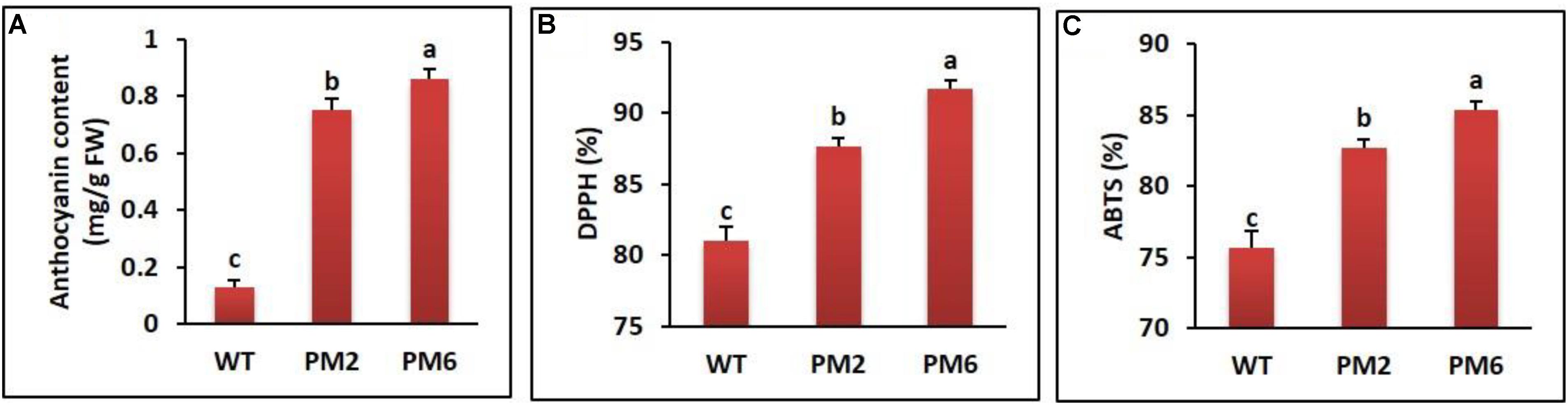
FIGURE 1. Comparison of anthocyanin content (A) and ROS-scavenging activities assessed by DPPH (B) and ABTS (C) assays in the T2-PM6, T2-PM2, and the wild-type (WT) plants grown in the greenhouse for 6 weeks. Error bars indicate the SEM. FW, fresh weight.
Phylogenetic Analysis of RsMYB1
The ROS-scavenging activity experiments demonstrated that the T2-PM6 and T2-PM2 plants expressing RsMYB1 had higher-ROS scavenging activity than the WT plants. Thus, it was of interest to compare the degree of abiotic stress tolerance in the T2-PM6 and T2-PM2 plants to that in the WT plants. Before the stress treatment, we clarified the potential role of RsMYB1 in abiotic stress tolerance by building a phylogenetic tree based on the full-length amino acid sequences of 35 R2R3-MYB TFs isolated from different species that have been found to be tolerant to different abiotic stresses (cold, drought, salt, and heavy metals). The resulting tree indicated that RsMYB1 was phylogenetically related to other MYB TFs and was clustered with six TFs (IbMYB1, OsMYB4, GmMYB92, DwMYB2, OsMYB2, and TaMYB19), which confer tolerance to different abiotic stresses in various crops (Figure 2). This suggested that RsMYB1 has the same functional role as that of the six TFs. According to the phylogenetic tree, RsMYB1 has high sequence similarity with IbMYB1, which is associated with anthocyanin accumulation and salt tolerance.
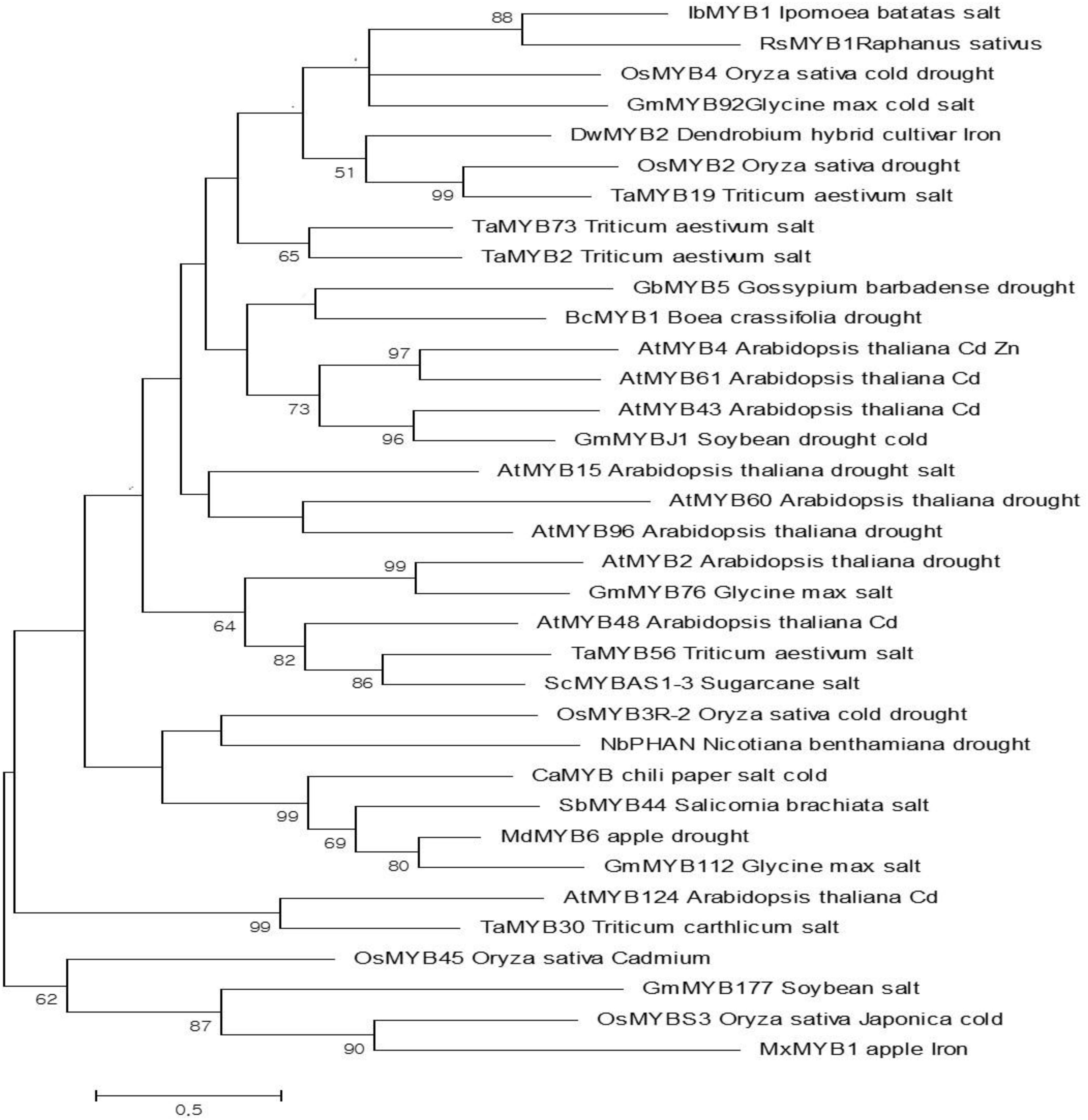
FIGURE 2. Phylogenetic relationships of the RsMYB1 transcription factor (TF) with other MYB TFs that confer tolerance to various abiotic stresses. The evolutionary history was inferred by using the maximum likelihood method based on the Poisson correction model [1]. The tree with the highest log likelihood (–4439.60) is shown. Initial tree(s) for the heuristic search were obtained automatically by applying neighbor-joining and BioNJ algorithms to a matrix of pairwise distances estimated using a JTT model and then selecting the topology with superior log likelihood value. The tree is drawn to scale, with branch lengths measured in the number of substitutions per site. The analysis involved 35 amino acid sequences. All positions containing gaps and missing data were eliminated. There were 55 positions in the final dataset. Evolutionary analyses were conducted in MEGA7 [2].
Assessment of Plant Growth Parameters Under Heavy Metal Stress
The growth of the T2-PM6, T2-PM2, and WT plants in response to the stress of various heavy metals (CuSO4, ZnSO4, MnSO4, and K2Cr2O7), as well as under normal growing conditions (without heavy metals), was evaluated 30 days after the treatments began. Survival of the control plants was high, and the plants produced well-developed, broad leaves and regular roots. When the plants were treated with CuSO4, ZnSO4, K2Cr2O7 (25 μM each), or MnSO4 (100 μM) for 10 days, the growth parameters were not significantly different from those of the plants grown under normal conditions (data not shown). However, the growth parameters started to decrease when the plants were continuously treated with higher concentrations of heavy metals (50 μM of CuSO4, ZnSO4, K2Cr2O7, or 250 μM MnSO4) for another 10 days (data not shown), and they significantly decreased when the plants were subjected to the highest concentrations (100 μM of CuSO4, ZnSO4, K2Cr2O7, or 500 μM MnSO4) for an additional 10 days. The T2-PM6 plants were found to be more tolerant to heavy metal stress than the T2-PM2 plants were, followed by the WT plants (i.e., PM6 > PM2 > WT), which was similar to the growth parameter responses in the plants (i.e., PM6 > PM2 > WT) (Figures 3A,B). In addition, the degree of tolerance to the heavy metals varied depending on the heavy metal used. CuSO4 and ZnSO4 were found to be the most toxic to the plants, particularly the WT plants. Overall, the presence of high concentrations of different heavy metals significantly inhibited plant growth compared to that under normal growing conditions. Furthermore, more severe toxicity was clearly observed in the WT plants, followed by the T2-PM2 and T2-PM6 plants. Therefore, the anthocyanin-enriched plants had enhanced resistance to heavy metal stress. To demonstrate tolerance of the anthocyanin-enriched plants to heavy metal stress, physio-biochemical factors (e.g., chlorophyll content, RWC, anthocyanin content, ROS-scavenging activities, and stomatal density), accumulation of the heavy metals, and expression levels of genes related to metal detoxification and antioxidant activities were measured subsequently in the plants.
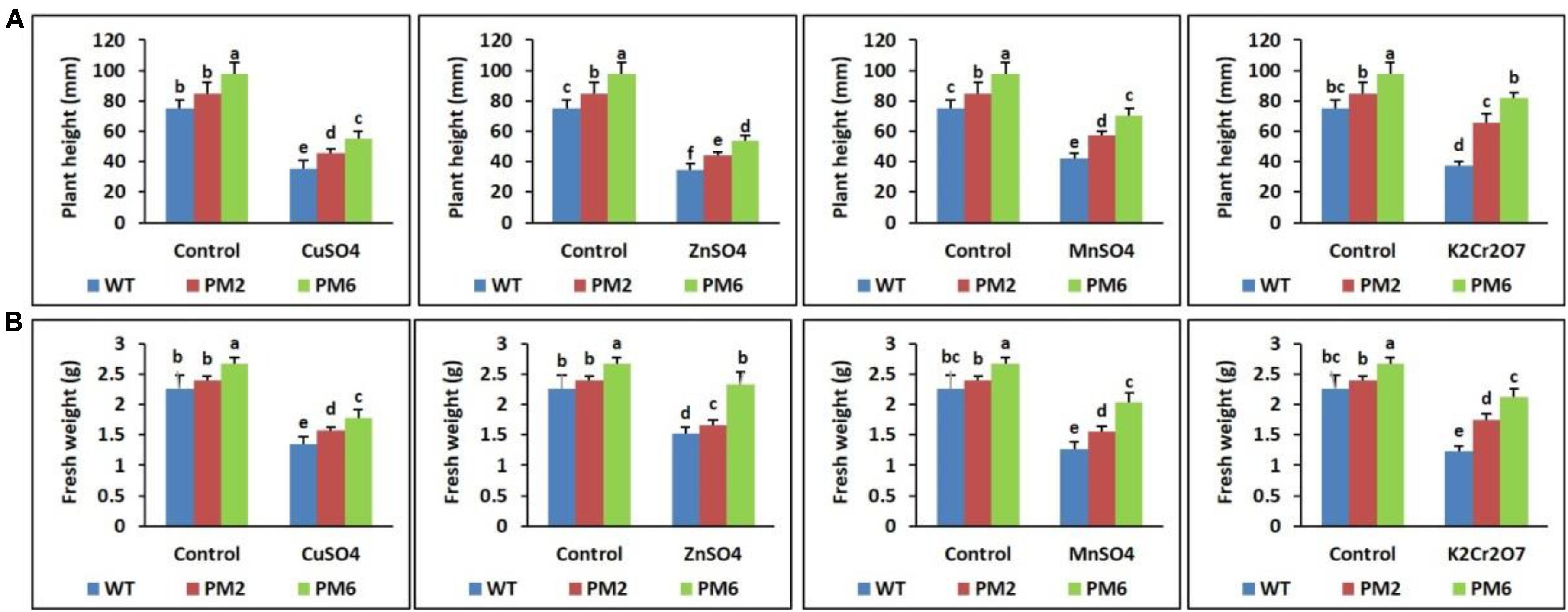
FIGURE 3. Comparisons of plant height (A) and fresh weight (B) among the T2-PM6, T2-PM2, and the WT plants treated with the indicated heavy metal salts. Data were acquired on the thirtieth day after starting the experiments. Error bars show the SEM.
Assessment of Chlorophyll Content and RWC
Following stress, the chlorophyll content and RWC of the T2-PM6, T2-PM2, and WT plants were lower under stress conditions than under normal growing conditions (control). The levels detected in the T2-PM6 plants were significantly higher than those in the T2-PM2 followed by the WT plants, particularly under stress conditions (Figures 4, 5).
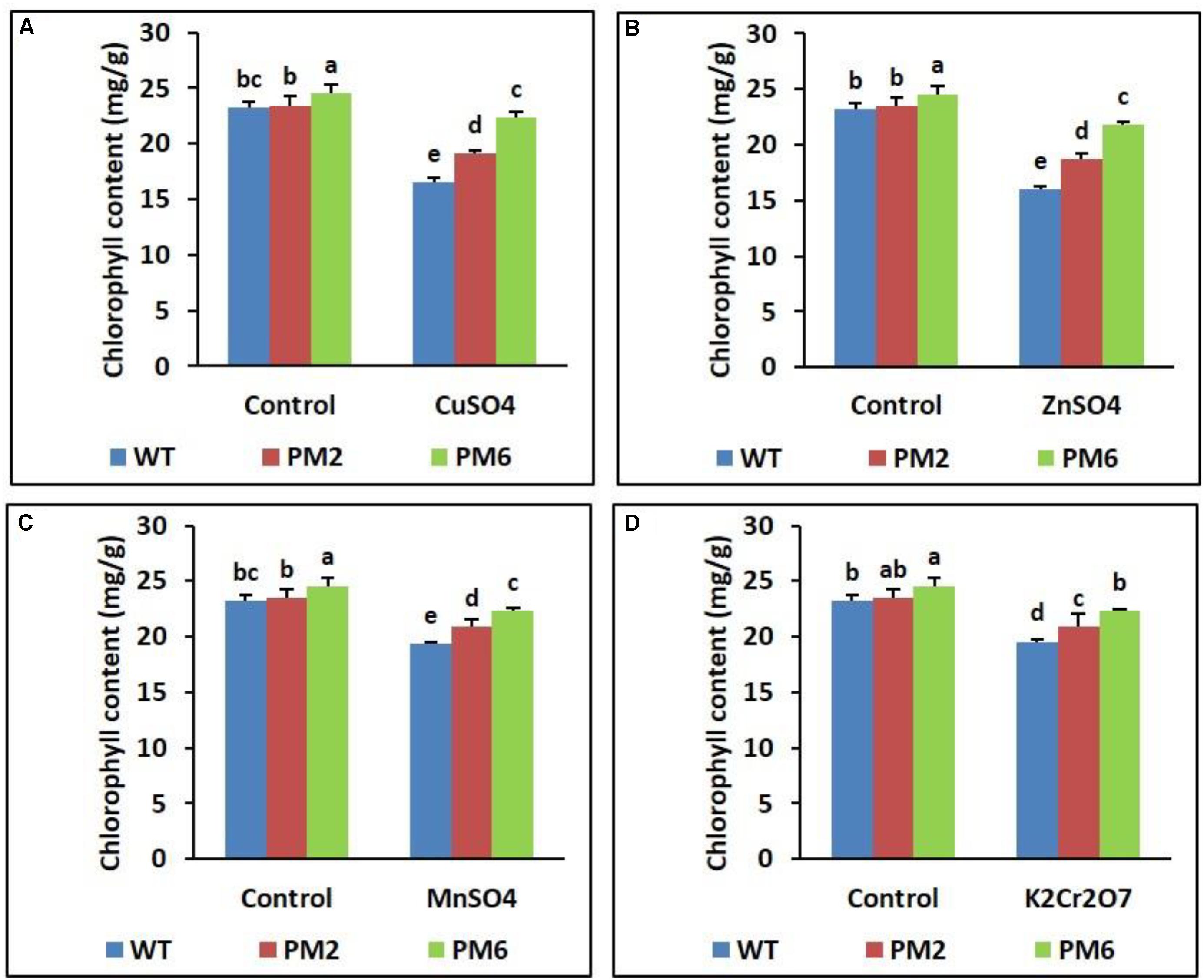
FIGURE 4. Comparison of chlorophyll content in the T2-PM6, T2-PM2, and WT plants after exposure to the indicated heavy metals (A, CuSO4; B, ZnSO4; C, MnSO4; D, K2Cr2O7). Data were acquired on the thirtieth day after starting the experiments. Error bars show the SEM.
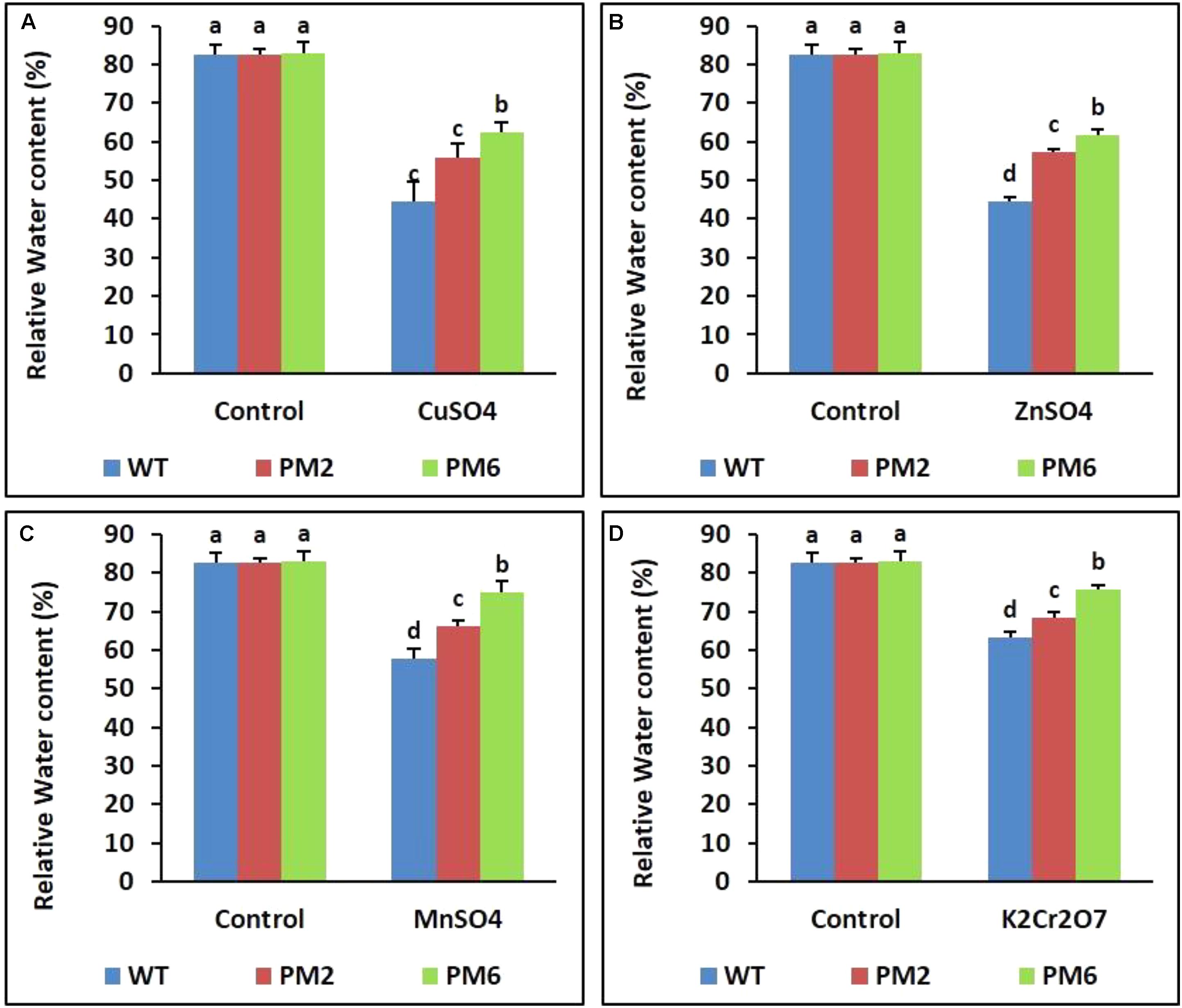
FIGURE 5. Comparison of relative water content (RWC) in the T2-PM6, T2-PM2, and WT plants after exposure to the indicated heavy metals (A, CuSO4; B, ZnSO4; C, MnSO4; D, K2Cr2O7). Data were acquired on the thirtieth day after starting the experiments. Error bars show the SEM.
Assessment of Anthocyanin Content and ROS-Scavenging Activity
The anthocyanin content and ROS-scavenging activity (ABTS and DPPH assays) in the T2-PM6, T2-PM2, and WT plants were measured. Significant reductions in these values were observed in all plants under stress conditions. However, a more severe reduction was observed in the WT plants than in the T2-PM6 and T2-PM2 plants (Figures 6A–C).
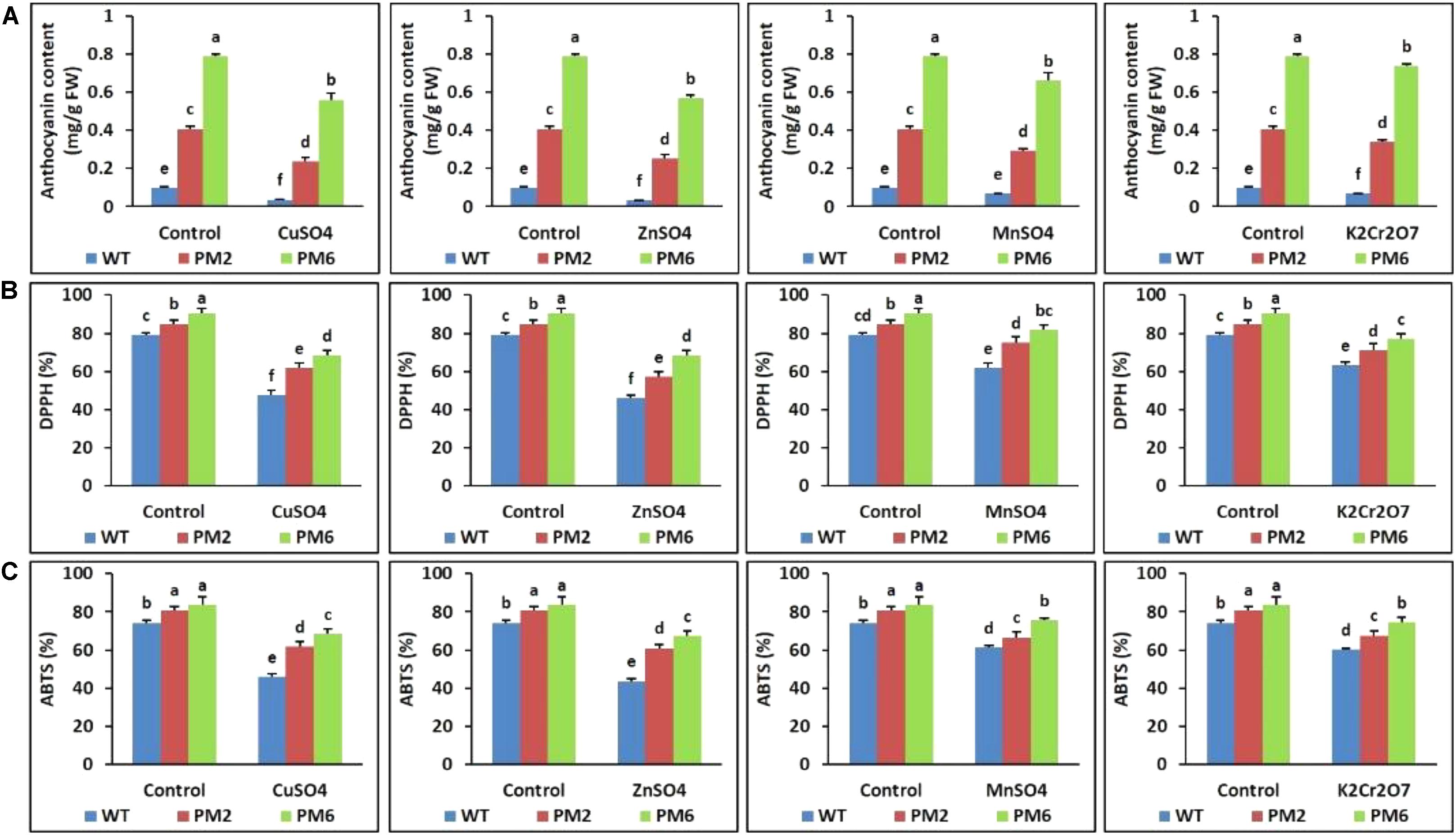
FIGURE 6. Comparison of anthocyanin content (A) and ROS-scavenging activities assessed by DPPH (B) and ABTS (C) assays in the T2-PM6, T2-PM2, and WT plants after exposure to the indicated heavy metals. Data were collected on the thirtieth day after starting the experiments. Error bars show the SEM.
Reduction in Stomatal Density Under Heavy Metal Stress
The stomatal densities of the T2-PM6, T2-PM2, and WT plants were investigated using an SEM to determine whether heavy metals affected stomatal density. Under normal growth conditions (control), high stomatal density was observed in the T2-PM6, T2-PM2, and WT plants. However, stomatal density decreased when the plants were exposed to the heavy metals (Figure 7). In addition, the extent of stomatal reduction varied depending on the type of heavy metal used.
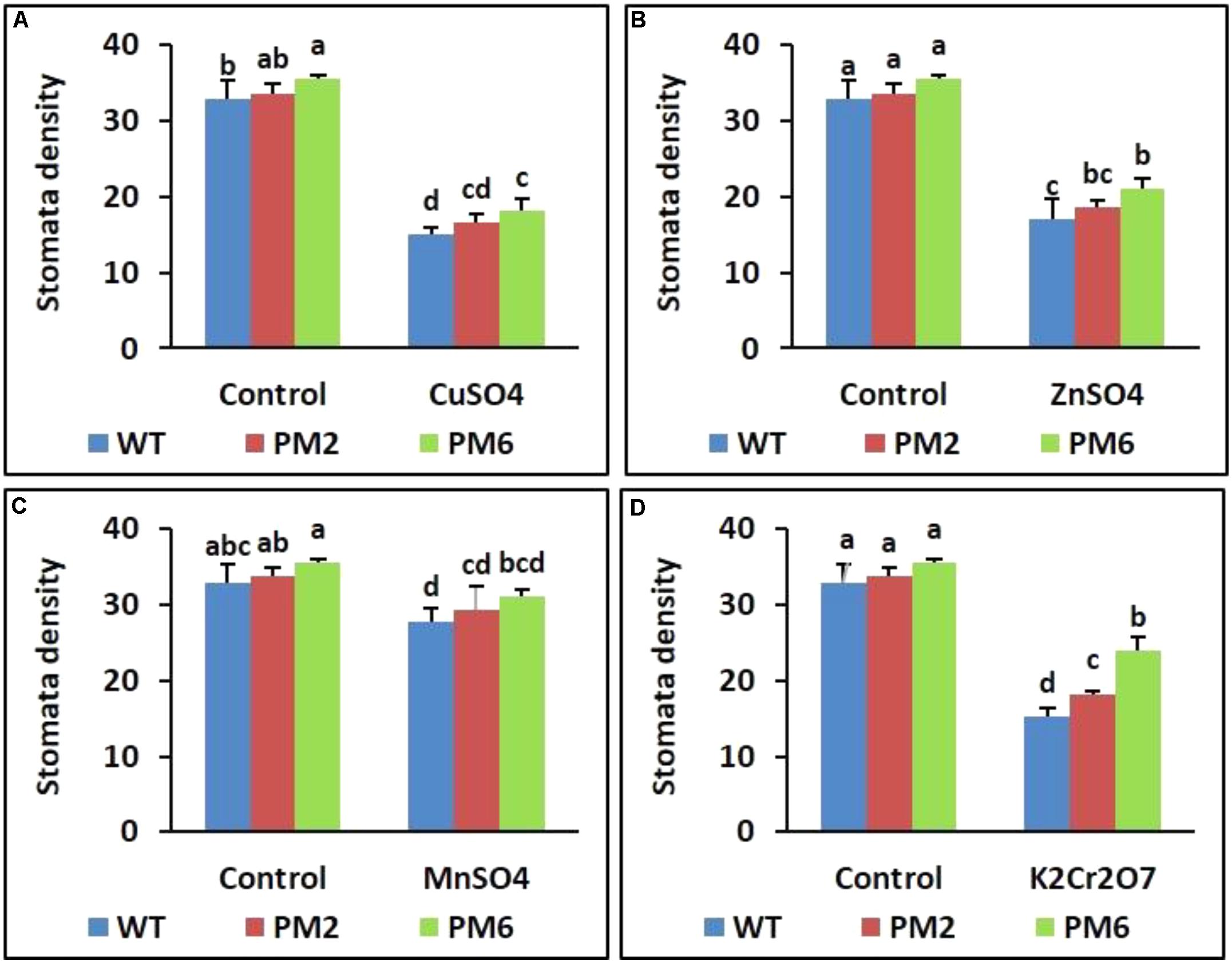
FIGURE 7. Comparison of stomatal density in the T2-PM6, T2-PM2, and WT plants after exposure to the indicated heavy metals (A, CuSO4; B, ZnSO4; C, MnSO4; D, K2Cr2O7). Data were taken on the thirtieth day after starting the experiments. Error bars show the SEM.
Expression Profiles of Genes Related to Antioxidant Activity
qRT-PCR was used to clarify the expression profile of antioxidant genes (i.e., SOD, CAT, and POX) in the T2-PM6, T2-PM2, and WT plants with and without heavy metal treatment. The results show that the stress treatments increased the transcript levels of the tested genes in all plants compared with those under normal growing conditions (control). However, the genes were more highly expressed in T2-PM6, followed by T2-PM2, and then WT plants under stress conditions with all heavy metals (Figures 8A–C). Therefore, the expression levels of the genes paralleled the degree of tolerance of the plants to heavy metal stress.
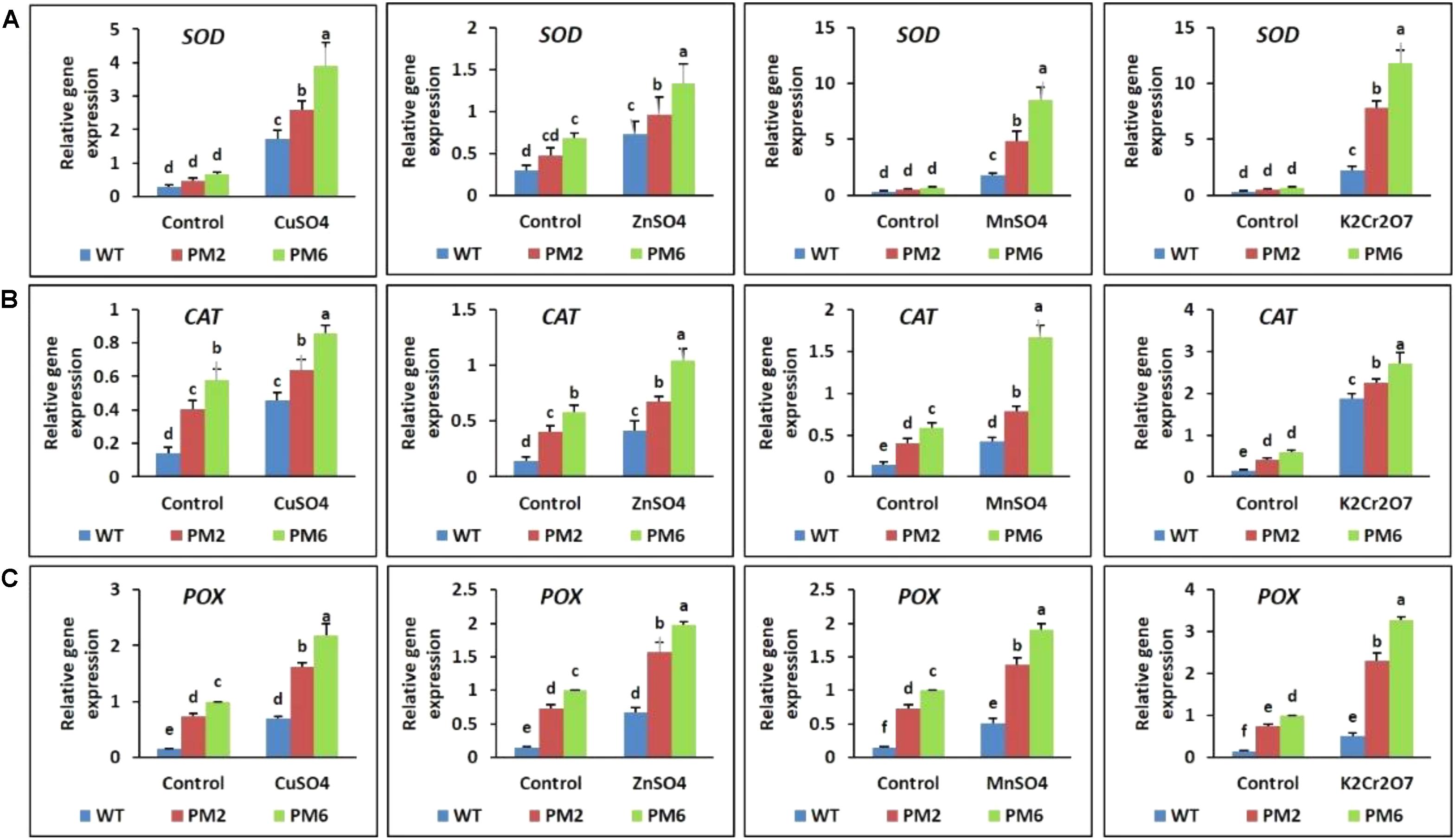
FIGURE 8. Expression analysis of the antioxidant-related genes superoxide dismutase [SOD, (A)], catalase [CAT, (B)], and peroxidase [POX, (C)] in the T2-PM6, T2-PM2, and WT plants after exposure to the indicated heavy metals. Data were taken on the thirtieth day after starting the experiments. Error bars show the SEM.
Expression Profiles of Genes Related to Metal Detoxification
The expression levels of genes related to metal detoxification (i.e., GST and PCS), which are normally regulated by heavy metal stress, were analyzed by qRT-PCR. When the plants were subjected to heavy metal stress, their expression patterns of GST and PCS were similar to the antioxidant gene patterns described in section 3.7. Expression of the genes in the T2-PM6, T2-PM2, and WT plants was low under normal growth conditions, but their expression increased when the plants were exposed to heavy metal stress. However, the stress-induced increases in expression were higher in the T2-PM6 plants, followed by the T2-PM2 and WT plants (Figures 9A,B), which was consistent with the degree of heavy metal tolerance of the plants (i.e., T2-PM6 > T2-PM2 > WT).
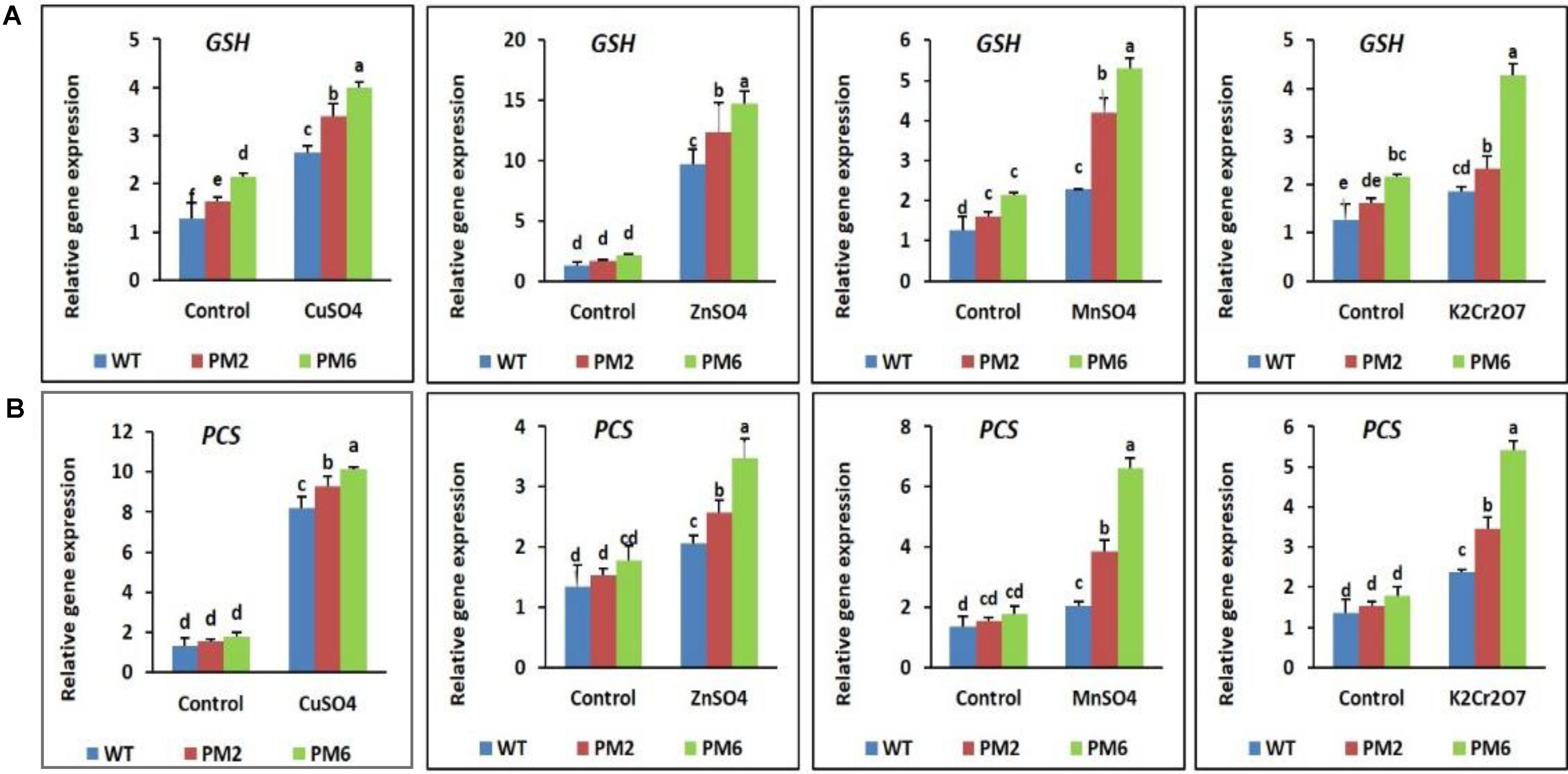
FIGURE 9. Expression analysis of the heavy metal stress tolerance genes glutathione S-transferase [GST, (A)] and phytochelatin synthase [PCS, (B)] in the T2-PM6, T2-PM2, and WT plants after exposure to the indicated heavy metals. Data were taken on the thirtieth day after starting the experiments. Error bars show the SEM.
Accumulation of Heavy Metals in the T2-PM6, T2-PM2, and WT Plants
Under normal growth conditions, the heavy metal content of the T2-PM6, T2-PM2, and WT plants was quite low, and the three plant types did not significantly differ in their content. However, when the plants were exposed to different heavy metals, metal uptake significantly increased, but the total content of detectable metals per plant was significantly higher in the order T2-PM6 > T2-PM2 > WT (Figure 10).
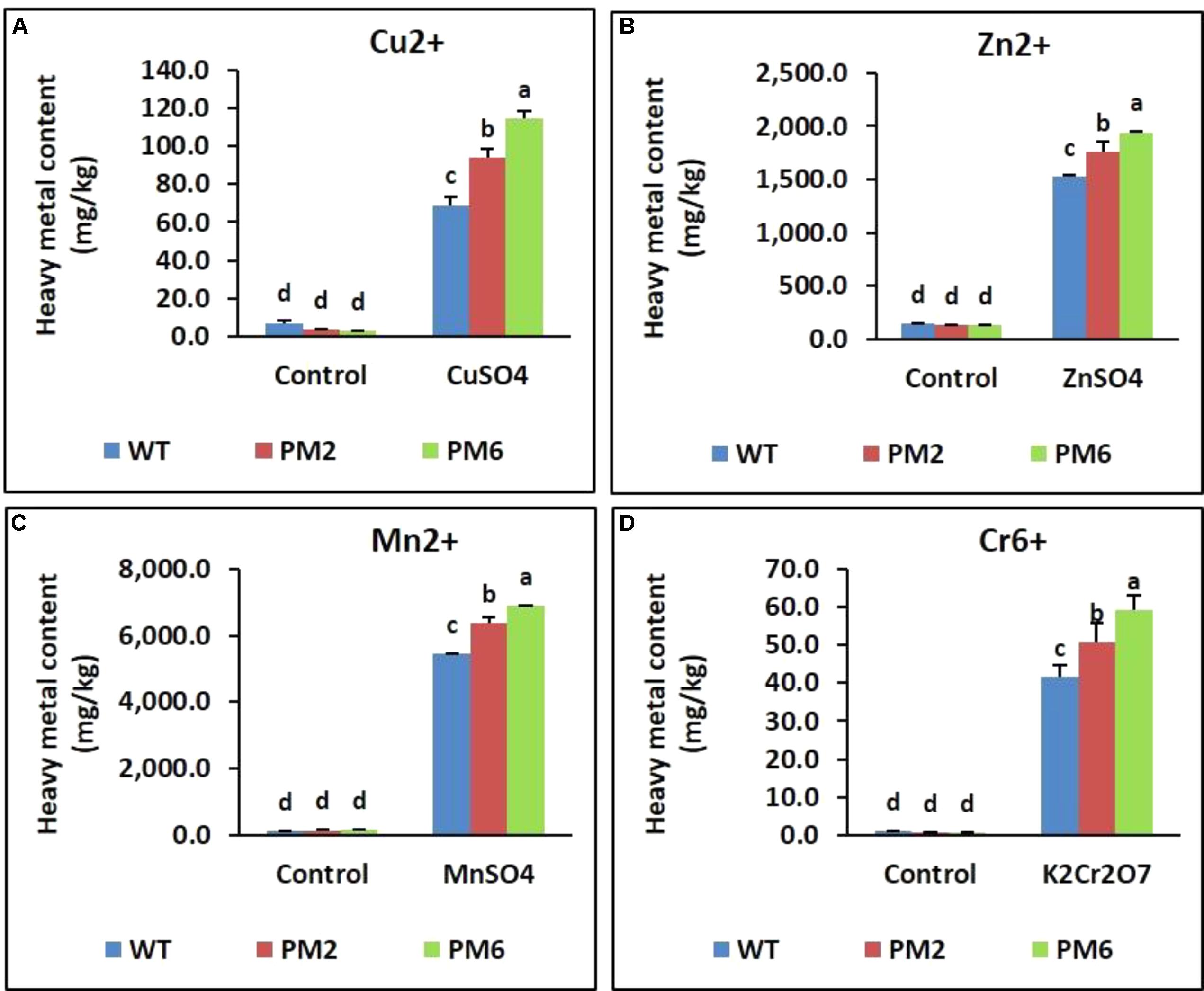
FIGURE 10. Heavy metal content [Cu2+ (A), Zn2+ (B), Mn2+ (C), and Cr6+ (D)] accumulated in leaf tissue following heavy metal-stress treatment of the T2-PM6, T2-PM2, and WT plants. Data were taken on the thirtieth day after starting the experiments. Error bars indicate the SEM.
Taken together, overexpression of RsMYB1 in transgenic petunias enhanced anthocyanin content, which led to higher antioxidant activity to scavenge the ROS induced by heavy metal stress. Moreover, higher expression levels of genes related to metal detoxification and antioxidant activity were detected in the transgenic lines expressing RsMYB1. The sequence of RsMYB1 is similar to that of other MYBs that confer tolerance to various abiotic stresses. We suggest that the transgenic lines have advantages that impart a greater capability to tolerate heavy metal stress when the plants are treated for 30 days.
Discussion
Anthropogenic activities have led to a continuous increase in heavy metal contamination of agricultural soil. This is becoming a major concern worldwide (Rascio and Navari-Izzo, 2011; Villiers et al., 2011) because these contaminated soils negatively affect plant physiological processes through the generation of ROS, which results in lower crop yields (DalCorso et al., 2008; Hossain et al., 2009, 2010; Rascio and Navari-Izzo, 2011; Villiers et al., 2011). Therefore, heavy metal toxicity and the defense mechanisms used by plants to scavenge ROS and detoxify heavy metals need to be elucidated (Rascio and Navari-Izzo, 2011). Previous studies have shown that antioxidants are involved in the scavenging of ROS generated by heavy metal stress (Hirschi et al., 2000; Mittler et al., 2004; Tseng et al., 2007; Ai et al., 2018). In addition, the roles of GSH and PCS in the detoxification of heavy metals and ROS scavenging have been documented (Millar et al., 2003; Freeman et al., 2004; Foyer and Noctor, 2005; Hirata et al., 2005; Shao et al., 2008). The enhancement of antioxidant activity and stress tolerance in anthocyanin-enriched plants has been reported for various plant species (Winkel-Shirley, 2002; Dixon et al., 2005; Agati et al., 2011; Dehghan et al., 2014; Ai et al., 2018). Recently, the overexpression of MYB TFs in various species has been shown to enhance anthocyanin accumulation and abiotic stress tolerance (Cheng et al., 2013; Meng et al., 2014; Qi et al., 2015; Yuan et al., 2015). In our previous study, the petunia transgenic lines, PM6 and PM2, which express RsMYB1, distinctly and consistently enhanced anthocyanin accumulation (Ai et al., 2017); however, we did not investigate whether these lines were tolerant to heavy metal stress.
Before commencing the stress experiment, we produced homozygous T2-PM6 and T2-PM2 plants by successive self-pollination of T0 and T1 plants. The T2-PM6 and T2-PM2 plants showed stable anthocyanin-containing phenotypes and contained higher anthocyanin levels and ROS-scavenging activities (as assessed by ABTS and DPPH assays) than did the WT plants, suggesting that the higher anthocyanin accumulation is linked to greater ROS-scavenging activity, as has been reported previously (Fini et al., 2011; Nakabayashi et al., 2014). Moreover, these results support the findings of Lim et al. (2016), who reported that RsMYB1 overexpression in Arabidopsis strongly enhances anthocyanin production and promotes ROS-scavenging activity; however, the authors did not examine the role of transgenic Arabidopsis plants overexpressing RsMYB1 in protecting against abiotic stress conditions. According to our phylogeny results, the sequence of RsMYB1 is phylogenetically related to six MYBs (i.e., IbMYB1, OsMYB4, GmMYB92, DwMYB2, OsMYB2, and TaMYB19), which confer tolerance to various abiotic stresses in these crops. Owing to the presence of high anthocyanin levels and ROS-scavenging activity, as well as sequence similarity with other MYBs that confer tolerance to abiotic stress, we wanted to investigate the heavy metal stress tolerance of the T2-PM6, T2-PM2, and WT plants by measuring physiological and biochemical parameters and expression levels of genes involved in the tolerance to heavy metal stress.
In this study, the T2-PM6, T2-PM2, and WT plants survived under normal growth conditions and their growth parameters (i.e., plant height, root length, and fresh weight) were not significantly different. However, when they were exposed to different heavy metal salts (i.e., CuSO4, ZnSO4, MnSO4, or K2Cr2O7), signs of plant growth inhibition, water deficiency, and chlorophyll degradation were observed, which were more severe in the WT plants. This was corroborated with the data showing reduced chlorophyll and RWC in the T2-PM6, T2-PM2, and WT plants (with respect to reductions, WT > T2-PM2 > T2-PM6). It seems that heavy metal stress reduced the formation of roots, which take up water, and interrupted the synthesis of chlorophyll, which is required for plant photosynthesis, leading to the reduction in RWC and chlorophyll content. Among the heavy metals tested, the toxicity caused by CuSO4 and ZnSO4 was more severe than that of the others, because leaf chlorosis and plant growth inhibition were more severe in the plants treated with these metals. The effects of CuSO4 and ZnSO4 exposure in this study confirmed the results previously reported by Ebbs and Kochian (1997) and Lewis et al. (2001). Heavy metals have been reported to be toxic to plants and cause injuries through the generation of ROS, which disturb physiological functions (Ebbs and Kochian, 1997; Lewis et al., 2001; Sharma et al., 2003; Scoccianti et al., 2006). However, in this study, the degree of tolerance to heavy metals was T2-PM6 > T2-PM2 > WT plants, which is probably due to the greater ROS-scavenging ability (determined via DPPH and ABTS assays) in the anthocyanin-enriched plants (T2-PM6 > T2-PM2) than in the WT plants. The role of anthocyanin-induced ROS-scavenging activity in the tolerance to different abiotic stresses has been established in previous studies (Hossain et al., 2009; Cheng et al., 2013; Nakabayashi et al., 2014; Qi et al., 2015; Yuan et al., 2015). In addition, the presence of higher RWC in the T2-PM6 and T2-PM2 plants than that in the WT plants could be caused by higher ROS-scavenging activities in the former plants, because these activities can detoxify heavy metals accumulated in the roots, allowing the roots to take up water easily (Hirschi et al., 2000; Tseng et al., 2007). However, ROS-scavenging activity and anthocyanin content were also lower in the plants under stress conditions than in the plants under normal growing conditions. This outcome might be due to antioxidant activity to defend against metal-induced ROS stress. In addition, the occurrence of higher toxicity of heavy metals in the WT than in the T2-PM6 and T2-PM2 plants could be caused by the absence of sufficient anthocyanin, which scavenges ROS, suggesting that high ROS-scavenging activity is necessary to counter heavy metal-induced stress.
Theoretically, stomata play a critical role in photosynthesis because they are responsible for uptake of carbon dioxide from the atmosphere and release of oxygen. In this study, inhibition of plant growth under heavy metal stress was associated with a reduction in stomatal density. This suggests that the stomatal density reduction caused by heavy metal stress would decrease leaf photosynthesis, thereby reducing plant growth. These results support the findings reported by Yilmaz et al. (2009) and Kambhampati et al. (2005), who also reported that heavy metals reduced stomatal density.
The expression levels of the antioxidant genes (SOD, CAT, and POX) responsible for scavenging or neutralizing ROS were investigated to reveal the mechanisms underlying the higher tolerance of the plants to heavy metal stress (T2-PM6 > T2-PM2 > WT plants). The expression levels of these genes were significantly higher under heavy metal stress than under normal growth conditions for the T2-PM6, T2-PM2, and WT plants. This might occur because the plants enhanced gene expression to defend against ROS formation caused by the heavy metals. This supports the findings of previous studies (Singh et al., 2013; Bashri and Prasad, 2015). However, the greater inhibition of WT growth compared with that of the T2 plants (T2-PM6 > T2-PM2) suggests that the induction of antioxidant activity was significantly lower in the WT plants than in the T2 plants. Furthermore, gene upregulation in the WT plants seems to have been insufficient to properly scavenge ROS. Under the same conditions, the higher gene expression in the T2 plants than that in the WT plants could be explained by the presence of anthocyanin, which is regulated by RsMYB1, or by RsMYB1 directly binding to the proteins involved in antioxidant production. High anthocyanin accumulation is linked to high antioxidant activity (Winkel-Shirley, 2002; Dixon et al., 2005; Agati et al., 2011; Dehghan et al., 2014; Naing et al., 2017). Therefore, these results support the hypothesis that the degree of stress tolerance in the plants (T2-PM6 > T2-PM2 > WT) depends on their antioxidant content.
The roles of other genes, such as GST and PCS, which are involved in antioxidant defense mechanisms and heavy metal detoxification, were also investigated. GST and PCS expression levels increased in response to heavy metal stress in the T2-PM6, T2-PM2, and WT plants. Furthermore, their expression levels were higher in the T2 plants (T2-PM6 > T2-PM2) than in the WT plants; this corresponds with the degree of tolerance to heavy metals in the former plants (i.e., T2-PM6 > T2-PM2 > WT). Thus, it is likely that RsMYB1 directly binds to the proteins involved in metal detoxification. The high expression levels of both genes suggest that they play major roles in scavenging ROS, detoxifying xenobiotics and heavy metals, and maintaining ionic homeostasis (Freeman et al., 2004; Foyer and Noctor, 2005; Hirata et al., 2005; Shao et al., 2008). Overexpression of these genes in other plants (e.g., Brassica juncea, Arabidopsis, Populus canescens, and Nicotiana tabacum) has also been found to enhance tolerance to heavy metal stress (Bittsánszkya et al., 2005; Cairns et al., 2006; Singla-Pareek et al., 2006; Gasic and Korban, 2007a,b). In this study, despite enhanced expression of the GST and PCS genes under heavy metal stress, there was a reduction in plant growth when the plants were exposed to heavy metals, which indicates that the GST and PCS expression levels were insufficient to defend completely against heavy metal stress, particularly in the WT plants. This strongly suggests that the heavy metal tolerance mechanism requires high GST and PCS enzymatic activity.
More heavy metals accumulated in the T2 plant shoots than in the WT plant shoots. This also suggests that the T2 plants were more tolerant to heavy metals than the WT plants, which led to the increased uptake of heavy metals. Another explanation for this observation is that the roots of the T2 plants were less damaged by the metals than the WT roots were because of increased anthocyanin levels (higher ROS-scavenging activity) and higher expression levels of antioxidant (SOD, CAT, and POX) and metal detoxification genes (GST and PCS). This would have led to greater alleviation of the negative effects of metal stress on root water uptake, because the RWC detected in the T2 plants was higher than that in the WT plants. These results support the findings of Zhu et al. (1999) and Wawrzyński et al. (2006), who reported that plants expressing PCS and GSH synthetic genes showed enhanced Cd accumulation and tolerance. Bennett et al. (2003) also claimed that overproduction of GSH in mustard led to accumulation of 2.4- to 3-fold more Cr, Cu, and Pb than that in WT plants.
Conclusion
RsMYB1 has been found to play a regulatory role in anthocyanin accumulation in petunias. According to phylogenetic analysis, it has sequence similarity with other MYBs that confer tolerance to various abiotic stresses. However, its regulatory role in the expression of genes related to antioxidant activity and metal detoxification during abiotic stress was not known. Therefore, in this study, we characterized the functional involvement of RsMYB1 in tolerance to heavy metal stress using RsMYB1-overexpressing plants (T2-PM6 and T2-PM2 plants) and WT plants. The results suggest that T2-PM6 and T2-PM2 plants are more capable of tolerating heavy metal stress because RsMYB1 induces higher expression levels of genes related to metal detoxification and antioxidant activity. Therefore, RsMYB1 could be exploited as a dual function gene that will improve anthocyanin production and heavy metal stress tolerance in horticultural and agricultural crops.
Author Contributions
AN and TA designed the study, conducted the experiments, and wrote the manuscript. SL assisted in the conduction of the experiments. CK and B-WY supervised the experiments at all stages and performed the critical revisions of the manuscript. All authors read and approved the final manuscript.
Funding
This work was supported by a grant from the Next-Generation BioGreen 21 Program (Project no. PJ01368505), Rural Development Administration, Republic of Korea.
Conflict of Interest Statement
The authors declare that the research was conducted in the absence of any commercial or financial relationships that could be construed as a potential conflict of interest.
Acknowledgments
This manuscript was released as a preprint hosted by bioRxiv https://www.biorxiv.org/content/early/2018/03/22/286849.
References
Agati, G., Biricolti, S., Guidi, L., Ferrini, F., Fini, A., and Tattini, M. (2011). The biosynthesis of flavonoids is enhanced similarly by UV radiation and root zone salinity in L. vulgare leaves. J. Plant Physiol. 168, 204–212. doi: 10.1016/j.jplph.2010.07.016
Ai, T. N., Naing, A. H., Arun, M., Jeon, S. M., and Kim, C. K. (2017). Expression of RsMYB1 in Petunia enhances anthocyanin production in vegetative and floral tissues. Sci. Hort. 214, 58–65. doi: 10.1016/j.scienta.2016.11.016
Ai, T. N., Naing, A. H., Yun, B. W., and Kim, C. K. (2018). Overexpression of RsMYB1 enhances heavy metal stress tolerance in transgenic petunia by elevating the transcript levels of stress. bioRxiv [Preprint]. doi: 10.1101/286849
Baek, S. A., Han, T., Ahn, S. K., Kang, H., Cho, M. R., Lee, S. C., et al. (2012). Effects of heavy metals on plant growths and pigment contents in Arabidopsis thaliana. Plant Pathol. J. 28, 446–452. doi: 10.5423/PPJ.NT.01.2012.0006
Bashri, G., and Prasad, S. M. (2015). Indole acetic acid modulates changes in growth, chlorophyll fluorescence and antioxidant potential of Trigonella foenum-graecum L. grown under cadmium stress. Acta Physiol. Plant. 37:49. doi: 10.1007/s11738-014-1745-z
Bennett, L. E., Burkhead, J. L., Hale, K. L., Terry, N., Pilon, M., and Pilon-Smits, E. A. H. (2003). Analysis of transgenic Indian mustard plants for phytoremediation of metal-contaminated mine tailings. J. Environ. Qual. 32, 432–440. doi: 10.2134/jeq2003.4320
Bittsánszkya, A., Kfmives, T., Gullner, G., Gyulai, G., Kiss, J., Heszky, L., et al. (2005). Ability of transgenic poplars with elevated glutathione content to tolerate zinc(2 +) stress. Environ. Interact. 31, 251–254. doi: 10.1016/j.envint.2004.10.001
Cairns, N. G., Pasternak, M., Wachter, A., Cobbett, C. S., and Meyer, A. J. (2006). Maturation of Arabidopsis seeds is dependent on glutathione biosynthesis within the embryo. Plant Physiol. 141, 446–455. doi: 10.1104/pp.106.077982
Cataldi, T. R. I., Margiotta, G., Del Fiore, A., and Bufo, S. A. (2003). Ionic content in plant extracts determined by ion chromatography with conductivity detection. Phytochem. Anal. 4, 176–183. doi: 10.1002/pca.700
Cheng, Y. J., Kim, M. D., Deng, X. P., Kwak, S. S., and Chen, W. (2013). Enhanced salt stress tolerance in transgenic potato plants expressing IbMYB1, a sweet potato transcription factor. J. Microbiol. Biotechnol. 23, 1737–1746. doi: 10.4014/jmb.1307.07024
DalCorso, G., Farinati, S., Maistri, S., and Furini, A. (2008). How plants cope with cadmium: staking all on metabolism and gene expression. J. Integr. Plant Biol. 50, 1268–1280. doi: 10.1111/j.1744-7909.2008.00737.x
Davidson, J. F., and Schiestl, R. H. (2001). Mitochondrial respiratory electron carriers are involved in oxidative stress during heat stress in Saccharomyces cerevisiae. Mol. Cell. Biol. 21, 8483–8489. doi: 10.1128/MCB.21.24.8483-8489.2001
Dehghan, S., Sadeghi, M., Pöppel, A., Fischer, R., Lakes-Harlan, R., Kavousi, H. R., et al. (2014). Differential inductions of phenylalanine ammonia-lyase and chalcone synthase during wounding, salicylic acid treatment, and salinity stress in safflower, Carthamus tinctorius. Biosci. Rep. 34, 273–282. doi: 10.1042/BSR20140026
Dixon, R. A., Xie, D. Y., and Sharma, S. B. (2005). Proanthocyanidins: a final frontier in flavonoid research? New Phytol. 165, 9–28.
Ebbs, S. D., and Kochian, L. V. (1997). Toxicity of zinc and copper to Brassica species: implications for phytoremediation. J. Environ. Qual. 26, 776–781. doi: 10.2134/jeq1997.00472425002600030026x
Fini, A., Brunetti, C., Di Ferdinando, M., Ferrini, F., and Tattini, M. (2011). Stress-induced flavonoid biosynthesis and the antioxidant machinery of plants. Plant Signal. Behav. 6, 709–711. doi: 10.4161/psb.6.5.15069
Fontes, R. L. S., and Cox, F. R. (1998). Zinc toxicity in soybean grown at high iron concentration in nutrient solution. J. Plant Nutr. 21, 1723–1730. doi: 10.1080/01904169809365517
Foyer, C. H., and Noctor, G. (2005). Redox homeostasis and antioxidant signaling: a metabolic interface between stress perception and physiological responses. Plant Cell 17, 1866–1875. doi: 10.1105/tpc.105.033589
Fozia, A., Muhammad, A. Z., Muhammad, A., and Zafar, M. K. (2008). Effect of chromium on growth attributes in sunflower (Helianthus annuus L.). J. Environ. Sci. 20, 1475–1480. doi: 10.1016/S1001-0742(08)62552-8
Freeman, J. L., Persans, M. W., Nieman, K., Albrecht, C., Peer, W., Pickering, I. J., et al. (2004). Increased glutathione biosynthesis plays a role in nickel tolerance in Thlaspi nickel hyperaccumulators. Plant Cell 16, 2176–2191. doi: 10.1105/tpc.104.023036
Gasic, K., and Korban, S. S. (2007a). Expression of Arabidopsis phytochelatin synthase in Indian mustard (Brassica juncea) plants enhances tolerance for Cd and Zn. Planta 225, 1277–1285.
Gasic, K., and Korban, S. S. (2007b). Transgenic Indian mustard (Brassica juncea) plants expressing an Arabidopsis phytochelatin synthase (AtPCS1) exhibit enhanced As and Cd tolerance. Plant Mol. Biol. 64, 361–369. doi: 10.1007/s11103-007-9158-7
Hirata, K., Tsuji, N., and Miyamoto, K. (2005). Biosynthetic regulation of phytochelatins, heavy metal-binding peptides. J. Biosci. Bioeng. 100, 593–599. doi: 10.1263/jbb.100.593
Hirschi, K. D., Korenkov, V. D., Wilganowski, N. L., and Wagner, G. J. (2000). Expression of Arabidopsis CAX2in tobacco. Altered metal accumulation and increased manganese tolerance. Plant Physiol. 124, 125–133. doi: 10.1104/pp.124.1.125
Hossain, M. A., Hasanuzzaman, M., and Fujita, M. (2010). Up-regulation of antioxidant and glyoxalase systems by exogenous glycinebetaine and proline in mung bean confer tolerance to cadmium stress. Physiol. Mol. Biol. Plants 16, 259–272. doi: 10.1007/s12298-010-0028-4
Hossain, M. A., Hossain, M. Z., and Fujita, M. (2009). Stress-induced changes of methylglyoxal level and glyoxalase I activity in pumpkin seedlings and cDNA cloning of glyoxalase I gene. Aust. J. Crop Sci. 3, 53–64.
Izaguirre-Mayoral, M. L., and Sinclair, T. R. (2005). Soybean genotypic difference in growth, nutrient accumulation and ultrastructure in response to manganese and iron supply in solution culture. Ann. Bot. 96, 149–158. doi: 10.1093/aob/mci160
Kambhampati, M. S., Begonia, G. B., Begonia, M. F. T., and Bufford, Y. (2005). Morphological and physiological responses of morning glory (Ipomoea lacunosa L.) grown in a lead- and chelate-amended soil. Int. J. Environ. Res. Public Health 2, 299–303. doi: 10.3390/ijerph2005020014
Keunen, E., Remans, T., Bohler, S., Vangronsveld, J., and Cuypers, A. (2011). Metal-induced oxidative stress and plant mitochondria. Int. J. Mol. Sci. 12, 6894–6918. doi: 10.3390/ijms12106894
Kim, G. R., Jung, E. S., Lee, S., Lim, S. H., Ha, S. H., and Lee, C. H. (2014). Combined mass spectrometry-based metabolite profiling of different pigmented rice (Oryza sativa L.) seeds and correlation with antioxidant activities. Molecules 19, 15673–15686. doi: 10.3390/molecules191015673
Lewis, S., Donkin, M. E., and Depledge, M. H. (2001). Hsp70 expression in Enteromorpha intestinalis (Chlorophyta) exposed to environmental stressors. Aquat. Toxicol. 51, 277–291. doi: 10.1016/S0166-445X(00)00119-3
Li, M., Hao, P., and Cao, F. (2017). Glutathione-induced alleviation of cadmium toxicity in Zea mays. Plant Phys. Biochem. 19, 240–249. doi: 10.1016/j.plaphy.2017.09.005
Lim, S. H., Song, J. H., Kim, D. H., Kim, J. K., Lee, J. Y., Kim, Y. M., et al. (2016). Activation of anthocyanin biosynthesis by expression of the radish R2R3-MYB transcription factor gene RsMYB1. Plant Cell Rep. 35, 641–653. doi: 10.1007/s00299-015-1909-3
Liu, D., An, Z., Mao, Z., Ma, L., and Lu, Z. (2015). Enhanced heavy metal tolerance and accumulation by transgenic sugar beets expressing Streptococcus thermophilus STGCS-GS in the presence of Cd, Zn and Cu alone or in combination. PLoS One 10:e0128824. doi: 10.1371/journal.pone.0128824
Meng, X., Yin, B., Feng, H. L., Zhang, S., Liang, X. Q., and Meng, Q. W. (2014). Over-expression of R2R3-MYB gene leads to accumulation of anthocyanin and enhanced resistance to chilling and oxidative stress. Biol. Plant. 58, 121–130. doi: 10.1007/s10535-013-0376-3
Millar, A. H., Mittova, V., Kiddle, G., Heazlewood, J. L., Bartoli, C. G., Theodoulou, F. L., et al. (2003). Control of ascorbate synthesis by respiration and its implications for stress responses. Plant Physiol. 133, 443–447. doi: 10.1104/pp.103.028399
Mittler, R., Vanderauwera, S., Gollery, M., and Van Breusegem, F. (2004). Reactive oxygen gene network of plants. Trends Plant Sci. 9, 490–498. doi: 10.1016/j.tplants.2004.08.009
Naing, A. H., Park, K. I., Ai, T. N., Chung, M. Y., Han, J. S., Kang, Y. W., et al. (2017). Overexpression of snapdragon Delila (Del) gene in tobacco enhances anthocyanin accumulation and abiotic stress tolerance. BMC Plant Biol. 17:65. doi: 10.1186/s12870-017-1015-5
Naing, A. H., Park, K. I., Chung, M. Y., Lim, K. B., and Kim, C. K. (2015). Optimization of factors affecting efficient shoot regeneration in chrysanthemum cv. Shinma. Braz. J. Bot. 39, 975–984. doi: 10.1007/s40415-015-0143-0
Nakabayashi, R., Yonekura-Sakakibara, K., Urano, K., Suzuki, M., Yamada, Y., Nishizawa, T., et al. (2014). Enhancement of oxidative and drought tolerance in Arabidopsis by overaccumulation of antioxidant flavonoids. Plant J. 77, 367–379. doi: 10.1111/tpj.12388
Prasad, A. S. (2012). Discovery of human zinc deficiency: 50 years later. J. Trace Elem. Med. Biol. 26, 66–69. doi: 10.1016/j.jtemb.2012.04.004
Qi, L., Yang, J., Yuan, Y., Huang, L., and Chen, P. (2015). Overexpression of two R2R3-MYB genes from Scutellaria baicalensis induces phenylpropanoid accumulation and enhances oxidative stress resistance in transgenic tobacco. Plant Physiol. Biochem. 94, 235–243. doi: 10.1016/j.plaphy.2015.06.007
Rascio, N., and Navari-Izzo, F. (2011). Heavy metal hyperaccumulating plants: how and why do they do it? And what makes them so interesting? Plant Sci. 180, 169–181. doi: 10.1016/j.plantsci.2010.08.016
Rezai, K., and Farboodnia, T. (2008). Manganese toxicity effects on chlorophyll content and antioxidant enzymes in pea plant (Pisum sativum L. c.v qazvin). Agric. J. 3, 454–458.
Ricachenevsky, F. K., Menguer, P. K., Sperotto, R. A., Williams, L. E., and Fett, J. P. (2013). Roles of plant metal tolerance proteins (MTP) in metal storage and potential use in biofortification strategies. Front. Plant Sci. 4:144. doi: 10.3389/fpls.2013.00144
Scoccianti, V., Crinelli, R., Tirillini, B., Mancinelli, V., and Speranza, A. (2006). Uptake and toxicity of Cr (Cr3 + ) in celery seedlings. Chemosphere 64, 1695–1703. doi: 10.1016/j.chemosphere.2006.01.005
Shanker, A. K., Cervantes, C., Loza-Tavera, H., and Avudainayagam, S. (2005). Chromium toxicity in plants. Environ. Int. 31, 739–753. doi: 10.1016/j.envint.2005.02.003
Shao, H. B., Chu, L. Y., Lu, Z. H., and Kang, C. M. (2008). Primary antioxidant free radical scavenging and redox signaling pathways in higher plant cells. Int. J. Biol. Sci. 4, 8–14. doi: 10.7150/ijbs.4.8
Sharma, D. C., Sharma, C. P., and Tripathi, R. D. (2003). Phytotoxic lesions of chromium in maize. Chemosphere 51, 63–68. doi: 10.1016/S0045-6535(01)00325-3
Singh, S., Parihar, P., Singh, R., Singh, V. P., and Prasad, S. M. (2015). Heavy metal tolerance in plants: role of transcriptomics, proteomics, metabolomics, and ionomics. Front. Plant Sci. 6:1143. doi: 10.3389/fpls.2015.01143
Singh, V. P., Srivastava, P. K., and Prasad, S. M. (2013). Nitric oxide alleviates arsenic-induced toxic effects in ridged Luffa seedlings. Plant Physiol. Biochem. 71, 155–163. doi: 10.1016/j.plaphy.2013.07.003
Singla-Pareek, S. L., Yadav, S. K., Pareek, A., Reddy, M. K., and Sopory, S. K. (2006). Transgenic tobacco overexpressing glyoxalase pathway enzymes grow and set viable seeds in zinc-spiked soils. Plant Physiol. 140, 613–623. doi: 10.1104/pp.105.073734
Tseng, M. J., Liu, C. W., and Yiu, J. C. (2007). Enhanced tolerance to sulfur dioxide and salt stress of transgenic Chinese cabbage plants expressing both superoxide dismutase and catalase in chloroplasts. Plant Physiol. Biochem. 45, 822–833. doi: 10.1016/j.plaphy.2007.07.011
Villiers, F., Ducruix, C., Hugouvieux, V., Jarno, N., Ezan, E., Garin, J., et al. (2011). Investigating the plant response to cadmium exposure by proteomic and metabolomic approaches. Proteomics 11, 1650–1663. doi: 10.1002/pmic.201000645
Warne, M. S., Heemsbergen, D., Stevens, D., McLaughlin, M., Cozens, G., Whatmuff, M., et al. (2008). Modeling the toxicity of copper and zinc salts to wheat in 14 soils. Environ. Toxicol. Chem. 27, 786–792. doi: 10.1897/07-294.1
Wawrzyński, A., Kopera, E., Wawrzyńska, A., Kamińska, J., Bal, W., and Sirko, A. (2006). Effects of simultaneous expression of heterologous genes involved in phytochelatin biosynthesis on thiol content and cadmium accumulation in tobacco plants. J. Exp. Bot. 57, 2173–2182. doi: 10.1093/jxb/erj176
Wellburn, A. R. (1994). The spectral determination of chlorophylls a and b, as well as total carotenoids, using various solvents with spectrophotometers of different resolution. J. Plant Physiol. 144, 307–313. doi: 10.1016/S0176-1617(11)81192-2
Williams, L. E., and Pittman, J. K. (2010). “Dissecting pathways involved in manganese homeostasis and stress in higher plants,” in Cell Biology of Metals and Nutrients, Plant Cell Monographs, Vol. 17, eds R. Helland and R. R. Mendal (Berlin: Springer-Verlag), 95–117.
Winkel-Shirley, B. (2002). Biosynthesis of flavonoids and effects of stress. Curr. Opin. Plant Biol. 5, 218–223. doi: 10.1016/S1369-5266(02)00256-X
Xu, Z., Ge, Y., Zhang, W., Zhao, Y., and Yang, G. (2018). The walnut JrVHAG1 gene is involved in cadmium stress response through ABA-signal pathway and MYB transcription regulation. BMC Plant Biol. 18:19. doi: 10.1186/s12870-018-1231-7
Yang, X., Feng, Y., He, Z., and Stoffell, P. J. (2005). Molecular mechanisms of heavy metal hyper accumulation and phytoremediation. J. Trace Elem. Med. Biol. 18, 339–353. doi: 10.1016/j.jtemb.2005.02.007
Yilmaz, K., Akinci, I. E., and Akinci, S. (2009). Effect of lead accumulation on growth and mineral composition of eggplant seedlings (Solanum melongena). New Zeal. J. Crop Hort. 37, 189–199. doi: 10.1080/01140670909510264
Yuan, Y., Qi, L., Yang, J., Wu, C., Liu, Y., and Huang, L. (2015). A Scutellaria baicalensis R2R3-MYB gene, SbMYB8, regulates flavonoid biosynthesis and improves drought stress tolerance in transgenic tobacco. Plant Cell Tissue Organ Cult. 120, 961–972. doi: 10.1007/s11240-014-0650-x
Zhu, Y. L., Pilon-Smits, E. A. H., Jouanin, L., and Terry, N. (1999). Overexpression of glutathione synthetase in Indian mustard enhances cadmium accumulation and tolerance. Plant Physiol. 119, 73–79. doi: 10.1104/pp.119.1.73
Keywords: abiotic stress, gene expression, genetic transformation, MYB transcription factor, phylogenetic analysis, plant growth
Citation: Ai TN, Naing AH, Yun B-W, Lim SH and Kim CK (2018) Overexpression of RsMYB1 Enhances Anthocyanin Accumulation and Heavy Metal Stress Tolerance in Transgenic Petunia. Front. Plant Sci. 9:1388. doi: 10.3389/fpls.2018.01388
Received: 08 June 2018; Accepted: 31 August 2018;
Published: 20 September 2018.
Edited by:
Wim Van den Ende, KU Leuven, BelgiumReviewed by:
Iwona Małgorzata Morkunas, Poznań University of Life Sciences, PolandRavi Valluru, Cornell University, United States
Copyright © 2018 Ai, Naing, Yun, Lim and Kim. This is an open-access article distributed under the terms of the Creative Commons Attribution License (CC BY). The use, distribution or reproduction in other forums is permitted, provided the original author(s) and the copyright owner(s) are credited and that the original publication in this journal is cited, in accordance with accepted academic practice. No use, distribution or reproduction is permitted which does not comply with these terms.
*Correspondence: Byung-Wook Yun, bwyun@knu.ac.kr Chang Kil Kim, ckkim@knu.ac.kr
†These authors have contributed equally as first authors