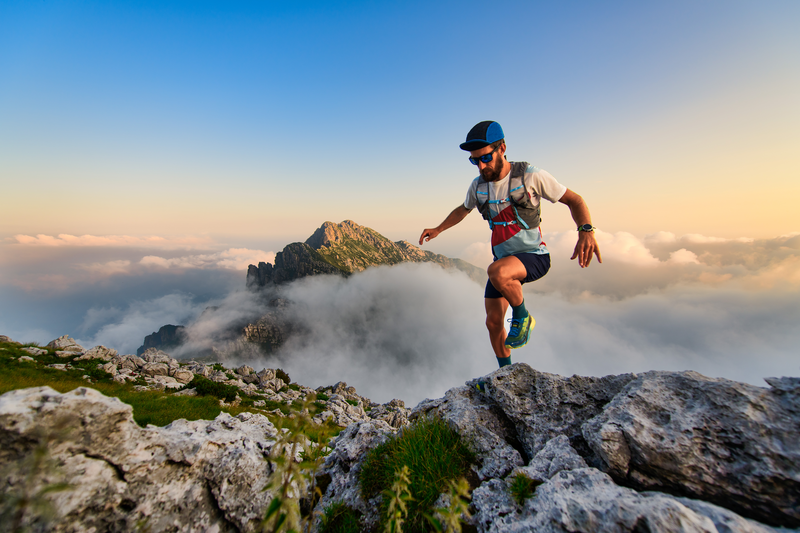
94% of researchers rate our articles as excellent or good
Learn more about the work of our research integrity team to safeguard the quality of each article we publish.
Find out more
ORIGINAL RESEARCH article
Front. Plant Sci. , 19 September 2018
Sec. Plant Physiology
Volume 9 - 2018 | https://doi.org/10.3389/fpls.2018.01363
Stomata play a critical role in the regulation of gas exchange between the interior of the leaf and the exterior environment and are affected by environmental and endogenous stimuli. This study aimed to evaluate the effect of the arbuscular mycorrhizal (AM) fungus, Rhizophagus irregularis, on the stomatal behavior of wheat (Triticum aestivum L.) plants under combination with elevated CO2 and NaCl stress. Wheat seedlings were exposed to ambient (400 ppm) or elevated (700 ppm) CO2 concentrations and 0, 1, and 2 g kg−1 dry soil NaCl treatments for 10 weeks. AM symbiosis increased the leaf area and stomatal density (SD) of the abaxial surface. Stomatal size and the aperture of adaxial and abaxial leaf surfaces were higher in the AM than non-AM plants under elevated CO2 and salinity stress. AM plants showed higher stomatal conductance (gs) and maximum rate of gs to water vapor (gsmax) compared with non-AM plants. Moreover, leaf water potential (Ψ) was increased and carbon isotope discrimination (Δ13C) was decreased by AM colonization, and both were significantly associated with stomatal conductance. The results suggest that AM symbiosis alters stomatal morphology by changing SD and the size of the guard cells and stomatal pores, thereby improving the stomatal conductance and water relations of wheat leaves under combined elevated CO2 and salinity stress.
Arbuscular mycorrhizal (AM) fungi belong to the monophyletic phylum Glomeromycota and form symbiotic associations with the roots of over 80% of land plant species (Smith and Read, 2008). AM fungi (AMF) are obligate biotrophs that have many benefits for the host plants, such as increased nutrient uptake, plant productivity, and abiotic and biotic stress resistance (Zhu et al., 2016a). In turn, the host plants provide the fungi with photosynthetic carbon (Smith and Read, 2008). AM symbiosis has been demonstrated to affect plant performance as it leads to a series of morphological, physiological, biochemical, and molecular changes.
Stomata are formed by two small symmetric guard cells on the epidermis of higher plants that play a central role in the regulation of gas exchange between the inner air space of the leaf and the outer atmosphere (Lawson, 2009). Stomata enable CO2 entry into the leaf for photosynthesis while limiting water loss, thus influencing global water and carbon cycles (Blatt et al., 2017). Stomatal conductance (gs) is regulated primary by the aperture of the stomatal pore (SA) and stomatal density (SD), as well as the water transport capacity of the guard cells on the leaf surface (Yan et al., 2012; Huang and Xu, 2015; Gamage et al., 2018). AM symbiosis often alters the stomatal behavior of the host (Augé et al., 2016; Chitarra et al., 2016). gs differs between AM and non-AM plants; however, the effect of AM on gs is unpredictable, and its mechanism remains unclear (Smith and Read, 2008; Augé et al., 2015). Changes in gs are always accompanied by changes in leaf water potential (Ψ) or osmotic adjustment. The influence of AM on gs may also be associated with altered chemical signals and carbon dynamics of the plant leaves (Ruiz-Lozano and Aroca, 2010; Augé et al., 2015).
Stomatal behavior is modulated by environmental factors, such as elevated atmospheric CO2 concentration and salinity stress. To date, the concentration of atmospheric CO2 has risen to over 400 μmol l−1 (NOAA-ESRL, 2018), and about 7% of the total global land area is occupied by saline soils (Sheng et al., 2008). In the future, global climate change will result in the exacerbation of increasing atmospheric CO2 concentration and soil salinization. Therefore, elevated CO2 concentration and salinity are important factors that influence plant growth and crop productivity. Major physiological processes are regulated by increased CO2 and saline stress, both of which can directly affect gas exchange and water relations (Eller et al., 2014; Yu et al., 2015). Accumulated evidence indicates that gs is decreased under elevated CO2 and salt stress, and elevated CO2 improves water use efficiency and Ψ under saline conditions (Pérez-López et al., 2012b; Zaghdoud et al., 2013). However, the underlying mechanisms for the regulation of stomatal formation and development under elevated CO2 and salinity remain largely elusive. Studies have documented that the response of SA and SD to environmental conditions are contradictory, implying complexity in the regulation of stomatal morphology and behavior by environmental cues (Yan et al., 2012; Sun et al., 2014).
Importantly, little is known about the effect of AM on plant growth and physiology under combined elevated CO2 concentrations and soil salt stress. Some studies have reported that AMF influences the stomatal behavior of leaves exposed to salinity stress or elevated CO2 regime. Under salinity stress, AM plants showed higher gs and lower intercellular CO2 concentration and water potential, hereby maintaining a favorable gas exchange capacity and water use efficiency (Sheng et al., 2008; Evelin et al., 2009; Kapoor et al., 2013; Frosi et al., 2018). At elevated CO2, the response of gs to AM symbiosis varies. Syvertsen and Graham (1999) found that the difference in gs was not significant in AM and non-AM citrus plants grown at elevated CO2 concentration. However, Baslam et al. (2012) and Goicoechea et al. (2014) reported that 5-weeks-old AM seedlings of alfalfa had higher gs when grown at elevated CO2 for 2 weeks and lower gs when grown at elevated CO2 for 4 weeks than non-AM plants. Under combined elevated CO2 and saline stress, gs and stomatal morphology in AM and non-AM wheat plants are altered, as reported by Zhu et al. (2016b).
Wheat (Triticum aestivum L.) is one of the world's most important cereal crops and grown on about 220 million hectares and yielding more over 715 million tons of grain in 2013 (FAOSTAT, 2015). Our previous study found that AM symbiosis alleviated salinity stress in wheat plants under elevated CO2 concentration (Zhu et al., 2016b). In this study, we examined whether AM symbiosis altered the stomatal behavior of wheat plants under elevated CO2 concentration and salt stress. To test this, stomatal conductance and stomatal morphology in wheat leaves under different CO2 regimes and NaCl levels were determined. In addition, plant growth, leaf water potential, and carbon isotope discrimination (Δ13C) were measured to investigate the interaction between water relations and stomatal behavior under combined elevated CO2 and salinity stress.
The AMF (Rhizophagus irregularis (Blaszk., Wubet, Renker & Buscot) Sieverd., G.A. Silva & Oehl.) used in this experiment was obtained from INOQ GmbH, Schnega, Germany. The AM inoculum was a mixture of vermiculite with spores, hyphae and root residues (mycorrhiza units, 210 spores per mL substrate).
The soil was sandy loam and collected from the experimental farm of the Department of Plant and Environmental Sciences, Faculty of Science, University of Copenhagen, Taastrup, Denmark. The characteristics of the soil included a pH of 6.8, total C 11.8 g kg−1, total N 1.15 g kg−1, and Olsen-P 19.2 mg kg−1. The soil was sieved by passing through a 2 mm mesh, and then sterilized in the oven at 95°C for 4 h for three consecutive days.
The experiment was conducted with two AM inoculation levels (inoculated with R. irregularis and not inoculated as the control), two atmospheric CO2 concentrations (ambient, 400 ppm and elevated, 700 ppm CO2), and three NaCl levels (0, 1 and 2 g kg−1 dry soil) treatments. The experimental pots were 4 L (15.2 cm diameter and 25 cm deep), and the bottom of the pots was covered with mesh (1.5 mm). The pots were filled with 6.0 kg of soil. Twenty mL of AM inoculum was mixed into the soil for the inoculation treatment, and 20 mL sterilized inoculum plus 10 mL AMF-free filtrate from the inoculum suspension was added for the non-inoculation treatment. Half of the pots were then placed into a glasshouse cell with ambient CO2 concentration, and the other half were placed into another glasshouse cell with elevated CO2 concentration. The CO2 concentration in the glasshouse cells was monitored every 6 s by a CO2 Transmitter Series GMT220 (Vaisala Group, Helsinki, Finland). Eight seeds of wheat (var. Tuareg) were sown in each pot, and tap water was used for irrigation. The climate conditions in the glasshouse were set at: 25/16 ± 2°C day/night air temperature, 60% relative humidity, 16 h photoperiod and >500 μmol m−2 s−1 photosynthetic active radiation (PAR) supplied by sunlight plus meta-halide lamps. Three weeks later, the eight seedlings were thinned to four per pot, and 1.0 g N and 0.8 g K per pot were applied to meet the nutrient requirements for plant growth. After 4 weeks, the seedlings were subjected to salinity treatments with NaCl solution. The experiment was a complete randomized block design with four replicates for each treatment.
Ten weeks after sowing, the gs of the leaf adaxial and abaxial surfaces was measured by a leaf porometer (Decagon Devices, Inc., Pullman, WA, USA) at 11:00 a.m. Then stomatal morphology was measured according to Zhu et al. (2016b). Briefly, fingernail polish imprints were obtained from the adaxial and abaxial surfaces of the leaf, using clean cellophane tape to transfer the impression to a microscope slide. The imprints were observed by a LEITZ DMRD microscope camera system (Leica Microscope and System GmbH, D 35530, Wetzlar, Germany). Stomatal density (SD), guard cell length (Lg), guard cell width (Wg), stomatal pore length (Lp), and stomatal pore width (Wp) of the leaf adaxial and abaxial surfaces were measured and stomatal size (SS), stomatal aperture (SA) and anatomical maximum stomatal conductance to water vapor (gsmax) were then calculated using the following equation:
where d is the diffusivity of water in air (24.6 × 10−6 m2 s−1 at 25°C), amax is the mean maximum stomatal pore area, ν is the molar volume of air (24.4 × 10−3 m3 mol−1 at 25 °C and 101.3 kPa) and π is the mathematical constant (approximated to 3.142) (Franks and Farquhar, 2001).
Leaf area was measured by a leaf area meter (Li-3100, Li-Cor Inc., Lincoln, NB, USA). Leaf Ψ was measured by a pressure chamber (Soil Moisture Equipment Corp., Santa Barbara, CA, USA) on the same leaves (Zhu et al., 2016b).
Leaf samples were oven-dried at 75°C for 3 d and were ground to a fine powder. Plant C and 13C concentrations were analyzed using the Dumas dry combustion method in a system consisting of an ANCA-SL Elemental Analyzer coupled to a 20–20 mass spectrometer (Europa Scientific Ltd, Creve, UK). 13C composition (δ13C) was calculated as:
where Rsample is the 13C:12C ratio of the sample and Rstandard is the 13C:12C of the Pee Dee Belemnite (PDB) standard. Δ13C was calculated as:
where the subscripts a and p refer to air and plant, respectively. The δa value was taken as −7.7‰ (Yan et al., 2012).
The data were statistically analyzed using Microsoft Excel (Microsoft Corporation, Albuquerque, NM, USA) and SPSS 16.0 (SPSS Inc., Chicago, IL, USA) software. The effects of AMF, CO2, and NaCl treatments as well as their interactions on variables were analyzed using analysis of variance (ANOVA). Differences between treatments were considered significant when the P-value was less than 0.05 by Tukey's test. Pearson's correlation coefficients between variables were computed in SPSS 16.0.
AM, CO2, and NaCl treatments significantly affected the leaf area of the wheat plants (Figure 1, Table 1). Under ambient CO2, the leaf area of the AM plants was increased by 27.2, 29.4, and 29.7% at 0, 1, and 2 g kg−1 dry soil NaCl levels, and by 10.5, 26.2, and 32.1% under elevated CO2 condition, respectively, compared to non-AM plants. Elevated CO2 increased but salinity stress decreased leaf area. A significant interactive effect between CO2 and NaCl treatments was observed.
Figure 1. Leaf area of wheat plants inoculated (+M) or not (–M) with Rhizophagus irregularis at ambient (aC) and elevated (eC) CO2 and 0, 1, and 2 g kg−1 dry soil NaCl levels. Vertical bars represent the means ± standard errors.
Table 1. Probabilities of significance for main effects and factor interactions for the variables measured and analyzed using three-way ANOVA.
AM, CO2, and NaCl treatments significantly affected guard cell length (Lg) and width (Wg), and stomatal pore length (Lp), and width (Wp) of the wheat leaf adaxial and abaxial surfaces, while adaxial Wg was not affected by AM and CO2 treatments (Tables 2, 3). In general, elevated CO2 and salt stress decreased Lg, Wg, Lp, and Wp, and AM fungus increased the Lg, Lp, and Wp of the wheat leaves. There were significant interactive effect of AM × NaCl on abaxial Lg, CO2 × NaCl on Wp, and AM × CO2 × NaCl on abaxial Lg, Wg, Lp, and Wp.
Table 2. Stomatal morphology in the adaxial leaves of wheat plants inoculated (+M) or not inoculated (–M) with Rhizophagus irregularis (AM) at CO2 and NaCl treatments.
Table 3. Stomatal morphology in the abaxial leaves of wheat plants inoculated (+M) or not inoculated (–M) with Rhizophagus irregularis (AM) at CO2 and NaCl treatments.
SD, SS, and SA of the leaf adaxial and abaxial surfaces were significantly affected by AM, CO2, and NaCl treatments, whereas adaxial SD and SS were not affected by AM and abaxial SS was not affected by CO2 (Figure 2, Table 1). Salt stress decreased SD, SS, and SA. AM colonization increased abaxial SD, and adaxial and abaxial SS and SA under elevated CO2 and salt stress. Additionally, a significant interactive effect of CO2 × NaCl on SA was observed.
Figure 2. Stomatal density (SD), stomatal size (SS) and stomatal aperture (SA) on the adaxial and abaxial leaf surfaces of wheat plants inoculated (+M) or not (–M) with Rhizophagus irregularis at ambient (aC) and elevated (eC) CO2 and 0, 1, and 2 g kg−1 dry soil NaCl levels. Vertical bars represent the means ± standard errors.
Elevated CO2 and salinity significant decreased the gs of the adaxial and abaxial leaf surfaces (Figure 3, Table 1). However, AM plants had greater gs than non-AM plants. For adaxial gs, at 400 ppm CO2 level, AM colonization increased by 7.5, 10.2, and 17.4% at 0, 1, and 2 g kg−1 dry soil NaCl levels, and by 10.6, 18.2, and 43.8% at 700 ppm CO2 level. For abaxial gs, at 400 ppm CO2 level, AM colonization increased by 5.5, 17.1, and 22.4% at 0, 1, and 2 g kg−1 dry soil NaCl levels, and by 15.0, 24.4, and 18.9% at 700 ppm CO2 level.
Figure 3. Stomatal conductance (gs) maximum rate of gs to water vapor (gsmax) on the adaxial and abaxial leaf surfaces of wheat plants inoculated (+M) or not (–M) with Rhizophagus irregularis at ambient (aC) and elevated (eC) CO2 and 0, 1, and 2 g kg−1 dry soil NaCl levels. Vertical bars represent the means ± standard errors.
Leaf adaxial and abaxial gsmax were significantly affected by AM, CO2, and NaCl treatments, while abaxial gsmax was not affected by CO2 (Figure 3, Table 1). There was a significant interactive effect of AM × CO2 × NaCl on abaxial gsmax. Severe (2 g kg−1 dry soil NaCl) salt stress decreased adaxial and abaxial gsmax. AM colonization enhanced the gsmax of the adaxial and abaxial surfaces.
Leaf Δ13C was affected by AM, CO2, and NaCl treatments (Figure 4, Table 1). Wheat plants had higher Δ13C under elevated CO2 compared with ambient CO2. Under elevated CO2 level, salt stress increased Δ13C, and AMF decreased Δ13C. Moreover, there was a significant interactive effect of CO2 × NaCl on Δ13C.
Figure 4. Carbon isotope discrimination (Δ13C) and water potential (Ψ) of wheat leaves inoculated (+M) or not (–M) with Rhizophagus irregularis at ambient (aC) and elevated (eC) CO2 and 0, 1, and 2 g kg−1 dry soil NaCl levels. Vertical bars represent the means ± standard errors.
Leaf Ψ was affected by AM, CO2 and salt treatments (Figure 4, Table 1). Elevated CO2 and NaCl stress significantly reduced the Ψ, and AM colonization enhanced the Ψ of the wheat plants. Under ambient CO2, the leaf Ψ of the AM plants was enhanced by 16.9, 15.3, and 7.8% at 0, 1, and 2 g kg−1 dry soil NaCl levels, and by 29.0, 13.3, and 10.7% under elevated CO2 condition, respectively, compared to non-AM plants.
It was found that the gs and gsmax of the adaxial and abaxial leaf surfaces were positively correlated with SD, SA, SS, and Ψ (Table 4). Nonetheless, a negative linear relationship between Δ13C and gs, gsmax, SA, SS, and Ψwas found across all the treatments.
Stomata play a key role in regulating gas exchange on the leaf surface (Lawson, 2009). Plants are constantly faced with environmental stress conditions and have evolved a series of mechanisms to regulate stomatal behavior in response to this stress (Casson and Hetherington, 2010). AM symbiosis has been shown to alter the stomatal behavior of the host plants (Augé et al., 2015). However, the mechanisms underlying the effects of AMF on stomatal traits remain largely elusive, particularly for host plants grown under multiple environmental regimes. Therefore, this study aimed to investigate the effect of AM on gs and the stomatal morphology of wheat plants under combined elevated CO2 and NaCl stress.
In the present study, the gs of the adaxial and abaxial leaf surfaces in the AM wheat plants was higher than in non-AM plants. AM plants often show higher gs than non-AM plants under unstressed conditions, elevated CO2 concentration and salt stress (Aroca et al., 2013; Goicoechea et al., 2014; Augé et al., 2016; Frosi et al., 2018). A meta-analysis by Augé et al. (2015) indicated that the gs in AM plants was increased by an average of 24% compared with non-AM plants for sufficiently watered and drought-stressed plants. Moreover, both NaCl stress and elevated CO2 decreased gs, which is in agreement with the results by Pérez-López et al. (2012b), Zaghdoud et al. (2013) and Piñero et al. (2014). CO2 elevation increased CO2 flux into the leaves because the gradient of CO2 between the outer and the inner leaf increases (Pérez-López et al., 2012b). Salt stress affected the diffusion of CO2 into the leaves due to closed stomata and decreased stomatal and mesophyll conductance (Piñero et al., 2014). Under elevated CO2 and NaCl stress, AM plants had higher gs than non-AM plants, which may be due to decreased photorespiration in AM plants, increased sink strength of AM fungal roots, and a higher capability for CO2 assimilation (Aroca et al., 2013; Goicoechea et al., 2014; Zhu et al., 2016b). We also observed a lower reduction in gs in AM plants compared with non-AM plants with increasing levels of salinity and elevated CO2, which suggested that CO2 diffusion through the stomata was diminished less, and thus the water status may be better in AM plants (Chen et al., 2017).
Anatomical gsmax represents the maximum rate of gs to water vapor as calculated by the density, size and geometry of the stomata when fully open (Dow et al., 2014; McElwain et al., 2016). gsmax has been a useful tool for assessing stomatal development with respect to external environmental factors, such as air CO2 concentration and salinity (Dow et al., 2014). Our results showed that elevated CO2 decreased adaxial gsmax, and AMF increased gsmax of both the adaxial and abaxial leaf surfaces. Lammertsma et al. (2011) reported that increased global CO2 has led to reduced gsmax in Florida vegetation through SD and amax adaptation within their phenotypic plasticity, which likely represents the adaptation of plants to increased intrinsic water use efficiency via optimizing carbon gain to water loss. gsmax, like gs, was greater in AM plants than non-AM plants, which indicated that AM colonization enhanced gas exchange capacity and the potential productivity of the plants. Multiple combinations of increased stomatal density and pore geometries resulted in increased gsmax in AM plants (McElwain et al., 2016). AM was also found to enhance the functioning of the guard cells at maximum turgor pressure or aperture size, thereby increasing gas exchange (Dow and Bergmann, 2014).
In our study, gs was significantly correlated with SD and SA, which is consistent with the results reported by Kumar et al. (2014) in Jatropha curcas grown under elevated CO2 condition. Such relationships indicated that both SD and SA control gs in wheat plants. Franks and Farquhar (2007) suggested several strategies for increasing gs that were aimed ultimately at increasing the sum of stomatal pore area/depth per unit leaf area, and the simplest and possibly the most accessible option was to increase SD. It is generally accepted that SD is changed by atmospheric CO2 concentration, salinity and other environmental factors (Chitarra et al., 2016; Gamage et al., 2018). However, the response of SD to environmental changes is varied and complex. Differences appear to exist among plant species and/or genotypes, experimental facilities, and experimental conditions (Yan et al., 2017). In the present study, NaCl stress decreased SD, and elevated CO2 and AM colonization increased SD, which indicated that the response of SD to environmental factors differs. Chitarra et al. (2016) found that SD in AM tomato plants was almost twice that of non-AM plants. Some genes that regulate stomatal development were altered significantly only in the presence of AM symbiosis, and LeEPFL9 transcript levels were associated with increased SD in AM plants (Chitarra et al., 2016). Moreover, some studies have shown that an increase in SD is not accompanied by a decrease in the size of the stomata under altered environmental conditions (Yan et al., 2012). In contrast, experimental evidence has demonstrated that SD is negatively associated with SS (Lawson and Blatt, 2014). A greater SD associated with smaller stomata was observed, which suggests that dense, small stomatarespond to environmental changes more rapidly, and thus plants reach a high gs rapidly under favorable conditions and reduce gs promptly under unfavorable conditions (Hetherington and Woodward, 2003; Drake et al., 2013). AM wheat plants had greater numbers of stomatal compared with non-AM plants, which resulted in higher gs and better growth under unfavorable conditions.
When plants are exposed to changing environmental conditions for a short period, SA may be the main factor influencing gs, whereas changes in gs may be determined by the alternation of both SA and SD because SA is more dynamic while SD is more static in response to a changed environment for a long period (Franks and Farquhar, 2007; Yan et al., 2012). SA is regulated by a series of internal signals and environmental cues that serve to “set” stomatal apertures to adapt to the prevailing environmental regimes (Murray et al., 2016). In our plants, as observed in other experiments (Zaghdoud et al., 2013; Zhu et al., 2016b), elevated CO2 and NaCl stress caused stomatal closure by affecting the size of the guard cells and stomata. However, AM plants have greater SS and SA than non-AM plants, which indicated that AM symbiosis is able to maintain higher stomatal opening. Therefore, it may be concluded that the increment in gs of AM wheat plants observed in this study could have been attributed to both increased stomatal number and openings in response to growth at elevated CO2 and salt stress. Moreover, SA is primarily determined by the turgor pressure of the guard cells, which is mediated via ion concentration including K+, Cl−, and Ca2+ (Gamage et al., 2018). AM inoculation may have decreased the activity of outward rectifying K+ channels relative to that of inward rectifying K+ channels, and/or decreased the Cl− release from the guard cells and decreased the Ca2+ concentration within them, resulting in stomatal opening (Araújo et al., 2011; Gamage et al., 2018).
The present results showed that NaCl stress and elevated CO2 decreased the Ψ of the wheat leaves, which suggests that plants have to reduce their water potential to maintain a continued uptake of water (Pérez-López et al., 2012a). Concurrently, a positive correlation between gs and Ψ also proved that plants ensure a lower gs to diminish water losses of the leaf surface. However, our results showed that AM plants had a similar Ψ to non-AM plants under non-stressed conditions, as well as a higher Ψ than non-AM plants under elevated CO2 and NaCl stress. The results indicated that under elevated CO2 and salinity stress, AM plants are able to maintain better water status and consequently more open stomata and higher gs compared with their non-AM counterparts. Chitarra et al. (2016) suggested that stomatal closing upon water stress in AM plants is probably not regulated by active ABA-mediated mechanisms but could rather be driven by passive hydraulic-mediated mechanisms. The higher gs of the AM plants has been associated with a higher Ψ (Augé et al., 2015; Zhu et al., 2016b), and thus the AM symbiosis promotion of leaf hydration would naturally be related to altered stomatal behavior under elevated CO2 and salt stress.
Moreover, we noticed a negative linear relationship between gs and Δ13C. Δ13C is often used as an indirect evaluation of water use efficiency (Yan et al., 2012). In this study, we confirmed that elevated CO2 increased the Δ13C of the wheat plants, which was in agreement with the results of Pérez-López et al. (2014). Under elevated CO2 concentration, Δ13C in the AM plants was lower than the non-AM plants. The Δ13C value represents a long-term mean of the intercellular to the atmospheric CO2 concentration ratio (Farquhar et al., 1989). Significant differences in Δ13C caused by AM, elevated CO2 and salinity suggested that these environmental cues have altered the ratio of the intercellular to the atmospheric CO2 concentration. Sekiya and Yano (2008) demonstrated that both the increased photosynthetic rate and the decreased SA similarly lower the ratio of the intercellular CO2 concentration to the atmospheric CO2 concentration, resulting in a reduction in Δ13C. Our results showed that Δ13C was negatively correlated with SA, which indicated that increased SA was associated with the optimization of leaf gas exchange, such that an increment of water use efficiency (Sun et al., 2014). Similarly, some studies reported that SD was negatively associated with leaf Δ13C, indicating that greater SD is associated with higher water use efficiency (Sekiya and Yano, 2008; Yan et al., 2012). Therefore, it is evident that the increment in SA and gs of the AM wheat plants observed in this study could decrease Δ13C and thus improve water use efficiency in response to growth at elevated CO2 and salinity stress.
It is well documented that the effect of AM on stomatal behavior is associated with AM-induced changes in plant size (Augé et al., 2015). In this study, leaf area decreased under NaCl stress and increased under elevated CO2 concentration. This is consistent with the results that elevated CO2 mitigates the detrimental effect of salt stress on plant growth (Geissler et al., 2009; Yu et al., 2015). Under elevated CO2 and NaCl stress, the leaf area of AM plants was higher than the non-AM counterparts. A higher leaf area, and thus larger plant size, might affect plant water relations and stomatal behavior. However, according to the meta-analysis by Augé et al. (2015), the effect of AM on stomatal behavior can be independent of the AM promotion of shoot size, although the AM-induced increase in gs without growth promotion was smaller than the overall AM promotion.
In short, our study showed that AM symbiosis altered the stomatal behavior of wheat plants exposed to elevated CO2 concentration and NaCl stress. Compared to non-AM plants, AM wheat plants had higher gs, SD and SA of the adaxial and abaxial leaf surfaces under combined elevated CO2 and NaCl stress. Better stomatal conductance and stomatal development allowed the AM plants to optimize stomatal movement and gas exchange, leading to an improvement in water status, as exemplified by the increased Ψ. Our results suggest that the response of stomatal characteristics to environmental changes differs, which poses a significant challenge to the prediction of the effects of climate change on water flux through the stomata (Yan et al., 2017). Our findings also elucidate the effect of AM symbiosis on the stomatal behavior of plants and will be useful in understanding the underlying mechanism of the regulation of stomatal behavior and development by AM symbiosis in response to future climate change scenarios.
XZ conceived and designed the study; XZ, QC, and LS performed the experiments; XZ, QC, and XY analyzed the data; and XZ wrote the article with contributions by QC, WY, and HZ.
This study was supported by the Youth Innovation Promotion Association of the Chinese Academy of Sciences (2018264) and the One-Three-Five Strategic Planning Program of the Chinese Academy of Sciences (IGA−135–04).
The authors declare that the research was conducted in the absence of any commercial or financial relationships that could be construed as a potential conflict of interest.
Araújo, W. L., Fernie, A. R., and Nunes-Nesi, A. (2011). Control of stomatal aperture: a renaissance of the old guard. Plant Signal. Behav. 6, 1305–1311. doi: 10.4161/psb.6.9.16425
Aroca, R., Ruiz-Lozano, J. M., Zamarre-o, A. M., Paz, J. A., García-Mina, J. M., Pozo, M. J., et al. (2013). Arbuscular mycorrhizal symbiosis influences strigolactone production under salinity and alleviates salt stress in lettuce plants. J. Plant Physiol. 170, 47–55. doi: 10.1016/j.jplph.2012.08.020
Augé, R. M., Toler, H. D., and Saxton, A. M. (2015). Arbuscular mycorrhizal symbiosis alters stomatal conductance of host plants more under drought than under amply watered conditions: a meta-analysis. Mycorrhiza 25, 13–24. doi: 10.1007/s00572-014-0585-4
Augé, R. M., Toler, H. D., and Saxton, A. M. (2016). Mycorrhizal stimulation of leaf gas exchange in regulation to root colonization, shoot size, leaf phosphorus and nitrogen: a quantitative analysis of the literature using meta-regression. Front. Plant Sci. 7:1084. doi: 10.3389/fpls.2016.01084
Baslam, M., Erice, G., and Goicoechea, N. (2012). Impact of arbuscular mycorrhizal fungi (AMF) and atmospheric CO2 concentration on the biomass production and partitioning in the forage legume alfalfa. Symbiosis 58, 171–181. doi: 10.1007/s13199-012-0199-6
Blatt, M. R., Brodribb, T. J., and Torii, K. U. (2017). Small pores with a big impact. Plant Physiol. 174, 467–469. doi: 10.1104/pp.17.00642
Casson, S., and Hetherington, A. M. (2010). Environmental regulation of stomatal development. Curr. Opin. Plant Biol. 13, 90–95. doi: 10.1016/j.pbi.2009.08.005
Chen, J., Zhang, H., Zhang, X., and Tang, M. (2017). Arbuscular mycorrhizal symbiosis alleviates salt stress in black locust through improved photosynthesis, water status, and K+/Na+ homeostasis. Front. Plant Sci. 8:1739. doi: 10.3389/fpls.2017.01739
Chitarra, W., Pagliarani, C., Maserti, B., Lumini, E., Siciliano, I., Cascone, P., et al. (2016). Insights on the impact of arbuscular mycorrhizal symbiosis on tomato tolerance to water stress. Plant Physiol. 171, 1009–1023. doi: 10.1104/pp.16.00307
Dow, G. J., and Bergmann, D. C. (2014). Patterning and processes: how stomatal development defines physiological potential. Curr. Opin. Plant Biol. 21, 67–74. doi: 10.1016/j.pbi.2014.06.007
Dow, G. J., Bergmann, D. C., and Berry, J. A. (2014). An integrated model of stomatal development and leaf physiology. New Phytol. 201, 1218–1226. doi: 10.1111/nph.12608
Drake, P. L., Froend, R. H., and Franks, P. J. (2013). Smaller, fast stomata: scaling of stomatal size, rate of response, and stomatal conductance. J. Exp. Bot. 64, 495–505. doi: 10.1093/jxb/ers347
Eller, F., Lambertini, C., Nguyen, L. X., and Brix, H. (2014). Increased invasive potential of non-native Phragmites australis: elevated CO2 and temperature alleviate salinity effects on photosynthesis and growth. Global Change Biol. 20, 531–543. doi: 10.1111/gcb.12346
Evelin, H., Kapoor, R., and Giri, B. (2009). Arbuscular mycorrhizal fungi in alleviation of salt stress: a review. Ann. Bot. 104, 1263–1280. doi: 10.1093/aob/mcp251
FAOSTAT (2015). Food and Agriculture Organization of the United Nations. Available online at: http://faostat3.fao.org/download/Q/QC/E (Accessed May, 2015).
Farquhar, G. D., Ehleringer, J. R., and Hubick, K. T. (1989). Carboon isotope discrimination and photosynthesis. Ann. Rev. Plant Physiol. Plant Mol. Biol. 40, 503–537. doi: 10.1146/annurev.pp.40.060189.002443
Franks, P. J., and Farquhar, G. D. (2001). The effect of exogenous abscisic acid on stomatal development, stomatal mechanics, and leaf gas exchange in Tradescantia virginiana. Plant Physiol. 125, 935–942. doi: 10.1104/pp.125.2.935
Franks, P. J., and Farquhar, G. D. (2007). The mechanical diversity of stomata and its significance in gas-exchange control. Plant Physiol. 143, 78–87. doi: 10.1104/pp.106.089367
Frosi, G., Barros, V. A., Oliveira, M. T., Santos, M., Ramos, D. G., Maia, L. C., et al. (2018). Arbuscular mycorrhizal fungi and foliar phosphorus inorganic supply alleviate salt stress effects in physiological attributes but only arbuscular mycorrhizal fungi increased biomass in woody species of a semiarid environment. Tree Physiol. 38, 25–36. doi: 10.1093/treephys/tpx105
Gamage, D., Thompson, M., Sutherland, M., Hirotsu, N., Makino, A., and Seneweera, S. (2018). New sights into the cellular mechanisms of plant growth at elevated atmospheric carbon dioxide concentrations. Plant Cell Environ. 41, 1233–1246. doi: 10.1111/pce.13206
Geissler, N., Hussin, S., and Koyro, H. W. (2009). Elevated atmospheric CO2 concentration ameliorates effects of NaCl salinity on photosynthesis and leaf structure of Aster tripolium L. J. Exp. Bot. 60, 137–151. doi: 10.1093/jxb/ern271
Goicoechea, N., Baslam, M., Erice, G., and Irigoyen, J. J. (2014). Increased photosynthetic acclimation in alfalfa associated with arbuscular mycorrhizal fungi (AMF) and cultivated in greenhouse under elevated CO2. J. Plant Physiol. 171, 1774–1781. doi: 10.1016/j.jplph.2014.07.027
Hetherington, A. M., and Woodward, F. I. (2003). The role of stomata in sensing and driving environmental change. Nature 424, 901–908. doi: 10.1038/nature01843
Huang, B., and Xu, Y. (2015). Cellular and molecular mechanisms for elevated CO2-regulation of plant growth and stress adaptation. Crop Sci. 55, 1–20. doi: 10.2135/cropsci2014.07.0508
Kapoor, R., Evelin, H., Mathur, P., and Giri, B. (2013). “Arbuscular mycorrhiza: approaches for abiotic stress tolerance in crop plants for sustainable agriculture,” in Plant Acclimation to Environmental Stress, eds N. Tuteja, and S. S. Gill (New York, NY: Springer), 359–401.
Kumar, S., Chaitanya, B. S. K., Ghatty, S., and Reddy, A. R. (2014). Growth, reproductive phenology and yield responses of a potential biofuel plant, Jatropha curcas grown under projected 2050 levels of elevated CO2. Physiol. Plant. 152, 501–519. doi: 10.1111/ppl.12195
Lammertsma, E. I., de Boer, H. J., Dekker, S. C., Dilcher, D. L., Lotter, A. F., and Wagner-Cremer, F. (2011). Global CO2 rise leads to reduced maximum stomatal conductance in Florida vegetation. PNAS 108, 4035–4040. doi: 10.1073/pnas.1100371108
Lawson, T. (2009). Guard cell photosynthesis and stomatal function. New Phytol. 181, 13–34. doi: 10.1111/j.1469-8137.2008.02685.x
Lawson, T., and Blatt, M. R. (2014). Stomatal size, speed, and responsiveness impact on photosynthesis and water use efficiency. Plant Physiol. 164, 1556–1570. doi: 10.1104/pp.114.237107
McElwain, J. C., Yiotis, C., and Lawson, T. (2016). Using modern plant trait relationships between observed and theoretical maximum stomatal conductance and vein density to examine patterns of plant macroevolution. New Phytol. 209, 94–103. doi: 10.1111/nph.13579
Murray, R. R., Emblow, M. S. M., Hetherington, A. M., and Foster, G. D. (2016). Plant virus infections control stomatal development. Sci. Rep. 6:34507. doi: 10.1038/srep34507
NOAA-ESRL (2018). National Oceanic and Atmospheric Administration-Earth System Research Laboratory. Atmospheric CO2 for May, 2018.
Pérez-López, U., Mena-Petite, A., and Mu-oz-Rueda, A. (2012a). “Interaction between salinity and elevated CO2: a physiological approach,” in Progress in Botany, Vol 73, Part III: Physiology, eds U. Lüttge, W. Beyschlag, B. Büdel, and D. Francis (Heidelberg: Springer), 97–126.
Pérez-López, U., Mena-Petite, A., and Mu-oz-Rueda, A. (2014). Will carbon isotope discrimination be useful as a tool for analyzing the dunctional response of barley plants to salinity under the future atmospheric CO2 conditions? Plant Sci. 226, 71–81. doi: 10.1016/j.plantsci.2014.05.011
Pérez-López, U., Robredo, A., Lacuesta, M., Mena-Petite, A., and Mu-oz-Rueda, A. (2012b). Elevated CO2 reduces stomatal and metabolic limitations on photosynthesis caused by salinity in Hordeum vulgare. Photosyn. Res. 111, 269–283. doi: 10.1007/s11120-012-9721-1
Piñero, M. C., Houdusse, F., Garcia-Mina, J. M., Garnica, M., and del Amor, F. M. (2014). Regulation of hormonal responses of sweet pepper as affected by salinity and elevated CO2 concentration. Physiol. Plant. 151, 375–389. doi: 10.1111/ppl.12119
Ruiz-Lozano, J. M., and Aroca, R. (2010). “Host response to osmotic stresses: stomatal behaviour and water use efficiency of arbuscular mycorrhizal plants,” in Arbuscular mycorrhizas: Physiology and Function, eds H Koltai and Y Kapulnik (Springer), 239–256. doi: 10.1007/978-90-481-9489-6_11
Sekiya, N., and Yano, K. (2008). Stomatal density of cowpea correlates with carbon isotope discrimination in different phosphorus, water and CO2 environments. New Phytol. 179, 799–807. doi: 10.1111/j.1469-8137.2008.02518.x
Sheng, M., Tang, M., Chen, H., Yang, B. W., Zhang, F. F., and Huang, Y. H. (2008). Influence of arbuscular mycorrhizae on photosynthesis and water status of maize plants under salt stress. Mycorrhiza 18, 287–296. doi: 10.1007/s00572-008-0180-7
Sun, Y., Yan, F., Cui, X., and Liu, F. (2014). Plasticity in stomatal size and density of potato leaves under different irrigation and phosphorus regimes. J. Plant Physiol.. 171, 1248–1255. doi: 10.1016/j.jplph.2014.06.002
Syvertsen, J. P., and Graham, J. H. (1999). Phosphorus supply and arbuscular mycorrhizas increase growth and net gas exchange responses of two Citrus spp. grown at elevated [CO2]. Plant Soil 208, 209–219. doi: 10.1023/A:1004553315041
Yan, F., Sun, Y., Song, F. B., and Liu, F. L. (2012). Differential responses of stomatal morphology to partial root-zone drying and deficit irrigation in potato leaves under varied nitrogen rates. Sci. Hortic. 145, 76–83. doi: 10.1016/j.scienta.2012.07.026
Yan, W., Zhong, Y., and Shangguan, Z. (2017). Contrasting responses of leaf stomatal characteristics to climate change: a considerable challenge to predict carbon and water cycles. Global Change Biol. 23, 3781–3793. doi: 10.1111/gcb.13654
Yu, J. J., Sun, L. H., Fan, N. L., Yang, Z. M., and Huang, B. R. (2015). Physiological factors involved in positive effects of elevated carbon dioxide concentration on Bermudagrass tolerance to salinity stress. Environ. Exp. Bot. 115, 20–27. doi: 10.1016/j.envexpbot.2015.02.003
Zaghdoud, C., Mota-Cadenas, C., Carvajal, M., Muries, B., Ferchichi, A., and Martínez-Ballesta, M. C. (2013). Elevated CO2 alleviates negative effects of salinity on broccoli (Brassica oleracea L. var Italica) plants by modulating water balance through aquaporins abundance. Envrion. Exp. Bot. 95, 15–24. doi: 10.1016/j.envexpbot.2013.07.003
Zhu, X. C., Song, F. B., Liu, S. Q., and Liu, F. L. (2016a). Arbuscular mycorrhiza improve growth, nitrogen uptake, and nitrogen use efficiency in wheat grown under elevated CO2. Mycorrhiza 26, 133–140. doi: 10.1007/s00572-015-0654-3
Keywords: carbon isotope discrimination, stomatal aperture, stomatal conductance, stomatal density, water potential
Citation: Zhu X, Cao Q, Sun L, Yang X, Yang W and Zhang H (2018) Stomatal Conductance and Morphology of Arbuscular Mycorrhizal Wheat Plants Response to Elevated CO2 and NaCl Stress. Front. Plant Sci. 9:1363. doi: 10.3389/fpls.2018.01363
Received: 27 June 2018; Accepted: 28 August 2018;
Published: 19 September 2018.
Edited by:
Benoit Schoefs, University of Maine, FranceReviewed by:
Raffaella Balestrini, Consiglio Nazionale Delle Ricerche (CNR), ItalyCopyright © 2018 Zhu, Cao, Sun, Yang, Yang and Zhang. This is an open-access article distributed under the terms of the Creative Commons Attribution License (CC BY). The use, distribution or reproduction in other forums is permitted, provided the original author(s) and the copyright owner(s) are credited and that the original publication in this journal is cited, in accordance with accepted academic practice. No use, distribution or reproduction is permitted which does not comply with these terms.
*Correspondence: Xiancan Zhu, emh1eGlhbmNhbkBpZ2EuYWMuY24=
Disclaimer: All claims expressed in this article are solely those of the authors and do not necessarily represent those of their affiliated organizations, or those of the publisher, the editors and the reviewers. Any product that may be evaluated in this article or claim that may be made by its manufacturer is not guaranteed or endorsed by the publisher.
Research integrity at Frontiers
Learn more about the work of our research integrity team to safeguard the quality of each article we publish.